ABSTRACT
Live-attenuated rotavirus vaccine has shown low protection in underdeveloped or developing countries. However, the inactivated rotavirus vaccine may have the potential to overcome some of these challenges. In the present study, the immunogenicity and protective efficacy of a bivalent inactivated rotavirus vaccine by parenteral administration were elevated in a neonatal rhesus monkey model. A bivalent inactivated rotavirus vaccine containing G1P[8] (ZTR-68 strain) and G9P[8] (ZTR-18 strain) was administered to pregnant rhesus monkeys twice at an interval of 14 days. Neutralizing antibodies against RV strains ZTR-68, ZTR-18, SA11, WA, UK, and Gottfried emerged in pregnant rhesus monkeys and were transplacentally transmitted to the offspring. In the vaccine group, clinical symptoms of diarrhea, viral load in the gut tissue and histopathological changes were significantly reduced in the neonatal rhesus monkeys following oral challenge with the SA11 strain.
Introduction
Rotavirus, a genus of the Reoviridae family, is generally considered to be the main pathogen responsible for severe gastroenteritis among children younger than 5 years of age worldwide.Citation1,Citation2 Rotaviruses of species A comprise multiple G and P genotypes, and these are determined by the outer capsid proteins VP7 (for glycoprotein) and VP4 (for protease susceptibility), respectively. At present, at least 57 P and 41 G genotypes have been classified for rotaviruses, with the G1, G2, G3, and G4 genotypes being responsible for most cases of rotavirus gastroenteritis worldwide.Citation3,Citation4 Studies have shown that G9 rotaviruses have become more prevalent in the last few years.Citation5–7 To date, no specific drugs for the treatment of RV infections have been identified.Citation8 Therefore, the development of an effective vaccine against rotavirus gastroenteritis is an urgent public health priority.Citation9–13
Although several live attenuated virus vaccines against rotaviruses have been approved in many countries, rotavirus-induced severe diarrhea still caused 128 500 deaths and 258 173 300 episodes of diarrhea among children younger than 5 years in 2016 worldwide.Citation14–17 Due to the influence of maternal antibodies and the intestinal microbial environment, the vaccine effect is poor in some low-income countries.Citation18–21 In addition, rare and serious adverse reactions, such as intussusception and Kawasaki disease, may also occur.Citation22–25
To improve the safety and efficacy of rotavirus vaccines, a strategy currently being investigated is vaccination via parenteral administration of inactivated rotavirus.Citation26–30 Parenteral immunity can produce a strong serum antibody reaction, and serum antibodies can neutralize the virus to mediate their protective effects.Citation31,Citation32 Epidemiologic and clinical studies demonstrate that homotypic and heterotypic protective immunity are generated after RV infection or immunization.Citation33–36 However, with the increasing emergence of new rotavirus G and P types, the monovalent vaccine may not confer cross protection against non-vaccine strains. Heterotypic protection of the rotavirus vaccine is important to effectively reduce the rotavirus disease burden.Citation37 Therefore, multivalent vaccines may be necessary for the future development of RV vaccines.
In the current study, we prepared a bivalent inactivated rotavirus vaccine containing two human rotavirus strains, ZTR-68 (G1P[8]) and ZTR-18 (G9P[8]), that were isolated from the fecal specimens of two children in China. To evaluate bivalent inactivated rotavirus, we immunized pregnant monkeys and challenged their babies with the SA11 strain. The results showed that the bivalent inactivated rotavirus vaccine stimulated humoral immunity in pregnant monkeys and that antibody produced by the pregnant monkeys induced protection against diarrhea in their offspring.
Materials and methods
Virus and cells
Six rotavirus strains were used for the viral cross neutralizing antibody assay, namely, Wa (G1P[8]), SA11 (G3P[2]), UK (G6P[11]), Gottfried (G4P[6]), ZTR-68 (G1P[8]) and ZTR-18 (G9P[8]). They were propagated in green monkey kidney MA104 cells. Prior to infection, the rotavirus was activated with 20 μg/ml trypsin (Gibco, 15090–046) and 600 μg/ml CaCl2 (Sigma, C7902) at 37°C for 45 min. After being absorbed for 90 min at 37°C, the rotavirus was cultured for 48 ~ 72 h in serum-free MEM containing 1 µg/ml trypsin at 37°C. Infected cultures were harvested by freezing at −20°C and thawing at room temperature, followed by centrifugation at 8873 g for 20 min. The virus titers were determined by a plaque assay with MA104 cells.
MA104 cells were maintained in MEM supplemented with 10% fetal bovine serum (Gibco, 16000–044) and grown to a confluent monolayer in roller bottles (850 cm2) (Corning, 430849). Vero cells were maintained in 8% fetal bovine serum.
Detection of RV antigen by ELISA
The wells of the ELISA plate were coated with a goat anti-Rotavirus polyclonal antibody (Millipore, AB1129) diluted at 1:1000 (v/v) in 0.05 M carbonate-bicarbonate buffer (pH9.6) overnight at 4°C. Washing three times with 50 mM PBS (pH7.0) containing 0.05% (v/v) Tween-20 (PBS-T), the plates were blocked with 3% (w/v) BSA (Biosharp, BS043D) in PBS at 37°C for 2 h. The antigen samples (100ul/well) were serially diluted (dilution range from 1:2 to 1:4096) and incubated at 37°C for 1 h. After washing, a rabbit anti-RV polyclonal antibody conjugated with HRP was used to detect bound rotavirus antigen at dilution of 1:2000 (v/v) in PBS at 37°C for 1 h. TMB (TIANGEN, PA107-01) substrate was added for color development and reaction was terminated by 2 mol/L H2SO4. Optical density (OD) at 450 nm and 630 nm was measured with a universal microplate reader (Elx800, Bio-Tek). A sample was determined to be positive if the OD value was greater than or equal to the average OD value of the negative control×2.Citation38 The RV antigen content can be expressed as 10 times of the reciprocal of the highest antigen-positive dilution, and the unit is EU/ml.
Preparation of a rotavirus bivalent inactivated vaccine
The wild-type RV ZTR-68 (G1) and ZTR-18 (G9) strains were isolated from fecal samples from infants in Yunnan Province, China. They were cultivated in Vero cells. After propagation, the viruses were harvested by centrifugation at 8873 g for 20 min, and the supernatant was concentrated 30-fold by ultrafiltration. Purification was performed on a Q-FF and G-25 column. The purified viruses were inactivated with 250 μg/ml formalin at 37°C for 6 days. After three generations of blind passage, whether or not the completeness of inactivation was detected by immunofluorescence. The completely inactivated viruses were emulsified in 0.8 mg/ml Al(OH)3 adjuvant and diluted to 80 EU (ELISA unit, EU) G1 and 160 EU G9/dose (0.5 ml).
Immunization of pregnant rhesus monkeys and challenge tests in rhesus monkeys with SA11
Six pregnant rhesus monkeys were divided into two groups. The first immunization occurred about 30 days before parturition. The vaccine group was intramuscularly immunized with the rotavirus inactivated vaccine containing 80 EU G1 and 160 EU G9 antigen (0.5 ml) twice at an interval of 15 days. The control group received the 0.8 mg/ml Al(OH)3 adjuvant (0.5 ml). The monkeys were tested for neutralizing antibodies in peripheral blood taken before the primary immunization and 2 weeks after every immunization.
Neonatal rhesus monkeys aged 15 days were inoculated with 108 PFU rotavirus SA11 via oral gavage. The monkeys were sacrificed by electric shock with anesthesia at day 3 postchallenge to examine the pathological lesions and analyze the viral loads in some of the organs ().
Neutralization and cross-neutralization test
Heat-inactivated sera were diluted 1:8, and then twofold serial dilutions were performed to generate a dilution range from 1:8 to 1:512. The activated RVs were adjusted 100PFU/100 μl in serum-free MEM. The diluted RVs and serum samples were mixed with each and incubated at 37°C for 2 h. The mixtures were transferred to the 12-well plates covered with a confluent monolayer of MA104 cell grown on glass coverslips and were cultured at 37°C for 16 h. The glass coverslides were fixed in the cold paraformaldehyde and methanol for 30 min, blocked with 2% bovine serum albumin in PBS, then incubated with goat anti-RV polyclonal antibody (prepared in our laboratory) in 1:1000 dilution at 37°C for 90 min. Following three washes with PBS, cells were stained with fluorescein isothiocyanate (FITC)-conjugated rabbit anti-goat antiserum at 37°C for 60 min. The cells were washed again and stained with 40, 6-diamidino-2-phenylindole (DAPI) and detected under a fluorescence microscopy. Virus-neutralizing antibody titers were expressed as the reciprocal of the highest serum dilution, which resulted in an 50% or greater reduction in fluorescent foci.
Determination of rotavirus specific IgA in serum or milk samples
The wells of the ELISA plate were coated with rotavirus antigen diluted at 1:50 (v/v, 20.48EU) in a carbonate–bicarbonate buffer overnight at 4°C. Washing three times with PBS containing 0.05% (v/v) Tween-20 (PBS-T), the plates were blocked with 3% (w/v) BSA (Biosharp, BS043D) in PBS at 37°C for 2 h. The serum or milk samples were diluted in two-fold dilution steps starting at 1:2 and plates were incubated for 1 h at 37°C. After washing, detection occurred with a HRP-conjugated goat anti-monkey IgA antibody (KPL,074–11-011). All ELISA were developed using TMB (TIANGEN, PA107-01) substrate to generate a colorimetric reaction and were terminated by 2 mol/L H2SO4. Optical density (OD) at 450 nm and 630 nm was measured with a universal microplate reader (Elx800, Bio-Tek). The antibody titer in the serum or milk was defined as the reciprocal of the highest dilution giving a mean OD greater than the cutoff value of 0.105.
Fecal samples collection and processing
The fecal samples were collected each morning in a fecal collector by gently pressing the abdomen of the neonatal rhesus monkeys 2 h after the monkey’s first meal. According to several criteria,Citation39,Citation40 such as the color, hardness, and quantity of the feces, diarrhea was scored from 1 to 4, and more than 2 points was considered to indicate diarrhea. The fecal sample was suspended in 10% (s/v) cold PBS, followed by centrifugation at 8873 g for 20 min at 4°C. The supernatant was collected and stored at −80°C for subsequent study.Citation38
Histopathological examination of the small intestine
The small intestine of the infected monkeys was fixed in 10% (v/v) formalin in PBS for at least 48 h, dehydrated in a 70% (v/v) graded ethanol series, and embedded in paraffin before being sectioned at a thickness of 4.0 μm for further hematoxylin and eosin (H&E) staining. Histopathological analysis of the small intestine was performed under a light microscope.Citation38
Viral RNA extraction and qRT-PCR assay
Viral RNA was isolated from fresh tissue of the experimental animals, including the duodenum, jejunum, ileum and mesenteric lymph nodes (MLNs), by TRIzol (Ambion, 15596026). RT-PCR assays were performed using TransScript II Probe One-Step qRT-PCR SuperMix (TRANS, AQ321-01) in a real-time system (CFX96, BIO-RAD, USA). The reaction system included 5 μl of RNA template, 20 nM sense primer, 20-nM antisense primer, 20-nM FAM-labeled probe and E-mix in a 20-μl total reaction volume. The NSP3-specific sequences for the primers were as follows: sense primer, 5ʹ-GTTGTCATCTATGCATAACCCTC-3ʹ; antisense primer, 5ʹ-ACATAACGCCCCTAT AGCCA-3ʹ; and FAM-labeled probe, 5ʹ-ATGAGCACAATAGTTAAAAGCTAACAC TGTCAA-3ʹ. The following protocol was used for all RT-PCR analyses: 5 min at 50◦C and 30 s at 94◦C, followed by 40 cycles of 94◦C for 5 s and 60◦C for 30 s. SA11 virus RNA was also obtained by TRIzol and RT-PCR assays were also performed using TransScript II Probe One-Step qRT-PCR SuperMix. A standard reference curve was obtained by measuring the serially diluted SA11 virus RNA (dilution range from 1:10 to 1:104). According to the standard reference curve, the viral load was quantified in each sample.Citation38
Results
The neutralizing antibody response induced by rotavirus inactivated vaccine immunization in pregnant rhesus monkeys
To confirm that the rotavirus bivalent (G1 and G9) inactivated vaccine can stimulate humoral immunity in pregnant monkeys, we tested whether the sera of pregnant monkeys 14 days after the first immunization and 14 days after the second immunization could neutralize the parental strains ZTR-68 and ZTR-18. The results showed that the geometric mean value of neutralizing antibody against ZTR-68 in the serum of pregnant monkeys reached 40.3 14 days after the first immunization. On day 14 after the second immunization, it increased to 50.8 (). The neutralizing antibody titer against ZTR-18 was higher than that against ZTR-68.
Figure 2. Neutralizing antibody titer of anti G1 (ZTR-68) strain induced by the vaccine at 14 days after second immunization. Serum with different dilutions and 1000 PFU rotavirus were mixed with each other at 37°C for 2 h, and then the mixtures were transferred to the 6-well plate covered with a confluent monolayer of MA104 cells and were cultured for 16 h for immunofluorescence. (a) 1000PFU ZTR-68; (b) 1000PFU ZTR-68 and 1:8 diluted serum; (c) 1000PFU ZTR-68 and 1:16 diluted serum; (d) 1000PFU ZTR-68 and 1:32 diluted serum; (e) 1000PFU ZTR-68 and 1:64 diluted serum; (f) 1000PFU ZTR-68 and 1:128 diluted serum; (g) 1000PFU ZTR-68 and 1:256 diluted serum; (h) 1000PFU ZTR-68 and 1:512 diluted serum; (i) cell control. Magnification×10. Bar, 100 μm.
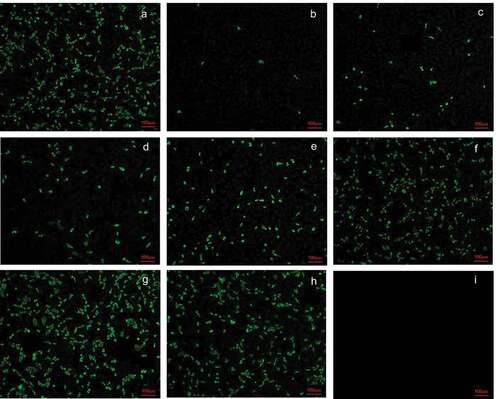
The cross-neutralizing antibody of antiserum induced by rotavirus inactivated vaccine immunization in pregnant rhesus monkeys
Cross-neutralization detection was performed on four rotavirus strains with different genotypes and serotypes using serum collected from rotavirus bivalent (G1+ G9) inactivated vaccine-immunized rhesus pregnant monkeys at 14 days after the first and second immunizations. The results indicated that the geometric means of titers against SA11, WA, UK, and Gottfried were 128, 80.6, 20.2 and 256, respectively, at 14 days after the first immunization and 256, 161.3, 32 and 406.3, respectively, at 14 days after the second immunization ().
Figure 4. Cross-neutralization antibody titer of anti SA11 strain induced by the vaccine at 14 days after second immunization. Serum with different dilutions and 1000 PFU rotavirus were mixed with each other at 37°C for 2 h, and then the mixtures were transferred to the 6-well plate covered with a confluent monolayer of MA104 cells and were cultured for 16 h for immunofluorescence. (a) 1000PFU SA11; (b) 1000PFU SA11 and 1:8 diluted serum; (c) 1000PFU SA11 and 1:16 diluted serum; (d) 1000PFU SA11 and 1:32 diluted serum; (e) 1000PFU SA11 and 1:64 diluted serum; (f) 1000PFU SA11 and 1:128 diluted serum; (g) 1000PFU SA11 and 1:256 diluted serum; (h) 1000PFU SA11 and 1:512 diluted serum; (i) cell control. Magnification×10. Bar, 100 μm.
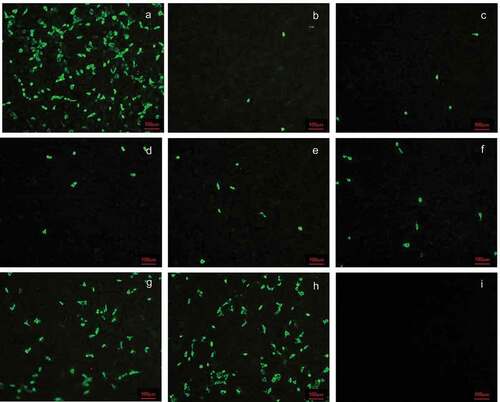
IgA antibody titers induced by rotavirus inactivated vaccine immunization in pregnant rhesus monkeys
We measured the titer of rotavirus-specific secretory IgA in the milk of pregnant monkeys after parturition. The results showed that rotavirus-specific secretory IgA could be produced in the milk of pregnant monkeys immunized with the inactivated rotavirus vaccine, the geometric mean IgA antibody titer was 25.4 (*P < .01), and undetectable rotavirus-specific secretory IgA titers in the milk in the control group were detected ()). The results suggested that secretory IgA in the milk will be induced after immunization with the inactivated rotavirus vaccine.
Figure 6. IgA antibody titers in milk in pregnant rhesus monkeys and neutralizing antibody in newborn rhesus monkeys. (a) IgA antibody titers in milk induced by the vaccine (80EU G1 and 160EU G9) or placebo (0.8 mg/ml Al(OH)3 adjuvant) in pregnant rhesus monkeys at 14 days after parturition; (b) maternal-specific rotavirus cross-neutralization antibody titers in newborn rhesus monkeys at 14 days after childbirth. Data are expressed as the mean ± SD, n = 3, *P ≤ 0.05, **P ≤ 0.01.
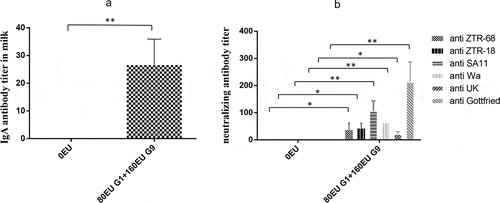
Maternal-specific rotavirus antibody titers in newborn rhesus monkeys
To confirm that the pregnant monkeys could pass the antibody to neonatal rhesus monkeys, we measured the specific neutralizing antibody titers in neonatal rhesus monkeys. The geometric means of neutralizing antibody titers against ZTR-68, ZTR-18, SA11, WA, UK, and Gottfried were 32, 40.3, 101.6, 64, 20.2 and 203.2, respectively, at 15 days after birth in neonatal rhesus monkeys ()). These results indicated that the pregnant monkeys passed on the rotavirus antibodies to their offspring transplacentally.
Parenteral immunization with the rotavirus inactivated vaccine induces protection against clinical diarrhea in offspring
Findings on whether the inactivated rotavirus vaccine can induce protection in rhesus monkeys have not been reported. To address this question, we immunized pregnant rhesus monkeys and challenged their newborn offspring at the age of 15 days with 108 PFU (3.38DD50) of SA11 and evaluated the monkeys for diarrhea. Compared to the control group, in the vaccine group, the degree of diarrhea was obviously lower. On 1 dpi, there was diarrhea, but the severity of diarrhea was not as serious as that in the control group. On 2 dpi, diarrhea was alleviated, and on 3 dpi, diarrhea was further reduced. Beginning at 3 dpi, no viral antigen was detected in the feces ( and )). Further pathological examination revealed that none of the obvious pathological lesions were found in the vaccine group. In contrast, we found notable pathological damage, massive cellular vacuolation, and the absence of mild inflammatory cell infiltration in the small intestinal tissue ()). In addition, we found viral load variations in the duodenum, jejunum, ileum and mesenteric lymph nodes at 3 dpi. We observed a viral load of approximately 104 ~ 105 copies per 100 mg in the small intestine at 3 dpi in the control group. In contrast, in the vaccine group, the viral loads decreased to 1%–5% in the small intestine at 3 dpi. A viral load of 106 copies/100 mg tissue was detected in the mesenteric lymph nodes at 3 dpi in the control group, but no viral load was detected in the vaccine group ().
Table 1. Diarrhea score of the immunized neonatal rhesus monkeys from 1 dpi to 3 dpi
Figure 7. Preliminary analysis of immunoprotection in neonatal rhesus monkeys. The neonatal rhesus monkey’ mothers were immunized with 80EU G1 and 160EU G9 inactivated vaccine or placebo (0.8 mg/ml Al(OH)3 adjuvant). (a) The RV antigen-shedding in feces of neonatal rhesus monkeys inoculated with 108PFU SA11 from 0dpi to 3dpi; (b) histopathological changes in the jejunum of neonatal rhesus monkeys infected with 108PFU SA11 at 3dpi in the control group; (c) histopathological changes in the jejunum of neonatal rhesus monkeys infected with 108PFU SA11 at 3dpi in the vaccine group. Magnification ×10. Bar, 100 μm.
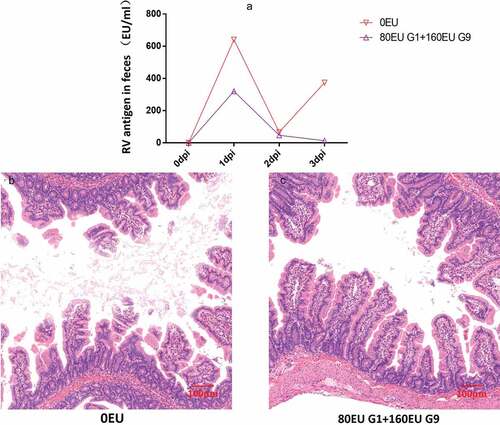
Figure 8. Comparison of viral load in different organs of neonatal rhesus monkeys inoculated with SA11 in vaccine group and control group. (a) Viral load in the duodenum of neonatal rhesus monkeys inoculated with SA11 at 3 dpi; (b) viral load in the jejunum of neonatal rhesus monkeys inoculated with SA11 at 3 dpi; (c) viral load in the ileum of neonatal rhesus monkeys inoculated with SA11 at 3 dpi; (d) viral load in the MLN of neonatal rhesus monkeys inoculated with SA11 at 3 dpi. Data are expressed as the mean ± SD, n = 3, **P ≤ 0.01.
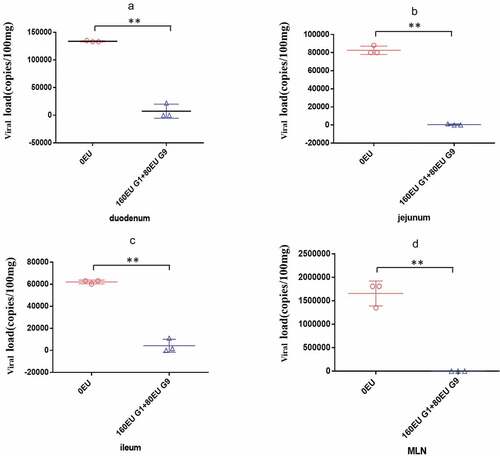
Discussion
The currently most-used vaccines, RotaTeqTM and RotarixTM licensed in more than 100 countries, are live-attenuated rotavirus vaccines. They play an important role in reducing the mortality and morbidity of rotavirus-associated diarrhea worldwide. Both RotarixTM and RotaTeqTM have proven to be highly efficacious in developed countries at reducing severe rotavirus disease (80–90%) in clinical trials.Citation41,Citation42 However, they were less efficacious in developing countries (30–50%), mainly in sub-Saharan Africa and S.E. Asia.Citation43,Citation44 Nevertheless, the occurrence of intussusception remains a public health concern.Citation22–24 However, the inactivated rotavirus vaccine could have the potential to overcome some of the challenges associated with oral live-attenuated vaccines. Compared with the live attenuated vaccine, the parenteral vaccine basically does not cause intussusception, which greatly improves the safety of the vaccine. In addition, intestinal flora and maternal antibody have less effect on inactivated vaccine. So parenteral RV vaccine is considered as potentially preferable to live attenuated vaccines in low- and middle-income countries. The inactivated rotavirus vaccine is a form of parenteral immunization, which can produce a strong serum antibody reaction.Citation45 Serum antibodies may leak to the surface of the intestine and inhibit viral attachment or replication to mediate the protective effect. Some studies indicated that subunit rotavirus vaccine, 2/6/7-VLPs and 2/4/6/7-VLPs, demonstrated strong IgG responses and neutralizing antibody response to several strains of rotavirus in mice models.Citation30 Another subunit rotavirus vaccine P2-VP8-P[8] vaccinated infants also demonstrated strong IgG responses in vaccinated infants.Citation46 An inactivated rotavirus vaccine has also been developed by the CDC for parenteral administration. It has been tested in pig models and demonstrated a heterotypic neutralizing antibody response.Citation47
A rotavirus strain could induce broad cross-reactive neutralizing antibody to different rotavirus genotypes. There is substantial evidence for natural infection with rotavirus or vaccination with rotavirus vaccine can elicit broad homologous or heterologous cross protection in children.Citation33–35 Epidemiological studies show that the G1P[8] and G9P[8] strains are the most common rotavirus strains. Therefore, G1P[8] and G9 P[8] were isolated from the feces of infants and young children, and inactivated vaccines were prepared. Pregnant monkeys were immunized with a mixture of 80 EU G1 and 160 EU G9. To answer the question of whether bivalent inactivated rotavirus induces cross-reactive immunity to different rotavirus genotypes, we analyzed sera from pregnant monkeys vaccinated with bivalent inactivated rotavirus and their offspring to assess homotypic and heterotypic neutralizing activity against rotavirus strains of different genotypes, including the ZTR-68, ZTR-18, SA11, WA, UK, and Gottfried strains. Animals that received two doses of inactivated rotavirus vaccine developed a cross-neutralization reaction. It has higher neutralizing antibody titers against Gottfried (GMT = 406.3) and lower titers against the UK (GMT = 32). The sera from their offspring also have higher neutralizing antibody titers against these rotavirus strains.
RV-specific antibodies, especially of the IgA class, are recognized as being important.Citation48 The correlation of RV-specific serum IgA antibodies with protection is controversial. Some studies in developing countries indicated that rotavirus-specific serum IgA levels are not an optimal correlation of protection following vaccination.Citation49 Some researchers found that neutralization by VP6-specific IgG was much more efficient than by VP6-specific IgA.Citation50 Some studies showed that children with an IgA titer >1:800 had a lower risk of rotavirus infection and diarrhea.Citation32 IgA exists in both serum and milk. IgA in serum cannot be transferred to infant monkeys through the placenta, but IgA in milk can be transferred to infant monkeys through lactation. Therefore, we measured the titer of rotavirus-specific secretory IgA in the milk of pregnant monkeys after immunization with rotavirus. Compared with that of the control group, the IgA titer in the pregnant monkeys of the vaccine group increased in their milk.
In previous studies, we established a rotavirus infant monkey diarrhea model.Citation38 In this study, we applied the model to preliminarily evaluate an experimental inactivated rotavirus vaccine. We confirmed that neonatal rhesus monkeys with an average age of 15–20 days were susceptible to RV infection. Studies have shown that injection of IRV into pregnant cows, horses or mice can effectively provide passive immunity and protect their newborn offspring from diseases.Citation51–54 Therefore, we immunized pregnant monkeys, evaluated the protective effect of maternal antibodies in neonatal rhesus monkeys, and indirectly evaluated whether the inactivated experimental rotavirus vaccine had a protective effect in rhesus monkeys. Our results indicated that although the maternal antibodies induced by the experimental rotavirus inactivated vaccine were not able to completely prevent diarrhea caused by a G3P[2] strain, they did contribute to reducing the symptoms of diarrhea.
In summary, we have demonstrated that two doses of IRV were able to induce neutralizing antibodies in the pregnant monkeys against homologous or heterologous rotavirus strains and induced protection from rotavirus SA11 infection in the offspring. Our findings provide evidence for further clinical development of the rotavirus inactivated vaccine as an alternative candidate vaccine. However, there are several limitations in this study. Firstly, although we examined protection against challenge with multiple homologous or heterologous rotavirus strains in vitro, we only examined protection against challenge with a heterologous G3P[2] strain in the neonatal rhesus monkeys. Second, although rhesus monkey is a potential rotavirus model, due to the limitation of sensitive age of animal model, we cannot carry out whole-course immunization on the animal model. So we only indirectly evaluated the candidate vaccine through passive immunity. More work is required to assess the validity of this animal model for the assessment of RV vaccine candidates.
Disclosure statement
No potential conflict of interest was reported by the authors.
Additional information
Funding
References
- Crawford SE, Ramani S, Tate JE, Parashar UD, Svensson L, Hagbom M, Franco MA, Greenberg HB, O’Ryan M, Kang G, et al. Rotavirus infection. Nat Rev Dis Primers. 2017;3(1):17083. doi:10.1038/nrdp.2017.83 .
- Sadiq A, Bostan N, Yinda KC, Naseem S, Sattar S. Rotavirus: genetics, pathogenesis and vaccine advances. Rev Med Virol. 2018;28:e2003. doi:10.1002/rmv.2003 .
- Matthijnssens J, Ciarlet M, Rahman M, Attoui H, Banyai K, Estes MK, Gentsch JR, Iturriza-Gómara M, Kirkwood CD, Martella V, et al. Recommendations for the classification of group A rotaviruses using all 11 genomic RNA segments. Arch Virol. 2008;153(8):1621–29. doi:10.1007/s00705-008-0155-1 .
- https://rega.kuleuven.be/cev/viralmetagenomics/virus-classification/rcwg .
- Bulut Y, Yenisehirli G, Durmaz R. Molecular epidemiology of rotavirus strains in under five children. Indian J Pediatr. 2018;85:364–68. doi:10.1007/s12098-017-2540-9 .
- Tavakoli Nick S, Mohebbi SR, Ghaemi A, Hosseini SM. Human rotavirus in Iran; molecular epidemiology, genetic diversity and recent updates on vaccine advances. Gastroenterol Hepatol Bed Bench. 2019;12:98–109 .
- Li W, Xiang W, Li C, Xu J, Zhou D, Shang S. Molecular epidemiology of rotavirus A and adenovirus among children with acute diarrhea in Hangzhou, China. Gut Pathog. 2020;12(1):19. doi:10.1186/s13099-020-00359-4 .
- Leung AK, Robson WL. Acute gastroenteritis in children: role of anti-emetic medication for gastroenteritis-related vomiting. Paediatr Drugs. 2007;9(3):175–84. doi:10.2165/00148581-200709030-00006 .
- Yen C, Tate JE, Patel MM, Cortese MM, Lopman B, Fleming J, Lewis K, Jiang B, Gentsch JR, Steele AD, et al. Rotavirus vaccines: update on global impact and future priorities. Hum Vaccin. 2011;7(12):1282–90. doi:10.4161/hv.7.12.18321 .
- Patton JT. Rotavirus diversity and evolution in the post-vaccine world. Discov Med. 2012;13:85–97 .
- Burnett E, Parashar UD, Tate JE. Real-world effectiveness of rotavirus vaccines, 2006-19: a literature review and meta-analysis. Lancet Glob Health. 2020;8:e1195–e202. doi:10.1016/S2214-109X(20)30262-X .
- Burke RM, Tate JE, Kirkwood CD, Steele AD, Parashar UD. Current and new rotavirus vaccines. Curr Opin Infect Dis. 2019;32:435–44. doi:10.1097/QCO.0000000000000572 .
- Soares-Weiser K, Bergman H, Henschke N, Pitan F, Cunliffe N. Vaccines for preventing rotavirus diarrhoea: vaccines in use. Cochrane Database Syst Rev. 2019;3:CD008521. doi:10.1002/14651858.CD008521.pub4 .
- Tate JE, Burton AH, Boschi-Pinto C, Parashar UD; World Health Organization-Coordinated Global Rotavirus Surveillance N. Global, regional, and national estimates of rotavirus mortality in children <5 years of age, 2000-2013. Clin Infect Dis. 2016;62(Suppl 2):S96–S105. doi:10.1093/cid/civ1013 .
- Parashar UD, Hummelman EG, Bresee JS, Miller MA, Glass RI. Global illness and deaths caused by rotavirus disease in children. Emerg Infect Dis. 2003;9:565–72. doi:10.3201/eid0905.020562 .
- Li J, Zhang Y, Yang Y, Liang Z, Tian Y, Liu B, Gao Z, Jia L, Chen L, Wang Q, et al. Effectiveness of Lanzhou lamb rotavirus vaccine in preventing gastroenteritis among children younger than 5 years of age. Vaccine. 2019;37:3611–16. doi:10.1016/j.vaccine.2019.03.069 .
- Troeger C, Khalil IA, Rao PC, Cao S, Blacker BF, Ahmed T, Armah G, Bines JE, Brewer TG, Colombara DV, et al. Rotavirus vaccination and the global burden of rotavirus diarrhea among children younger than 5 years. JAMA Pediatr. 2018;172:958–65. doi:10.1001/jamapediatrics.2018.1960 .
- Kotloff KL, Nataro JP, Blackwelder WC, Nasrin D, Farag TH, Panchalingam S, Wu Y, Sow SO, Sur D, Breiman RF, et al. Burden and aetiology of diarrhoeal disease in infants and young children in developing countries (the Global Enteric Multicenter Study, GEMS): a prospective, case-control study. Lancet. 2013;382:209–22. doi:10.1016/S0140-6736(13)60844-2 .
- Otero CE, Langel SN, Blasi M, Permar SR. Maternal antibody interference contributes to reduced rotavirus vaccine efficacy in developing countries. PLoS Pathog. 2020;16:e1009010. doi:10.1371/journal.ppat.1009010 .
- Desselberger U. Differences of rotavirus vaccine effectiveness by country: likely causes and contributing factors. Pathogens. 2017;6:65. doi:10.3390/pathogens6040065 .
- Parker EP, Ramani S, Lopman BA, Church JA, Iturriza-Gomara M, Prendergast AJ, Grassly NC. Causes of impaired oral vaccine efficacy in developing countries. Future Microbiol. 2018;13:97–118. doi:10.2217/fmb-2017-0128 .
- Reddy SN, Nair NP, Tate JE, Thiyagarajan V, Giri S, Praharaj I, Mohan VR, Babji S, Gupte MD, Arora R. Intussusception after Rotavirus Vaccine Introduction in India. N Engl J Med. 2020;383(20):1932–40. doi:10.1056/NEJMoa2002276 .
- Group IISNS. Risk of intussusception after monovalent rotavirus vaccine (Rotavac) in Indian infants: a self-controlled case series analysis. Vaccine. 2021;39:78–84. doi:10.1016/j.vaccine.2020.09.019 .
- Huang WT, Juan YC, Liu CH, Yang YY, Chan KA. Intussusception and Kawasaki disease after rotavirus vaccination in Taiwanese infants. Vaccine. 2020;38:6299–303. doi:10.1016/j.vaccine.2020.07.038 .
- Yin S, Liubao P, Chongqing T, Xiaomin W. The first case of Kawasaki disease in a 20-month old baby following immunization with rotavirus vaccine and hepatitis A vaccine in China: a case report. Hum Vaccin Immunother. 2015;11:2740–43. doi:10.1080/21645515.2015.1050571 .
- Offit PA. Challenges to developing a rotavirus vaccine. Viral Immunol. 2018;31:104–08. doi:10.1089/vim.2017.0121 .
- Burnett E, Parashar U, Tate J. Rotavirus vaccines: effectiveness, safety, and future directions. Paediatr Drugs. 2018;20:223–33. doi:10.1007/s40272-018-0283-3 .
- Jiang B, Estes MK, Barone C, Barniak V, O’Neal CM, Ottaiano A, Madore HP, Conner ME. Heterotypic protection from rotavirus infection in mice vaccinated with virus-like particles. Vaccine. 1999;17:1005–13. doi:10.1016/S0264-410X(98)00317-X .
- Crawford SE, Estes MK, Ciarlet M, Barone C, O’Neal CM, Cohen J, Conner ME. Heterotypic protection and induction of a broad heterotypic neutralization response by rotavirus-like particles. J Virol. 1999;73(6):4813–22. doi:10.1128/JVI.73.6.4813-4822.1999 .
- Blutt SE, Warfield KL, O’Neal CM, Estes MK, Conner ME. Host, viral, and vaccine factors that determine protective efficacy induced by rotavirus and virus-like particles (VLPs). Vaccine. 2006;24(8):1170–79. doi:10.1016/j.vaccine.2005.08.090 .
- Liu GF, Hille D, Kaplan SS, Goveia MG. Postdose 3 G1 serum neutralizing antibody as correlate of protection for pentavalent rotavirus vaccine. Hum Vaccin Immunother. 2017;13(10):2357–63. doi:10.1080/21645515.2017.1356522 .
- Velazquez FR, Matson DO, Guerrero ML, Shults J, Calva JJ, Morrow AL, Glass R, Pickering L, Ruiz‐Palacios G. Serum antibody as a marker of protection against natural rotavirus infection and disease. J Infect Dis. 2000;182(6):1602–09. doi:10.1086/317619 .
- Nair N, Feng N, Blum LK, Sanyal M, Ding S, Jiang B, Sen A, Morton JM, He X-S, Robinson WH. VP4- and VP7-specific antibodies mediate heterotypic immunity to rotavirus in humans. Sci Transl Med. 2017;9(395):eaam5434. doi:10.1126/scitranslmed.aam5434 .
- Velazquez FR, Matson DO, Calva JJ, Guerrero L, Morrow AL, Carter-Campbell S, Glass RI, Estes MK, Pickering LK, Ruiz-Palacios GM, et al. Rotavirus infection in infants as protection against subsequent infections. N Engl J Med. 1996;335(14):1022–28. doi:10.1056/NEJM199610033351404 .
- Ruiz-Palacios GM, Perez-Schael I, Velazquez FR, Abate H, Breuer T, Clemens SC, Cheuvart B, Espinoza F, Gillard P, Innis BL, et al. Safety and efficacy of an attenuated vaccine against severe rotavirus gastroenteritis. N Engl J Med. 2006;354(1):11–22. doi:10.1056/NEJMoa052434 .
- Vesikari T, Karvonen A, Prymula R, Schuster V, Tejedor JC, Cohen R, Meurice F, Han HH, Damaso S, Bouckenooghe A, et al. Efficacy of human rotavirus vaccine against rotavirus gastroenteritis during the first 2 years of life in European infants: randomised, double-blind controlled study. Lancet. 2007;370(9601):1757–63. doi:10.1016/S0140-6736(07)61744-9 .
- Steele AD, Neuzil KM, Cunliffe NA, Madhi SA, Bos P, Ngwira B, Witte D, Todd S, Louw C, Kirsten M, et al. Human rotavirus vaccine Rotarix provides protection against diverse circulating rotavirus strains in African infants: a randomized controlled trial. BMC Infect Dis. 2012;12:213. doi:10.1186/1471-2334-12-213 .
- Yin N, Yang FM, Qiao HT, Zhou Y, Duan SQ, Lin XC, Wu J-Y, Xie Y-P, He Z-L, Sun M-S, et al. Neonatal rhesus monkeys as an animal model for rotavirus infection. World J Gastroenterol. 2018;24:5109–19. doi:10.3748/wjg.v24.i45.5109 .
- Boshuizen JA, Reimerink JH, Korteland-van Male AM, van Ham VJ, Koopmans MP, Buller HA, Dekker J, Einerhand AWC. Changes in small intestinal homeostasis, morphology, and gene expression during rotavirus infection of infant mice. J Virol. 2003;77:13005–16. doi:10.1128/JVI.77.24.13005-13016.2003 .
- Li JT, Wei J, Guo HX, Han JB, Ye N, He HY, Yu -T-T, Wu Y-Z. Development of a human rotavirus induced diarrhea model in Chinese mini-pigs. World J Gastroenterol. 2016;22:7135–45. doi:10.3748/wjg.v22.i31.7135 .
- Rha B, Tate JE, Payne DC, Cortese MM, Lopman BA, Curns AT, Parashar UD. Effectiveness and impact of rotavirus vaccines in the United States - 2006-2012. Expert Rev Vaccines. 2014;13:365–76. doi:10.1586/14760584.2014.877846 .
- Jonesteller CL, Burnett E, Yen C, Tate JE, Parashar UD. Effectiveness of Rotavirus Vaccination: a Systematic Review of the First Decade of Global Postlicensure Data, 2006-2016. Clin Infect Dis. 2017;65:840–50. doi:10.1093/cid/cix369 .
- Armah GE, Sow SO, Breiman RF, Dallas MJ, Tapia MD, Feikin DR, Binka FN, Steele AD, Laserson KF, Ansah NA. Efficacy of pentavalent rotavirus vaccine against severe rotavirus gastroenteritis in infants in developing countries in sub-Saharan Africa: a randomised, double-blind, placebo-controlled trial. Lancet. 2010;376(9741):606–14. doi:10.1016/S0140-6736(10)60889-6 .
- Madhi SA, Cunliffe NA, Steele D, Witte D, Kirsten M, Louw C, Ngwira B, Victor JC, Gillard PH, Cheuvart BB, et al. Effect of human rotavirus vaccine on severe diarrhea in African infants. N Engl J Med. 2010;362:289–98. doi:10.1056/NEJMoa0904797.
- Resch TK, Wang Y, Moon SS, Joyce J, Li S, Prausnitz M, Jiang, B. Inactivated rotavirus vaccine by parenteral administration induces mucosal immunity in mice. Sci Rep. 2018;8:561. doi:10.1038/s41598-017-18973-9.
- Groome MJ, Fairlie L, Morrison J, Fix A, Koen A, Masenya M, Jose, L, Madhi, SA, Page, N, McNeal, M, et al. Safety and immunogenicity of a parenteral trivalent P2-VP8 subunit rotavirus vaccine: a multisite, randomised, double-blind, placebo-controlled trial. Lancet Infect Dis. 2020;20:851–63. doi:10.1016/S1473-3099(20)30001-3.
- Wang Y, Azevedo M, Saif LJ, Gentsch JR, Glass RI, Jiang B. Inactivated rotavirus vaccine induces protective immunity in gnotobiotic piglets. Vaccine. 2010;28:5432–36 .
- Clarke E, Desselberger U. Correlates of protection against human rotavirus disease and the factors influencing protection in low-income settings. Mucosal Immunol. 2015;8:1–17. doi:10.1038/mi.2014.114 .
- Angel J, Franco MA, Greenberg HB. Rotavirus immune responses and correlates of protection. Curr Opin Virol. 2012;2:419–25. doi:10.1016/j.coviro.2012.05.003 .
- Caddy SL, Vaysburd M, Wing M, Foss S, Andersen JT, O’Connell K, Mayes K, Higginson K, Iturriza-Gómara M, Desselberger U, et al. Intracellular neutralisation of rotavirus by VP6-specific IgG. PLoS Pathog. 2020;16:e1008732. doi:10.1371/journal.ppat.1008732 .
- Snodgrass DR. Development of calf diarrhoea vaccines. Ann Rech Vet. 1983;14:519–21 .
- Castrucci G, Frigeri F, Angelillo V, Ferrari M, Cilli V, Aldrovandi V. Field trial evaluation of an inactivated rotavirus vaccine against neonatal diarrhea of calves. Eur J Epidemiol. 1987;3:5–9. doi:10.1007/BF00145064 .
- Powell DG, Dwyer RM, Traub-Dargatz JL, Fulker RH, Whalen JW Jr., Srinivasappa J, Acree, WM, Chu, HJ. Field study of the safety, immunogenicity, and efficacy of an inactivated equine rotavirus vaccine. J Am Vet Med Assoc. 1997;211:193–98.
- Nemoto M, Inagaki M, Tamura N, Bannai H, Tsujimura K, Yamanaka T, Kokado, H. Evaluation of inactivated vaccines against equine group A rotaviruses by use of a suckling mouse model. Vaccine. 2018;36:5551–55. doi:10.1016/j.vaccine.2018.07.057.