ABSTRACT
Fusobacterium nucleatum (F. nucleatum) is closely correlated with tumorigenesis in colorectal cancer (CRC). We aimed to investigate the effects of host norepinephrine on the carcinogenicity of F. nucleatum in CRC and reveal the underlying mechanism. The results revealed that both norepinephrine and bacterial quorum sensing (QS) molecule auto-inducer-2 (AI-2) were positively associated with the progression of F. nucleatum related CRC (p < 0.01). In vitro studies, norepinephrine induced upregulation of QS-associated genes and promoted the virulence and proliferation of F. nucleatum. Moreover, chronic stress significantly increased the colon tumour burden of ApcMin/+ mice infected with F. nucleatum (p < 0.01), which was decreased by a catecholamine inhibitor (p < 0.001). Our findings suggest that stress-induced norepinephrine may promote the progression of F. nucleatum related CRC via bacterial QS signalling. These preliminary data provide a novel strategy for the management of pathogenic bacteria by targeting host hormones-bacterial QS inter-kingdom signalling.
Introduction
It has been estimated that over 100 trillion microbes reside in the human intestine as symbionts, and that they are mutually beneficial to the host [Citation1]. However, dysregulation of the gut microbiota is related to a variety of diseases, including colorectal cancer (CRC), which is the third leading cause of cancer-related deaths worldwide [Citation2]. An increasing body of evidence has demonstrated that F. nucleatum, previously recognized as a major constituent of the normal oral microbial community, is enriched in tumour tissues and stools of CRC patients compared to healthy controls [Citation3–6]. An ectopic accumulation of F. nucleatum leads to a proinflammatory microenvironment that promotes CRC cellular proliferation [Citation6,Citation7]. F. nucleatum adhere to and invade epithelial cells via fadA and promotes CRC by modulating E-cadherin/β-catenin signalling [Citation8–10]. Besides, F. nucleatum is capable of stimulating LC3-II expression, autophagic flux, and autophagosome synthesis in CRC cells which results in chemoresistance [Citation11]. As an opportunistic pathogen, F. nucleatum also exists in healthy gut [Citation12]. However, the mechanism that determines the pathogenicity of F. nucleatum are not entirely clear.
Interbacterial communication relies on the quorum sensing (QS) system, which utilizes signal molecules termed autoinducers (AIs) to regulate bacterial behaviour such as growth, virulence, and pathogenicity [Citation13,Citation14]. As a major molecule of QS, autoinducer-2 (AI-2), a furanosyl borate diester or tetrahydroxy furan [Citation15], has been reported to be recognized by many Gram-negative and Gram-positive bacteria [Citation16] and is closely correlated with the pathogenicity of a variety of bacteria in the gastrointestinal tract [Citation17]. A previous study showed that F. nucleatum derived AI-2 mediate the interaction between the F. nucleatum and periodontopathogens via biofilm formation, coaggregation and enhanced expression of adhesion molecules [Citation18]. AI-2 also regulating macrophage infiltration into tumour microenvironments and induces CRC occurrence [Citation19]. Our previous work showed that bacteria-derived AI-2 was associated with tumour immunity in CRC patients through inducing tumour-associated macrophages and reducing CD4/CD8 ratio in a TNFSF9-dependent manner [Citation20]. Recently, we found that gut microbiota-derived AI-2 could modulate lung inflammation through the gut-lung axis [Citation21]. Nevertheless, few studies have addressed the influence of host factors on bacterial QS [Citation22], which remains an important area of investigation in a variety of diseases caused by microbiota imbalance, such as CRC.
It has been well recognized that a variety of hormones are secreted into the gastrointestinal tract, and influence the gastroenteric function [Citation23,Citation24]. Chronic stress induces norepinephrine (NE) secretion from the enteric nervous system via the brain-gut axis [Citation25]. NE is partly produced in the enteric nervous system [Citation26] and reaches concentrations up to 50 ng/g in the colon [Citation27]. Gastrointestinal stress hormones can not only modulate intestinal motility, endocrine signalling, and immunity but also regulate the composition and behaviour of gut microbiota [Citation28]. It has been reported that NE can promote growth, virulence, and QS signalling in some anaerobic bacteria [Citation29–31]. Thus, we hypothesized that host NE might promote the progression of F. nucleatum related CRC involving bacterial QS system.
In the current study, we examined the abundance of F. nucleatum and concentrations of AI-2 and NE in 40 CRC patients at different stages. In vitro studies were used to investigate the role of NE in the pathogenicity of F. nucleatum, which was further explored in ApcMin/+ mouse model fed with F. nucleatum. Our results suggest that NE promotes the progression of F. nucleatum related CRC, possibly through bacterial QS signalling.
Materials and methods
Clinical sample collection
Tumors and adjacent normal tissues were collected from 40 patients with CRC who underwent surgery at the Affiliated Hospital of North Sichuan Medical College (Nanchong, China). Fecal samples were collected from CRC patients before surgery and from 40 healthy volunteers. All samples were processed as previously described [Citation32]. Clinicopathological characteristics of the samples are listed in Supplementary Table S1. CRC patients and healthy controls were age- and sex-matched. Informed consent was obtained from all participants, and the study was approved by the Institutional Review Board of the Affiliated Hospital of North Sichuan Medical College (Nanchong, China) (Approval ID: No. K2017027).
Quantification of F. nucleatum by qRT-PCR
qRT-PCR was used to calculate the relative abundance of F. nucleatum in tissues [Citation33,Citation34]. Total DNA was extracted from the tissues using the kit (DP304–03, TIANGEN, China) according to the manufacturer’s instructions and was used as the template for qRT-PCR. qRT-PCR using specific primers targeting F. nucleatum was performed to estimate the relative abundance of F. nucleatum. Gapdh was set as a reference gene to normalize the F. nucleatum abundance. The relative abundance of F. nucleatum was calculated using the 2−ΔΔCT method [Citation35]. Each experiment was repeated in triplicate.
Primers were listed as follows:
F. nucleatum:forward: 5’-ACCTAAGGGAGAAACAGAACCA-3;’
F. nucleatum:reverse: 5’-CCTGCCTTTAATTCATCTCCAT-3.’
Gapdh:forward: 5’-ACGGGAAGCTCACTGGCATGGCCTT-3;’
Gapdh:reverse:5’-CATGAGGTCCACCACCCTGTTGCTG-3.’
Analysis of Fusobacterium adhesin A (fadA) and luxS expression by qRT-PCR
The bacterial cultures with OD 600 = 0.7, were harvested for RNA extraction using TRIzol (R0016; Beyotime Biotechnology, China) according to the manufacturer’s protocol [Citation5]. qRT-PCR using specific primers targeting luxS or fadA were performed to estimate the level of luxS or fadA. Primers targeting F. nucleatum-specific 16S rRNA was set as a reference to normalize the the level of fadA. The relative level of luxS or fadA was calculated using the 2−ΔΔCT method [Citation35] and normalized to the F. nucleatum-specific 16S rRNA. To quantify the fadA or luxS gene copies, plasmid carrying fadA or luxS was serially diluted to 102–108 fadA or luxS copies/ml and used to generate standard curves for Ct values. The fadA or luxS gene copies in the clinical samples were calculated based on the standard curves. Each experiment was repeated in triplicate [Citation9].
Primers were listed as follows:
FadA:forward: 5’-GAAGAAAGAGCACAAGCTGA-3;’
FadA:reverse:5’- GCTTGAAGTCTTTGAGCTCT-3.’
LuxS:forward: 5’-AAGCCCCTTATGTGCGTATC-3;’
LuxS:reverse: 5’ -GGATAATCTCAGCGACTAAATGC-3;’
16s rRNA:forward: 5’-ACCTAAGGGAGAAACAGAACCA-3;’
16s rRNA:reverse: 5’-CCTGCCTTTAATTCATCTCCAT-3.’
Fluorescent in situ hybridization (FISH)
FISH was performed as previously described [Citation36]. The sequence of the FITC – labelled F. nucleatum - targeted probe, FUS664, was: 5ʹ - CGCAATACAGAGTTGAGCCCTGC − 3ʹ. To quantify F. nucleatum abundance, three blind observers assessed five random fields with 200 × magnification per sample and calculated the density of F. nucleatum.
Bacteria culture and drug treatment
The F. nucleatum used in our study was separated anaerobically from a right-sided colon tumour and a single isolate F. nucleatum (F01) was obtained in our previous study [Citation5]. The F01 strain was sequenced and submitted to GenBank (accession number: SUB1766768 Seq01 KX692281). The cells were cultured in fastidious anaerobic medium (FAB) supplemented with 10% foetal bovine serum in an incubator (containing 85% N2, 10% H2 and 5% CO2, 37°C) (Yuejin, Shanghai, China). Vibrio harveyi (V. harveyi) BB170 was obtained from Guangdong Culture Collection Center (Guangzhou, China) and cultured at 30°C in autoinducer bioassay (AB) medium. NE and propranolol were obtained from Solarbio, China, and used to treat F. nucleatum at 1 ng/ml.
Bacterial growth assays
A serial dilution series of F. nucleatum (10−5-10−8) in 1 ml medium was transferred to agar plates containing 10% defibrillated sheep blood. After incubation at 37°C for 48 h in an anaerobic chamber, bacterial colonies were counted manually.
AI-2 determination
V. harveyi strain BB170 was used to detect the concentration of AI-2, as previously described [Citation37,Citation38]. A standard solution of dihydroxy-2, 3-pentanedione (DPD) (710374–30–4, Omm Scientific Inc., USA), an AI-2 precursor, was prepared as previously described [Citation39]. Briefly, 10 µl of each sample was added to 90 µl of V. harveyi BB170 followed by incubation at 30°C for 4.5 hours in a shaker at 160 rpm. The concentration of AI-2 was calculated based on the bioluminescence value.
Mouse CRC models
All animal experiments and protocols were approved by the Animal Care and Animal Experiment Ethics Committee of the North Sichuan Medical College (Approval ID: No. 201702004). Six-week-old male C57BL/6-ApcMin/+ mice were purchased from Jiangsu JiCui Yaokang Biotechnology Co., Ltd. and raised in an SPF environment at the Animal Experiment Center of North Sichuan Medical College. Fifty C57BL/6-ApcMin/+ mice were randomly assigned into five groups: (1) Control; (2) F. nucleatum; (3) F. nucleatum + D-Ribose (D-Rib); (4) F. nucleatum + Stress; (5) F. nucleatum + Stress + α-methyl-p-tyrosine (AMPT). The C57BL/6-ApcMin/+ mice were fed by gavage with F. nucleatum at approximately 109 colony-forming units (cfu) of F. nucleatum in FAB medium daily for 8 consecutive weeks. D-Rib, an AI-2 inhibitor by competitively binding with the AI-2 receptor RbsB [Citation40], was diluted in drinking water (100 mM final concentration) and replaced every other day. On Day 40 of F. nucleatum administration, mice were subjected to a chronic stress paradigm. For the chronic stress model, mice administered F. nucleatum for 40 days daily were placed in a well-ventilated 50 mL centrifuge tube for 2 h per day for 20 consecutive days [Citation41]. Contemporaneously, AMPT (OKA, China, 100 mg/kg) was administered by intraperitoneal injection for 20 consecutive days.
Enzyme-linked immunosorbent assay (ELISA)
ELISA was used to detect the concentration of NE in human and mouse tumours [Citation42,Citation43]. Intestinal tumour tissues were mixed with PBS at a ratio of 1:9 (w/v). After homogenization and centrifugation (4°C, 5000 rpm, 20 min), the supernatant was collected and stored at −20°C. To determine the concentration of NE, 20 μl supernatant was subjected to mouse or human NE ELISA kit (Andygen, U.S. AD11667Hu or AD3383Mo). A standard curve was generated using the reference standard set provided by the kit. The samples were measured according to the manufacturer’s instructions accompanying the kit. The NE concentration was calculated using a standard curve.
Transcriptome sequencing
The total RNA was extracted from F. nucleatum with/without NE treatment. The mRNA was purified from total RNA for library preparation. The mRNA was randomly fragmented and used as the template for the synthesis of first strand cDNA. The second strand cDNA was synthesized with dNTP that containing dUTP instead of dTTP in the DNA polymerase I system. After adenylation and adaptor ligation, the second strand cDNA was fragmented, followed by purification with AMPure XP system (Beckman Coulter, Beverly, USA) to obtain fragments of 370 ~ 420 bp in length. Then these fragments were amplified by PCR and purified for quality control on the Agilent Bioanalyzer 2100. Next, the qualified index-coded samples were clustered using TruSeq PE Cluster Kit v3-cBot-HS (Illumina) according to the provided protocol. At last, the library sequencing was performed on an Illumina Novaseq platform and the paired-end reads of 150 bp were generated. For the transcriptome sequencing analysis, the data were blasted with the reference sequence (NZ_CP053468.1). The gene transcription level was evaluated with the RSEM package, and the up- or down-regulated genes were selected for functional analysis.
Results
F. nucleatum and AI-2 accumulated with the progression of CRC
We used Fluorescent in Situ Hybridization (FISH) analysis to label F. nucleatum in the tumours and adjacent normal tissues of patients with CRC. Consistent with previous findings, we observed an accumulation of F. nucleatum in tumour tissue and less accumulation in nearby non-neoplastic tissues (). To quantify the abundance of F. nucleatum in each sample, qRT-PCR analysis was performed, and the results were consistent with the FISH data (p < 0.0001) (). Furthermore, we noted that the abundance of F. nucleatum was higher in advanced stage 4 tumours than in the early clinical stages (stages 1 and 2, p < 0.01) (), suggesting that F. nucleatum may be correlated with CRC progression.
Figure 1. F. nucleatum increased along with CRC progression and AI-2 accumulation (a) Representative images of FISH detecting F. nucleatum in CRC and NC tissues. (b) qRT-PCR data showed the significantly increased abundance of F. nucleatum in CRC tissues (n = 40) compared with that in NC tissues (n = 40). The relative abundance of F. nucleatum in tissues was estimated by qRT-PCR using the specific primers targeting F. nucleatum, and primers targeting gapdh were used to confirm the equal quantity of tissues. (c) qRT-PCR data showed the increased abundance of F. nucleatum along with the cancer progression. (d) the concentration of AI-2 is significantly higher in CRC tissues compared with NC tissues, and (e) increased along with the cancer progression. (f) Pearson’s correlation analysis showed a positive correlation between F. nucleatum abundance and AI-2 concentration in tissues of CRC patients.
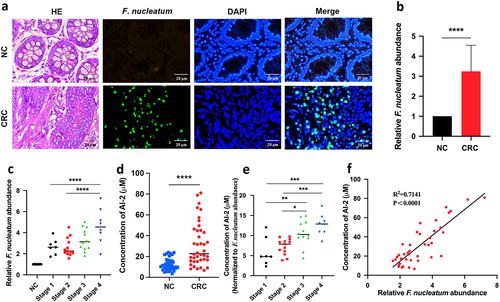
Next, we evaluated the concentration of AI-2, a major molecule in the bacterial QS system. Concomitant with increased F. nucleatum abundance in tumour tissue, the concentration of AI-2 in tumour tissue was significantly higher than that in adjacent normal tissue (p < 0.0001) () and continued to increase along with the clinical stage of cancer (). In addition, AI-2 concentration was significantly higher in stools from CRC patients (n = 40) than in healthy volunteers (n = 40, p < 0.0001) (Fig. S1A) and increased with CRC progression (Fig. S1B). Notably, Pearson’s correlation analysis showed a positive correlation between F. nucleatum abundance and AI-2 concentration in CRC tumour samples (p < 0.0001) ().
Taken together, these results suggest that the bacterial QS molecule AI-2 May be associated with the progression of F. nucleatum related CRC.
NE increased with the progression of F. nucleatum related CRC
We determined the concentrations of NE in both tumour and adjacent normal tissues using ELISA. The concentration of NE was significantly higher in the tumours (n = 40) than in the adjacent normal tissues (n = 40, p < 0.0001) ().
Figure 2. NE increased with the progression of F. nucleatum related CRC (a) the concentration of NE was significantly higher in CRC tissues (n = 40) compared with NC tissues (n = 40). (b) the concentration of NE in tumours increased along with the progression of CRC. (c, d) Pearson’s correlation analysis showed positive correlation between NE concentration and F. nucleatum abundance (c) or AI-2 concentration (d) in CRC tissues.
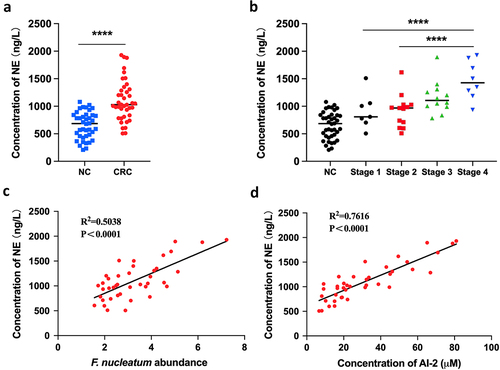
Notably, the concentration of NE increased with CRC progression (). Additionally, we found a positive correlation between NE concentration and F. nucleatum abundance (Pearson’s correlation, r = 0.5038; p < 0.0001), as well as between NE concentration and AI-2 concentration (Pearson’s correlation, r = 0.7616; p < 0.0001) (). These results suggest that host-derived NE may be associated with the progression of F. nucleatum related CRC, possibly involving bacterial AI-2 signalling.
NE promoted the virulence and proliferation of F. nucleatum with upregulation of QS system
To study the role of NE in F. nucleatum activity, we analysed the gene expression profile of F. nucleatum after NE treatment for 24 h. NE treatment induced a QS system that utilized autoinducers (AIs) (). The QS-associated genes ccfA, ABC.PE.S, and, to a lesser extent, oppA, blcC, and crp were among the upregulated genes in the volcano plot and heatmap (), which are consistent with previous studies [Citation44–47]. Moreover, DNA replication was observed in the transcriptomic analysis, which may suggest proliferation of F. nucleatum after NE treatment ().
Figure 3. NE promoted the virulence and proliferation of F. nucleatum with upregulation of QS system (a) F. nucleatum was treated with NE for 24 hours followed by subjection to RNA-Seq. KEGG analysis of pathway enrichment showed the induction of QS after NE treatment. (b) volcano plot of the transcriptome in NE group versus NC group. QS associated genes (ccfA, ABC.PE.S, oppA, crp, blcC) and DNA replication associated genes (holB, dnaG, dnaN) were labelled. (c) Heatmap of differentially regulated genes associated with QS and DNA replication. (d) Representative images of the colony formation of F. nucleatum subjected to NE and propranolol treatments. (e) statistics of F. nucleatum density based on the colonies in (d). (f) qRT-PCR data showed that fadA expression was increased significantly with treatment of NE, and decreased by propranolol. All experiments were repeated three times, and one-way analysis of variance was used.
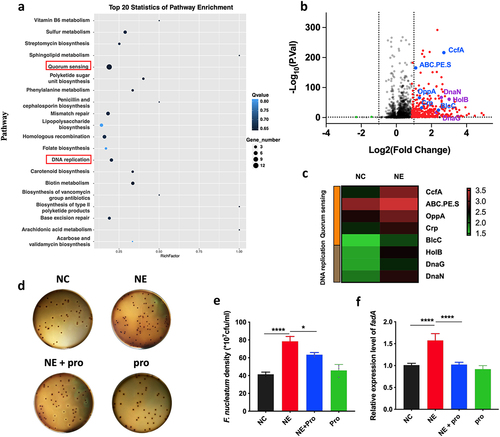
Therefore, we tested whether NE affected the growth and virulence of F. nucleatum. Using a colony formation assay, we found that NE promoted F. nucleatum proliferation (), whereas propranolol, an NE inhibitor, reversed the growth rate (p < 0.05) (). FadA of F. nucleatum, a virulence factor that regulates cell adhesion and invasive properties, was upregulated in response to NE treatment (Fig. S2), whereas its inhibitor decreased fadA expression (p < 0.05) (). These findings suggest that host NE may enhance the growth and virulence of F. nucleatum possibly through bacterial QS signalling.
AI-2 is essential for NE enhanced virulence and proliferation of F. nucleatum
In addition, the level of AI-2 was significantly increased by NE (p < 0.05) (). Next, we treated F. nucleatum with D-Rib, an AI-2 competitive inhibitor, in combination with NE. The results showed that D-Rib inhibited the growth of F. nucleatum induced by NE (p < 0.05) (). Moreover, D-Rib significantly inhibited fadA expression of F. nucleatum induced by NE in F. nucleatum (p < 0.05) (). These results suggest that AI-2 signalling is required for the growth and virulence of F. nucleatum induced by NE.
Figure 4. AI-2 is essential for NE enhanced virulence and proliferation of F. nucleatum (a) statistics of AI-2 concentration in the supernatant of F. nucleatum culture induced by NE. (b) Representative images illustrating the colony formation of F. nucleatum subjected to NE treatments with and without AI-2 inhibitor. (c) statistics of F. nucleatum density based on the colonies in (b). (d) qRT-PCR data showed that D-Rib significantly inhibited the FadA expression of F. nucleatum induced by NE. All experiments were repeated three times, and paired t test was used.
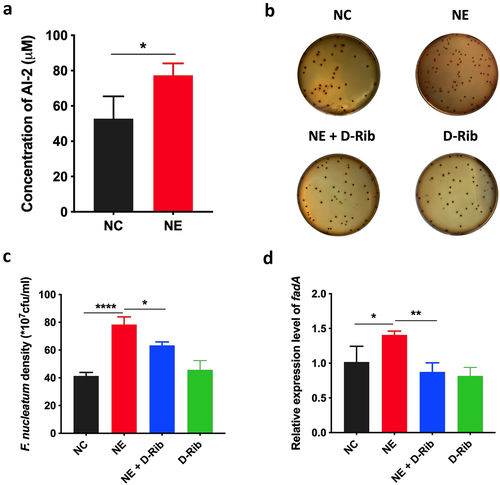
Stress accelerated the progression of colorectal tumor in ApcMin/+ mice infected with F. nucleatum
Our previous studies have demonstrated that F. nucleatum administration can potentiate colorectal tumorigenesis in mice [Citation48], and that AI-2 concentration was increased in both colorectal tissue and stool of CRC patients [Citation20]. To investigate the role of AI-2 in F. nucleatum related CRC in vivo, we treated F. nucleatum fed ApcMin/+ mice with D-Rib, an AI-2 inhibitor. The tumour number and size in the intestine were lower in D-Rib-treated mice than in F. nucleatum group (p < 0.01, p < 0.05, respectively), suggesting that AI-2 signalling is required to enhance F. nucleatum induced colorectal tumours in vivo ().
Figure 5. Stress promoted the progression of colorectal tumour in ApcMin/+ mice fed with F. nucleatum (a) Representative images showing the tumor formation and F. nucleatum invasion in tumours. (b, c) quantification of tumour number and size in each group. D-Rib significantly decreased the tumour number and size in mice fed with F. nucleatum. the tumour size in F. nucleatum + stress group was significantly higher than in F. nucleatum group. Both the tumour number and size were decreased significantly by catecholamine inhibitor AMPT. (d) qRT-PCR analysis highlighting F. nucleatum burden in tumours. AMPT significantly decreased the abundance of F. nucleatum induced by host stress. (e) F. nucleatum administration significantly increased AI-2 level in tumours, which was inhibited by D-Rib or AMPT.
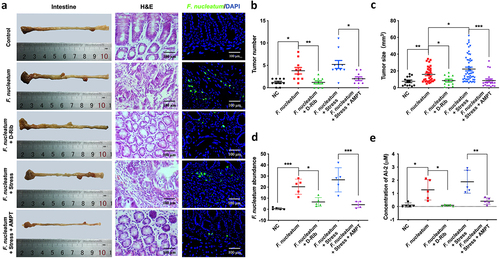
To investigate the role of NE in F. nucleatum related colorectal tumours in vivo, we used a mouse model in which NE production was induced by subjecting the mice to chronic stress. The concentration of NE significantly increased in the tumours of mice subjected to stress (p < 0.05), which was counteracted by AMPT treatment (p < 0.01) (Fig. S3). Based on F. nucleatum administration, chronic stress accelerated intestinal tumour formation, with increased tumour number (although not significant) and size (p < 0.01) (). Moreover, both the tumour number and size were significantly decreased by the NE inhibitor AMPT (p < 0.05) (). Additionally, AMPT significantly decreased the abundance of F. nucleatum induced by host stress (p < 0.001) (). In this mouse model, we also evaluated the concentration of AI-2, showing that F. nucleatum fed mice showed higher AI-2 level, which was rescued by D-Rib or AMPT ().
Discussion
Previous studies have reported that F. nucleatum is enriched in tumour tissues and stools of CRC patients and is positively correlated with tumour stage [Citation3]. However, as an opportunistic pathogen, F. nucleatum also exists in the healthy gut, raising the question of how F. nucleatum pathogenicity in CRC is regulated by host factors. In the present study, we found that the stress hormone NE can support the proliferation and virulence of F. nucleatum in vitro and mainly drive an increase in tumour size in APCMin/+ mice fed F. nucleatum, which was counteracted by the catecholamine inhibitor AMPT in vivo and in vitro. These results suggest that the host stress hormone NE promotes the progression of F. nucleatum related CRC.
It has been shown that F. nucleatum adhere to and invade epithelial cells via virulence factors, including fadA, Fusobacterium autotransporter protein 2 (Fap2), and Fusobacterial outer membrane protein A (FomA) [Citation9,Citation49–51]. Here, we found that NE promoted the growth of F. nucleatum in vitro and induced fadA expression. These effects were inhibited by an AI-2 inhibitor, suggesting that the pathogenicity of F. nucleatum is regulated by QS signalling.
Although it has been reported that stress promotes CRC progression [Citation52], the underlying mechanism remains to be elucidated, especially in F. nucleatum related CRCs. Our results revealed a positive correlation between NE concentrations and AI-2 levels in human CRC tissues. We further analysed the gene expression profile of F. nucleatum after NE treatment and found that QS-associated genes, such as ccfA and ABC. PE. S, were upregulated. These interesting findings indicate, for the first time, that host stress may promote the progression of F. nucleatum related CRC through NE/AI-2 signalling.
To our knowledge, this is the first study to address the mechanism of F. nucleatum related CRC with a focus on the brain-gut axis and the inter-kingdom signalling communication between the host neuroendocrine system and bacteria. Our results suggest a new strategy for the management of F. nucleatum related CRC by targeting inter-kingdom signalling between host stress hormones and the bacterial QS system. This strategy may also benefit other diseases associated with brain-gut axis dysfunction or gut microbiota dysbiosis, such as inflammatory bowel disease (IBD) and irritable bowel syndrome (IBS).
Ethics statement
This study was approved by the Institutional Review Board of the Affiliated Hospital of North Sichuan Medical College (Nanchong, China), and informed consent was obtained from all participants prior to enrolment (Approval ID: No. K2017027).
All animal experiments and protocols were approved by the Animal Care and Animal Experiment Ethics Committee of the North Sichuan Medical College (Approval ID: No. 201702004). Six-week-old male C57BL/6-ApcMin/+ mice were purchased from Jiangsu JiCui Yaokang Biotechnology Co., Ltd. and raised in an SPF environment at the Animal Experiment Center of North Sichuan Medical College.
Consent for publication
The manuscript is approved by all the participants.
Author contributions
The work reported in this paper was performed by the authors unless clearly specified in the text. XHD, ZZT, and LY conducted the experiments and analysed the data. XHD, ZZT, LY and MZ drafted the manuscript. MZ and XSF refined and approved the final manuscript. XSF conceived and designed the study. Other co-authors performed helpful work. All authors have contributed to the manuscript and approved the submitted version.
Fig S4.tiff
Download TIFF Image (606.5 KB)Fig S1.tif
Download TIFF Image (14.1 MB)Fig S2.tiff
Download TIFF Image (84.6 KB)Supplementary table S1.docx
Download MS Word (19 KB)Fig S3.tif
Download TIFF Image (3.6 MB)Disclosure statement
No potential conflict of interest was reported by the author(s).
Data Availability statement
The authors confirm that the data supporting the findings of this study are available in the article and its supplementary materials.
Supplemental data
Supplemental data for this article can be accessed online at https://doi.org/10.1080/21505594.2024.2350904.
Additional information
Funding
References
- Larroya-García A, Navas-Carrillo D, Orenes-Piñero E. Impact of gut microbiota on neurological diseases: diet composition and novel treatments. Crit Rev Food Sci Nutr. 2018;59(19):3102–11. doi: 10.1080/10408398.2018.1484340
- Siegel RL, Miller KD, Jemal A. Cancer statistics, 2019. Ca A Cancer J Clinicians. 2019;69(1):7–34. doi: 10.3322/caac.21551
- Wu J, Li Q, Fu X. Fusobacterium nucleatum contributes to the carcinogenesis of colorectal cancer by inducing inflammation and suppressing Host immunity. Transl Oncol. 2019;12(6):846–851. doi: 10.1016/j.tranon.2019.03.003
- Chen T, Li Q, Zhang X, et al. TOX expression decreases with progression of colorectal cancers and is associated with CD4 T-cell density and Fusobacterium nucleatum infection. Hum Pathol. 2018;79:93–101. doi: 10.1016/j.humpath.2018.05.008
- Chen Y, Peng Y, Yu J, et al. Invasive Fusobacterium nucleatum activates beta-catenin signaling in colorectal cancer via a TLR4/P-PAK1 cascade. Oncotarget. 2017;8(19):31802–31814. doi: 10.18632/oncotarget.15992
- Kostic A, Chun E, Robertson L, et al. Fusobacterium nucleatum potentiates intestinal tumorigenesis and modulates the tumor-immune microenvironment. Cell Host Microbe. 2013;14(2):207–215. doi: 10.1016/j.chom.2013.07.007
- Yang Y, Weng W, Peng J, et al. Fusobacterium nucleatum increases proliferation of colorectal cancer cells and tumor development in mice by activating toll-like receptor 4 signaling to nuclear Factor−κB, and up-regulating expression of MicroRNA-21. Gastroenterology. 2017;152(4):851–866.e24. doi: 10.1053/j.gastro.2016.11.018
- Dadashi M, Hajikhani B, Faghihloo E, et al. Proliferative effect of FadA recombinant protein from Fusobacterium nucleatum on SW480 colorectal cancer cell line. Infect Disord Drug Targets. 2021;21(4):623–628. doi: 10.2174/1871526520666200720113004
- Rubinstein MR, Wang X, Liu W, et al. Fusobacterium nucleatum promotes colorectal carcinogenesis by modulating E-cadherin/β-catenin signaling via its FadA adhesin. Cell Host Microbe. 2013;14(2):195–206. doi: 10.1016/j.chom.2013.07.012
- Rubinstein MR, Baik JE, Lagana SM, et al. Fusobacterium nucleatum promotes colorectal cancer by inducing Wnt/β-catenin modulator annexin A1. EMBO Rep. 2019;20(4):e47638. doi: 10.15252/embr.201847638
- Yu T, Guo F, Yu Y, et al. Fusobacterium nucleatum promotes chemoresistance to colorectal cancer by modulating autophagy. Cell. 2017;170(3):548–563.e16. doi: 10.1016/j.cell.2017.07.008
- Bashir A, Miskeen AY, Bhat A, et al. Fusobacterium nucleatum: an emerging bug in colorectal tumorigenesis. Eur J Cancer Prev. 2015;24(5):373–385. doi: 10.1097/CEJ.0000000000000116
- Thompson JA, Oliveira R, Djukovic A, et al. Manipulation of the Quorum Sensing Signal AI-2 affects the Antibiotic-Treated Gut Microbiota. Cell Rep. 2015;10(11):1861–1871. doi: 10.1016/j.celrep.2015.02.049
- Fuqua WC, Winans SC, Greenberg EP. Quorum sensing in bacteria: the LuxR-LuxI family of cell density-responsive transcriptional regulators. J Bacteriol. 1994;176(2):269–275. doi: 10.1128/jb.176.2.269-275.1994
- Rettner RE, Saier MH, Jr. The autoinducer-2 exporter superfamily. J Microb Physiol. 2010;18(4):195–205. doi: 10.1159/000316420
- Wang Y, Wang Y, Sun L, et al. The LuxS/AI-2 system of streptococcus suis. Appl Microbiol Biotechnol. 2018;102(17):7231–7238. doi: 10.1007/s00253-018-9170-7
- Li Q, Ren Y, Fu X. Inter-kingdom signaling between gut microbiota and their host. Cell Mol Life Sci. 2019;76(12):2383–2389. doi: 10.1007/s00018-019-03076-7
- Jang Y, Choi Y-J, Lee S-H, et al. Autoinducer 2 of Fusobacterium nucleatum as a target molecule to inhibit biofilm formation of periodontopathogens. Arch Oral Biol. 2013;58(1):17–27. doi: 10.1016/j.archoralbio.2012.04.016
- Wu J, Wang Y, Jiang Z. Fusobacterium nucleatumImmune induction identified by TMT proteomics analysis in autoinducer-2 treated macrophages. Expert Rev Proteomics. 2020;17(2):175–185. doi: 10.1080/14789450.2020.1738223
- Li Q, Peng W, Wu J, et al. Autoinducer-2 of gut microbiota, a potential novel marker for human colorectal cancer, is associated with the activation of TNFSF9 signaling in macrophages. Oncoimmunology. 2019;8(10):e1626192. doi: 10.1080/2162402X.2019.1626192
- Zeng X, Yue H, Zhang L, et al. Gut microbiota-derived autoinducer-2 regulates lung inflammation through the gut-lung axis. Int Immunopharmacol. 2023;124:110971. doi: 10.1016/j.intimp.2023.110971
- Dicks LMT. How does quorum sensing of intestinal bacteria affect our health and mental status? Microorganisms. 2022;10(10):1969. doi: 10.3390/microorganisms10101969
- Martin AM, Sun EW, Keating DJ. Mechanisms controlling hormone secretion in human gut and its relevance to metabolism. J Endocrinol. 2020;244(1):R1–R15. doi: 10.1530/JOE-19-0399
- Rehfeld JF. Gastrointestinal hormones and their targets. Adv Exp Med Biol. 2014;817:157–175.
- Asano Y, Hiramoto T, Nishino R, et al. Critical role of gut microbiota in the production of biologically active, free catecholamines in the gut lumen of mice. Am J Physiol Gastrointest Liver Physiol. 2012;303(11):G1288–G1295. doi: 10.1152/ajpgi.00341.2012
- Moreira CG, Russell, R., Mishra, A.A., et al. Bacterial adrenergic sensors regulate virulence of enteric pathogens in the gut. MBio. 2016;7(3):e00826–16.
- Boukerb AM, Cambronel M, Rodrigues S, et al. Inter-kingdom signaling of stress hormones: sensing, transport and modulation of bacterial physiology. Front Microbiol. 2021;12:690942. doi: 10.3389/fmicb.2021.690942
- Mittal R, Debs LH, Patel AP, et al. Neurotransmitters: the Critical Modulators Regulating Gut–brain axis. J Cell Physiol. 2017;232(9):2359–2372. doi: 10.1002/jcp.25518
- Roberts A, Matthews JB, Socransky SS, et al. Stress and the periodontal diseases: effects of catecholamines on the growth of periodontal bacteria in vitro. Oral Microbiol Immunol. 2002;17(5):296–303. doi: 10.1034/j.1399-302X.2002.170506.x
- Kinney KS, Austin CE, Morton DS, et al. Norepinephrine as a growth stimulating factor in bacteria—mechanistic studies. Life Sci. 2000;67(25):3075–3085. doi: 10.1016/S0024-3205(00)00891-2
- Lyte M, Frank CD, Green BT. Production of an autoinducer of growth by norepinephrine cultured Escherichia coli O157: H7. FEMS Microbiol Lett. 1996;139(2–3):155–159. doi: 10.1111/j.1574-6968.1996.tb08196.x
- Raut N, Joel S, Pasini P, et al. Bacterial autoinducer-2 detection via an engineered quorum sensing protein. Anal Chem. 2015;87(5):2608. doi: 10.1021/ac504172f
- Chen Y, Chen Y, Zhang J, et al. Fusobacterium nucleatum promotes metastasis in colorectal cancer by activating autophagy signaling via the Upregulation of CARD3 Expression. Theranostics. 2020;10(1):323–339. doi: 10.7150/thno.38870
- Parhi L, Alon-Maimon T, Sol A, et al. Breast cancer colonization by Fusobacterium nucleatum accelerates tumor growth and metastatic progression. Nat Commun. 2020;11(1):3259. doi: 10.1038/s41467-020-16967-2
- Schmittgen T, Livak K. Analyzing real-time PCR data by the comparative C(T) method. Nat Protoc. 2008;3(6):1101–1108. doi: 10.1038/nprot.2008.73
- Yu YN, Yu T-C, Zhao H-J, et al. Berberine may rescue Fusobacterium nucleatum-induced colorectal tumorigenesis by modulating the tumor microenvironment. Oncotarget. 2015;6(31):32013–32026. doi: 10.18632/oncotarget.5166
- Bassler BL, Greenberg EP, Stevens AM. Cross-species induction of luminescence in the quorum-sensing bacterium vibrio harveyi. J Bacteriol. 1997;179(12):4043–4045. doi: 10.1128/jb.179.12.4043-4045.1997
- Stephens K, Pozo M, Tsao C-Y, et al. Bacterial co-culture with cell signaling translator and growth controller modules for autonomously regulated culture composition. Nat Commun. 2019;10(1):4129. doi: 10.1038/s41467-019-12027-6
- Song J, Qin Q, Li T, et al. Impact of carbohydrates on autoinducer-2 secretion of Bifidobacterium longum subsp. longum BBMN68. Lett Appl Microbiol. 2018;66(4):340–346. doi: 10.1111/lam.12854
- James D, Shao H, Lamont RJ, et al. The Actinobacillus actinomycetemcomitans ribose binding protein RbsB interacts with cognate and heterologous autoinducer 2 signals. Infect Immun. 2006;74(7):4021–4029. doi: 10.1128/IAI.01741-05
- Liu J, Deng G-H, Zhang J, et al. The effect of chronic stress on anti-angiogenesis of sunitinib in colorectal cancer models. Psychoneuroendocrinology. 2015;52:130–142. doi: 10.1016/j.psyneuen.2014.11.008
- Perego M, Tyurin VA, Tyurina YY, et al. Reactivation of dormant tumor cells by modified lipids derived from stress-activated neutrophils. Sci Transl Med. 2020;12(572):eabb5817. doi: 10.1126/scitranslmed.abb5817
- Weir G, Ramage LE, Akyol M, et al. Substantial metabolic activity of human brown adipose tissue during warm conditions and cold-induced lipolysis of local triglycerides. Cell Metab. 2018;27(6):1348–1355.e4. doi: 10.1016/j.cmet.2018.04.020
- Khan SR, Farrand SK. The BlcC (AttM) lactonase of agrobacterium tumefaciens does not quench the quorum-sensing system that regulates Ti plasmid conjugative transfer. J Bacteriol. 2009;191(4):1320–1329. doi: 10.1128/JB.01304-08
- Kurnia D, Rachmawati P, Satari MH. Antibacterial of dibenzo-p-dioxi-2,8-dicarboxylic acid against pathogenic oral bacteria E. faecalis ATCC 29212 peptide pheromones: quorum sensing of in vitro and in silico study. Drug Des Devel Ther. 2020;14:3079–3086.
- Laganenka L, Sander T, Lagonenko A, et al. Quorum sensing and metabolic state of the Host Control Lysogeny-Lysis switch of bacteriophage T1. MBio. 2019;10(5):e01884–19. doi: 10.1128/mBio.01884-19
- Yang M, Meng F, Gu W, et al. Influence of polysaccharides from polygonatum kingianum on Short-Chain Fatty Acid Production and quorum sensing in lactobacillus faecis. Front Microbiol. 2021;12:758870. doi: 10.3389/fmicb.2021.758870
- Wu Y, Wu J, Chen T, et al. Fusobacterium nucleatum potentiates intestinal tumorigenesis in mice via a toll-like receptor 4/p21-activated kinase 1 cascade. Dig Dis Sci. 2018;63(5):1210–1218. doi: 10.1007/s10620-018-4999-2
- Abed J, Emgård JM, Zamir G, et al. Fap2 mediates Fusobacterium nucleatum colorectal adenocarcinoma enrichment by binding to tumor-expressed gal-GalNAc. Cell Host Microbe. 2016;20(2):215–225. doi: 10.1016/j.chom.2016.07.006
- Gur C, Ibrahim Y, Isaacson B, et al. Binding of the Fap2 protein of Fusobacterium nucleatum to human inhibitory receptor TIGIT protects tumors from immune cell attack. Immunity. 2015;42(2):344–355. doi: 10.1016/j.immuni.2015.01.010
- Nakagaki H, Sekine S, Terao Y, et al. Fusobacterium nucleatum envelope protein FomA is immunogenic and binds to the salivary statherin-derived peptide. Infect Immun. 2010;78(3):1185–1192. doi: 10.1128/IAI.01224-09
- Bandapalli OR, Xu J, Liang F, et al. Effect of chronic psychological stress on liver metastasis of colon cancer in mice. PLOS ONE. 2015;10(10):e0139978. doi: 10.1371/journal.pone.0139978