ABSTRACT
Flagella play a crucial role in the invasion process of Salmonella and function as a significant antigen that triggers host pyroptosis. Regulation of flagellar biogenesis is essential for both pathogenicity and immune escape of Salmonella. We identified the conserved and unknown function protein STM0435 as a new flagellar regulator. The ∆stm0435 strain exhibited higher pathogenicity in both cellular and animal infection experiments than the wild-type Salmonella. Proteomic and transcriptomic analyses demonstrated dramatic increases in almost all flagellar genes in the ∆stm0435 strain compared to wild-type Salmonella. In a surface plasmon resonance assay, purified STM0435 protein-bound c-di-GMP had an affinity of ~8.383 µM. The crystal structures of apo-STM0435 and STM0435&c-di-GMP complex were determined. Structural analysis revealed that R33, R137, and D138 of STM0435 were essential for c-di-GMP binding. A Salmonella with STM1987 (GGDEF protein) or STM4264 (EAL protein) overexpression exhibits completely different motility behaviours, indicating that the binding of c-di-GMP to STM0435 promotes its inhibitory effect on Salmonella flagellar biogenesis.
Introduction
Salmonella is an important intracellular, gram-negative bacterium that enters the host through contaminated food or water, causing gastroenteritis and typhoid fever [Citation1–3]. In the past decade, Salmonella has caused more than 650,000 deaths annually; making it a major public health threat worldwide [Citation4,Citation5]. Salmonella with resistance to antimicrobial drugs is now widespread. Developing a comprehensive understanding of the Salmonella pathogenic mechanisms is critical for the reduction and treatment of severe infections.
Flagellum-dependent motility is essential for Salmonella to establish bacterial infections and to evade the host immune system. Flagellum-dependent swimming motility enables pathogens to reach the host infection site [Citation6,Citation7]. At target sites, flagella can act as a “bridge” that allows Salmonella to adhere to the target surface [Citation8,Citation9]. Flagella are also crucial for Salmonella biofilm formation, a long-lasting form of pathogenic bacteria that can enhance the persistence of its transmission and colonization in vivo [Citation10,Citation11]. In addition to contributing to motility and invasion, the flagellar filaments FliC and FljB can be recognized by the TLR5 receptor, Nalp3, and Ipaf, leading to the activation of the host’s immune response and inflammation [Citation12–14]. Hence, flagella play a key role in Salmonella invasion and infection.
The YajQ family of proteins is broadly distributed in bacteria, with one member present in almost every species [Citation15]. In Xanthomonas campestris, a plant pathogenic bacterium, the YajQ family member was identified as a c‐di‐GMP receptor protein and was found to affect virulence and biofilm formation. In this bacterium, YajQ promotes DNA binding and transcriptional activity of the transcription factor LysR through protein-protein interactions. However, the binding of c-di-GMP to YajQ can inhibit YajQ-LysR interaction, leading to virulence and biofilm gene regulation [Citation16]. Similarly, YajQ homologs in Pseudomonas aeruginosa and Stenotrophomonas maltophilia control virulence [Citation17]. However, the role of the YajQ protein in Salmonella and whether it can act as a c-di-GMP receptor are not well understood. In Salmonella, the PilZ domain is one of the c-di-GMP binding protein domains, present in proteins such as the flagellar brake protein YcgR and the cellulose synthesis enzyme BcsA [Citation18–20]. Another c-di-GMP-binding protein, SicA, functions as one of the T3SS-1chaperone and serves as a bridge of c-di-GMP to exert its regulatory effects on T3SS–1 [Citation21]. The functional roles of these proteins are affected by their c-di-GMP-bound state.
In this study, we found that the YajQ family protein STM0435 is a potential c-di-GMP effector in Salmonella. The stm0435 gene is evolutionarily conserved among bacteria. This protein influences virulence in Salmonella by regulating flagellar biosynthesis. STM0435-mediated flagellar control occurs before the transcription and expression of FlhDC, the “master switch” of flagellar genes. In the presence of STM0435, the expression levels of three classes of flagellar genes were significantly decreased, and the rdar morphotype, a multicellular behaviour of Salmonella characterized by the expression of the adhesive extracellular matrix components cellulose and curli fimbriae, was downregulated. In vitro data showed that STM0435 binds to c-di-GMP with an affinity of 8.383 µM. Further structural analysis showed that STM0435 binds to one c-di-GMP molecule in the N domain at R33 and one in the C domain at R137 and D138. The STM1987 or STM4264 overexpression assay demonstrated that c-di-GMP acts as an activator of the inhibitory effect of STM0435 on Salmonella flagellar biogenesis. In summary, this work provides direct evidence for the interaction between STM0435 and c-di-GMP, newly defining an additional receptor of c-di-GMP in Salmonella. The c-di-GMP-STM0435 complex regulates flagellar synthesis, allowing Salmonella to survive in the host.
Materials and methods
Plasmid construction
The DNA fragment of the STM0435 gene was amplified by PCR from Salmonella Typhimurium ATCC 14028s genomic DNA. After purification and restriction enzyme digestion, the PCR fragment was inserted into the PGL01 vector to construct the expression plasmid pGL01-STM0435. The STM0435 mutant (R33H, R137H D138H, R33H R137H D138H) was generated by PCR using the recombinant plasmid pGL01-STM0435. After purification and digestion, linear PCR fragments were ligated and cyclized to generate the pGL01-STM0435mut plasmid. The plasmids, primers, and strains constructed in this study are detailed in Supplementary Tables 2, 3, and 1, respectively.
Protein expression and purification
E. coli BL21 (DE3) cells were used to express proteins in E. coli. Cells were grown in LB medium containing 100 μg/mL Ampicillin at 37°C to logarithmic phase. The expression of protein was induced overnight by addition of 0.6 mM IPTG at 16°C. The cells were harvested at 4,000 × g for 20 min and resuspended in loading buffer (25 mM Tris-HCl pH 8.0, 200 mM NaCl). After ultrasonic fragmentation and centrifugation, the cells were lysed and the His-tagged proteins were purified with Ni2+ His-Trap HP. Protein quality and concentration were determined by SDS-PAGE followed by coomassie blue staining. All protein purification steps were performed at 4°C.
Swimming motility
Activated bacterial cells were grown in LB medium. At an OD600 of 1.2, cells from 1 mL of culture were gently centrifuged and resuspended in 30 μL of PBS. Next, 10 μL bacterial suspensions were applied to 0.25% LB agar plates (pH 5.0, adjusted with acetic acid) and incubated at 30°C.
Growth curves assay
The growth curve assay was performed using a Bioscreen C Automatic Growth Analyzer at 37°C in LB medium. The cultures were diluted 100-fold in fresh medium and added to each well of a honeycomb microplate at a final volume of 300 μL. At 600 nm, bacterial growth was measured every 5 min for 24 h. All strains were assayed in triplicate.
Cytotoxicity assay
The cytotoxicity of the bacteria was measured using the Promega CytoTox 96 nonradioactive cytotoxicity assay kit, according to the manufacturer’s protocol. In brief, the murine RAW264.7 macrophage-like cell line (ATCC) was seeded in triplicate in 96 well plates at a cell density of 1 × 105 cells per well. Bacteria were cultured to log phase and then seeded on RAW264.7 cells at a multiplicity of infection (MOI) of 10. At different time points after infection, the supernatants were collected, and freshly prepared reagents were added and incubated for 30 min in the dark at room temperature. The reaction was stopped by adding a stop solution. The OD490 absorption was measured, and the percent cytotoxicity was quantified.
Crystallization and structure determination
Crystals of STM0435 were grown by hanging drop vapour diffusion at 16°C. The crystal culture buffer contained 20% PEG3350 and 0.2 M Lithium acetate dihydrate. For crystals of STM0435-c-di-GMP, 1 mM c-di-GMP was added to 100 µM STM0435. The crystals were obtained in a buffer containing 20% PEG3350 and 0.2 M lithium acetate dihydrate. The diffraction data of the obtained crystals were collected using beamline BL17u1 at the Shanghai Synchrotron Radiation Facility (SSRF). The datasets were processed using the HKL2000 software suite. The atomic model was built using Coot software and refined using PHENIX. The data collection and structure refinement statistics are summarized in Supplementary Table S4. Structural figures were generated using PyMol software.
Construction of STM0435 knock-out strain
The STM0435 knockout strain was constructed using the one-step gene inactivation method with the lambda Red recombinase system, as described previously [Citation22].
RNA preparation and real-time quantitative PCR
Total RNA of bacteria was obtained using the TRIzol reagent extraction method and then reverse transcribed using the RevertAid cDNA Synthesis Kit according to the manufacturer’s instructions. The cDNA produced and the corresponding primers were used in a real-time quantitative PCR assay and detected using iTaq Universal SYBR Green SuperMix in an Applied Biosystems 7500 sequence detection system. The relative mRNA expression of the target genes was calculated using the 2-ΔΔct method and 16sRNA was used as an internal control.
Congo red assay
Congo red (CR) is widely used to stain biofilm cells because of its ability to bind to cellulose. Briefly, seed cultures were grown in LB medium overnight and then diluted 100-fold in fresh medium, and cultured at 37 °C. At the mid-log phase, 2 μL bacterial cultures were applied to Congo red plates and incubated at 37°C. Congo red plates were prepared as tryptone broth without salt with the addition of 200 µg/mL Congo red and 1.0% agar.
RNA-sequencing analysis
Wild-type and ∆stm0435 Salmonella strains were cultured overnight in LB medium, diluted 1:100 in fresh medium, and grown to mid-log phase. Cells from 10 mL of culture were collected by centrifugation (4000 rpm, 20 min) and washed three times with PBS before freezing in liquid nitrogen. RNA sequence analysis was performed by Novogene Co. Ltd. Briefly, total RNA was extracted using TRIzol reagent. The quantification and qualification of RNA were performed using 1% agarose gels and an Agilent 2100 Bioanalyzer. After the removal of rRNA and random fragmentation, the RNA samples were used to synthesize cDNA. The DNA fragments were 3’ end- adenylated and ligated to adaptors for hybridization. The fragments were purified and selected using the AMPure XP system, the DNA products were further purified, and library quality was assessed using the Agilent BioAnalyst 2100 system. Finally, the libraries were sequenced using the Illumina NovaSeq platform.
Tandem mass tag quantitative proteomic analysis
Tandem mass tag (TMT) quantitative proteomic analysis was performed by Novogene Co., Ltd. Bacterial samples were collected as described above (RNA sequencing analysis). Total protein was extracted by ultrasonication on ice in lysis buffer (2% SDS, 100 mM DTT, 100 mM Tris-HCl, pH 7.6). Cell lysates were collected with acetone and quantified using the BCA protein assay kit. Protein samples were trypsin-digested overnight and desalted using a Strata X-C18-SPE column. The eluents from each sample were collected and lyophilized. TMT-labelled peptides were obtained using 0.5 M TEAB according to the manufacturer’s protocol. The peptides were subjected to liquid chromatography-tandem mass spectrometry (LC-MS/MS) and analysed using an Agilent 300Extend C18 column (5 μm particles, 4.6 mm inner diameter, 250 mm length).
SPR assay
An SPR assay was used to measure STM0435-c-di-GMP interaction in vitro, and was performed using a Biacore T200 system at 25°C in a PBS-P+ running buffer (2.7 mM KCl, 137 mM NaCl, 0.05% surfactant P20, pH 7.4). Purified proteins were immobilized on a CM7 sensor chip using an NHS/EDC (N-hydroxysuccinimide/1-ethyl-3-(3-dimethylaminopropyl)-carbodiimide) amine coupling kit in 10 mM sodium acetate buffer at pH 4.0. The ligand densities of STM0435 and STM0435mut were 8600 and 9600 response units (RU), respectively. To determine the binding affinity of proteins and c-di-GMP, solutions of c-di-GMP at a range of concentrations were loaded into the chambers with immobilized proteins. The concentration of c-di-GMP or GMP ranged from 0.97 to 62.5 μM.
Invasion assay
To examine the impact of STM0435 on Salmonella invasion, cultures at OD600 = 0.5 were washed and resuspended in RPMI-1640 medium. Bacterial cells were seeded on HT-29 monolayers at a multiplicity of infection of 1. At 30 min post-exposure, HT-29 cells were placed in RPMI-1640 medium containing 100 μg/mL gentamicin for 1 h to kill extracellular bacteria. The cells were rinsed twice with RPMI-1640 medium and lysed with 1% Triton X-100. Dilutions were plated on LB agar plates and colony-forming unit (CFU) counts were determined.
Survival curve of BALB/c mice after infection
Activated bacterial cells were cultured to mid-log phase and then resuspended in sterile normal saline at 1 × 106 cells/mL. Six- to eight-week-old female BALB/c mice were used for the survival study. Mice (five per group) were infected with 2 × 105 cells/mouse by intraperitoneal injection, and the deaths of mice were closely monitored every 2–3 h. The natural death time of mice was recorded. For bacterial counts, the mice were sacrificed 12 h postinfection, and the numbers of bacteria in the spleen and liver were measured as CFU counts of viable colonies. All animal procedures were approved by the Ethics Committee of Animal Care and Use of the Institute of Basic Medicine, Shandong Academy of Medical Sciences (approval number: IBMSAMSC Number 096, approval date: 1 February 2023).
Results
STM0435 is evolutionarily well-conserved in Salmonella
STM0435 has been previously annotated as a hypothetical protein in the Salmonella proteome. The sequence encodes a small (163 residues) monomeric protein belonging to the YajQ family with an unknown structure and uncharacterized function. When functional information is lacking for a protein, characterization of its phylogenetic relationships may provide insights into its molecular function. To obtain insights into the function of STM0435, its phylogeny, gene expansion, and contraction at each branch of evolution were investigated. We screened bacterial genomes from GenBank using the PSI-BLAST program with the STM0435 protein sequence (identity threshold of 50% and length coverage threshold of 0.8). The results were mapped to large phylogenetic trees using the AnnoTree program, based on the target taxonomy ID. The analysis showed a broad distribution of stm0435 in bacteria but not in eukaryotic organisms. Among bacteria, stm0435 was significantly enriched in Gammaproteobacteria. A total of 2778 genomes in Gammaproteobacteria were enriched in the Genome Taxonomy Database, accounting for nearly 48% of the Gammaproteobacteria whole genomes and 29% of the Proteobacteria whole genomes (Figure S1A). Surprisingly, homologs of STM0435 were conserved in common pathogenic bacteria including Vibrio cholerae, Ralstonia solanacearum, Pseudomonas aeruginosa, Mycobacterium tuberculosis, Bacillus halodurans, Campylobacter jejuni, Yersinia pestis, Pasteurella multocida, Salmonella Typhimurium and Escherichia coli (Figure S1B). Although the function of STM0435 is poorly understood, the distribution of STM0435 homologs suggests its important role in common pathogenic microorganisms. The STM0435 protein sequence is highly conserved between the two Salmonella species, S. bongori and S. enterica [Citation23–25] (Figure S1C). S. enterica frequently causes diseases in humans and animals, whereas S. bongori is primarily associated with reptile diseases. Possibly due to host differences, there are differences in the amino acid sequences of STM0435 for the two species, with S. enterica STM0435 being only 88% identical to S. bongori YajQ (WP_001138915.1). S. enterica showed a high amino acid consistency within the species (identity 99.4 ~ 100%). Homogeneity analysis demonstrated that STM0435 homologs are associated with clinical pathogens; however, the function of this gene has not yet been described.
∆stm0435 strain exhibits higher pathogenicity than wild-type strain
To determine the function of STM0435 in Salmonella growth, we compared the growth of WT and ∆stm0435 strains. The growth curves of the WT and ∆stm0435 strains were similar in LB medium, indicating that deletion of the stm0435 gene from Salmonella genome had no effect on the growth properties of Salmonella under these culture conditions (). The pathogenicity of Salmonella is primarily determined by its virulence; therefore, we explored whether STM0435 affected the pathogenicity of Salmonella. Salmonella pathogenesis process includes reaching the optimal host site, colonization or invasion, maintenance at the infection site, and postinfection dispersal [Citation26,Citation27]. Therefore, we investigated the virulence phenotype of Salmonella at both cellular and animal levels. First, HT-29 cells were infected with WT or ∆stm0435 at the same multiplicity of infection (MOI). Remarkably, the invasion ability of the ∆stm0435 strain was enhanced compared to that of the WT strain, with increases of 5.3- fold (). Next, the cell cytotoxicity of WT and ∆stm0435 was determined by measuring lactate dehydrogenase (LDH) release, indicating cell membrane damage. The LDH levels in ∆stm0435 were significantly higher than those in WT group (). Finally, the effects of STM0435 on Salmonella virulence were investigated in vivo. The results of the animal infection experiments showed that all mice infected with the ∆stm0435 strain died within 49 h, but those infected with the WT strain died only after approximately 73 h, revealing that these strains are significantly different in pathogenicity (). The ∆stm0435 strain also exhibited significantly enhanced ability to colonize the spleen and liver after intraperitoneal infection (). Therefore, STM0435 affects the pathogenicity of Salmonella, the exact mechanism underlying this effect remains unknown.
Figure 1. STM0435 reduces pathogenicity in Salmonella. (a) Growth curves of the wild-type and ∆stm0435 strains cultivated in LB medium. (b) Invasion capability of the two strains for HT-29 cells. (c) Lactate dehydrogenase (LDH) release from RAW 264.7 macrophages after infection with Salmonella. The levels of LDH released from RAW 264.7 macrophages indicate bacterial cytotoxic effects against HeLa cell lines. (d) Survival curve of BALB/c mice infected with equal numbers of the WT and ∆stm0435 strains. Survival of mice was observed every 2 to 3 h. (e and f) Numbers of Salmonella organisms in the liver and spleen of BALB/c mice after infection with equal numbers of strains by intraperitoneal injection. Data are shown as the mean ± SEM, n = 3. ***p < 0.001; **p < 0.01; *p < 0.05; n.s., p > 0.05.
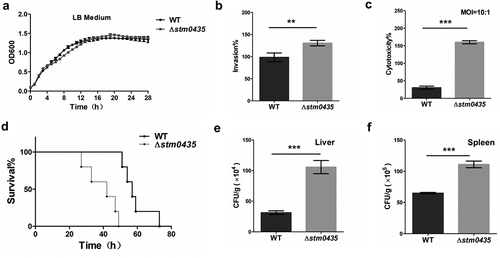
STM0435 affects flagellar assembly in Salmonella
Transcriptomics and quantitative proteomics are effective tools for predicting protein function. To better understand the mechanism of STM0435-mediated virulence phenotypes of Salmonella, WT and ∆stm0435 strains were cultured to the mid-log phase, and then RNA-sequencing analysis and mass spectrometry-based proteomics were performed (Figure S3). The results of integrative omics analysis indicated the enrichment of genes significantly upregulated in Δstm0435 compared to WT, mainly in pathways related to flagellar assembly and bacterial chemotaxis, which depend on flagellum motility (). Based on transcriptomic data analysis of the flagellar pathway, we found that 43 flagellar genes, which belonged to class I, class II, and class III, were significantly increased in the ∆stm0435 strain compared to the WT strain ().
Figure 2. STM0435 inhibits the flagellar synthesis pathway of Salmonella. (a) Combined transcriptome and proteome analyses showed that flagellum-dependent cell motility and chemotaxis pathways were significantly enriched and upregulated in the ∆stm0435 strain. Red and green points indicate the upregulated and downregulated genes, respectively. (b and c) Changes in transcription levels of genes associated with flagellar synthesis. (b) Volcano plots were generated to visualize the transcriptomic results. Red and green points indicate the upregulated and downregulated genes, respectively. (c) Heatmap showing the relative transcript abundance of genes involved in flagellar synthesis, as determined from transcriptomic data. Data are shown as the mean ± SEM, n = 3. ***p < 0.001; **p < 0.01; *p < 0.05; n.s., p > 0.05.
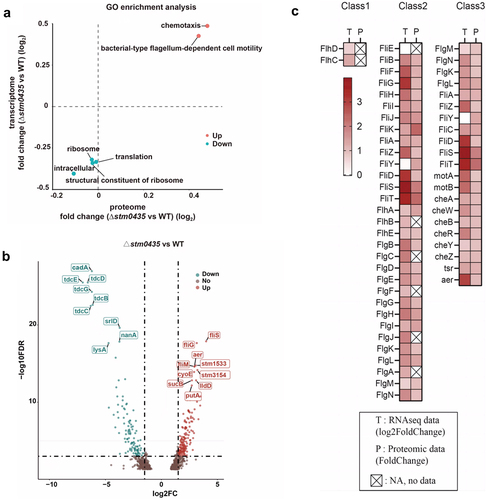
In addition, the tdcA-E operon, which affects the flagellar biosynthesis of Salmonella, was also influenced by STM0435. The result of transcriptomic data analysis showed that tdcA-E operon genes and flagellar genes exhibited opposite transcriptional directions in the Δstm0435 strain compared to the WT strain ().
STM0435 regulates flagella biogenesis by inhibiting the transcription of flhDC
To verify the integrative omics analysis results, the motility behaviour of WT and ∆stm0435 strains were assayed using semisolid agar (0.25%) plates. The results indicated that the ∆stm0435 strain exhibited an increase in motility compared to the WT strain, indicating that STM0435 inhibited cell motility in Salmonella (). Based on the inverse regulation between flagella synthesis and biofilm formation in Salmonella, we investigated the colony morphologies and colours of Salmonella by growing the strains on Congo red plates. After growth at 37 °C for one day, a distinct rdar morphotype was observed. The WT strain appeared red and rough, and ∆stm0435 colonies were white and smooth (). Thus, this phenotype suggests that STM0435 affects biofilm formation. The transcription levels of three flagellar genes (flhD, fliA, and fliC) in the WT and ∆stm0435 strains were detected by RT-PCR, using 16sRNA as an internal control. Consistent with the transcriptomic analysis, the transcription levels of flhD, fliA and fliC were markedly upregulated in the ∆stm0435 strain compared to those in the WT strain under the same culture conditions ().
Figure 3. STM0435 decrease the expression of flagellum and promote biofilm rdar morphotype formation. (a and b) The motility of the wild-type and ∆stm0435 strains was measured using 0.25% semisolid agar plates. (c) The biofilm phenotypes of wild-type and ∆stm0435 strains. The formation of rdar morphotype in bacteria grown for 24 hours on Congo red plates at 37°C. (d) The expression levels of flhD, fliA and fliC in the wild-type and ∆stm0435 strains were measured by qRT-PCR. Data are shown as the means and SEM, n = 3. ***, p < 0.001; **, p < 0.01; *, p < 0.05; n.s., p > 0.05.
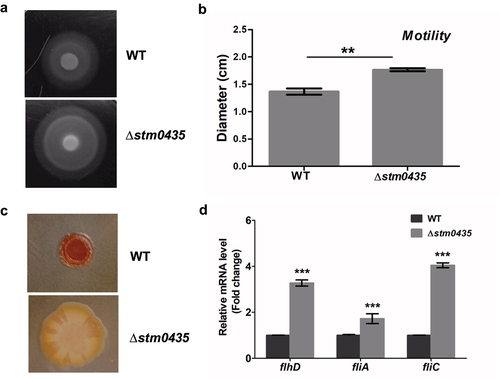
Previous studies have determined that the EAL-like protein STM1344 acts as a flagellar regulator. Its overexpression can completely inhibit flagellar synthesis in Salmonella [Citation28]. To demonstrate that STM0435 primarily affects the pathogenicity of Salmonella through its inhibition of flagella biogenesis, we constructed strains overexpressing STM1344 using wild-type and Δstm0435 Salmonella strains. The resulting WTstm1344 and Δstm0435pstm1344 strains completely lost their ability for flagellar synthesis. The results of HT-29 cell invasion assays revealed that WTpstm1344 and Δstm0435pstm1344 strains exhibited nearly identical invasion abilities (Figure S4A). Additionally, the release of LDH was higher in WTstm1344 compared to the Δstm0435pstm1344 strain, in contrast to the results in the WT and Δstm0435 groups (Figure S4B). Therefore, STM1344 impacts the HT-29 cell invasion rate and cell cytotoxicity of both WT and Δstm0435 strains, suggesting that the pathogenicity of Salmonella is mainly affected by STM0435 through its influence on flagellar assembly.
STM0435 binds c-di-GMP with medium affinity
The transcription and translation of flhDC genes are controlled by multiple factors, including QseB/C, H-NS, cAMP-CAP, RcsB, CsrA, and c-di-GMP [Citation29–32]. The YajQ family protein XC_3703 was identified as a novel c-di-GMP effector in the plant pathogen Xanthomonas campestris [Citation16]. Considering that STM0435 belongs to the YajQ family and is evolutionarily conserved in bacteria, we investigated whether STM0435 can bind c-di-GMP using an SPR assay. The changes in the response units indicated that c-di-GMP bound to STM0435 with a Kd of 8.383 µM (). This observed binding affinity is within the physiological range of a number of established c-di-GMP effector proteins, which range from ~ 0.1 to ~10 µM [Citation33]. Besides, STM0435 showed no detectable binding signals of GMP (Figure S6). Above all, these findings suggest that STM0435 specifically binds to c-di-GMP. A NanoDrop spectrophotometer was used to estimate the STM0435 protein concentration, which measures the absorbance of tyrosine, tryptophan, and cysteine at 280 nm. Therefore, the measurement of protein concentration made with this instrument is highly dependent on the content of these amino acids in the protein. There is only a single tryptophan residue in STM0435, so the concentration of the protein was analysed and modified using Protean software.
Structural insights of STM0435-c-di-GMP complex
We analysed the crystal structures of the STM0435 and STM0435-c-di-GMP complexes to assess how STM0435 binds to c-di-GMP. The STM0435 structure was solved using selenium single-wavelength anomalous diffraction at 1.48 Å resolution. The overall structure of STM0435 exhibited a conformation with two identical topological structure domains, which formed an elongated conformation. Each domain consists of a four-stranded antiparallel β-sheet, which is flanked by two α-helices on one side. The N-domain is composed of residues 1–100, and the C-domain includes residues 101–163 (). The structure of STM0435 is similar to the RNA-recognition motif (RRM) α/β sandwich fold, suggesting nucleotide binding activity [Citation34]. The structure of YajQ family members HI1034 from H. influenzae and XC_3703 from Xanthomonas campestris have been solved. STM0435 is the first YajQ family protein in Salmonella to be structurally analysed [Citation35,Citation36]. After performing sequence alignment of stm0435 genes from Proteus vulgaris, Yersinia pestis, Haemophilus influenzae, Pasteurella multocida, Escherichia coli, Shigella flexneri, Salmonella Typhimurium, Ralstonia solanacearum, Vibrio cholerae, Pseudomonas aeruginosa, and Xanthomonas campestris pv. Campestris, we found two highly spatially conserved (≥80%) domains in the STM0435 structure. Domain I consists of residues 1–5 and 131–140, while residues 29, 31, 56, and 60 are close to each other and comprise domain II (). These conserved domains may be the active centres of STM0435.
Figure 5. Interaction between STM0435 and c-di-GMP. (a) Schematic representation of the domain architecture of STM0435. The grey boxes indicate the N-terminal domain and the green box indicates the C-terminal domain. The conserved sites in the sequence are highlighted. (b) Overview of STM0435. The structure of STM0435 is shown in a cartoon. The N-terminal domain is shown in grey, and the C-terminal domain is shown in green. (c) Sequence conservation of STM0435 among 13 different bacterial strains. The overall structure of STM0435 is shown in surface mode. Highly conserved residues are coloured in purple and less-conserved residues are coloured in grey. (d) Overall view of STM0435-c-di-GMP complex, with c-di-GMP shown as orange sticks. (e and f) Structural comparison of the STM0435 and STM0435-c-di-GMP complexes, in pink and green, respectively, with c-di-GMP and Arg33/Arg137/Asp138 shown in the stick model. (e and f) The binding of c-di-GMP in STM0435 shifts the position of the Arg33/Arg137/Asp138 residues in STM0435, as indicated by the arrows.
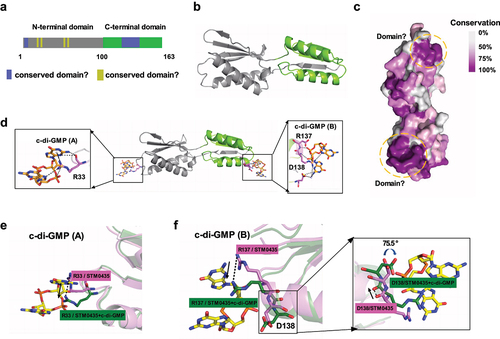
Previously, XC_3703 apoform crystals were soaked with c-di-GMP to try to crystallize the protein-ligand complex, but this strategy failed [Citation36]. To further investigate the interaction between this protein and c-di-GMP, we crystallized the STM0435-c-di-GMP complex. The crystals diffracted to about 2.28 Å resolution and belonged to space group P21 with unit cell parameters of a = 21.984, b = 51.697 Å, c = 126.267 Å, α= β= γ = 90° (Supplementary Table S4). In the STM0435-c-di-GMP complex structure, STM0435 binds to c-di-GMP(A) at the N-terminus and c-di-GMP(B) at the C-terminus (). c-di-GMP interacted with STM0435 mainly via a network of hydrogen bonds. The STM0435 and STM0435-c-di-GMP complex structures can be superimposed (Figure S7). Structural analysis showed that binding to c-di-GMP changes the location of amino acids R33, R137, and D138 in STM0435, suggesting that these residues may be key binding sites (). R137 and D138 were located in the C-terminal conserved region, and only arginine/lysine (basic amino acid) residues were observed at amino acid 33 in all bacteria with this gene, suggesting a uniform mechanism for c-di-GMP binding.
c-di-GMP acts as a positive regulator for STM0435-mediated regulation of flagellar synthesis
Mutagenesis was used to validate structural analyses. Strains overexpressing WT or mutated (R33H, R137H D138H and R33H R137H D138H) stm0435 were constructed (). We first measured the binding of c-di-GMP to STM0435 mutants using SPR. The STM0435 mutant proteins bound to c-di-GMP, as indicated by changes in response units, with a Kd of 15.2 µM for STM0435R33H, 25.3 µM for STM0435R137H D138H, and 33.18 µM for STM0435mut (representing STM0435R33H R137H D138H). This observed c-di-GMP binding affinity was lower than that of native STM0435, indicating that these three amino acid residues, R33, R137, and D138, are essential for its binding to c-di-GMP ( and S8). We also investigated the motility of Salmonella. The STM0435 overexpression strain displayed decreased motility compared to the ∆stm0435 strain, supporting the conclusion that STM0435 decreased flagellar synthesis in Salmonella (). Furthermore, the cellular levels of c-di-GMP were altered through the overexpression of STM1987 or STM4264, which encodes a DGC or PDE respectively. The results indicated that the ∆stm0435 strain exhibited increased motility compared to the WT strain, supporting the conclusion that STM0435 decreased flagellar synthesis in Salmonella. In the stm1987 overexpressed strains, a motility defect was exhibited compared to that of the stm1987 non-overexpressed strains, with a total loss of motility observed for WTpstm1987 (). While the overexpression of STM4264 eliminated the inhibitory effect of STM0435 on flagella synthesis in Salmonella (). This suggests that STM0435-mediated regulation of flagellar synthesis is enhanced by its c-di-GMP binding ability. Our work showed that c-di-GMP enhances STM0435-mediated flagellar synthesis regulation in Salmonella, and the key sites for the STM0435-c-di-GMP interaction are R33, R137, and D138.
Figure 6. c-di-GMP promotes the STM0435-mediated regulation of flagellar synthesis. (a) Schematic diagram of the mutant stm0435 (R33H, R137H, and D138H) strain. (b) SPR analysis for the binding of c-di-GMP to STM0435 protein. Solutions with series concentrations of c-di-GMP were injected into the STM0435-coated sensor chip chamber. The change of response units is shown. (c and d) Motility of WTpstm1987 and ∆stm0435pstm1987 strains. (e and f) Motility of WTpstm4264 and ∆stm0435pstm4264 strains. Data are shown as the mean ± SEM (n = 3). ***, p < 0.001; **, p < 0.01; *, p < 0.05; n.s., p > 0.05.
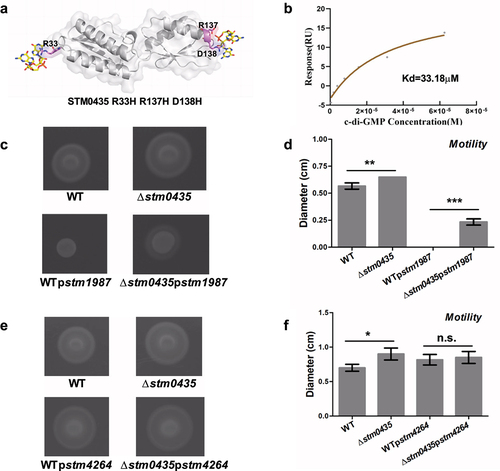
Discussion
Salmonella protein STM0435, which belongs to the YajQ protein family, has an unknown function. Some members of the YajQ family of Xanthomonas campestris, Pseudomonas aeruginosa and Stenotrophomonas maltophilia have been shown to affect the virulence of pathogens [Citation16,Citation17]. In this study, we found that STM0435 affected the pathogenicity of Salmonella, which is consistent with the conclusions of earlier studies. In general, the ability of Salmonella to cause infections in humans or animals depends on virulence genes that can evade and resist host defences [Citation37–39]. Salmonella pathogenicity islands (SPIs), fimbriae, flagella, and biofilm-related proteins can help bacteria to survive within the host. SPI-1 is necessary for the invasion of host cells and induction of macrophage apoptosis, whereas SPI-2 is required for systemic infection and replication within macrophages [Citation40,Citation41]. Fimbriae are filamentous surface structures that enable Salmonella to adhere to epithelial cells and improve its invasive ability [Citation42]. Flagella confer motility to Salmonella allowing movement towards host targets. Importantly, flagella also promote adherence and invasion to trigger adaptive and innate immune defence [Citation9]. How STM0435 affects pathogenicity of Salmonella has not yet been determined.
We further discovered that STM0435 influenced Salmonella pathogenicity by inhibiting the flagellar synthesis pathway. Flagellar synthesis is a highly orderly process, requiring more than 70 genes in 25 operons. These genes belong to three classes according to their transcriptional sequences [Citation43,Citation44]. Class I includes the flhDC operon, and FlhD and FlhC assemble into an FlhD4C2 heterohexamer. The FlhD4C2 complex acts as a transcription factor to activate the transcription of the class II genes [Citation45,Citation46]. Products of class II flagellar genes include flagellar basal structure, hook-basal body complex, and transcriptional activator FliA. Class III flagellar proteins are related to filament formation, flagellar rotation, and chemotaxis [Citation47–49]. Our group previously identified three flagellar regulators, STM1697, STM1344 (also known as YdiV) and YdiU, and all three target flhDC, the “master switch” of the flagellar synthesis pathway [Citation50–52]. Unlike the findings of our previous studies, STM0435 reduced the RNA levels of the class I flagellar protein FlhDC, which is the most critical regulatory protein in the flagellar synthesis pathway, due to its ability to bind to c-di-GMP. The tdcA-E operon is crucial for the degradation of L-serine and L-threonine in Salmonella. Previous studies have shown that the upregulation of this operon is associated with increased flagellar biosynthesis in Salmonella under oxygen-limited conditions [Citation53]. Interestingly, the tdcA-E genes and flagellar genes exhibited opposite transcriptional directions in the RNA-sequencing analysis results, which may contradict previous studies. In these studies, the tdcA mutation lead to a decrease in the transcription of some flagellar genes. When comparing the culture conditions of bacteria, we noticed that the cells were cultured to the log-phase and then subjected to an anaerobic shock. Although the bacteria we used were also in the log-phase, they did not undergo subsequent anaerobic shock treatment. These factors may have contributed to the inconsistent experimental results.
In our study, the regulation of flagellar synthesis by STM0435 was promoted by its interaction with c-di-GMP at the key sites of R33, R137, and D138. c-di-GMP enhances STM0435-mediated inhibition on flagellar synthesis in Salmonella. Interestingly, c-di-GMP interaction with XC_3703, a YajQ family protein in the plant pathogenic bacterium Xanthomonas campestris, suppressed the ability of XC_3703 to promote flagella synthesis [Citation16]. Flagellar regulation mediated by YajQ family proteins and c-di-GMP interactions may occur through different mechanisms in bacteria, while their overall function remained conserved. Based on our experimental results, we constructed a model of STM0435-mediated flagellar control in Salmonella that responds to c-di-GMP (). In the WT strain, the transcriptional levels of flagellum genes were inhibited by STM0435 protein. Binding of c-di-GMP to STM0435 enhances the inhibitory effect of the protein on flagellar gene transcription, thereby allowing normal expression of the flagellum. In the stm0435-knockout strain, increased expression of flagella was observed in Salmonella. This leads to pyroptosis in macrophages and stronger activation of the inflammasome, and the stm0435-knockout strain exhibits higher pathogenicity. Overall, this study identified a crucial factor influencing the pathogenesis of Salmonella.
Figure 7. Model of STM0435-mediated flagellar control in Salmonella that responsed to c-di-GMP. In the WT strain, the transcriptional levels of flagellar genes were decreased by STM0435 protein. Binding of c-di-GMP enhanced the inhibitory effect of STM0435 on flagellar gene transcription. Salmonella exhibits normal expression of the flagellum, causing pyroptosis in macrophages and inflammasome activation. The WT strain exhibited normal pathogenicity. In the stm0435-knockout strain, the absence of STM0435 resulted in increased flagellar gene expression in Salmonella. This leads to pyroptosis of macrophages and stronger activation of the inflammasome. Consequently, the stm0435-knockout strain exhibits higher pathogenicity.
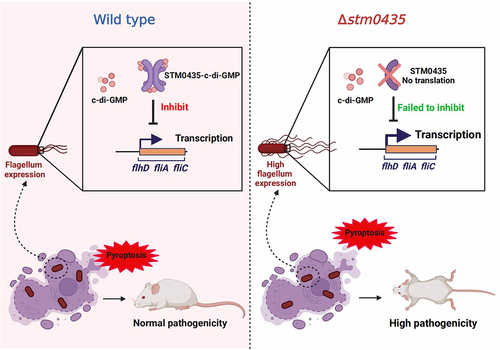
Author contributions
BL contributed to the conception and design of the study. WW conceived and drafted the manuscript. YD, RL, YY, and NS conducted the study. YD, MZ, and HJ performed structural analyses. RL, XL, and ZM performed the sequence analyses. QL, XL, and XG performed statistical analyses. All authors have contributed to the manuscript and approved the submitted version.
STM0435 Supplementary Material.docx
Download MS Word (1.6 MB)Disclosure statement
No potential conflict of interest was reported by the author(s).
Data Availability statement
The X-ray structures (coordinates and structure factor files) of STM0435 were submitted to the PDB under accession numbers 8K4I (Apo-STM0435) and 8K5Q (STM0435-c-di-GMP). Proteomic data were submitted to ProteomeX change via the PRIDE database (https://proteomecentral.proteomexchange.org/cgi/GetDataset?ID=PXD044133). The transcriptional data were submitted to the GEO database (https://www.ncbi.nlm.nih.gov/geo/query/acc.cgi?acc=GSE239303). Original data for this study can be found in the Mendeley Dataset (http://doi.org/10.17632/wvm6scwmdt.1).
Supplemental data
Supplemental data for this article can be accessed online at https://doi.org/10.1080/21505594.2024.2331265.
Additional information
Funding
References
- Smith SI, Seriki A, Ajayi A. Typhoidal and non-typhoidal Salmonella infections in Africa. Eur J Clin Microbiol Infect Dis. 2016;35(12):1913–14. doi: 10.1007/s10096-016-2760-3
- Galan JE. Salmonella typhimurium and inflammation: a pathogen-centric affair. Nat Rev Microbiol. 2021;19(11):716–25. doi: 10.1038/s41579-021-00561-4
- Whiley H, Gardner MG, Ross K. A review of Salmonella and squamates (lizards, snakes and amphisbians): implications for public health. Pathogens. 2017;6(3):6. doi: 10.3390/pathogens6030038
- Balasubramanian R, Im J, Lee JS, et al. The global burden and epidemiology of invasive non-typhoidal salmonella infections. Hum Vaccin Immunother. 2019;15(6):1421–6. doi: 10.1080/21645515.2018.1504717
- Pulford CV, Perez-Sepulveda BM, Canals R, et al. Stepwise evolution of Salmonella Typhimurium ST313 causing bloodstream infection in Africa. Nat Microbiol 2021; 6:327–338. 3 doi: 10.1038/s41564-020-00836-1
- Subramanian S, Kearns DB. Functional regulators of bacterial flagella. Annu Rev Microbiol. 2019;73(1):225–46. doi: 10.1146/annurev-micro-020518-115725
- Mahajan A, Currie CG, Mackie S, et al. An investigation of the expression and adhesin function of H7 flagella in the interaction of Escherichia coli O157: H7 with bovine intestinal epithelium. Cell Microbiol. 2009;11(1):121–37. doi: 10.1111/j.1462-5822.2008.01244.x
- Chaban B, Hughes HV, Beeby M. The flagellum in bacterial pathogens: for motility and a whole lot more. Semin Cell Dev Biol 2015;46:91–103. doi: 10.1016/j.semcdb.2015.10.032
- Haiko J, Westerlund-Wikstrom B. The role of the bacterial flagellum in adhesion and virulence. Biology. 2013;2(4):1242–67. doi: 10.3390/biology2041242
- Guttenplan SB, Kearns DB. Regulation of flagellar motility during biofilm formation. FEMS Microbiol Rev. 2013;37(6):849–71. doi: 10.1111/1574-6976.12018
- Wang F, Deng L, Huang F, et al. Flagellar Motility Is Critical for Salmonella enterica Serovar Typhimurium Biofilm Development. Front Microbiol 2020;11:1695. doi: 10.3389/fmicb.2020.01695
- Stewart MK, Cummings LA, Johnson ML, et al. Regulation of phenotypic heterogeneity permits Salmonella evasion of the host caspase-1 inflammatory response. Proc Natl Acad Sci USA. 2011;108(51):20742–7. doi: 10.1073/pnas.1108963108
- Sourjik V, Wingreen NS. Responding to chemical gradients: bacterial chemotaxis. Curr Opin Cell Biol. 2012;24(2):262–8. doi: 10.1016/j.ceb.2011.11.008
- Kanto S, Okino H, Aizawa S, et al. Amino acids responsible for flagellar shape are distributed in terminal regions of flagellin. J Mol Biol. 1991;219(3):471–80. doi: 10.1016/0022-2836(91)90187-B
- Miron S, Borza T, Saveanu C, et al. 1H, 13C and 15N resonance assignment of YajQ, a protein of unknown structure and function from Escherichia coli. J Biomol NMR 2001; 20:287–288. 3 10.1023/A:1011234905675
- An SQ, Caly DL, McCarthy Y, et al. Novel cyclic di-GMP effectors of the YajQ protein family control bacterial virulence. PLoS Pathog. 2014;10(10):e1004429. doi: 10.1371/journal.ppat.1004429
- Han S, Shen D, Wang YC, et al. A YajQ-LysR-like, cyclic di-GMP-dependent system regulating biosynthesis of an antifungal antibiotic in a crop-protecting bacterium, lysobacter enzymogenes. Mol Plant Pathol. 2020;21(2):218–29. doi: 10.1111/mpp.12890
- Zorraquino V, Garcia B, Latasa C, et al. Coordinated cyclic-di-GMP repression of Salmonella motility through YcgR and cellulose. J Bacteriol. 2013;195(3):417–28. doi: 10.1128/JB.01789-12
- Zogaj X, Nimtz M, Rohde M, et al. The multicellular morphotypes of Salmonella typhimurium and Escherichia coli produce cellulose as the second component of the extracellular matrix. Mol Microbiol. 2001;39(6):1452–63. doi: 10.1046/j.1365-2958.2001.02337.x
- Ryjenkov DA, Simm R, Romling U, et al. The PilZ domain is a receptor for the second messenger c-di-GMP: the PilZ domain protein YcgR controls motility in enterobacteria. J Biol Chem. 2006;281(41):30310–4. doi: 10.1074/jbc.C600179200
- Li S, Sun H, Li J, et al. Autoinducer-2 and bile salts induce c-di-GMP synthesis to repress the T3SS via a T3SS chaperone. Nat Commun. 2022;13(1):6684. doi: 10.1038/s41467-022-34607-9
- Datsenko KA, Wanner BL. One-step inactivation of chromosomal genes in Escherichia coli K-12 using PCR products. Proc Natl Acad Sci U S A. 2000;97(12):6640–5. doi: 10.1073/pnas.120163297
- Crosa JH, Brenner DJ, Ewing WH, et al. Molecular relationships among the salmonelleae. J Bacteriol. 1973;115(1):307–15. doi: 10.1128/jb.115.1.307-315.1973
- Criscuolo A, Issenhuth-Jeanjean S, Didelot X. et al. The speciation and hybridization history of the genus salmonella. Microb Genom 2019;5(8). doi: 10.1099/mgen.0.000284
- Fookes M, Schroeder GN, Langridge GC, et al. Salmonella bongori provides insights into the evolution of the salmonellae. PLoS Pathog. 2011;7(8):e1002191. doi: 10.1371/journal.ppat.1002191
- Takaya A, Yamamoto T, Tokoyoda K. Humoral immunity vs. Salmonella Front Immunol 2019;10:3155. doi: 10.3389/fimmu.2019.03155
- Ohl ME, Miller SI. Salmonella: a model for bacterial pathogenesis. Annu Rev Med. 2001;52(1):259–74. doi: 10.1146/annurev.med.52.1.259
- Yue Y, Wang W, Ma Y, et al. Cooperative regulation of flagellar synthesis by two EAL-Like proteins upon Salmonella entry into host cells. Microbiol Spectr. 2023;11(2):e0285922. doi: 10.1128/spectrum.02859-22
- Sperandio V, Torres AG, Kaper JB. Quorum sensing Escherichia coli regulators B and C (QseBC): a novel two-component regulatory system involved in the regulation of flagella and motility by quorum sensing in E. coli. Mol Microbiol. 2002;43(3):809–21. doi: 10.1046/j.1365-2958.2002.02803.x
- Soutourina O, Kolb A, Krin E, et al. Multiple control of flagellum biosynthesis in Escherichia coli: role of H-NS protein and the cyclic AMP-catabolite activator protein complex in transcription of the flhDC master operon. J Bacteriol. 1999;181(24):7500–8. doi: 10.1128/JB.181.24.7500-7508.1999
- Wei BL, Brun-Zinkernagel AM, Simecka JW, et al. Positive regulation of motility and flhDC expression by the RNA-binding protein CsrA of Escherichia coli. Mol Microbiol. 2001;40(1):245–56. doi: 10.1046/j.1365-2958.2001.02380.x
- Yuan X, Khokhani D, Wu X, et al. Cross-talk between a regulatory small RNA, cyclic-di-GMP signalling and flagellar regulator FlhDC for virulence and bacterial behaviours. Environ Microbiol. 2015;17(11):4745–63. doi: 10.1111/1462-2920.13029
- Pultz IS, Christen M, Kulasekara HD, et al. The response threshold of salmonella PilZ domain proteins is determined by their binding affinities for c-di-GMP. Mol Microbiol. 2012;86(6):1424–40. doi: 10.1111/mmi.12066
- Barret M, Egan F, Fargier E, et al. Genomic analysis of the type VI secretion systems in pseudomonas spp.: novel clusters and putative effectors uncovered. Microbiology (Reading). 2011;157(6):1726–39. doi: 10.1099/mic.0.048645-0
- Teplyakov A, Obmolova G, Bir N, et al. Crystal structure of the YajQ protein from Haemophilus influenzae reveals a tandem of RNP-like domains. J Struct Funct Genomics. 2003;4(1):1–9. doi: 10.1023/A:1024620416876
- Zhao Z, Wu Z, Zhang J. Crystal structure of the YajQ-family protein XC_3703 from Xanthomonas campestris pv. campestris. Acta Crystallogr F Struct Biol Commun. 2016;72(9):720–5. doi: 10.1107/S2053230X16013017
- Fabrega A, Vila J. Salmonella enterica serovar typhimurium skills to succeed in the host: virulence and regulation. Clin Microbiol Rev. 2013;26(2):308–41. doi: 10.1128/CMR.00066-12
- Foley SL, Johnson TJ, Ricke SC, et al. Salmonella pathogenicity and host adaptation in chicken-associated serovars. Microbiol Mol Biol Rev. 2013;77(4):582–607. doi: 10.1128/MMBR.00015-13
- Foley SL, Lynne AM, Nayak R Salmonella challenges: prevalence in swine and poultry and potential pathogenicity of such isolates. J Anim Sci 2008; 86:E149–62. suppl_14 doi: 10.2527/jas.2007-0464
- Leung KY, Siame BA, Snowball H, et al. Type VI secretion regulation: crosstalk and intracellular communication. Curr Opin Microbiol. 2011;14(1):9–15. doi: 10.1016/j.mib.2010.09.017
- Marcus SL, Brumell JH, Pfeifer CG, et al. Salmonella pathogenicity islands: big virulence in small packages. Microbes Infect. 2000;2(2):145–56. doi: 10.1016/S1286-4579(00)00273-2
- Collinson SK, Liu SL, Clouthier SC, et al. The location of four fimbrin-encoding genes, agfA, fimA, sefA and sefD, on the Salmonella enteritidis and/or S. typhimurium XbaI-BlnI genomic restriction maps. Gene. 1996;169(1):75–80. doi: 10.1016/0378-1119(95)00763-6
- Frye J, Karlinsey JE, Felise HR, et al. Identification of new flagellar genes of Salmonella enterica serovar Typhimurium. J Bacteriol. 2006;188(6):2233–43. doi: 10.1128/JB.188.6.2233-2243.2006
- Chilcott GS, Hughes KT. Coupling of flagellar gene expression to flagellar assembly in Salmonella enterica serovar typhimurium and Escherichia coli. Microbiol Mol Biol Rev. 2000;64(4):694–708. doi: 10.1128/MMBR.64.4.694-708.2000
- Yanagihara S, Iyoda S, Ohnishi K, et al. Structure and transcriptional control of the flagellar master operon of salmonella typhimurium. Genes Genet Syst 1999; 74:105–111. 3 doi: 10.1266/ggs.74.105
- Kutsukake K, Ohya Y, Iino T. Transcriptional analysis of the flagellar regulon of salmonella typhimurium. J Bacteriol. 1990;172(2):741–7. doi: 10.1128/jb.172.2.741-747.1990
- Ide N, Ikebe T, Kutsukake K Reevaluation of the promoter structure of the class 3 flagellar operons of Escherichia coli and Salmonella. Genes Genet Syst 1999; 74:113–116. S doi: 10.1266/ggs.74.113
- Karlinsey JE, Tanaka S, Bettenworth V, et al. Completion of the hook–basal body complex of the Salmonella typhimurium flagellum is coupled to FlgM secretion and fliC transcription. Mol Microbiol 2000; 37:1220–1231. 5 doi: 10.1046/j.1365-2958.2000.02081.x
- Tomoyasu T, Takaya A, Isogai E, et al. Turnover of FlhD and FlhC, master regulator proteins for salmonella flagellum biogenesis, by the ATP-dependent ClpXP protease. Mol Microbiol. 2003;48(2):443–52. doi: 10.1046/j.1365-2958.2003.03437.x
- Li B, Li N, Wang F, et al. Structural insight of a concentration-dependent mechanism by which YdiV inhibits Escherichia coli flagellum biogenesis and motility. Nucleic Acids Res. 2012;40(21):11073–85. doi: 10.1093/nar/gks869
- Li B, Yue Y, Yuan Z, et al. Salmonella STM1697 coordinates flagella biogenesis and virulence by restricting flagellar master protein FlhD4C2 from recruiting RNA polymerase. Nucleic Acids Res. 2017;45(17):9976–89. doi: 10.1093/nar/gkx656
- Ma Y, Yue Y, Jia H, et al. Switching off bacterial flagellar biogenesis by YdiU-mediated UMPylation of FlhDC. MBio 2022; 13:e0024922. 10.1128/mbio.00249-22
- Kim M, Lim S, Kim D, et al. A tdcA mutation reduces the invasive ability of Salmonella enterica serovar typhimurium. Mol Cells 2009; 28:389–395. doi: 10.1007/s10059-009-0133-9