ABSTRACT
The cell membrane forms a fundamental part of all living cells and participates in a variety of physiological processes, such as material exchange, stress response, cell recognition, signal transduction, cellular immunity, apoptosis, and pathogenicity. Here, we review the mechanisms and functions of the membrane structure (lipid components of the membrane and the biosynthesis of unsaturated fatty acids), membrane proteins (transmembrane proteins and proteins contributing to membrane curvature), transcriptional regulation, and cell wall components that influence the virulence and pathogenicity of filamentous fungi.
Introduction
The cell membrane not only determines the cell shape but also affects various cellular activities that are influenced by membrane homeostasis in terms of the structural and functional integrity and fluidity of the membrane [Citation1,Citation2]. Cell membranes are found in all living organisms [Citation3] and are composed largely of lipids and proteins, with an amphiphilic lipid bilayer forming the overall framework of the membrane [Citation4,Citation5]. The cell membrane is responsible for both the physical and structural integrity of the cell [Citation6].
Filamentous fungi undergo a complex development that includes conidial germination, polarized morphogenesis, and the differentiation of sexual and asexual structures [Citation7,Citation8]. The cell membrane represents a key barrier for resting conidia surviving in nature, and any damage to the membrane will lead to a loss of conidial viability [Citation9]. Fungal cell membranes are responsible for the early recognition of nutrient availability, impending stress, or pathogen attack [Citation10]. Although the mechanisms controlling cell membrane domains and the functional consequences of this organization, including membrane composition and the structure and functions of membrane components, have been reviewed in yeast [Citation11], there has been little discussion of the role of cell membrane homeostasis in filamentous fungi. The overall influence of the morphology, integrity, permeability, and homeostasis of cell membranes on the growth and virulence of pathogenic filamentous fungi has not been discussed and, to date, there is no review of how cell membrane homeostasis affects the pathogenicity of these fungi.
Here, we summarize the literatures on fungal cell membrane homeostasis and its roles in fungal pathogenicity by discussing the roles of the fungal transmembrane protein-lipid microdomains, cell wall-lipid microdomains, cytoskeleton-transmembrane protein microdomains, and membrane curvature.
Effect of membrane structure on fungal pathogenicity
Membrane lipid components and their biological roles
The rich and diverse lipid composition of the cell membrane affects the shape and structure of the membrane. There tends to be greater lipid diversity in eukaryotic membranes, indicating the complex role of membrane lipids in various cell functions [Citation12]. Cell membrane lipids are usually arranged in bilayers and can participate directly in various cellular events as messengers or regulators of signal transduction [Citation13,Citation14]. Certain lipids, such as phosphatidylserine [Citation15], phosphatidylcholine [Citation16], ergosterol [Citation17], C8-desaturated and C9-methylated glycosylceramide (GlcCer) [Citation18], and ceramide [Citation19] tend to accumulate in specific regions (domains) of the membrane forming membrane/lipid rafts (MLRs) [Citation20], which have evolutionarily conserved roles in the integrity and repair of the cell membrane.
Membrane lipids also play important roles in the regulation of asexual spore germination and mycelial growth. In Pestalotiopsis microspora, the absence of the choC or choA genes affects the conversion of phosphatidyl ethanolamine to phosphatidylcholine, which impedes the production of fungal spores or conidia and destroys the cell wall integrity [Citation16]. Similarly, several choline precursors of membrane lipids also play important roles in the morphology of Aspergillus nidulans mycelia [Citation21]. Ergosterol directly affects the growth and virulence of fungi and in treating fungal diseases caused by infection with Aspergillus fumigatus [Citation17]. In Aspergillus nidulans, C8-desaturated and C9-methylated glycosylceramides (GlcCer), fungal-specific sphingolipids, influence both growth and virulence of the fungi [Citation18]. Acyl-CoA-dependent ceramide synthetase (BarA) regulates cytoskeletal organization and mycelial morphogenesis by controlling ceramide synthesis to form specialized lipid microdomains [Citation19]. In addition, some lipid-associated domains can recruit specific proteins regulating membrane-protein participation in important cellular functions such as signal transduction [Citation13]. For example, spectrin, which forms filamentous networks on the cytoplasmic surface of the fungal cell membrane, participates in the structural integrity of the membrane and controls the lateral mobility of membrane proteins [Citation22].
Membrane lipids also make important contributions to biological processes such as vegetative growth, morphological development, and host infection of pathogenic filamentous fungi [Citation23,Citation24]. Cell membrane homeostasis is not only closely related to membrane lipid but also depends on membrane proteins which thus modulate fungal pathogenicity. For example, the targeting of Scp2 by peroxisomes is essential for maintaining the virulent function of Ustilago maydis. Such conserved eukaryotic proteins can ensure the correct distribution of peroxisomes by fine-tuning the lipid composition of the membrane to maintain biotrophy during pathogen infection [Citation25]. In Magnaporthe oryzae, MoVps13 regulates fungal pathogenicity by contributing to impaired plasma membrane homeostasis through sphingolipid synthesis [Citation26]. In addition, the lipid bilayer plays a central role in the physicochemical environment and shape of the membrane by influencing the arrangement and function of membrane proteins [Citation27]. Lipids, especially membrane lipids, are important for the integrity of the cytoplasmic membrane. In addition, many fungal membrane lipid components, such as sphingolipids, ergosterol, and phospholipids, participate in signalling pathways, which regulate the integrity of fungal cell walls [Citation28]. Therefore, the lipid composition is a major factor influencing the homeostasis of the cell membrane and, in turn, the pathogenicity of filamentous fungi.
Biosynthesis of unsaturated fatty acid
Studies have shown that UFAs (Unsaturated Fatty Acids) contribute to cell membrane integrity and thus influence the ability of cells to respond to various environmental stresses such as that induced by high salt [Citation29]. The fatty acid synthetase β-subunit dehydrogenase (FAS1) enzyme in Magnaporthe oryzae is involved in the process of lipid synthesis, which may allow expansion for the oppressor to regulate the processes of fungal germination and host penetration [Citation30]. In addition, in the pathogenic filamentous fungus M. oryzae, the hydrolysis of stored lipids to fatty acids and glycerol facilitates conidial germination, which is critical for fungal virulence [Citation31]. In conclusion, the length and degree of saturation of fatty acid chains can provide reserve energy for pathogenic filamentous fungi by allowing greater signalling diversity on the one hand [Citation32–34] while, on the other hand, UFAs provide turgor pressure for fungal growth, germ tube germination, and spore maturation, allowing the spore to contribute to the early stages of infection.
The proportion of unsaturated fatty acids (UFAs) in the membrane is a major determinant of membrane integrity and permeability in fungi, affecting both membrane homeostasis and pathogenicity. Higher proportions of UFAs increase the fluidity of the membrane, affecting the rotation and movement of functional proteins [Citation35]. Most fungi contain both saturated (such as palmitic acid and stearic acid) and unsaturated (such as oleic acid, linoleic acid, and linolenic acid) fatty acids. The product of the delta 9-fatty acid desaturase gene (Ole1) introduces a double bond into saturated acyl-CoA to form a monounsaturated fatty acid [Citation36,Citation37]. The anabolic fatty acid biosynthesis pathway is conserved in filamentous fungi [Citation38]. For example, the Ole1 enzyme participates in the synthesis of ultra-long fatty acid chains and plays a crucial role in cytoplasmic membrane homeostasis [Citation39,Citation40].
The expression of Ole1 is regulated by upstream transcription factors, such as the ER membrane proteins MGA2/SPT23 (yeast) [Citation39,Citation41,Citation42] and HapX (Beauveria bassiana) [Citation43], amongst others. In Aspergillus flavus, FarA (a Zn2-Cys6 transcription factor) positively regulates the expression of stearic acid desaturase genes required for oleic acid synthesis [Citation44]. In addition, Ubx2p is a key upstream regulator of the essential fatty acid desaturase Ole1p. The absence of Ubx2p affects the transcription of Ole1, causing fatty acid desaturation and abnormal nuclear membrane morphology [Citation45]. In Aspergillus giganteus, 2-hydroxyfat n-acyl-δ3(E)-desaturase controls the level of saturation of fatty acid chains and interferes with cytoplasmic membrane integrity [Citation46]. The absence of BbSAY1 decreases the contents of oleic acid and membrane permeability, leading to a significant reduction in the fungal virulence of Bassiana bassiana [Citation47].
Effect of transmembrane proteins on cell membrane homeostasis in pathogenic filamentous fungi
Transmembrane proteins and other essential components
Fungal transmembrane proteins, such as G protein-coupled receptors (GPCRs), glycosylphosphatidylinositol-anchored proteins (GPI-APs), GTPases, andCan1 membrane compartment as arginine/H+ transporters (MCCs), form complex domains within the cell membrane, helping to regulate various physiological functions of the membrane [Citation48,Citation49]. Magnaporthe grisea GPCRs (PTH11) was shown to be an integral membrane protein required for pathogenicity [Citation50]. GPCRs with the CFEM (common in fungal extracellular membrane) domain have also been shown to be important membrane proteins regulating fungal development and/or pathogenicity in entomopathogenic fungi, such as Beauveria bassiana [Citation47] and Metarhizium Robertsii [Citation51]. These proteins regulate membrane transport through interactions with lipid and cell wall components, as well as with intracellular cytoskeletal components, to maintain the homeostasis of the cell membrane.
Transmembrane proteins are present in almost all cells and they play important roles in development, cell adhesion, cell signalling, and development, as well as in the infection and evasion of the host defence in fungi [Citation52]. The cell membrane, as a selective osmotic barrier for living cells, is essential for cell survival and function [Citation53]. Transmembrane proteins are responsible for maintaining normal concentrations of various substances inside and outside the membrane, thus ensuring normal membrane functioning. GPCRs are known to be involved in a wide range of membrane transport activities and are capable of initiating or modulating signal transduction at multiple membrane sites [Citation54]. GPI-APs are attached to the outer surface of the cell membrane through the GPI post-translational modification and play important roles in cell signalling, cell adhesion, cell wall metabolism, the immune response, and cell membrane homeostasis [Citation55]. Proteins of the FgDnf gene family mediate transmembrane transport of substances and are involved in vesicle budding, charge distribution, and intramembrane protein diffusion, and are critical for vegetative growth, development, and pathogenicity in Fusarium graminearum [Citation56].
Various fungal membrane proteins interact with chitin, lipids, and actin, and are widely involved in the transport of different substances, thus playing important roles in cell function and structure.Fungal transmembrane proteins can form dynamic and stable structures with the cytoskeleton to maintain the homeostasis of the cell membrane. In fungi, two principal mechanisms, namely, turgor and the actin cytoskeleton, regulate cell membrane tension (PMTS) that modulates the connection between cell membrane and the cell wall. These systems function together with the cell membrane to facilitate adhesion to the cell wall. Depolymerization of F-actin leads to the rapid formation of endocytic pits while preventing their separation from the cell membrane [Citation57]. Actin filaments can be organized into a variety of structures that are capable of exerting or resisting mechanical forces in the cellular environment [Citation58]. In Aspergillus fumigatus, GTPases combine with septin (AspA-E), a cytoskeletal component, to regulate chitin deposition, separation, cytokinesis, and sporulation of the fungal cell wall, thus contributing to host invasion and virulence in mycogenic fungi [Citation59,Citation60].
The cytoskeleton not only helps stabilize the structure of the cell through the cell membrane but also regulates mycelium and spore formation to a certain extent. Phosphatidylinositol-(4,5)-bisphosphate (PI[4,5]P2) and septin in Candida albicans regulate the caspofungin response and the development of fungal mycelia in early fungal stages [Citation61,Citation62]. Septin also colocalizes with sterol-rich regions in the membrane and promotes the recruitment of cell-wall synthases during cell-wall remodelling [Citation63]. In addition, a protein kinase associated with septin ring assembly, Gin4, is an important regulator of fungal cell membrane asymmetry [Citation64]. Fungal membrane proteins maintain homeostasis of the cell membrane by forming dynamic and stable structures within the cell wall. The cell walls of filamentous fungi are mainly composed of chitin and glucan. Fungal chitin synthetases form a large protein family in filamentous fungi. These proteins contain transmembrane domains and are transported to the cell membrane by secretory vesicles where they participate in cell-wall synthesis [Citation65]. In Trichoderma atroviride, the LysM domain protein TAL6 binds to certain forms of polymerized chitin, protecting the fungus from plant chitinases [Citation66]. Furthermore, Sur7, one of the many proteins that constitute the MCC, is involved in membrane-related cellular events and regulates fungal spore formation, cell wall integrity, stress tolerance, and virulence [Citation67]. These data suggest that transmembrane proteins assist in cell wall synthesis through the transportation of cell wall components. Therefore, fungal membrane proteins may play crucial roles in cell wall homeostasis.
Membrane curvature
Membrane curvature is a major factor essential for cell growth, division, and movement; and curvature and curvature-sensing proteins help vesicle budding and vesicle transport [Citation68]. The shape of the cell membrane is a conserved phenotypic feature. The membrane shape/curvature has a significant effect on the biological function of the cell [Citation69]. Certain intracellular proteins can bend lipid bilayers to generate the bent membrane required for substrate transport [Citation70]. The cell membrane acts as a flexible barrier surrounding the cell and its compartments and its shape changes during cell locomotion and on receptor binding. Proteins are inserted into the membrane in a wedge-like manner, thus altering the curvature of the membrane [Citation68]. For example, BAR-domain proteins assist in the recruitment of other proteins to specific locations where the membrane needs to be bent [Citation71].
In fungi, membrane curvature is closely associated with both the cytoskeleton and the lipid content. Transmission electron microscopy of high-pressure frozen sections was used to evaluate the membrane structure during fungal infection. This showed that host cells infected by powdery mildew fungi form a haustorium in the outer membrane that is thicker than other cell membranes and is highly coiled, especially near the distal end of the haustorium neck [Citation72]. Septin plays an important role as it promotes changes in the fungal cell shape and organizes the F-actin cytoskeleton to promote aggressive growth [Citation73–75]. Septins in Magnaporthe grisea support F-actin via the ezrin-radixin-moesin protein Tea1 and interact with the phosphatidyl side of the appressorium membrane, providing cortical rigidity and membrane curvature to the appressorium. The fungi physically destroy the cuticle of the leaf to enter the plant tissue [Citation76]. Studies in mice demonstrate that the E2-like enzyme, Atg3, facilitates LC3/GABARAP lipidation only on membranes exhibiting local lipid-packing defects. Atg3 is designed to work at highly curved membranes, perhaps including the limiting edge of the growing phagophore [Citation77]. Among filamentous fungi, there are few reports on ATG3. Beauveria bassiana ATG3 is phosphorylated by ATG1 to participate in the autophagy process of fungi, thus regulating the development and pathogenicity of fungi [Citation78]. Studies in human cells have shown that membrane curvature is an important parameter for defining the morphology of cells, organelles, and local membrane subdomains. The generation and maintenance of curvature is essential for maintaining traffic and cellular function. In addition to providing cell or organelle shape, local curvature can also affect processes such as membrane division and fusion, as well as protein concentrations and enzyme activation on the membrane [Citation79]. In pathogenic filamentous fungi, the curvature of the cytoplasmic membrane not only promotes the formation of mycelia but also affects growth and spore formation. Overall, the curvature of the cell membrane contributes to fungal virulence ().
Figure 1. Formation of membrane curvature in pathogenic filamentous fungi. A. Morphology of fungi during early spore germination. B. The dividing or germinating germ tube in yeast cells infected with pathogenic fungus. C. Hypha of pathogenic filamentous fungi. The red boxes indicate the positions of potential membrane curvature at different developmental stages. D. Factors contributing to the formation of membrane curvature. a. Induction of curvature in the cell membrane by the insertion of mosaic membrane proteins. b. Effects of membrane curvature on the combination of polar fatty acid chains and various lipid components. c. Membrane bending caused by interaction between membrane proteins and cytoskeletal proteins.
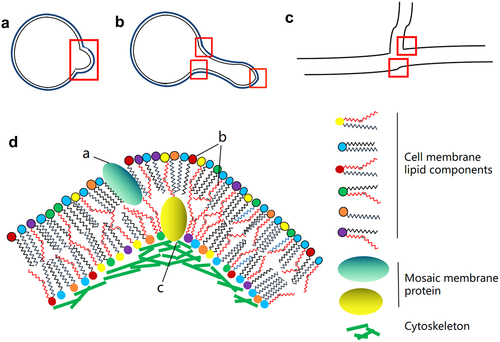
Cell wall components, regulation and modifications affecting cell membrane homeostasis in pathogenic filamentous fungi
Cell growth, membrane tension, and cell wall synthesis are interdependent. The interplay between membrane tension and cell wall mechanical stress enables cells to rapidly rectify mismatches between the rates of the membrane and cell wall synthesis during cell growth, thus ensuring balanced and normal growth [Citation80]. Cell walls isolate cells from their surroundings and protect them from environmental stressors. Cell wall integrity is maintained by highly regulated processes of cell wall biosynthesis [Citation81]. Maintenance of mutual structural integrity between the cell wall and membrane is one of the important conditions for cell wall homeostasis. Studies in Candida albicans showed that cell growth is regulated by two powerful complementary inducible forces, where membrane tension prevents cell wall expansion by inhibiting the synthesis of peptidoglycans, major components of the cells wall, while mechanical stress within the cell wall promotes the irreversible expansion of the cell wall. Only optimal membrane tension and cell wall stress ensure the balance between cell membrane and cell wall synthesis [Citation80]. The sensor of cell wall mechanical stress, Wsc1 (MCW), participates in the regulation of homeostasis between the cell membrane and cells wall, as well as the apical growth of fungal cells and the protection of membrane domains under conditions of stress [Citation82].
The components of the fungal cell wall provide mechanical protection against damage to the cell membrane. Filamentous fungi regulate the growth of both the cell wall and membrane as well as pathogenicity through endocytosis and exocytosis [Citation83]. In Beauveria bassiana, BbLec1, which binds to chitosan and chitin in fungal cell walls, plays a wide range of physiological roles including defence responses and host-pathogen interactions [Citation84]. A loss of Bbmcm1 results in impaired cell integrity, distorted cell wall structure, altered cell wall composition, and delayed pathogenicity in insects [Citation85]. MoVps13, an important virulence factor in Magnaporthe oryzae, plays an important role in cell wall integrity and cell membrane homeostasis by participating in cell wall and sphingolipid synthesis [Citation26]. Hex1 regulates osmotic stress and modulates the integrity of the cell wall and membrane, and has been shown to influence the colonization and pathogenicity of Verticillium dahliae [Citation86]. Chitosan can inhibit conidial germination by destroying the integrity of the conidial membrane [Citation87]. In Fusarium species, target of rapamycin (TOR), calcium/calcineurin, and cell wall integrity pathways are important in responses to environmental signals [Citation88].
Fungal cell wall components also affect the tension of the cell membrane and the mechanical tension of the cell wall, providing suitable turgor pressure for fungal germination and even host infection. In Metarhizium acridum, the absence of MaPmt1 makes fungi more sensitive to cell wall disruptors, with the cell wall becoming thinner with changes in its composition, thus reducing the pressure resulting from appressorium dilation and an inability to maintain the normal appressorium dilation pressure through the host cuticle [Citation89].
Transcriptional regulation of cell membrane and cell wall homeostasis in pathogenic filamentous fungi
Filamentous pathogenic fungi are widely distributed in nature and infect diverse host species, including higher animals, plants, and lower animals, necessitating extensive interactions between the fungus and the host [Citation90]. Cell membrane homeostasis is closely related to the pathogenicity of fungi in both plant and animal hosts. In filamentous fungi, the involvement of transcriptional regulation of cell membrane homeostasis in fungal pathogenicity is rarely reported. For example, Beauveria bassiana HapX is an indispensable bZIP transcription factor in iron acquisition. It plays an important role in the early stage of fungal infection by regulating conidial oleic acid homeostasis and cell membrane function via initiation downstream gene BbOle1 [Citation84]. In Aspergillus fumigatus, ΔhacA mutants have increased sensitivity to antifungal agents that damage the membrane or cell wall, and decreased toxicity [Citation91].
There have been many reports of involvement in fungal pathogenicity through regulation of cell wall component synthesis or cell wall integrity. Garcia-Rubio et al. reviewed recent studies on transcriptional regulation of the synthesis and integrity of cell wall components on fungal growth and development and pathogenicity in Candida, Cryptococcus, and Aspergillus Species [Citation92]. In addition, there have been some reports of other filamentous pathogenic fungi. Such as, the loss of Bbpmr1 resulted in a sharp decrease in the tolerance of B. bassiana to cell wall stressors and a loss of half of the virulence of the fungus [Citation93]. Rho2 regulates the response of Fusarium oxysporum to inhibitors of cell wall synthesis [Citation94]. The transcription factors Con7p, Con7–1 and two identical Con7–2 encoded by F. oxysporum genes play an important role in the pathogenesis of fungi by regulating cell wall biogenesis [Citation95]. ChSat4 plays an important role in asexual development, cell wall integrity maintenance and pathogenicity of Colletotrichum higginsianum [Citation96].
The cell membrane also requires the homeostasis of other membrane-enclosed organelles
Several works show structurally and functionally dynamic contacts between mitochondria, the plasma membrane, the endoplasmic reticulum, and other subcellular organelles [Citation97]. The cell membrane is a complex system that also requires the homeostasis of other membrane-enclosed organelles such as the endoplasmic reticulum [Citation98,Citation99], mitochondrion [Citation100], and Golgi apparatus, as well as vacuolar membranes and the vesicular membrane system [Citation101]. Endoplasmic reticulum homeostasis is very important for the growth and virulence of Aspergillus fumigatus [Citation91]. In B. bassiana, vacuoles, as one of the membranous structures of cells, have also been shown to be involved in maintaining fungal pathogenicity [Citation102]. Peroxisome genes (AoPEX1 and AoPEX6) Are Required for Mycelial Growth, Conidiation, Stress Response, Fatty Acid Utilization, and Trap Formation in Arthrobotrys oligospora [Citation103]. The succinate dehydrogenase gene BbSdhC1 is critical for energy supply in the electron transport chain (ETC) by mediating the flow of electrons along the mitochondrial membrane, and disruption of this gene significantly reduces the virulence of the B. bassiana against the insect host [Citation104].
However, here, we have only focused on the homeostasis of the cell membrane and its influence on fungal pathogenicity. In terms of composition, membrane lipids and transmembrane proteins are indispensable factors determining the complexity and diversity of all cellular organelles and membrane systems. While the contributions of transmembrane proteins and lipid components to cell membrane homeostasis have been widely reported, it is, however, not clear how membrane microdomains formed by transmembrane proteins and various lipid components contribute to cell membrane homeostasis and pathogenicity in fungi.
A further important factor affecting the homeostasis of the fungal cell membrane [Citation105] is membrane curvature. Transmembrane proteins, the cytoskeleton, and microdomains formed by proteins all contribute to the degree of membrane curvature, which in turn affects the dimorphic transformation of filamentous fungi and the formation of invasive organs (such as appressoria) that contribute significantly to fungal virulence. However, most research on these topics has been performed by using biophysical simulation, with only a small amount of research focused on the mechanisms of host disease induction due to changes in the membrane curvature of fungi. There are still many unanswered questions surrounding pathogenic filamentous fungi. For instance, how do transmembrane proteins, membrane lipids, cell walls, and cytoskeletal components regulate interactions between the cell membrane and the cytoskeleton? How are the cell membrane and cell wall involved in conidial or blastospore (yeast cell) conversion to filamentous cells? How do they mediate fungal infection of the host? Nonetheless, unsaturated non-polar fatty acids also play important roles in determining fungal membrane curvature. Several genes suggested to influence the curvature of the fungal cell membrane have also been reported [Citation106]. The effect of membrane curvature on the homeostasis and pathogenicity of fungal cytoplasmic membranes could be a promising research direction. In addition, the actin cytoskeleton exerts mechanical forces on the membrane and thereby regulates various cellular and subcellular morphogenetic processes such as cell migration, cytoplasmic division, and membrane transport [Citation107]. This suggests that the cytoskeleton determines the homeostasis of fungal cell membranes. In all, the microdomains formed between membrane proteins, the cytoskeleton, the cell wall, and membrane lipid components are critical for the homeostasis of the cell membrane and the pathogenicity of fungal cells.
In conclusion, the contribution of cell membrane homeostasis to the pathogenicity of filamentous fungi is direct or indirect (, ). Membrane homeostasis is composed of membrane integrity, fluidity and permeability, and is closely related to membrane structure (membrane lipid type and saturation degree of non-polar fatty acid chains), transmembrane proteins and their transcriptional regulatory networks, membrane curvature, cell wall composition and cytoskeleton. These components and regulatory processes contribute to fungal virulence either by directly participating in the virulence of pathogenic fungi, or by regulating cell growth and development, and even stress.
Figure 2. Schematic diagram of the effects of cell membrane homeostasis on the growth and development of pathogenic filamentous fungi. This involves interactions between membrane lipid components, transmembrane proteins, cytoskeletal, and cell wall components, as well as the formation of membrane curvature, to maintain the integrity of the cell membrane assembly, structure, and function in pathogenic fungi at various stages of cell development, thus maintaining homeostasis of the cell membrane and contributing to fungal virulence. The blue and green notes respectively represent cell membrane homeostasis involved in the activity of filamentous fungi at the cytological level and microbial onto developmental level.
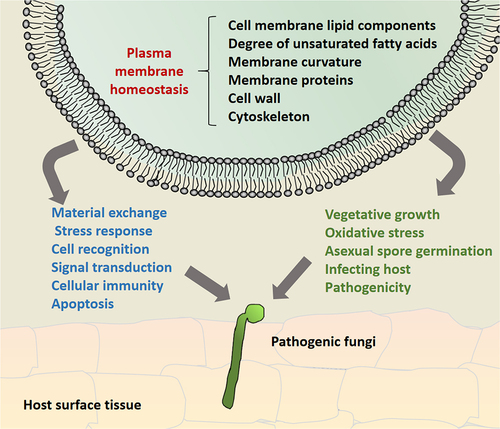
Table 1. Summarizes the substances involved in cell membrane homeostasis of filamentous fungi.
Acknowledgements
This work was financially supported by the Natural Science Foundation of Yunnan Province (202301AT070487), Scientific Research Fund Project of Education Department of Yunnan Province (2023Y0902), the Major Science and Technology Project of Yunnan and Kunming (202202AE090036), the Science and Technology Project of Yunnan provincial tobacco company (NO.2022530000241019), and Yunnan provincial Municipal Education Commission Innovation Team Project (2022[69]) grants.
Disclosure statement
No potential conflict of interest was reported by the author(s).
Data availability statement
Data availability is not applicable to this article as no new data were created or analysed in this study.
Correction Statement
This article has been republished with minor changes. These changes do not impact the academic content of the article.
Additional information
Funding
References
- Yadav DK, Kumar S, Choi EH, et al. Molecular dynamic simulations of oxidized skin lipid bilayer and permeability of reactive oxygen species. Sci Rep. 2019;9(1):4496. doi: 10.1038/s41598-019-40913-y
- Mychack A, Janakiraman A, Brun YV. Defects in the first step of lipoprotein maturation underlie the synthetic lethality of Escherichia coli lacking the inner membrane proteins YciB and DcrB. J Bacteriol. 2021;203(6). doi: 10.1128/JB.00640-20
- van Meer G, Voelker DR, Feigenson GW. Membrane lipids: where they are and how they behave. Nat Rev Mol Cell Biol. 2008;9(2):112–11. doi: 10.1038/nrm2330
- Nicolson GL. The fluid-mosaic model of membrane structure: still relevant to understanding the structure, function and dynamics of biological membranes after more than 40 years. Biochim Biophys Acta. 2014;1838:1451–1466. doi: 10.1016/j.bbamem.2013.10.019
- Sonnino S, Prinetti A. Membrane domains and the “lipid raft” concept. Curr Med Chem. 2013;20:4–21. doi: 10.2174/0929867311320010003
- Horn A, Jaiswal JK. Structural and signaling role of lipids in plasma membrane repair. Curr Top Membr. 2019;84:67–98.
- Baltussen T, Zoll J, Verweij PE, et al. Molecular mechanisms of conidial germination in aspergillus spp. Microbiol Mol Biol Rev. 2020;84(1). doi: 10.1128/MMBR.00049-19
- Fortwendel JR. Orchestration of morphogenesis in filamentous fungi: conserved roles for ras signaling networks. Fungal Biol Rev. 2015;29(2):54–62. doi: 10.1016/j.fbr.2015.04.003
- Cerioni L, Volentini SI, Prado FE, et al. Cellular damage induced by a sequential oxidative treatment on penicillium digitatum. J Appl Microbiol. 2010;109(4):1441–1449. doi: 10.1111/j.1365-2672.2010.04775.x
- Malinsky J, Opekarova M. New insight into the roles of membrane microdomains in physiological activities of fungal cells. Int Rev Cell Mol Biol. 2016;325:119–180.
- Ziolkowska NE, Christiano R, Walther TC. Organized living: formation mechanisms and functions of plasma membrane domains in yeast. Trends Cell Biol. 2012;22(3):151–158. doi: 10.1016/j.tcb.2011.12.002
- Harayama T, Riezman H. Understanding the diversity of membrane lipid composition. Nat Rev Mol Cell Biol. 2018;19(5):281–296. doi: 10.1038/nrm.2017.138
- Epand RM. Introduction to membrane lipids. Methods Mol Biol. 2015;1232:1–6.
- Escriba PV, Busquets X, Inokuchi J, et al. Membrane lipid therapy: modulation of the cell membrane composition and structure as a molecular base for drug discovery and new disease treatment. Prog Lipid Res. 2015;59:38–53. doi: 10.1016/j.plipres.2015.04.003
- Thomas FB, Omnus DJ, Bader JM, et al. Tricalbin proteins regulate plasma membrane phospholipid homeostasis. Life Sci Alliance. 2022;5(8):e202201430. doi: 10.26508/lsa.202201430
- Akhberdi O, Zhang Q, Wang H, et al. Roles of phospholipid methyltransferases in pycnidia development, stress tolerance and secondary metabolism in the taxol-producing fungus pestalotiopsis microspore. Microbiol Res. 2018;210:33–42. doi: 10.1016/j.micres.2018.03.001
- Beauvais A, Latge JP. Membrane and cell wall targets in aspergillus fumigatus. Drug Resist Updat. 2001;4(1):38–49. doi: 10.1054/drup.2001.0185
- Fernandes CM, de Castro PA, Singh A, et al. Functional characterization of the Aspergillus nidulans glucosylceramide pathway reveals that LCB Delta8-desaturation and C9-methylation are relevant to filamentous growth, lipid raft localization and Psd1 defensin activity. Mol Microbiol. 2016;102:488–505. doi: 10.1111/mmi.13474
- Li S, Du L, Yuen G, et al. Distinct ceramide synthases regulate polarized growth in the filamentous fungus Aspergillus nidulans. Mol Biol Cell. 2006;17(3):1218–1227. doi: 10.1091/mbc.e05-06-0533
- Head BP, Patel HH, Insel PA. Interaction of membrane/lipid rafts with the cytoskeleton: impact on signaling and function: membrane/lipid rafts, mediators of cytoskeletal arrangement and cell signaling. Biochim Biophys Acta. 2014;1838(2):532–545. doi: 10.1016/j.bbamem.2013.07.018
- Markham P, Robson GD, Bainbridge BW, et al. Choline: its role in the growth of filamentous fungi and the regulation of mycelial morphology. FEMS Microbiol Rev. 1993;10:287–300. doi: 10.1111/j.1574-6968.1993.tb05872.x
- Cotado-Sampayo M, Ramos PO, Perez RO, et al. Specificity of commercial anti-spectrin antibody in the study of fungal and oomycete spectrin: cross-reaction with proteins other than spectrin. Fungal Genet Biol. 2008;45(6):1008–1015. doi: 10.1016/j.fgb.2008.02.003
- Fontaine T. Sphingolipids from the human fungal pathogen Aspergillus fumigatus. Biochimie. 2017;141:9–15. doi: 10.1016/j.biochi.2017.06.012
- Hassing B, Candy A, Eaton CJ, et al. Localisation of phosphoinositides in the grass endophyte epichloë festucae and genetic and functional analysis of key components of their biosynthetic pathway in E. festucae symbiosis and Fusarium oxysporum pathogenesis. Fungal Genet Biol. 2022;159:103669. doi: 10.1016/j.fgb.2022.103669
- Redkar A, Di Pietro A. Adapt your shuttling proteins for virulence: a lesson from the corn smut fungusUstilago maydis. New Phytol. 2018;220(2):353–356. doi: 10.1111/nph.15429
- Zhu X, Li L, Wang J, et al. Vacuolar protein-sorting receptor MoVps13 regulates conidiation and pathogenicity in rice blast fungus magnaporthe oryzae. J Fungi (Basel). 2021;7(12):1084. doi: 10.3390/jof7121084
- Phillips R, Ursell T, Wiggins P, et al. Emerging roles for lipids in shaping membrane-protein function. Nature. 2009;459(7245):379–385. doi: 10.1038/nature08147
- Fabri J, Rocha MC, Malavazi I. Overview of the interplay between cell wall integrity signaling pathways and membrane lipid biosynthesis in fungi: perspectives for Aspergillus fumigatus. Curr Protein Pept Sci. 2020;21:265–283. doi: 10.2174/1389203720666190705164203
- Turk M, Abramovic Z, Plemenitas A, et al. Salt stress and plasma-membrane fluidity in selected extremophilic yeasts and yeast-like fungi. FEMS Yeast Res. 2007;7:550–557. doi: 10.1111/j.1567-1364.2007.00209.x
- Sangappillai V, Nadarajah K. Fatty acid synthase beta dehydratase in the lipid biosynthesis pathway is required for conidiogenesis, pigmentation and appressorium formation in magnaporthe oryzae S6. Int J Mol Sci. 2020;21(19):7224. doi: 10.3390/ijms21197224
- Wang ZY, Soanes DM, Kershaw MJ, et al. Functional analysis of lipid metabolism in magnaporthe grisea reveals a requirement for peroxisomal fatty acid beta-oxidation during appressorium-mediated plant infection. Mol Plant Microbe Interact. 2007;20:475–491. doi: 10.1094/MPMI-20-5-0475
- Goodrich-Tanrikulu M, Howe K, Stafford A, et al. Changes in fatty acid composition of Neurospora crassa accompany sexual development and ascospore germination. Microbiology (Reading). 1998;144(Pt 7):1713–1720. doi: 10.1099/00221287-144-7-1713
- Khunyoshyeng S, Cheevadhanarak S, Rachdawong S, et al. Differential expression of desaturases and changes in fatty acid composition during sporangiospore germination and development in mucor rouxii. Fungal Genet Biol. 2002;37(1):13–21. doi: 10.1016/S1087-1845(02)00028-2
- Thines E, Weber RW, Talbot NJ. MAP kinase and protein kinase A-dependent mobilization of triacylglycerol and glycogen during appressorium turgor generation by Magnaporthe grisea. Plant Cell. 2000;12:1703–1718. doi: 10.1105/tpc.12.9.1703
- Zhang L, Cao X, Wang Z, et al. Brassinolide alleviated chilling injury of banana fruit by regulating unsaturated fatty acids and phenolic compounds. Sci Hortic. 2022;297:110922. doi: 10.1016/j.scienta.2022.110922
- Resnick MA, Mortimer RK. Unsaturated fatty acid mutants of Saccharomyces cerevisiae. J Bacteriol. 1966;92(3):597–600. doi: 10.1128/jb.92.3.597-600.1966
- Wisnieski BJ, Kiyomoto RK. Fatty acid desaturase mutants of yeast: growth requirements and electron spin resonance spin-label distribution. J Bacteriol. 1972;109(1):186–195. doi: 10.1128/jb.109.1.186-195.1972
- Reich M, Gobel C, Kohler A, et al. Fatty acid metabolism in the ectomycorrhizal fungus Laccaria bicolor. New Phytol. 2009;182(4):950–964. doi: 10.1111/j.1469-8137.2009.02819.x
- Ballweg S, Ernst R. Control of membrane fluidity: the OLE pathway in focus. Biol Chem. 2017;398(2):215–228. doi: 10.1515/hsz-2016-0277
- Micoogullari Y, Basu SS, Ang J, et al. Dysregulation of very-long-chain fatty acid metabolism causes membrane saturation and induction of the unfolded protein response. Mol Biol Cell. 2020;31(1):7–17. doi: 10.1091/mbc.E19-07-0392
- Covino R, Ballweg S, Stordeur C, et al. A eukaryotic sensor for membrane lipid saturation. Mol Cell. 2016Jul 7;63(1):49–59. doi: 10.1016/j.molcel.2016.05.015
- Ogasawara Y, Kira S, Mukai Y, et al. Ole1, fatty acid desaturase, is required for Atg9 delivery and isolation membrane expansion during autophagy in Saccharomyces cerevisiae. Biol Open. 2017 Jan 15;6(1):35–40. doi: 10.1242/bio.022053.
- Peng YJ, Wang JJ, Lin HY, et al. HapX, an indispensable bZIP Transcription factor for iron acquisition, regulates infection initiation by orchestrating conidial oleic acid homeostasis and cytomembrane functionality in Mycopathogen Beauveria bassiana. mSystems. 2020;5(5). doi: 10.1128/mSystems.00695-20
- Luo X, Affeldt KJ, Keller NP. Characterization of the far transcription factor family in Aspergillus flavus. G3. 2016;6(6):3269–3281. doi: 10.1534/g3.116.032466
- Surma MA, Klose C, Peng D, et al. A lipid E-MAP identifies Ubx2 as a critical regulator of lipid saturation and lipid bilayer stress. Mol Cell. 2013;51(4):519–530. doi: 10.1016/j.molcel.2013.06.014
- Paege N, Warnecke D, Zauner S, et al. Species-Specific Differences in the Susceptibility of Fungi to the Antifungal Protein AFP Depend on C-3 Saturation of Glycosylceramides. mSphere. 2019;4(6). doi: 10.1128/mSphere.00741-19
- Peng YJ, Zhang H, Feng MG, et al. SterylAcetyl hydrolase 1 (BbSay1) links lipid homeostasis to conidiogenesis and virulence in the entomopathogenic fungus beauveria bassiana. J Fungi (Basel). 2022;8(3):292. doi: 10.3390/jof8030292
- Douglas LM, Konopka JB. Fungal membrane organization: the eisosome concept. Annu Rev Microbiol. 2014;68(1):377–393. doi: 10.1146/annurev-micro-091313-103507
- Guna A, Hegde RS. Transmembrane Domain Recognition during Membrane Protein Biogenesis and Quality Control. Curr Biol. 2018;28(8):R498–R511. doi: 10.1016/j.cub.2018.02.004
- DeZwaan TM, Carroll AM, Valent B, et al. Magnaporthe grisea pth11p is a novel plasma membrane protein that mediates appressorium differentiation in response to inductive substrate cues. Plant Cell. 1999;11(10):2013–2030. doi: 10.1105/tpc.11.10.2013
- Shang J, Tang G, Yang J, et al. Sensing of a spore surface protein by a drosophila chemosensory protein induces behavioral defense against fungal parasitic infections. Curr Biol. 2023;33:276–286. doi: 10.1016/j.cub.2022.11.004
- Ota K, Butala M, Viero G, et al. Fungal MACPF-like proteins and aegerolysins: bi-component pore-forming proteins? Subcell Biochem. 2014;80:271–291.
- Wang F, Wang Y, Zhang X, et al. Recent progress of cell-penetrating peptides as new carriers for intracellular cargo delivery. J Control Release. 2014;174:126–136. doi: 10.1016/j.jconrel.2013.11.020
- Eichel K, von Zastrow M. Subcellular Organization of GPCR Signaling. Trends Pharmacol Sci. 2018;39(2):200–208. doi: 10.1016/j.tips.2017.11.009
- Lebreton S, Zurzolo C, Paladino S. Organization of GPI-anchored proteins at the cell surface and its physiopathological relevance. Crit Rev Biochem Mol Biol. 2018;53(4):403–419. doi: 10.1080/10409238.2018.1485627
- Yun Y, Guo P, Zhang J, et al. Flippases play specific but distinct roles in the development, pathogenicity, and secondary metabolism ofFusarium graminearum. Mol Plant Pathol. 2020;21(10):1307–1321. doi: 10.1111/mpp.12985
- Mazheika I, Voronko O, Kamzolkina O. Early endocytosis as a key to understanding mechanisms of plasma membrane tension regulation in filamentous fungi. Biol Cell. 2020;112(12):409–426. doi: 10.1111/boc.202000066
- Blanchoin L, Boujemaa-Paterski R, Sykes C, et al. Actin dynamics, architecture, and mechanics in cell motility. Physiol Rev. 2014;94(1):235–263. doi: 10.1152/physrev.00018.2013
- Juvvadi PR, Fortwendel JR, Rogg LE, et al. Differential localization patterns of septins during growth of the human fungal pathogen Aspergillus fumigatus reveal novel functions. Biochem Biophys Res Commun. 2011;405(2):238–243. doi: 10.1016/j.bbrc.2011.01.017
- Vargas-Muniz JM, Renshaw H, Richards AD, et al. The Aspergillus fumigatus septins play pleiotropic roles in septation, conidiation, and cell wall stress, but are dispensable for virulence. Fungal Genet Biol. 2015;81:41–51. doi: 10.1016/j.fgb.2015.05.014
- Badrane H, Nguyen MH, Clancy CJ. Highly dynamic and specific phosphatidylinositol 4,5-bisphosphate, septin, and cell wall integrity pathway responses correlate with caspofungin activity against Candida albicans. Antimicrob Agents Chemother. 2016;60(6):3591–3600. doi: 10.1128/AAC.02711-15
- Badrane H, Nguyen MH, Blankenship JR, et al. Rapid redistribution of phosphatidylinositol-(4,5)-bisphosphate and septins during the Candida albicans response to caspofungin. Antimicrob Agents Chemother. 2012;56(9):4614–4624. doi: 10.1128/AAC.00112-12
- Mela A, Momany M. Septins coordinate cell wall integrity and lipid metabolism in a sphingolipid-dependent process. J Cell Sci. 2022;135(5). doi: 10.1242/jcs.258336
- Roelants FM, Su BM, von Wulffen J, et al. Protein kinase Gin4 negatively regulates flippase function and controls plasma membrane asymmetry. J Cell Bio. 2015;208(3):299–311. doi: 10.1083/jcb.201410076
- Takeshita N. Control of actin and calcium for chitin synthase delivery to the hyphal tip of Aspergillus. Curr Top Microbiol Immunol. 2020;425:113–129.
- Seidl-Seiboth V, Zach S, Frischmann A, et al. Spore germination of Trichoderma atroviride is inhibited by its LysM protein TAL6. FEBS J. 2013;280(5):1226–1236. doi: 10.1111/febs.12113
- Zhang LB, Tang L, Guan Y, et al. Subcellular localization of Sur7 and its pleiotropic effect on cell wall integrity, multiple stress responses, and virulence of Beauveria bassiana. Appl Microbiol Biotechnol. 2020;104(15):6669–6678. doi: 10.1007/s00253-020-10736-3
- McMahon HT, Gallop JL. Membrane curvature and mechanisms of dynamic cell membrane remodelling. Nature. 2005;438(7068):590–596. doi: 10.1038/nature04396
- Kunding AH, Mortensen MW, Christensen SM, et al. Intermembrane docking reactions are regulated by membrane curvature. Biophys J. 2011;101(11):2693–2703. doi: 10.1016/j.bpj.2011.09.059
- Roux A, Koster G, Lenz M, et al. Membrane curvature controls dynamin polymerization. Proc Natl Acad Sci, USA. 2010;107:4141–4146. doi: 10.1073/pnas.0913734107
- Mim C, Unger VM. Membrane curvature and its generation by BAR proteins. Trends Biochem Sci. 2012;37(12):526–533. doi: 10.1016/j.tibs.2012.09.001
- Celio GJ, Mims CW, Richardson EA. Ultrastructure and immunocytochemistry of the host–pathogen interface in poinsettia leaves infected with powdery mildew. Can J Bot. 2004;82:421–429. doi: 10.1139/b04-019
- Bridges AA, Jentzsch MS, Oakes PW, et al. Micron-scale plasma membrane curvature is recognized by the septin cytoskeleton. J Cell Bio. 2016;213(1):23–32. doi: 10.1083/jcb.201512029
- Cannon KS, Woods BL, Crutchley JM, et al. An amphipathic helix enables septins to sense micrometer-scale membrane curvature. J Cell Bio. 2019;218(4):1128–1137. doi: 10.1083/jcb.201807211
- Momany M, Talbot NJ. Septins focus cellular growth for host infection by pathogenic fungi. Front Cell Dev Biol. 2017;5:33. doi: 10.3389/fcell.2017.00033
- Dagdas YF, Yoshino K, Dagdas G, et al. Septin-mediated plant cell invasion by the rice blast fungus, magnaporthe oryzae. Science. 2012;336(6088):1590–1595. doi: 10.1126/science.1222934
- Nath S, Dancourt J, Shteyn V, et al. Lipidation of the LC3/GABARAP family of autophagy proteins relies on a membrane-curvature-sensing domain in Atg3. Nat Cell Biol. 2014;16(5):415–424. doi: 10.1038/ncb2940
- Lin HY, Ding JL, Peng YJ, et al. Proteomic and phosphoryproteomic investigations reveal that autophagy-related protein 1, a protein kinase for autophagy initiation, synchronously deploys phosphoregulation on the ubiquitin-like conjugation system in the mycopathogen beauveria bassiana. mSystems. 2022;7(1):e146321. doi: 10.1128/msystems.01463-21
- McMahon HT, Boucrot E. Membrane curvature at a glance. J Cell Sci. 2015;128(6):1065–1070. doi: 10.1242/jcs.114454
- Rojas ER, Huang KC, Theriot JA. Homeostatic cell growth is accomplished mechanically through membrane tension inhibition of cell-wall synthesis. Cell Syst. 2017;5:578–590. doi: 10.1016/j.cels.2017.11.005
- Radeck J, Lautenschlager N, Mascher T. The essential UPP phosphatase pair BcrC and UppP connects cell wall homeostasis during growth and sporulation with cell envelope stress response in Bacillus subtilis. Front Microbiol. 2017;8:2403. doi: 10.3389/fmicb.2017.02403
- Athanasopoulos A, Andre B, Sophianopoulou V, et al. Fungal plasma membrane domains. FEMS Microbiol Rev. 2019;43(6):642–673. doi: 10.1093/femsre/fuz022
- Commer B, Shaw BD. Current views on endocytosis in filamentous fungi. Mycology. 2020;12:1–9. doi: 10.1080/21501203.2020.1741471
- Peng YJ, Ding JL, Lin HY, et al. A virulence-related lectin traffics into eisosome and contributes to functionality of cytomembrane and cell-wall in the insect-pathogenic fungus Beauveria bassiana. Fungal Biol. 2021;125(11):914–922. doi: 10.1016/j.funbio.2021.06.005
- Zhao X, Yang X, Lu Z, et al. MADS-box transcription factor Mcm1 controls cell cycle, fungal development, cell integrity and virulence in the filamentous insect pathogenic fungus beauveria bassiana. Environ Microbiol. 2019;21(9):3392–3416. doi: 10.1111/1462-2920.14629
- Vangalis V, Papaioannou IA, Markakis EA, et al. Hex1, the major component of woronin bodies, is required for normal development, pathogenicity, and stress response in the plant pathogenic fungus verticillium dahliae. J Fungi (Basel). 2020Dec 7;6(4):344. doi: 10.3390/jof6040344
- Palma-Guerrero J, Huang IC, Jansson HB, et al. Chitosan permeabilizes the plasma membrane and kills cells of neurospora crassa in an energy dependent manner. Fungal Genet Biol. 2009;46(8):585–594. doi: 10.1016/j.fgb.2009.02.010
- Stepien L, Lalak-Kanczugowska J. Signaling pathways involved in virulence and stress response of plant-pathogenic fusarium species. Fungal Biol Rev. 2021;35:27–39. doi: 10.1016/j.fbr.2020.12.001
- Wen Z, Tian H, Xia Y, et al. MaPmt1, a protein O-mannosyltransferase, contributes to virulence through governing the appressorium turgor pressure in metarhizium acridum. Fungal Genet Biol. 2020;145:103480. doi: 10.1016/j.fgb.2020.103480
- Klein DA, Paschke MW. Filamentous fungi: the indeterminate lifestyle and microbial ecology. Microb Ecol. 2004;47(3):224–235. doi: 10.1007/s00248-003-1037-4
- Richie DL, Hartl L, Aimanianda V, et al. A role for the unfolded protein response (UPR) in virulence and antifungal susceptibility in Aspergillus fumigatus. PLOS Pathog. 2009;5(1):e1000258. doi: 10.1371/journal.ppat.1000258
- Garcia-Rubio R, de Oliveira HC, Rivera J, et al. The fungal cell wall: Candida, Cryptococcus, and Aspergillus species. Front Microbiol. 2019;10:2993. doi: 10.3389/fmicb.2019.02993
- Wang J, Zhou G, Ying SH, et al. P-type calcium ATPase functions as a core regulator of beauveria bassiana growth, conidiation and responses to multiple stressful stimuli through cross-talk with signalling networks. Environ Microbiol. 2013;15(3):967–979. doi: 10.1111/1462-2920.12044
- Liu J, Wang C, Kong L, et al. Rho2 involved in development, stress response and pathogenicity of Fusarium oxysporum. World J Microbiol Biotechnol. 2023;39(10):272. doi: 10.1007/s11274-023-03720-2
- Ruiz-Roldan C, Pareja-Jaime Y, Gonzalez-Reyes JA, et al. The transcription factor Con7-1 is a master regulator of morphogenesis and virulence in Fusarium oxysporum. Mol Plant Microbe Interact. 2015;28(1):55–68. doi: 10.1094/MPMI-07-14-0205-R
- Yang JY, Fang YL, Wang P, et al. Pleiotropic roles of ChSat4 in asexual development, cell wall integrity maintenance, and pathogenicity in Colletotrichum higginsianum. Front Microbiol. 2018;9:2311. doi: 10.3389/fmicb.2018.02311
- Lebiedzinska M, Szabadkai G, Jones AW, et al. Interactions between the endoplasmic reticulum, mitochondria, plasma membrane and other subcellular organelles. Int J Biochem Cell Biol. 2009;41:1805–1816. doi: 10.1016/j.biocel.2009.02.017
- Christianson JC, Ye Y. Cleaning up in the endoplasmic reticulum: ubiquitin in charge. Nat Struct Mol Biol. 2014;21(4):325–335. doi: 10.1038/nsmb.2793
- Christianson JC, Carvalho P. Order through destruction: how ER-associated protein degradation contributes to organelle homeostasis. EMBO J. 2022;41(6):e109845. doi: 10.15252/embj.2021109845
- Galluzzi L, Kepp O, Trojel-Hansen C, et al. Mitochondrial control of cellular life, stress, and death. Circ Res. 2012;111(9):1198–1207. doi: 10.1161/CIRCRESAHA.112.268946
- Nakatogawa H. Mechanisms governing autophagosome biogenesis. Nat Rev Mol Cell Biol. 2020;21(8):439–458. doi: 10.1038/s41580-020-0241-0
- Hu Y, Wang J, Ying S, et al. Five vacuolar Ca2+ exchangers play different roles in calcineurin-dependent Ca2+/Mn2+ tolerance, multistress responses and virulence of a filamentous entomopathogen. Fungal Genet Biol. 2014;73:12–19. doi: 10.1016/j.fgb.2014.09.005
- Liu Q, Li D, Jiang K, et al. AoPEX1 and AoPEX6 are required for mycelial growth, conidiation, stress response, fatty acid utilization, and trap formation in arthrobotrys oligospora. Microbiol Spectr. 2022;10(2):e27522. doi: 10.1128/spectrum.00275-22
- Ding JL, Li XH, Lei JH, et al. Succinate dehydrogenase subunit C contributes to mycelial growth and development, stress response, and virulence in the insect parasitic fungus beauveria bassiana. Microbiol Spectr. 2022;10(5):e289122. doi: 10.1128/spectrum.02891-22
- Larsen JB, Jensen MB, Bhatia VK, et al. Membrane curvature enables N-Ras lipid anchor sorting to liquid-ordered membrane phases. Nat Chem Biol. 2015;11(3):192–194. doi: 10.1038/nchembio.1733
- Martin SW, Douglas LM, Konopka JB. Cell cycle dynamics and quorum sensing in Candida albicans chlamydospores are distinct from budding and hyphal growth. Eukaryot Cell. 2005;4(7):1191–1202. doi: 10.1128/EC.4.7.1191-1202.2005
- Pedersen R, Drubin DG. Type I myosins anchor actin assembly to the plasma membrane during clathrin-mediated endocytosis. J Cell Bio. 2019;218(4):1138–1147. doi: 10.1083/jcb.201810005
- Peng YJ, Hou J, Zhang H, et al. Systematic contributions of CFEM domain-containing proteins to iron acquisition are essential for interspecies interaction of the filamentous pathogenic fungus beauveria bassiana. Environ Microbiol. 2022;24(8):3693–3704. doi: 10.1111/1462-2920.16032