ABSTRACT
Persistent human papillomavirus HPV infection is a necessary but insufficient condition for cervical cancer. Microorganisms are crucial environmental factors in cancers susceptibility and progression, recently attracting considerable attention. This study aimed to determine the infection status and relationship between high-risk HPV (HR-HPV) and lower genital tract infectious pathogens in cervical cancer and its precursors. From a retrospective and a prospective cohort analysis, Escherichia coli (E. coli) dominated the pathogens isolated from cervical discharges, and an isolation rate uptrend has been shown recently. HPV16 and E. coli’s coinfection rate gradually increased with the severity of cervical intraepithelial neoplasia. The adhesion and invasion abilities of the isolated E. coli to HPV16-positive SiHa cells were evaluated in vitro. The TCGA database and cervical tissues samples analysis showed that IL-10 was upregulated in cervical cancer. IL-10 expression levels increased in tissue samples with the severity of cervical cancer and its precursors with HPV16 and E. coli coinfection. Although no significant changes in IL-10 production were observed in the co-culture supernatant, we hypothesized that Treg immune cells in the tumour microenvironment might be responsible for the local IL-10 upregulation, according to our data showing Foxp3 upregulation and an upward trend with the cervical intraepithelial neoplasia grading to cancer and tumours with E. coli and HPV16 coinfection. Our data provide insights into the possible role of E. coli in cervical cancer progression and suggest that the application of HPV and E. coli screening programs may be an effective strategy to relieve the burden of cervical cancer and its precursor lesions.
Introduction
Cervical cancer is the fourth most common cancer that severely threatens women’s health worldwide. Global cancer data show approximately 604, 000 new cases of cervical cancer and 342,000 deaths in 2020 [Citation1]. According to the China Cancer Registry’s Annual Report, the incidence rate of cervical cancer in China is 11.35 per 100,000 women, with a mortality rate of 3.42 per 100,000 women [Citation2], showing the significant global burden of cervical cancer prevention and control. Reducing the current worldwide incidence by 2030 has been the priority toward the elimination of cervical cancer launched by the World Health Organization (WHO) [Citation3]. High-risk human papilloma virus (HPV) genotypes, such as HPV16 and HPV18, are well-established oncogenic factors involved in cervical carcinogenesis. Generally, 85%-90% of high-risk HPV infections are spontaneously cleared, only a small portion of women persistently infected with HPV progress to precancerous cervical intraepithelial neoplasia (CIN) and even invasive cervical carcinoma (ICC) [Citation4]. This writes down that other cofactors in the local cervicovaginal region are mandatory for cancer development.
Awarenesses of the multifactorial processes that eventually result in cancer has increased considerably in recent years. Many factors including bacteria or their components that, in combination, contribute to tumour formation, have now been added. H. pylori infection was the first pathogen confirmed to be involved in carcinogenesis [Citation5]. Escherichia coli (E. coli) has been identified as a potential risk factor for colorectal cancer (CRC), since several studies have confirmed the cancer-inducing properties of B2 E. coli strains in vivo [Citation6,Citation7]. A recent report from Nature revealed that past exposure to E. coli carrying the colibactin-producing PKS pathogenicity island is responsible for the mutational signatures in colorectal cancer [Citation8]. Recently, insights into the co-infection of viruses and bacteria resulting in disease exacerbation are emerging [Citation9,Citation10]. Earlier studies have also showed that the high prevalence of HPV co-infection with Chlamydia trachomatis may be associated with abnormal cervical cytology and an increased risk of cervical intraepithelial neoplasia [Citation11,Citation12]. A recent investigation pointed out that multiple infections potentially contribute to neoplastic progression by elucidating that C. trachomatis impedes HPV-induced mechanisms that maintain cellular and genomic integrity in the stem cells [Citation13]. However, few studies on the co-infection of HPV and non- sexually transmitted infectious pathogens in cervical cancer.
Here, we analysed the distribution and annual trend of pathogenic bacteria in the cervical discharges of patients diagnosed with HPV-infected cervical cancer and its precursor lesions in two independent cohorts. We mainly focused on the co-infection rate of E. coli and HPV16 in cervical cancer and its precursors in recent years, defined the phylogenetic grouping and virulence genotypes of isolated E. coli, and evaluated its adhesion and invasion abilities to HPV16 positive cancer cells. In addition, we found the higher IL-10 levels in cervical cancer patients and discussed the potential involvement of Tregs recruitment in tumour microenvironment during the cervical cancer development in the context of HPV16 and E. coli coinfection. This study makes a novel contribution to the literature by laying a foundation for HPV and E. coli combined screening programs in cervical cancer.
Materials and methods
Study cohort
To investigate the dominant pathogens isolated from patients diagnosed with cervical cancer (International Classification of Diseases-10th C53), we performed a retrospective (cohort #1, n = 137) study and a prospective (cohort #2, n = 80) study, separately. Patients’ characteristics and additional laboratory indicators were retrieved from the electronic medical records and medical laboratory information system between January 2019 and December 2022 at the Chongqing Health Center for Women and Children. The basic information is provided in . Eligibility criteria were biopsy-proven squamous cell carcinoma, adenocarcinoma, or adenosquamous carcinoma of the uterine cervix, and a diagnosis of stage IA-IIIC cervical cancer according to the International Federation of Gynaecology Reported and Obstetrics standard [Citation14]; an end time of treatment ≥3 months and age 18 years or older; Exclusion criteria were as follows: received radiotherapy, chemotherapy and immunotherapy before surgery; received endocervicectomy and other related treatments; history of systemic and vaginal local use of antibiotics within a month; sexual activity within 24 to 48 hours before the examination; vaginal irrigation, application of drugs or use of vaginal suppositories and vaginal diagnosis; history of taking antibiotics or other drugs in the past 3 months; and loss to follow-up or inability to cooperate with the experimenter due to physical reasons.
Table 1. Clinical characteristics of patients from the retrospective (#1) and prospective (#2) cohorts.
Ethics statement
This study was reviewed and approved by the Institutional Review Board and Independent Ethics Committee of the Chongqing Health Centers for Women and Children. Informed consent was obtained from all the participants in accordance with the Declaration of Helsinki. Patients from cohort #1 have given consent to participate as well as consent to publish the data. All the samples and data from cohort #2 were collected after a written informed consent was obtained from the participants.
Sample collection
Generally, biopsy cervical tissues, cervical secretions, exfoliated cervical cells and serum samples of patients are collected by the clinicians and nurses for pathological diagnosis, microbial culture, HPV genotype, and tumour markers detection, respectively. Specifically, pure colonies of the positive strain by traditional culture were emulsified in 2 ml 0.85% normal saline to be suspended at an optical density of 0.5–0.6 at 600 nm and then transferred to the PhoenixTM M50 (BD, Franklin Lakes, NJ, USA) for bacterial identification, the bacteria solution was mixed with 80% sterilized glycerine according to the ratio of bacteria solution: glycerine = 5: 2 and stored at −80℃. Formalin-fixed embedded paraffin slides of cervical tissue were stored by the Department of Pathology. Each slide was independently examined by two pathologists. The serum samples were collected and stored at −80℃ for further study.
HPV genotyping
Exfoliated cervical cells were collected and stored at 4°C in the standard medium by the clinician. The DNA was isolated and purified using an automatic nucleic acids extraction apparatus (BioPerfectus Technology, Taizhou, Jiangsu, China). HPV testing was performed using the Bio-Perfectus Multiplex Real-Time Polymerase chain reaction (PCR) assay (BMRT) (BioPerfectus Technology, Taizhou, Jiangsu, China) because of its high level of automation and ability to quantify HPV-16, HPV-18, and other genotypes [Citation15]. HPV genotyping performed using eight-tubes for three different genotypes in each tube with a human single-copy gene encoding DNA topoisomerase III (TOP3) as an internal control.
Phylogenetic grouping and Virulence Genotyping
The frozen strains were resuscitated at room temperature, inoculated on onto blood agar plates and incubated aerobically for 18–24 hours in a humid chamber (Thermo Fisher Scientific, Waltham, MA, USA) containing 5% CO2 at 35°C for subculture. Pure colonies were suspended in sterile distilled water and placed in a boiling water bath for 10 min, followed by centrifugation for 10 min, and the supernatant was used as a DNA template for amplification of virulence-associated genes by PCR-based method [Citation16]. Isolates were assigned to phylogenetic groups as follows: ChuA+ YjaA+, group B2; ChuA+ YjaA−, group D; ChuA−TspE4C2+ group B1; ChuA− TspE4C2−, group A. The PCR reactions were started with an initial activation at 94°C for 5 min; then, 30 cycles at 94°C for 30 s, 55°C for 30 s, 72°C for 30 s; and finally, extension at 72°C for 7 min. The PCR products were electrophoresed on 2% agarose gels for final analysis. The primers used are listed in .
Table 2. Primer sequences used in this study.
Cell culture
Human cervical squamous cell lines SiHa and C33A were obtained from the American Type Culture Collection (ATCC, Manassas, VA, USA). The cell lines were maintained in Dulbecco’s modified DMEM medium (Gibco BRL, Grand Island, NY, USA) supplemented with 10% foetal bovine serum (Gibco BRL, Grand Island, NY, USA), and 1% penicillin-streptomycin (Sangon Biotech, Shanghai, China). Cultured cells were incubated in a humidity chamber (Thermo Fisher Scientific, Waltham, MA, USA) containing 5% CO2 at 37°C.
Adhesion assays
Cervical squamous cell lines (SiHa and C33A) were cultured in 12-well plates at a density of 1 × 105 cells/well at 37°C for 24 h, infected with bacterial suspension (107 bacteria/ml; multiplicity of infection [MOI] = 100), and incubated at 37°C for 3 h [Citation17]. After incubation, infected cells were washed with PBS, fixed in methanol and Gram-stained for observation under microscope. The adhesion index measured for each isolate wad defined as the mean number of bacteria per cell after the examination of 100 visual fields in three independent experiments [Citation18].
Invasion assays
The ability of E. coli isolates to invade host cells was examined using the gentamicin protection assay [Citation19]. The cell lines (Siha and C33A) were cultured in 24-well plates (1 × 105 cells/well) for 24 h and infected with bacterial suspension (106 bacteria/ml; MOI = 50) and incubated at 37°C for 3 h. Cells were then washed with PBS and supplemented with fresh medium containing 100 μg/ml gentamicin (Sigma, St. Louis, MO, USA) and incubated further for 1 h. Finally, cell lysates dilutions lysed with a 1% Triton X-100 (Sigma, St. Louis, MO, USA) solution were plated on LB agar (Sangon Biotech, Shanghai, China). The invasion level defined as the percentage of intracellular bacteria compared to the initial inoculum, was then measured. An isolate was considered invasive if its invasion level was ≥ 0.1% [Citation20].
Immunohistochemistry (IHC)
Formalin-fixed, embedded histopathological slides were obtained from the Department of Pathology. Briefly, the slides were dewaxed and rehydrated with ethanol, and autoclaved at 121°C for 10 min. The sections were treated with 3% hydrogen peroxide for 30 min, permeabilized with 1% Triton X-100 for 15 min and incubated with normal goat serum for 15 minutes. Primary antibodies of IL-10 (GeneTex, Irvine, CA, USA) and Foxp3 (Santa Cruz Biotechnology, Santa Cruz, CA, USA) were incubated with sections at 4°C overnight before biotinylated secondary-antibodies were applied. All sections were then stained with DAB (Beyotime Biotechnology, Shanghai, China). Nuclei were counterstained with Meyer’s haematoxylin (Beyotime Biotechnology, Shanghai, China).
IL-10 analysis by ELISA
Cervical cancer cell lines (SiHa and C33A) were infected with E. coli cells as described above and incubated for 24 h. The release of IL-10 from the supernatants was measured using human enzyme-linked immunosorbent assay kits (Invitrogen, Carlsbad, CA, USA) according to the manufacturer’s instructions. Serum samples of patients from cohort #2 were collected and the levels of IL-10 were analysed using the Human IL-10 ELISA Kit (R&D Systems, Minneapolis, MN, USA) following the manufacturer’s instructions.
The cancer genome atlas analysis
Expression levels of gene and clinical information of cervical cancer patients were retrieved from The Cancer Genome Atlas (TCGA) (https://genome-cancer.ucsc.edu and https://tcga-data.nci.nih.gov/tcga/). A total of 267 samples contained Illumina GA RNA-Seq data (normalized) for IL-10 expression in the gene expression matrix. The median value of the measured IL-10 expression levels, based on the range of IL-10 expression in cervical cancer samples, was used to dichotomize the patients into high and low cohorts. Finally, overall survival (OS) according to the IL-10 levels was compared using the long-rank test. OS curves were plotted using the Kaplan-Meier methods.
Statistics
Descriptive statistics were used to analyse patient’s demographic and clinical characteristics. Categorical variables are described as percentages or frequencies. Continuous variables were first tested for normality. Differences in normally distributed data were described as mean ± SD (standard deviation) and compared using the t-test. Differences in non-normal data were expressed as the interquartile range (IQR) and compared using the Wilcoxon rank-sum test or chi-squared test. p values for the comparisons of gene expression between groups were obtained using unpaired t-tests or one-way analyses of variance (ANOVA). The overall survival difference according to the levels of IL-10 in patients with cervical cancer was compared using the Kaplan-Meier estimation, and the log-rank test was used to compare the survival differences. p < 0.05 was considered statistically significant.
Results
Patient characteristics
Clinical characteristics and laboratory indicators of eligible patients with cervical carcinoma (CC) and its precursors cervical intraepithelial neoplasia (CIN) in a retrospective cohort #1(n = 137) and prospective cohort #2 (n = 80) between January 2019 and December 2022 at theChongqing Health Center for Women and Children were collected and recorded. Overall, the basic data of the two independent cohorts showed no significance (). Specifically, the patients are ranged in age from 25 to 74 years, with an average age of 44.17 ± 10.12 years in cohort #1 and 45.31 ± 10.35 in cohort #2. The percentages of participants diagnosed with CC in cohorts #1 and #2 were 19.71% and 23.75%, respectively. A large population of patients were staged as IA1/2 or IB1/2. Additionally, of the 137 samples, 116 microorganisms were successfully isolated; 130 samples were HPV-positive in cohort #1, 72 microorganisms were identified, and 76 samples were HPV-positive in cohort #2. The other clinical features and laboratory indices are shown in .
E. coli dominated the microorganisms isolated from patients with cervical cancer and its precursors
In the retrospective cohort #1, 90.43% of the isolated strains were identified as gram-negative bacilli (n = 105), 6.09% were gram-positive cocci (n = 7), and 3.48% were fungi (n = 4) (). Specifically, E. coli (n = 81) occupied the largest proportion in gram-negative bacillus accounting for 69.83% as presented in . Klebsiella pneumoniae (n = 8) comprised 6.90%, followed by Enterococcus faecalis (6.03%), Proteobacteria (5.17%), Enterobacter cloacae (3.45%), Fungus (3.45%), Pseudomonas aeruginosa (2.59%), Morganell amorganii (1.72%), and Enterobacter aerogenes for 0.86%. Then we observed the pathogen distribution difference between CIN and CC patients and found that the isolation rate of E. coli in patients with cervical cancer was 62.96%, percentages of microorganisms identified as E. coli in patients with CINII and CINIII were 54.5% and 74.3%, respectively (). In addition, an increasing isolation rate of E. coli from cervical discharges in patients diagnosed with cervical cancer and its precursors was observed over the 3-year study period, despite a slight downtrend isolation in 2021 ().
Figure 1. E. coli dominates the pathogens isolated from cervical discharges of patients with cervical cancer and its precursors. (a and e) the overall distribution of pathogens isolated from cervical discharge of patients in the retrospective cohort #1 and prospective cohort #2, respectively; (b and f) difference in pathogen distribution in patients diagnosed with cervical cancer or cervical intraepithelial neoplasia in two independent cohorts; (c) annual trend of mainly pathogens isolation rate from patients in cohort #1; (d) annual trend of E. coli and HPV coinfection from cohort#1.
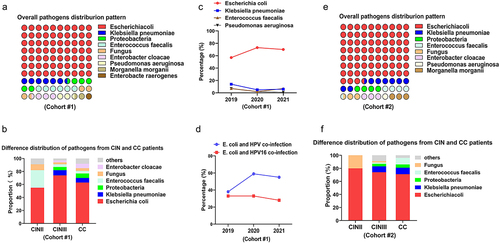
To further confirm whether E. coli was the most commonly isolated bacterium in patients diagnosed with cervical cancer and its precursors, we prospectively collected cervical secretions from patients (Cohort #2, n = 80) between January 2022 and December 2022. In general, 72 microorganisms were isolated and identified from 80 samples, and the overall isolation rate of E. coli was 73.6% (n = 53), followed by Klebsiella pneumoniae (n = 6, 8.3%), Proteobacteria and Enterococcus faecalis at 4.17%, as shown in . Specifically, about 71.4% of microorganisms isolated from patients with cervical cancer were identified as E. coli, and the isolation rate of E. coli in patients with CINII or CINIII was 80.0% and 73.9%, respectively (). These data suggest that E. coli dominates the pathogens isolated from patients diagnosed with cervical cancer or its precursors and has presented an upward trend in recent years.
Co-infection of HPV16 and E. coli in cervical cancer patients
The persistence high-risk HPV genotypes, particularly HPV16 and HPV18, is a well-established oncogenic factor in cervical carcinogenesis. We then analysed HPV subtype infections in patients with cervical cancer or its precursors. Data from cohort #1 in showed that the proportion of patients with a single HPV subtype infection (n = 88) was 67.7%, and 32.3% of whom had multiple subtype infections (defined as two or more subtypes) (n = 42). Furthermore, a large proportion of the patients diagnosed with CC or CIN had a single-subtype HPV infection (). Specifically, HPV16, HPV18, HPV52, and HPV58 were the most common infected HPV subtypes in patients, and the HPV16 subtype ranked the first in both single and mixed HPV subtype infections (). Similar results were observed in cohort #2 (). Interestingly, we found that the proportion of co-infection of E. coli and HPV, in particular genotype 16, gradually increased with the severity of cervical lesions both in cohorts #1 and #2 (), although there was no obvious upward trend in the co-infection rate of E. coli and HPV16 in patients from cohort #1 in the past three years as showed in . Together, our data suggest that co-infection of HPV16 and E. coli existed in patients diagnosed with cervical cancer.
Figure 2. Co-infection of E. coli and HPV16 in patients with cervical cancer and its precursors. HPV infection status in CC and CIN II/III patients from either cohort #1 (a) or cohort #2 (c); the proportion of mainly HPV subtypes in the context of HPV single or mixed infection in CC and CIN II/III patients from either cohort#1(b) or cohor#2; (d) the proportion of E. coli and HPV coinfection in patients with cervical cancer and precursor lesions from either cohort#1 (e) or cohor#2 (f).
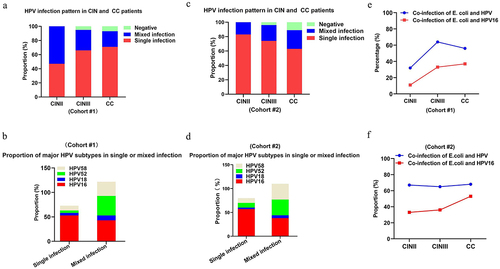
Higher adhesion and invasion of E. coli isolated from HPV16-infected cervical cancer patients
We performed a phylogenetic analysis of E. coli isolated from patients in cohort #2 (n = 42). Of the 42 isolates, 2 (4.76%), 6 (14.29%), 30 (71.43%), and 4 (9.52%) of E. coli isolations were identified as phylogroups A, B1, B2, and D, respectively (). Phylogroup A (12.5%) was detected only in the CIN III group, phylogroup B1 and D were mainly distributed in the CIN III and CC groups. Phylogroup B2 accounted for the largest proportion of phylogroups among the three groups, accounting for 100%, 56.25% and 76.19%, respectively (). it is noteworthy that the proportion of E. coli strains identified as phylogroups B2 from HPV16-infected cases ranked the first, accounting for 50%, compared to that in HPV52- or HPV58-infected cases ().
Figure 3. Phylogenetic analysis and virulence profiling of E. coli isolated from patients with cervical cancer and precursors from cohort#2. (a) the phylogenetic analysis of E. coli isolated in cervical discharges of patients from cohort #2; (b) phylotypes distribution of E. coli identified from patients with cervical cancer and precursors; (c) phylogroup analysis of E. coli isolated from mainly HPV subtypes infected patients; (d) Representative images of adhesion of E. coli to SiHa (HPV16 positive) and C33A (HPV16 negative) cells observed under light microscope or gram staining; (e) cell adhesion and invasion abilities of E. coli isolated from HPV16 infected patients to SiHa and C33A cells. Data are represented as mean ± s.D. Of three independent experiments. ** p < 0.01.
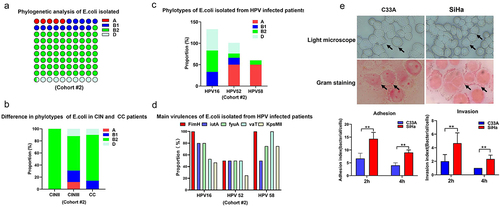
Next, E. coli virulence genes were analysed. As shown in , a higher prevalence (≥40%) was observed for the fimH, fyuA, iutA, kpsMTII, vaT, upaH, and focA (95.23%, 80.95%, 71.42%, 61.90%, 59.52%, 54.76%, and 40.47%, respectively). The expression levels of ireA, papC, papA, hlyA, afa3, ibeA, and cnf1 were less than 25% (23.80%, 21.42%, 16.66%, 14.28%, 9.52%, 7.14%, and 4.76%, respectively). Some isolates harboured the adhesive genes, either individually or in combination. The prevalence of genes encoding fimbrial adhesions pap was 38.1% (of 16/42). The prevalence of the afa gene was 9.52% (of 4/42), three strains possessed both pap and afa genes. In addition, E. coli defined as phylogenetic group B2, harboured most of the virulence genes, including fimH, yjaA, chuA, Tsp, fyuA, iutA, upaH, vaT, and kpsMII (73.8%, 71.42%, 69.04%, 66.66%, 61.90%, 52.38%, 45.23%, 42.85%, and 40.47%, respectively). Furthermore, fimH was also the most prevalent virulence genes expressed in E. coli isolated from patients infected with several major HPV subtypes ().
Table 3. Virulence genes detected by PCR in Escherichia coli strains isolated from cohort #2.
Finally, we assessed the ability of E. coli to adhere and invade by exposing HPV16 positive cervical cancer cell line SiHa cells to the strain isolated from HPV16-infected cervical cancer patients for 2 h, 4 h. The strain defined as phylogroup B2, harbouring most of the virulence genes, including fimH, was selected. As shown in , the adhesion index of E. coli to HPV16 positive SiHa cells was significantly higher than that to HPV16 negative C33A cells 2 h after infection, as observed under a light microscope. This result was confirmed by Gram staining. Gentamicin protection assays further revealed a higher invasion ability of E. coli to SiHa cells than that by C33A cells. Together, these data showed the higher adhesion and invasion abilities of E. coli belonging to phylogroup B2 isolated from HPV16 infected cervical cancer.
Enhanced IL-10 expression in HPV16-infected cervical cancer in the context of E. coli
Considering that IL-10 is central to maintaining the delicate balance between effective immunity and tissue protection, we investigated the effects of E. coli infection on IL-10 levels in patients diagnosed with CC or CIN. First, we accessed the RNA-Seq data of 267 cervical cancer patients from the TCGA CESC dataset to analyse the level of IL-10 expression and found that the expression of IL-10 was higher in the squamous carcinoma than in normal healthy control (). Additionally, patients with squamous carcinoma expressing high IL-10 levels had shorter survival rates than those expressing low IL-10 expression levels (). However, no significant difference was observed in the IL-10 expression levels in serum samples between the CIN and CC groups from cohort #2 measured by ELISA (). We then performed an in vitro co-culture experiment to observe the changes in IL-10 expression levels in SiHa and C33A cells. The results showed no significant differences in IL-10 expression levels in the co-culture supernatants between the groups (). Surprisingly, as shown in , in the absence of E. coli infection, IL-10 expression was slightly increased in cervical tissues of the HPV16-positive CIN II group compared to that in the HPV16-negative CIN II group, whereas an upregulation was observed in IL-10 expression in HPV16-positive CIN II group in the context of E. coli infection, as determined by Immunohistochemistry (IHC). Moreover, IL-10 expression in cervical tissues increased gradually with disease severity in the context of E. coli and HPV16 coinfection. These data prompted us to determine the potential association between IL-10 upregulation and other components such as immune cells in the cervical cancer microenvironment. We then detected Foxp3 expression, which is a hall marker of Treg cells in cervical tissues and found increased recruitment of Treg cells in HPV16-positive cervical cancer patients in the context of E. coli as Foxp3 expression increased gradually with the grading of disease severity (). These results indicate that IL-10 upregulation in HPV16-positive cervical cancer may be, in part, associated with Treg recruitment during E. coli infection.
Figure 4. Enhanced IL-10 expression in cervical cancer or cervical intraepithelial neoplasia patients in the context of HPV16 and E. coli coinfection. (a) unpaired t test using RNA-Seq mRNA expression data from the TCGA database to compare relative IL-10 expression between cervical cancer and normal healthy controls. (b) overall survival according to the levels of IL-10 in cervical patients with the log-rank test applied for comparison. (c) ELISA analysis of IL-10 expression in serum sample of patients with cervical cancer and cervical intraepithelial neoplasia. Data are represented as mean ± s.D. Of three independent experiments. ns means no significance. (d) the isolated E. coli was used to infect SiHa and C33A cells and the co-culture supernatant were collected for IL-10 expression analysis. Data are represented as mean ± s.D. Of three independent experiments. ns means no significance. (e) Representative images of IL-10 and Foxp3 expression levels in cervical tissue samples of patients with cervical cancer and cervical intraepithelial neoplasia by IHC analysis.
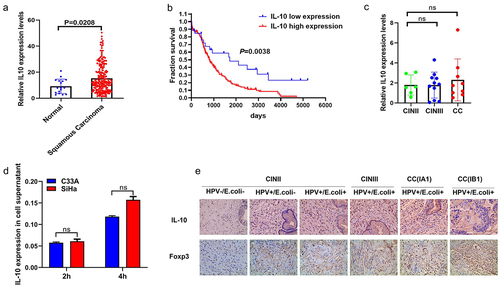
Discussion
Persistent HPV infection has been proved to be a necessary but not sufficient condition for cervical cancer and its precursor lesions. Despite the complex interactions of genes and the environmental factors involved in cancers susceptibility and progression reported in the literature, the role of microorganisms as a crucial environmental factor in the progression of cervical intraepithelial neoplasia to cervical cancer has not been fully elucidated. Herein, we showed that E. coli belonging mostly to phylotype B2 dominated the pathogens isolated from the cervical discharges of cervical cancer patients, and the isolation rate of E. coli showed an upward trend in recent years. Moreover, co-infection with HPV and E. coli was observed in some cases. More importantly, the proportion of HPV16 and E. coli belonging to phylotype B2 coinfection gradually increased with the severity of cervical intraepithelial neoplasia. In addition, we discussed the adhesion and invasion abilities of E. coli phylotype B2 isolated from cervical cancer patient to HPV16 positive cells. Furthermore, IL-10 expression was enhanced in the context of E. coli infection in tissues, although no obvious changes were observed in the co-culture supernatants and serum samples. Finally, we demonstrated that Treg recruitment to the cervical tumour microenvironment may be probably responsible for IL-10 upregulation.
Over the past few decades, we have become accustomed to the idea that human papillomavirus can cause tumours. Genetic and environmental factors, including microbiota, which make up the difference between the elimination of viral infection and the development of cancer, are therefore an area of active investigation [Citation21]. Evidence is emerging that specific microorganisms enriched in tumours, such as the gram-negative bacteria Salmonella, and Helicobacter, are the main strains in gallbladder cancer [Citation22]. Later research showed that anaerobic bacteria, such as Sneathiain, existed throughout the cervical carcinogenesis in the vagina [Citation23]. These findings led us to further investigate the pathogens involved in the progression of cervical cancer. Our data from the retrospective and prospective cohorts indicate that E. coli was the most common microorganisms isolated from the cervical discharges of patients diagnosed with cervical cancer and its precursors, which is consistent with the findings of another research team in China [Citation24]. It is well known that Lactobacillus are the predominant bacteria inhabitant in the healthy vagina. Depletion of Lactobacillus population can result in the overgrowth of aerobic bacteria and increases the severity of cervical intraepithelial neoplasia [Citation25,Citation26]. It should be pointed out that the potential role of overgrowth of aerobic bacteria, such as E. coli in disease progression is worth further discussion. Based on our data, we identified a potential association between E. coli and cervical cancer or its precursors in a retrospective cohort. Additionally, co-infection with E. coli and HPV genotype 16 has been observed in some cases. More importantly, the proportions of E. coli and HPV16 increased with the severity of cervical intraepithelial neoplasia in cancer. Certain bacteria have been reported to cause chronic infections or interrupt the cell cycle through toxins production. E. coli has various virulence factors, such as adhesion molecules, toxins, iron acquisition [Citation27]. A previous study revealed that E. coli infection could induce inflammation and enhance carcinogenesis in cancer development by releasing bacterial genotoxin colibactin [Citation28]. Recent studies have demonstrated that targeting FimH-expressing bacteria is a candidate for the treatment of Crohn’s Disease by suppressing intestinal inflammation without disturbing the intestinal microbiota [Citation29]. Our data showed the highest prevalence of the FimH gene in E. coli, and the largest proportion of E. coli isolated from HPV16-infected patients was defined as phylotype B2, a phylotypes that is believed to be a more frequent carriers of virulence genes than the other phylotypes and conformed to be cytotoxic in vitro [Citation30]. Our experimental results also revealed the higher adhesion and invasion abilities of E. coli belonging to phylotype B2 in HPV16 positive cervical cancer cells. However, further studies are needed to investigate the effect of targeting virulence factors, such as FimH, on cervical cancer progression in a subsequent study.
As an important immunosuppressive factor, IL-10 upregulation has been previously reported and mediated by HPV E2 protein in cervical cancer [Citation31,Citation32]. Here, we further confirmed higher IL-10 expression levels and potential association with disease severity and poor prognosis according to TCGA data analysis. It is well known that IL-10 could be secreted by a variety of cells including T regulatory cells (Treg), infected keratinocytes, tumour associated macrophages (TAM), and tumour cells [Citation33,Citation34]. Among these, Foxp3+ Treg cells, which represent a major IL-10-producing cell subset, play a vital role in inhibiting antitumor immune responses in tumour microenvironment (TME) [Citation33]. We observed that IL-10 production from cervical cancer cells was not enhanced significantly with HPV16 and E. coli coinfection in vitro. However, both IL-10 and Foxp3 expression levels were significantly upregulated and showed an upward trend with the grading of cervical intraepithelial neoplasia to cancer in the context of HPV16 and E. coli coinfection. It is well known that the type III secretion system (T3SS) expressed by gram-negative bacteria is a well-characterized weapon for microbial pathogens to disrupt host homoeostasis and immune defences [Citation35]. A probable explanation for the result of IL-10 analysis in vitro is that T3SS-bearing E. coli may not successfully or timely deliver bacterial effectors into host cell cytoplasm where they manipulate host cell functions by modifying or blocking NF-kB and MAPK signalling pathways in vitro [Citation36]. It seems that the elevated IL-10 and Foxp3 expression simultaneously indicated that the delivered E. coli effectors may enhance Tregs recruitment and IL-10 secretion in local cervical tumour microenvironment. Thus, it would be interesting to investigate the transcriptome levels changes of both E. coli and local cancer cells as well as immune cells in the context of E. coli and HPV16 coinfection by dual-RNA sequencing or single cell transcriptome sequencing [Citation37,Citation38].
This study had several limitations. First, the sample size and source need to be further expanded to analyse the co-infection of E. coli and HPV during cervical cancer development in multiple medical centres. Second, our observations warrant further research on the biological effects of E. coli on host cells, including experiments using animal models. Further studies are needed to elucidate the effect of specific E. coli virulence factor on IL-10 expression and the mechanisms by which these components modulate the tumour microenvironment. Finally, it would be interesting to determine whether E. coli infection shapes the tumour microenvironment in cervical cancer.
In conclusion, we found that E. coli isolated in this study primarily belongs to phylotype B2. Additionally, co-infection of HPV and E. coli in patients with cervical cancer and its precursors in recent years was observed, and further confirmed the higher adhesion and invasion of E. coli isolated from cervical cancer patients to HPV-16-positive cervical cancer cells. Lastly, we found no obvious changes in IL-10 expression levels in either serum samples or co-culture supernatants, but IHC analysis confirmed that both IL-10 and Foxp3 expression were upregulated in tissues in the context of E. coli infection in patients with HPV16 positive-cervical cancer or its precursors. Together, these findings are expected to provide inspirational insights into E. coli and HPV coinfection in cervical cancer development and lay a foundation for HPV and E. coli combined screening programs in cervical cancer prevention and therapy.
Disclosure statement
No potential conflict of interest was reported by the author(s).
Data availability statement
The RNA-seq datasets and clinical information of cervical cancer patients for overall survival analysis in the current study were retrieved from TCGA database (https://genome-cancer.ucsc.edu and https://tcga-data.nci.nih.gov/tcga/).
Additional information
Funding
References
- Sung H, Ferlay J, Siegel RL, et al. Global cancer statistics 2020: GLOBOCAN estimates of incidence and mortality worldwide for 36 cancers in 185 countries. CA Cancer J Clin. 2021 May;71(3):209–12
- He J, Wei WQ China cancer registry annual report 2020. Beijing: People’s Medical Publishing House. 2022. https://book.kongfz.com/575075/5231142426/ (In Chinese)
- Singh D, Vignat J, Lorenzoni V, et al. Global estimates of incidence and mortality of cervical cancer in 2020: a baseline analysis of the WHO global cervical cancer elimination initiative. Lancet Glob Health. 2023 Feb;11(2):e197–e206
- Crosbie EJ, Einstein MH, Franceschi S, et al. Human papillomavirus and cervical cancer. Lancet. 2013 Sep 7;382(9895):889–99. doi: 10.1016/S0140-6736(13)60022-7
- RM P Jr, Blaser MJ. Helicobacter pylori and gastrointestinal tract adenocarcinomas. Nat Rev Cancer. 2002 Jan;2(1):28–37. doi: 10.1038/nrc703
- Arthur JC, Perez-Chanona E, Mühlbauer M, et al. Intestinal inflammation targets cancer-inducing activity of the microbiota. Science. 2012 Oct 5;338(6103):120–3. doi: 10.1126/science.1224820
- Cougnoux A, Dalmasso G, Martinez R, et al. Bacterial genotoxin colibactin promotes colon tumour growth by inducing a senescence-associated secretory phenotype. Gut. 2014 Dec;63(12):1932–42
- Pleguezuelos-Manzano C, Puschhof J, Rosendahl Huber A, et al. Mutational signature in colorectal cancer caused by genotoxic pks+ E. coli. Nature. 2020 Apr;580(7802):269–273
- Griffiths EC, Pedersen AB, Fenton A, et al. The nature and consequences of coinfection in humans. J Infect. 2011 Sep;63(3):200–206. doi: 10.1016/j.jinf.2011.06.005
- Kaul D, et al. Author correction: microbiome disturbance and resilience dynamics of the upper respiratory tract during influenza a virus infection. Nat Commun. 2020;11(1):3132. doi: 10.1038/s41467-020-17020-y
- Chumduri C, Gurumurthy RK, Zadora PK, et al. Chlamydia infection promotes host DNA damage and proliferation but impairs the DNA damage response. Cell Host Microbe. 2013 Jun 12;13(6):746–58. doi: 10.1016/j.chom.2013.05.010
- Chen H, Luo L, Wen Y, et al. Chlamydia trachomatis and human papillomavirus infection in women from Southern Hunan Province in China: a large observational study. Front Microbiol. 2020 May 5;11:827. doi: 10.3389/fmicb.2020.00827
- Koster S, Gurumurthy RK, Kumar N, et al. Modelling chlamydia and HPV co-infection in patient-derived ectocervix organoids reveals distinct cellular reprogramming. Nat Commun. 2022 Feb 24;13(1):1030. doi: 10.1038/s41467-022-28569-1
- Bhatla N, Aoki D, Sharma DN, et al. Cancer of the cervix uteri: 2021 update. Int J Gynaecol Obstet. 2021 Oct;1(Suppl 1):28–44
- Sun Z, Zhang R, Liu Z, et al. Development of a fluorescence–based multiplex genotyping method for simultaneous determination of human papillomavirus infections and viral loads. BMC Cancer. 2015 Nov 6;15(1):860. doi: 10.1186/s12885-015-1874-9
- Clermont O, Christenson JK, Denamur E, et al. The Clermont Escherichia coli phylo-typing method revisited: improvement of specificity and detection of new phylo-groups. Environ Microbiol Rep. 2013 Feb;5(1):58–65
- Pekmezovic M, Hovhannisyan H, Gresnigt MS, et al. Candida pathogens induce protective mitochondria-associated type I interferon signalling and a damage-driven response in vaginal epithelial cells. Nat Microbiol. 2021 May;6(5):643–657
- Mange JP, Stephan R, Borel N, et al. Adhesive properties of Enterobacter sakazakii to human epithelial and brain microvascular endothelial cells. BMC Microbiol. 2006 Jun 26;6(1):58. doi: 10.1186/1471-2180-6-58
- Sokolovska A, Becker CE, Stuart LM. Measurement of phagocytosis, phagosome acidification, and intracellular killing of staphylococcus aureus. Curr Protoc Immunol. 2012 Nov;14(1):14.30.1–14.30.12. Chapter. doi: 10.1002/0471142735.im1430s99
- Kamali Dolatabadi R, Fazeli H, Emami MH, et al. Phenotypicand genotypic characterization of clinical isolates of intracellular adherent-invasive Escherichia coli among different stages, family history, and treated colorectal cancer patients in Iran. Front Cell Infect Microbiol. 2022 Jul 11;12:938477. doi: 10.3389/fcimb.2022.938477
- Sepich-Poore GD, Zitvogel L, Straussman R, et al. The microbiome and human cancer. Science. 2021 Mar 26;371(6536):eabc4552. doi: 10.1126/science.abc4552
- Tsuchiya Y, Loza E, Villa-Gomez G, et al. Metagenomics of microbial communities in gallbladder bile from patients with gallbladder cancer or Cholelithiasis. Asian Pac J Cancer Prev. 2018 Apr 25;19(4):961–967. doi: 10.22034/APJCP.2018.19.4.961
- Łaniewski P, Ilhan ZE, Herbst-Kralovetz MM. The microbiome and gynaecological cancer development, prevention and therapy. Nat Rev Urol. 2020 Apr;17(4):232–250. doi: 10.1038/s41585-020-0286-z
- Hou Y, Pan J, Kuerban G. [Pathogenic bacterium and drug resistance in cervical cancer patients complicated with reproductive tract infection. Zhong Nan Da Xue Xue Bao Yi Xue Ban. 2016 Jul;41(7):721–728. doi: 10.11817/j.issn.1672-7347.2016.07.011
- Plisko O, Zodzika J, Jermakova I, et al. Aerobic vaginitis-underestimated risk factor for cervical intraepithelial neoplasia. Diagnostics (Basel). 2021 Jan 9;11(1):97. doi: 10.3390/diagnostics11010097
- Liu H, Liang H, Li D, et al. Association of cervical dysbacteriosis, hpv oncogene expression, and cervical lesion progression. Microbiol Spectr. 2022 Oct 26;10(5):e0015122. doi: 10.1128/spectrum.00151-22
- Masseret E, Boudeau J, Colombel JF, et al. Genetically related Escherichia coli strains associated with crohn’s disease. Gut. 2001 Mar;48(3):320–5
- Cougnoux A, Dalmasso G, Martinez R, et al. Bacterial genotoxin colibactin promotes colon tumour growth by inducing a senescence-associated secretory phenotype. Gut. 2014;63(12):1932–1942. doi: 10.1136/gutjnl-2013-305257
- Chevalier G, Laveissière A, Desachy G, et al. Blockage of bacterial FimH prevents mucosal inflammation associated with crohn’s disease. Microbiome. 2021 Aug 23;9(1):176. doi: 10.1186/s40168-021-01135-5
- Wassenaar TE. Coli and colorectal cancer: a complex relationship that deserves a critical mindset. Crit Rev Microbiol. 2018 Sep;44(5):619–632. doi: 10.1080/1040841X.2018.1481013
- Torres-Poveda K, Bahena-Román M, Madrid-González C, et al. Role of IL-10 and TGF-β1 in local immunosuppression in HPV-associated cervical neoplasia. World J Clin Oncol. 2014 Oct 10;5(4):753–763. doi: 10.5306/wjco.v5.i4.753
- Saraiva M, O’Garra A. The regulation of IL-10 production by immune cells. Nat Rev Immunol. 2010 Mar;10(3):170–81. doi: 10.1038/nri2711
- Neumann C, Scheffold A, Rutz S. Functions and regulation of T cell-derived interleukin-10. Semin Immunol. 2019 Aug;44:101344. doi: 10.1016/j.smim.2019.101344
- Ouyang W, O’Garra A. IL-10 family cytokines IL-10 and IL-22: from basic science to clinical translation. Immunity. 2019 Apr 16;50(4):871–891. doi: 10.1016/j.immuni.2019.03.020
- Pinaud L, Sansonetti PJ, Phalipon A. Host cell targeting by enteropathogenic bacteria T3SS effectors. Trends Microbiol. 2018 Apr;26(4):266–283. doi: 10.1016/j.tim.2018.01.010
- Gao L, Han H, Wang H, et al. IL-10 knockdown with siRNA enhances the efficacy of doxorubicin chemotherapy in EBV-positive tumors by inducing lytic cycle via PI3K/p38 MAPK/NF-kB pathway. Cancer Lett. 2019 Oct 10;462:12–22. doi: 10.1016/j.canlet.2019.07.016
- Westermann AJ, Gorski SA, Vogel J. Dual RNA-seq of pathogen and host. Nat Rev Microbiol. 2012 Sep;10(9):618–30. doi: 10.1038/nrmicro2852
- Penaranda C, Hung DT. Single-cell RNA sequencing to understand host-pathogen interactions. ACS Infect Dis. 2019 Mar 8;5(3):336–344. doi: 10.1021/acsinfecdis.8b00369