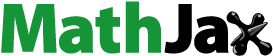
ABSTRACT
The present study was carried out to establish the proportion of Coarse Reclaimed Asphalt Pavement (C-RAP) containing varying percentages of asphalt for preparing Pavement Quality Concrete (PQC) mixes. RAP was collected from the 5-year-old pavement and separated into coarse RAP (C-RAP) and fine RAP (F-RAP) aggregates using a 4.75 mm sieve. Asphalt content in the C-RAP and F-RAP was found to be 4.12% and 4.33%, respectively. The C-RAP was immersed in the toluene solvent for a different predetermined time and asphalt content was reduced to 3.05%, 2.12%, and 1.24%. Using this exercise, C-RAP containing four different percentages of asphalt (4.12, 3.05, 2.12, and 1.24%) was produced. Then, the cement concrete mixes were prepared in the laboratory by replacing Natural Coarse Aggregate (NCA) with C-RAP varying from 25% to 100% with an increment of 25% in each type. Manufactured Sand (M-Sand)/Crushed Stone Sand (CSS) was used as a fine aggregate. Altogether, 17 concrete mixes (one control and 16 mixes containing C-RAP) were produced while keeping the water–cement ratio unchanged in all the mixes and evaluated their fresh and hardened concrete properties. As the asphalt presence in the RAP is sensitive to the temperature, the strength of concrete containing RAP at different temperatures was also examined. The current study showed that the amount of NCA substituted by C-RAP and the quantity of asphalt present in the C-RAP influenced the properties of concrete. The study also established that NCA up to 100% could be replaced with the C-RAP containing low asphalt content. Using test results, empirical models were developed to estimate the properties of hardened concrete by considering the percentage of C-RAP, percentage of asphalt in C-RAP, and compressive strength of concrete as predictor variables. The developed models were found statistically significant, supported by .
1.0 Introduction
The removed and processed asphalt concrete material from the asphalt pavements during resurfacing or reconstruction is called Reclaimed Asphalt Pavement (RAP). It contains two valuable materials, namely aggregates and aged asphalt binders. Millions of tons of RAP are being extracted from asphalt pavements every year worldwide. Proper utilization of RAP in constructing pavement layers provides several economic and environmental benefits in terms of reduction in consumption of natural aggregates and construction cost (Mansourkhaki et al., Citation2020; Saghafi et al., Citation2019; Singh et al., Citation2019; Xiao et al., Citation2007; Yu et al., Citation2017). RAP has been employed in preparing asphalt concrete mixes since the 1930s (Huang et al., Citation2005) and has proven very effective and durable material (Mohammadafzali et al., Citation2018; Visintine et al., Citation2013). However, because of various problems associated with using a higher percentage of RAP in the bituminous surface and in base layers, most of the agencies restricted usage of RAP up to a maximum of 30% (Abed et al., Citation2018). The limited use of RAP in bituminous layers has resulted in the disposal of surplus RAP, which is being accumulated in stockpiles and is used as a replacement of aggregates in unbound granular layers and filling low-lying areas (Noferini et al., Citation2017). Besides the usage of RAP in bituminous layers, if RAP is being used in place of natural aggregates in preparation Pavement Quality Concrete (PQC), maximum utilization of RAP is possible, and it can reduce the rate of depletion of natural aggregates (Hoyos et al., Citation2011).
Over the past 25 years, several researchers have studied the effect of RAP on the mechanical properties of concrete. Numerous studies have reported that the addition of RAP in the concrete invariably reduces some properties of the concrete, namely, compressive strength, flexural strength, elastic modulus, ultrasound pulse velocity, and splitting tensile strength as compared to the concrete made with virgin aggregates (Al-Oraimi et al., Citation2009; Berry et al., Citation2015; Erdem & Blankson, Citation2014; Ferrebee et al., Citation2014; Hossiney et al., Citation2010; Ibrahim et al., Citation2014; Katsakou & Kolias, Citation2007; Mahmoud et al., Citation2013; Mathias et al., Citation2009; Sachet et al., Citation2011; Shi et al., Citation2017; Solomon Debbarma et al., Citation2020; Su et al., Citation2014; Topcu & Isikdag, Citation2009). Other studies revealed that by increasing the percentage of RAP in the concrete, the strength is decreased and the toughness is increased, making the concrete fracture-resistant (Huang et al., Citation2005; Moaveni et al., Citation2016). Reduction in strength has been attributed to various reasons such as the presence of asphalt film around the aggregates, which causes poor bonding between cement paste and asphalt-coated aggregate (Huang et al., Citation2006); the presence of agglomerated particles on the surface of RAP (Singh et al., Citation2018a); the thickness of asphalt film on the aggregates (Settari et al., Citation2015); oxidation characteristics of the RAP (Singh et al., Citation2018a); and the loss of surface texture of RAP aggregates due to asphalt coating (Shi et al., Citation2018). Studies on bonding characteristics of cementitious materials with asphalt coated particles have attributed strength reduction to high porosity in the Interfacial Transition Zone (ITZ) around the RAP aggregates. High porosity in the ITZ around the RAP initiation of cracks, and it dominates in cohesive failure in the asphalt coating over the aggregates instead of ITZ cohesive failure of the cement–asphalt interface (Brand & Roesler, Citation2017a, Citation2017b).
As the reduction of concrete strength has a detrimental effect on the performance of concrete during its service life, several researchers have suggested several substitutes to minimize the loss of strength that may result from adding RAP in concrete. Huang et al. (Citation2005) recommended replacing a certain portion of natural coarse aggregates in concrete with coarse RAP only. Brand and Roesler (Citation2015) reported that using coarse fractionated-RAP in concrete up to 50% cannot affect strength, durability, shrinkage, and fracture properties. Singh et al. (Citation2017) recommended the removal of the agglomerated particles present in the RAP before using RAP in concrete in order to increase compressive, flexural, and split tensile strength. Brand et al. (Citation2014) reported that fracture properties of concrete with natural coarse aggregates and coarse fractionated reclaimed asphalt pavement up to 45% demonstrated similar results. Other researchers have proposed to use RAP in the lower concrete base/sub-base layers, such as Dry Lean Concrete (DLC; Surender Singh et al., Citation2018b), Roller Compacted Concrete (Fakhri & Amoosoltani, Citation2017; Modarres & Hosseini, Citation2014), the bottom left of two lifts concrete (Bentsen et al., Citation2013), and cement stabilized bases and sub-bases (Guthrie et al., Citation2007; Puppala et al., Citation2011; Taha, Citation2003).
The abovementioned studies have cited that the presence of asphalt film around the aggregates in RAP is primarily responsible for a poor bonding between RAP and cement. Owing to this, the strength of the concrete containing RAP aggregates is less as compared to the conventional concrete. Considering this, the authors have suggested reducing the percentage of RAP to be used in the concrete. The literature stated that the asphalt film thickness depends on the percentage of asphalt present in the RAP (Hmoud, Citation2011; Li et al., Citation2009; Sengoz & Agar, Citation2007), it implies that the more the asphalt content, more is the asphalt film thickness and vice versa. Also, the asphalt content present in RAP varies with the age of the construction. In the case of the fresh RAP, a higher percentage of asphalt is possible and in aged/oxidized RAP, asphalt content is low, accordingly, the film thickness varies (Zhang et al., Citation2020). Therefore, the asphalt content in the RAP is thought to significantly affect the mechanical properties of RAP concrete. With this background, the present study aims to determine the maximum percentage of RAP containing various amounts of asphalt to prepare cement concrete mixes. The RAP removed from a 5-year-old pavement and stockpiled for two months was collected from the field and separated into coarse RAP (C-RAP) and fine RAP (F-RAP) using a 4.75 mm sieve. Asphalt content in the C-RAP and F-RAP was determined to be 4.12% and 4.33%, respectively. The C-RAP was immersed in the toluene solvent for a different predetermined time, and asphalt content was reduced to different percentages 3.05%, 2.12%, and 1.24%. By this methodology, C-RAP materials containing four different percentages of asphalt (4.12%, 3.05%, 2.12%, and 1.24%) were produced. Then, the cement concrete mixes were prepared in the laboratory by replacing the coarse aggregate proportion with C-RAP varying from 25 to 100% with an increment of 25% and four different asphalt percentage combination. Manufactured Sand (M-Sand)/Crushed Stone Sand (CSS) is used as a fine aggregate. A total of 17 concrete mixes (one control and sixteen contains RAP) were produced, and then their fresh and hardened properties were determined. The presence and quantity of asphalt in the RAP change due to temperature, thereby influencing the strength due to internal structural changes. Hence, the compressive strength of concrete containing C-RAP was also determined at different temperatures 40°C, 50°C, 60°C, and 70°C besides the ambient temperature to ascertain the influence of varying temperature on strength. Based on the properties of hardened concrete, empirical models were developed for flexural strength, split tensile strength, and static elastic modulus by considering the percentage of C-RAP, percentage of asphalt in C-RAP, and compressive strength as predictor variables. This study was limited to the addition of C-RAP in the preparation of concrete mixes as the literature suggested that if the same quantity of either C-RAP or F-RAP is replaced with NCA, the mix containing C-RAP gives higher strength.
2.0 Objectives of the study
Several studies were conducted on concrete containing RAP. Based on the properties of RAP, the studies suggested replacing a maximum of 50% of either C-RAP/F-RAP or 30% of RAP containing both coarse and fine fractions. Believably, not only the proportion of RAP but also the percentage/quantity of asphalt presence in the RAP influence the concrete properties. In this direction, this research’s principal objective was to investigate the effect on the properties of concrete when the C-RAP containing different percentages of asphalt are added.
3.0 Materials
3.1 Reclaimed Asphalt Pavement (RAP)
RAP obtained from the surface layer (thickness 50 mm) of 5-year-old pavement and stockpiled for two months was collected from the field and separated into C-RAP and F-RAP using a 4.75 mm sieve. Close visual observation revealed that fine aggregate fractions are not entirely separated from coarse particles because of the adhesive properties of asphalt. The asphalt content in the C-RAP and F-RAP was determined by following ASTM D2172 (American Society for Testing and Materials) standard method and found to be 4.12% and 4.33%, respectively. The present study is limited to replacing coarse aggregates proportion in concrete with coarse RAP. Hence, each C-RAP weighing 1 kg was collected in three separate containers and toluene solvent was poured into it. The percentages of asphalt present in the C-RAP after 10, 30, and 60 min of simple immersion without extraction were 3.05, 2.12, and 1.24, respectively. It is worth mentioning here that the properties of asphalt change due to immersion of RAP in toluene solution, however, to reduce the bitumen content, this procedure was adopted.
Similarly, a bulk quantity of C-RAP required to prepare concrete specimens was also immersed in toluene for different durations of 10, 30, and 60 min in a separate container and thereafter removed and dried. In this process, most of the coarse and fine RAP fractions got separated. Dried C-RAP again sieved through 4.75 mm sieve and particles < 4.75 mm in size were removed. The amount of material passing through 4.75 mm sieve after pouring field C-RAP in the toluene solution was 13.5%, 17.8%, and 20.2% for 10, 30, and 60 min, respectively. Thus, ~100 kg of C-RAP (retained on 4.75 mm sieve) that comprised four different percentages of asphalt, 4.12, 3.05, 2.12, and 1.24% was produced. illustrates the C-RAP with varying percentages of asphalt.
Gradation of C-RAP containing different percentages of asphalt sieved through a standard set of sieves designated for the Pavement Quality Concrete (PQC) as per IRC: 44 [IRC, Citation2017] is presented in . As per the construction records, the maximum size of aggregate used in the surface layer was 26.5 mm and the Nominal Maximum Size was 19 mm. However, shows that some percentage of RAP aggregates are retained on the 26.5 mm sieve due to the finer particles sticking to the coarser particles, leading to the increase in size. Contrary to the above, the percentage of material passing was high for the C-RAP that has less asphalt content. This may be concluded that the finer fractions were wholly separated from the coarser fractions as the C-RAP dipped into the toluene solution for longer. Hence, the individual size of the RAP particles was reduced.
Table 1. Gradation of C-RAP containing different percentages of asphalt content.
Various tests were conducted on C-RAP by adhering with IS:2386-Part III [Bureau of Indian Standards BIS (Bureau of Indian Standards), Citation1963] guidelines and its physical properties were determined (). As per the table, dry rod bulk density, loose bulk density, and specific gravity of C-RAP decreased by increasing the percentage of asphalt in it. It was due to the high percentage of low-density asphalt and asphalt coated aggregates influenced the packing characteristics owing to poor interlocking behavior. There was less water absorption percentage of C-RAP that contained higher asphalt content. It can be concluded that the asphalt coated aggregates have low perviousness and the pores in the aggregates were filled by the bitumen, therefore, low water absorption.
Table 2. Physical properties of C-RAP.
3.2 Coarse and fine aggregates
Natural Coarse Aggregates (NCA) of sizes 20 mm down, 10 mm down, and Manufacture Sand (M-Sand)/Crushed Stone Sand (CSS) were procured locally and were used in the mix design. The petrographic analysis revealed the origin of both NCA and M-Sand from granite rock. Further, determined the physical properties of coarse aggregates and M-Sand as per IS:2368 – Part III (BIS (Bureau of Indian Standards), Citation1963) guidelines shown in and their gradation results sieved through a standard set of sieves designated for the PQC as per IRC: 44 (IRC (Indian Road Congress), Citation2017) provided in . Three representative samples from each aggregate size were tested to determine the properties and gradation, and the average value was reported.
Table 3. Characteristics of coarse aggregates and M-Sand.
Table 4. Gradation of coarse aggregates and M-Sand.
3.3 Cement
Ordinary Portland Cement (OPC) of grade 53 that confirmed to IS: 12,269 (BIS, Citation2013) was used to produce concrete mixes. The physical properties of cement were determined as per relevant BIS specifications and its chemical composition was assessed using X-Ray Fluorescence (XRF). Test results are presented in .
Table 5. Physical properties and chemical composition of cement.
4.0 Production of concrete mixes
Altogether, 17 concrete mixes shown in were produced following IRC: 44 (IRC (Indian Road Congress), Citation2017) guidelines. The mixes designated as control samples contained 100% of NCA, and mixes in which NCA was replaced partially/fully with C-RAP containing various percentages of asphalt. For instance, S.No. ‘3’ mix designated as 75CR4.12, which means 75% of C-RAP containing 4.12% of asphalt was replaced by 75% of NCA i.e. 25% of NCA were present in the mix. The amount of NCA, C-RAP, and M-Sand for each mix presented in were determined based on the combined sieve analysis carried out by mixing various percentages of NCA, M-Sand, and RAP to achieve the gradation within the specified limits as per IRC: 44 (IRC (Indian Road Congress), Citation2017) guidelines. In the control mix, 60% of NCA (30% of each 20 and 10 mm down aggregates) and 40% of M-Sand were mixed to achieve the required combined gradation. In other mixes, the NCA of 60% was replaced with a different percentage of C-RAP by keeping the 40% of M-Sand constant. The gradation of 20 mm down, 10 mm down, M-Sand, C-RAP, and the combined gradation for the mix designated as 75CR4.12 are illustrated in . The combined gradation within the defined upper and lower control limits for mix 75CR4.12 was obtained by blending 40% of M-Sand, 15% of NCA (7.5% of each 20 and 10 mm down aggregates), and 45% of C-RAP (75% of total NCA proportion). This exercise was undertaken for all the mixes with different proportions of C-RAP containing a range of asphalt content. Constant water/cement (w/c) ratio was maintained in all the mixes.
Table 6. Mixes designation and quantities of individual aggregates.
The fresh and hardened concrete properties were evaluated for the mixes prepared for the 17 concrete mix designs, as listed in . Concrete mixes were prepared in a pan mixer following the procedure described in IRC: 44 (IRC (Indian Road Congress), Citation2017). Natural coarse aggregates were added to the pan first, followed by C-RAP with some water and mixed for 30 sec. Then, M-sand and cement were added to the pan. While mixing, the rest of the water was added and homogenous mixing was ensured without adding admixtures. After removing the concrete from the pan, fresh concrete properties were determined. The concrete was filled in moulds of different sizes, completely covered with a plastic sheet, and left for 24 hours of curing at room temperature. Then, the samples were removed from the moulds and placed in a water bath for moist curing for different curing periods and evaluated the properties of hardened concrete.
5.0 Tests to determine properties of concrete
5.1 Tests to determine fresh concrete properties
The fresh concrete properties, namely slump value (in mm) and density (in kg/cu.m.) were determined for all 17 mixes in accordance with IS: 1199 – Part 2 (BIS, Citation2018a) and IS 1199 – Part 3 (BIS, Citation2018b), respectively.
5.2 Tests to determine hardened concrete properties
The hardened concrete properties, namely compressive strength, flexural strength, split tensile strength, modulus of elasticity, ultrasonic pulse velocity (UPV), and abrasion resistance for all 17 mixes were evaluated. A total of 238 (14 per mix in 17 mixes) cube specimens of size 150 mm x150 mm x150 mm were prepared and tested as per IS 516 – Part 1 (BIS, Citation2021) at 28 days of moist curing to evaluate the compressive strengths for all mixes. Asphalt is temperature susceptible; hence, to check the influence of concrete properties at elevated temperatures, the compressive strength of concrete containing RAP was determined at different temperatures (40°C, 50°C, 60°C, and 70°C) besides the ambient temperature at 28 days of moist curing. After completing the required wet curing period, tested the specimens on the compression testing machine of 300 tons capacity. The compressive strength was calculated using EquationEq. 1(1)
(1) .
Where, fc denotes compressive strength, in N/mm2(MPa); F is the maximum load, in N; and Ac represents the cross-sectional area of the specimen (in mm2).
An average of three values was taken as the representative of the batch and compressive strength was expressed to the nearest 0.5 N/mm2. The failure pattern of all specimens was observed and the failure reasons were analyzed. Tested cube specimens containing 100% C-RAP with 4.12% asphalt (mix designation – 100CR4.12) shown in .
Uniform distribution of RAP can be observed in the tested cube. However, the failure pattern was unsatisfactory as only one side of the specimen was damaged completely. The formation of tensile crack on the specimen’s surface due to two different materials (natural and RAP aggregates) may lead to this failure. A similar failure pattern was observed in tested cubes for the mixes designated as 100CR4.12, 75CR4.12, 100CR3.05, and 75CR3.0. The failure pattern of other mixes was satisfactory. Evidently, the percentage of asphalt content in the C-RAP has mainly influenced the failure pattern.
To evaluate the flexural strength of concrete, 51 beam samples (three per mix) of size 100 mmx100mmx500mm were produced and tested in accordance with IS 516 – Part 1 (BIS (Bureau of Indian Standards), Citation2021) at 28 days. The flexural strength was calculated using EquationEq. 2(2)
(2) and the value was expressed to the nearest 0.05 Mpa.
where fb denotes flexural strength, in MPa; P is the maximum load applied, in N; B and D are the lateral dimensions (breadth and height) of the specimen, mm; and L is the length of span (in mm) on which the specimen is supported.
shows a schematic diagram of the flexural strength setup. The beams failed mostly due to fracture at the center, that is, in between two top rollers.
Thirty four cylindrical moulds (two per mix) of size 150 mm diameter and 300 mm height were prepared and tested at 28 days for evaluating the split tensile strength in accordance with IS 516 – Part 1 (BIS (Bureau of Indian Standards), Citation2021) guidelines. The split tensile strength of hardened concrete was determined using Eq. 3 and expressed the value to the nearest 0.05Mpa.
where P = maximum load applied to the specimen, in N; l = length of the cylinder, in mm; and d = cross-sectional dimension of the cylindrical specimen, in mm.
The modulus elasticity of concrete is an essential input parameter used for the design of concrete pavement. Several empirical equations were used for the control mix to determine the modulus of elasticity based on the compressive strength of concrete. However, those equations may be invalid for the concrete prepared with the RAP aggregates. Therefore, a modulus of elasticity test was conducted for the hardened concrete contains C-RAP with different proportions. To determine the modulus of elasticity, 17 cylindrical samples (size, 150 mm diameter and 300 mm height) were prepared. Following the IS 516 – Part 8 (BIS, Citation2020) specifications, the samples were tested on the 300 tons compressive testing machine. depicts the test setup showing the placing of the sample and fixing of strain gauges. The basic stress equal to one-ninth (σb = fc/9 N/mm2) and ultimate loading stress equal to one-third (σb = fc/3) of 28-days compressive strength of cube was applied, and the corresponding strains (ɛb) and ɛa,, respectively, were measured. Using Eq. 4, the modulus of elasticity was determined for all the hardened concrete mixes.
Where σa (fc/3) denotes the upper loading stress, in N/mm2; σb (fc/9) is the basic stress, in N/mm2
Furthermore, to assess the quality of concrete and its packing characteristics, the Ultrasound Pulse Velocity (USPV) test was conducted () as per IS 516 – Part 5 (BIS, Citation2018c) and the time taken for the pulse to pass through 150 mmx150mmx150mm size cube samples was determined. The cube samples that were prepared to determine the compressive strength were used for conducting the UPV test.
Owing to the reduction of friction on the pavement surface, IRC: 58 (IRC (Indian Road Congress), Citation2015) rigid pavement design guidelines suggested increasing the PQC layer’s thickness by 10 mm over the designed thickness for allowing two-times retexturing during its design life of 30 years. Several studies have documented that abrasion/wearing of concrete pavement surface is caused either due to movement of heavily loaded traffic or the strength of concrete or aggregates properties, or quality of construction. This abrasion was responsible for reducing friction (García et al., Citation2012; Naik et al., Citation1994). Therefore, to evaluate resistance to abrasion of hardened concrete mixes in terms of loss of material, produced 102 cylindrical samples (six samples per mix) of 100 mm diameter and 100 mm height and tested at 28 days of moist curing in accordance with American Society for Testing and Materials, C1747(ASTM, Citation2013) guidelines. As per the guidelines, the weight of three specimens prepared from each mix was measured and placed in the Los-Angeles abrasion machine without the addition of abrasion charge in the form of steel spheres and was allowed to rotate for 500 revolutions at 33 rpm. Besides, three more specimens were placed with the addition of steel spheres. Subsequently, the specimens were removed from the abrasion machine, wiped with a cloth to remove the loose particles, and the weight of the specimens was recorded. Abrasion value is expressed in terms of loss of material reported as a percentage. It is the ratio of the difference in the weight of the sample before and after abrasion to the weight before abrasion and multiplied by 100. depicts the condition of the samples prepared with a mix designated as 50CR4.12 both before and after the abrasion test.
6.0 Results and discussions
6.1 Properties of fresh concrete
Slump value
The slump value of fresh concrete mixes recorded to the nearest 5 mm is shown in . Slump values increase with an increase in the percentage of C-RAP. Further, slump values also increased for the same percentage of C-RAP containing higher asphalt content. As an illustration, the slump value of mixes with 100% C-RAP comprises 4.12, 3.05, 2.12, and 1.24% of asphalt were increased to 60.71, 42.86, 42.86, and 28.57% as compared to the control mix. The rise in slump values might be because of less water absorption, removal of all dust/agglomerated particles on RAP and rounded shape RAP particles due to asphalt coating over it. However, changes in slump value were not significant compared to the control mix with the addition of 25% C-RAP irrespective of asphalt content present in it. Usage of admixtures for increasing the slump can be limited if RAP is added to the concrete.
Density
Contrary to the slump values, the density of fresh concrete mixes registered a decrease with an addition of C-RAP. Also, the further reduction was observed in density for the C-RAP containing higher asphalt content (). The highest percentage of reduction in density (3.81%) compared to the density of referral mix was observed for the mix designated as 100CR4.12, which was expected as that mix prepared by replacing the 100% of natural aggregates with 100% C-RAP containing asphalt content of 4.12%. However, the percentage reduction of density was 1.68 for the mix designated as 100CR1.24. This result demonstrates the low specific gravity of C-RAP than the virgin coarse aggregate was accountable for reducing density. The specific gravity of C-RAP is further influenced by the amount of asphalt present in it, that is, more the asphalt content in the RAP, less the specific gravity, and vice versa (ref. ).
6.2 Hardened concrete properties
Compressive strength
The compressive strengths of hardened concrete at 7 and 28 days of moist curing were depicted in .
With the increase in the percentage of C-RAP, the compressive strength decreased as compared to the control mix. Also, the reduction in compressive strength was high for the same percentage of C-RAP containing higher asphalt content. For instance, compared to the compressive strength of the control mix, the strength reduction for seven days of moist curing of concrete was 71.2, 58.6, 42.6, and 21.3% and for 28 days of moist curing, it was 57.2, 47.6, 30.0, and 12.2% with replacement of 100% C-RAP containing 4.12, 3.05, 2.12, and 1.24% of asphalt, respectively. The strength reduction may be attributed that the asphalt film on the aggregate surface acts as barrier between the aggregates and cement, which resulted weak mechanical interlocking between them. Besides, the percentage reduction of strength was more at 7 days of curing as compared to the 28 days curing period. This distinctiveness explains that the addition of RAP into a concrete mix required sufficient time to gain strength. Reduction of strength with the addition of C-RAP having low asphalt (1.24%) is less, which is due to two reasons, namely, less film thickness, and asphalt is partly coated over the surface of aggregates. Normally, the asphalt content in the surface layer of the pavement reduces with the age due to oxidization and it becomes stiff and brittle. If the hardened/aged asphalt concrete is removed through milling process, it is possible to get the RAP containing less percentage of asphalt and exposed aggregate faces (as the stiff asphalt separates from the aggregates during milling process). Using such RAP in the production of concrete does not have much influence on the strength.
As reported in various studies (S. Debbarma et al., Citation2019; Huang et al., Citation2005), a decrement in compressive strength with the addition of C-RAP aggregates was observed. However, contrary to most of the previous studies (Brand et al., Citation2014; Brand & Roesler, Citation2015), the reduction in strength was up to 50% for 100% addition of C-RAP. Instead, the percentage reduction of strength depends on both percentages of RAP aggregates in the concrete replaced with natural aggregates and the percentage of asphalt present in RAP.
IRC:44 (IRC (Indian Road Congress), Citation2017) guidelines stipulated that the laboratory target compressive strength at 28 days for the PQC should be 48.25 MPa. Besides the control mix, the compressive strength value was found to be ≥48.25 MPa for the mixes 75CR1.24, 50CR2.12, 50CR1.24, 25CR2.12, and 25CR1.24. The compressive strength at 28 days was >40 Mpa (minimum strength recommended for PQC mixes) for the mixes 100CR1.24, 75CR2.12, 50CR3.05, 25CR4.12, and 25CR3.05, and it is possible to achieve the required strength of these mixes with an increment of cement content or addition of cementitious admixtures.
As asphalt is sensitive to temperature, the compressive strength values for all the mixes containing C-RAP were checked at different temperatures 40°C, 50°C, 60°C, and 70°C besides the room temperature. After 28 days of moist curing, the cube specimens were removed from the curing tank, dried until the surface to make them free from moisture and kept in the four different ovens for 24 hours by maintaining the aforementioned temperatures and then tested. Test results are summarized in . For the control mix, no variation in compressive strength was noticed at different temperatures. For the mixes containing C-RAP, no change was observed in the strength up to 50°C, whereas a slight decrement in the strength was witnessed to a maximum of 5.4% and 8.3% at 60°C and 70°C, respectively, compared to the strength at room temperature. It was observed that the aged/hardened asphalt presence in the C-RAP did not change up to 50°C. Beyond this temperature, asphalt softened and disturbed the internal structure, resulting in a reduction in strength. However, at 60°C, the maximum possible pavement temperature during summer, the decrement in strength is < 5.4%; hence, temperature variation is not required to be considered seriously.
Flexural strength
The flexural strength for all 17 mixes was measured at 28 days of moist curing by averaging three beams of each mix. The results are presented in . Flexural strength values decreased on the addition of C-RAP to the concrete and mostly showed a similar trend of compressive strength. The extent of reduction is further dependent on the amount of asphalt presence in the C-RAP. With the addition of 100, 75, 50, and 25% of C-RAP containing 4.12% of asphalt, the percentage reduction in flexural strength was 39.58, 27.08, 16.67, and 10.42%, respectively, as compared to the control mix. With the replacement of 100% C-RAP containing 4.12, 3.05, 2.12, and 1.24% of asphalt, the reduction in strength was observed to be 39.58, 25.00, 14.58, and 10.42%, respectively. Further, the percentage of reduction in flexural strength related to the control mix was lower than the reduction of compressive strength at 28 days of moist curing of concrete mixes containing RAP aggregates. For instance, the reduction of flexural strength was 39.58, 25.00, 14.58, and 10.42%, and compressive strength was 57.2, 47.6, 30.0, and 12.2% as compared to the corresponding strengths of control mixes with the replacement of 100% C-RAP containing 4.12, 3.05, 2.12, and 1.24% asphalt content, respectively. This can be explained that RAP aggregates present in the concrete enhanced its flexibility, which delayed the sudden failure of the specimen as in the bending test.
Flexural strength is an essential input parameter that is considered for rigid concrete pavement design as the number of loading repetitions allowed on the pavement depends on it. The IRC:58 (IRC (Indian Road Congress), Citation2015) concrete pavement design guidelines suggested the minimum flexural strength of PQC should be 4.5 MPa at 28 days of moist curing. Besides the control mix, the flexural strength value was found to be ≥4.5 MPa for the mixes 100CR1.24, 75CR2.12, 75CR1.24, 50CR2.12, 50CR1.24, 25CR3.05, 25CR2.12, and 25CR1.24, which satisfied the requirements of the guidelines. The flexural strength values were between 4.0 and 4.5 MPa for the mixes 100CR2.12, 75CR3.05, 50CR4.12, 50CR3.05, and 25CR4.12, and the strength value of these mixes could be improved to achieve 4.5 MPa with the increasing of cement content up to the limiting value or addition of cementitious admixtures or reduce of RAP content in the mix. Results also showed that up to 75% of C-RAP can be added if the percentage of asphalt present in the RAP is 1.24. With a little increment of cement/cementitious admixtures, 100% of C-RAP containing 1.24 and 2.12% of asphalt, and 75% of C-RAP containing 2.12 and 3.05% asphalt can be added.
Split tensile strength
presents the split tensile strength results measured at 28 days of moist curing. The addition of C-RAP in the concrete exhibited a decrement in split tensile strength. As shown in the Figure, it follows a trend similar to compressive and flexural strength. Compared to the referred mix strength, ~ 44.1, 33.33, 25.00, and 16.67% decrement in strength was observed for 100, 75, 50, and 25% replacement of C-RAP containing 4.12% asphalt. Nevertheless, a lower percentage of asphalt in C-RAP demonstrated less strength variation than strength obtained in the control mix. For instance, after replacing 100% NCA with 100% C-RAP containing 4.12, 3.05, 2.12, and 1.24% asphalt content, the percentage reduction recorded in strength was 44.1, 36.11, 27.78, and 11.11. This reveals that RAP aggregates having lesser asphalt content did not have much influence on the hardened RAP concrete as they behave like natural aggregates with coarse/rough surface texture.
Modulus of elasticity
The modulus of elasticity is considered to be one of the strength parameters. Therefore, a trend similar to other strength parameters, as discussed above, was followed. depicts the modulus values obtained at 28 days of moist curing for hardened concrete mixes. As seen in , low modulus values were reported for the mixes prepared with higher fractions of RAP aggregates having a higher percentage of asphalt. For instance, decrement in modulus values to 40.8, 29.27, 22.57, and 12.25% with 100, 75, 50, and 25% of C-RAP having 4.12% of asphalt. Close observation of the percentage reduction in strength values related to the referral mix demonstrates that flexural strength, split tensile strength, and modulus of elasticity values followed a parallel trend. The minimum modulus of elasticity value of concrete recommended by IRC:58 (IRC (Indian Road Congress), Citation2015) at 28 days moist curing for PQC was 30,000 MPa. As per , except for the mix designated 100CR4.12, the modulus value was more than the trigger value for all other mixes.
Ultrasound pulse velocity
The average value of pulse velocity of three samples in each mix was recorded. The recorded pulse velocity value for all the mixes was > 4.5 km/sec. According to IS 516 – Part 5 (BIS (Bureau of Indian Standards), Citation2018c), the quality of concrete based on the USPV values is presented in .
Table 7. Quality criteria suggested for hardened concrete based on USPV values.
Based on the results, the quality of concrete mixes was found excellent. This showed that the specimens prepared by replacing the C-RAP of various percentages were in good condition, homogeneity in the mix, mixes were well compacted, and no internal flaws.
Abrasion resistance
The percentage loss of material in abrasion test with and without abrasion charge (in the form of steel spheres) for all the mixes is presented in . The loss of material without and with an abrasion charge due to abrasion of the control mix was 13.9% and 15.3%, respectively. The material loss was 23.8, 22.0, 19.1, and 15.9% without abrasion charge and 25.4, 23.7, 21.3, and 17.8% with abrasion charge for the concrete in which NCA replaced with 100, 75, 50, and 25% of C-RAP having 4.12% of asphalt. The abrasion loss of concrete contained RAP was more compared to the control mix. Owing to the poor bonding between aggregates contain asphalt film and cement was responsible. Also, abrasion was found to be more for the concrete containing RAP aggregates with a higher amount of asphalt. The more the asphalt in RAP, the more is the thickness of the film; this further reduces the bonding and causes additional loss. Loss of material with abrasion charge was ~10% more as compared to that without abrasion charge. This may attribute that with cement paste/mortar on the surface, further wear of material does not take place.
7.0 Development of models between various strength parameters
Various guidelines (refer to ) have suggested empirical models for estimating the concrete strength parameters, namely flexural strength (fb in, N/mm2), split tensile strength (fe in, N/mm2), and modulus of elasticity (Ec in, N/mm2) based on the compressive strength (fc in, N/mm2) of control/plain concrete at 28 days of curing.
Table 8. Empirical relationships between fb and fc;fe and fc;Ec and fc.
The empirical models provided in may not be valid for the concrete containing RAP aggregates. Therefore, separate models were developed to predict fb,fe, and Ec (all are expressed in N/mm2), using the following predictor variables which were expected to be highly correlated.
PC-RAP – Quantity of C-RAP in the concrete expressed in percentage (%)
Pb – Asphalt present in the C-RAP expressed in percentage (%)
fc – Compressive strength of concrete contains NCA/RAP aggregates expressed in N/mm2
7.1 Correlation Matrix for fb, fe, and Ec
First, a correlation matrix was developed between dependent and all predictor variables to check the degree of correlations between them. Hardened concrete parameters fb,fe, and Ec correlated well to all predicted variables, as presented in . It can be observed that fb,fe, and Ec showed a negative correlation to PC-RAP and Pb while a positive correlation to concrete compressive strength (fc). The negative correlation can be explained by either increasing the percentage of RAP in the concrete or more asphalt in the RAP, the concrete strength parameters decrease. The primary and most influential parameter for fb,fe, and Ec was compressive strength with a high correlation greater than 96% for all the cases, and the second influenced parameter was asphalt content in RAP with a correlation greater than 71%. In addition to the correlation matrix, the impact of predictor variables on the dependent variable was further studied using the P-value significance test at a 95% confidence interval. Moreover, the P-values were determined for each of the dependent and predictor variables (). It can be observed that the dependent parameters fb,fe, and Ec are well correlated with all predictor variables as the P-value is < 0.05 in all the cases.
Table 9. Correlation matrix and P- significance value (in parentheses) associated with fb,fe, and Ec models.
7.2 Models for predicting fb, fe, and Ec
Multiple nonlinear regression models were developed considering each fb,fe, and Ec as a dependent variable and PC-RAP,Pb, and fc predictor variables and presented as Equation (5), (6), and (7).
R2adj = 0.9278 (5)
R2adj = 0.9276 (6)
R2adj = 0.9284 (7)
Where fb = flexural strength of Concrete, in N/mm2 at 28 days; fe = split tensile strength of Concrete, in N/mm2 at 28 days; Ec = static modulus of elasticity of Concrete, in N/mm2 at 28 days; PC-RAP – Quantity of C-RAP in the concrete expressed in percentage (%); Pb – Asphalt present in the C-RAP expressed in percentage (%); fc – Compressive strength of concrete contains NCA/RAP aggregates expressed in N/mm2 at 28 days.
The coefficients of predictive model fb,fe, and Ec parameters, t-stat, standard error estimates, and upper and lower bound limits of the 95% confidence intervals are presented in . The percentage of C-RAP and asphalt content in the C-RAP had a negative correlation but the compressive strength had a positive correlation and was statistically significant.
Table 10. Parameters estimate for flexural strength model.
Table 11. Parameters estimate for split tensile strength model.
Table 12. Parameters estimate for static modulus of elasticity model.
7.3 Validation of the models
Validation of the models was carried out to check their predictive efficiency using the hardened concrete properties other than the mixes used for the development of models. For the validation, milled RAP was collected from two different sources (RAP1 and RAP2) of 4 years and 7.5 years old pavement. RAP was divided into coarse (C-RAP1, C-RAP2) and fine fractions (F-RAP1, F-RAP2). The asphalt content from the C-RAP1 and C-RAP2 was found at 4.68% and 3.75%, respectively. Mixes were prepared by replacing NCA with C- RAP of different fractions varying from 25% to 100% with an increment of 25% and M-Sand was used as fine aggregates. The mix proportions are provided in . As observed, cement content was varied for the mixes prepared with different fractions of C-RAP1 and C-RAP2. This was done to check the model perfections for the lower-grade mixes. Eight concrete mixes (4 each with C-RAP1 and C-RAP2) were prepared and their hardened properties evaluated. The validation results of fb,fe, and Ec are shown in . As seen in the figure, the predictions are very accurate as the R2 value was high.
Table 13. Mixes designation and quantities of individual aggregates.
8.0 Conclusions
The main objective of this study was to investigate the properties of concrete by replacing the natural coarse aggregates with different fractions of C-RAP aggregates having various percentages of asphalt. The study involved collection of reclaimed asphalt pavement aggregates, separating the RAP into coarse and fine fractions, reducing the asphalt content in the C-RAP, studying the mechanical properties of C-RAP, designing cement concrete mixes containing different fractions of C-RAP aggregates, preparing the cement concrete mixes, evaluating fresh and hardened concrete properties, and developing relationships between RAP concrete properties in its hardened state. The significant inferences from the present study are given below:
Properties of RAP aggregates: Owing to the presence of low-density asphalt in RAP, the density and specific gravity of RAP aggregates decreased with the increasing percentage of asphalt in it. The asphalt-coated aggregates display low absorptivity; therefore, RAP aggregates with higher asphalt content reported less water absorption and vice versa.
RAP concrete properties in its fresh state: Incorporation of C-RAP content in concrete, fresh concrete slump values increased, and density decreased compared to the control mix. Less water absorption and rounded shape of C-RAP aggregates were responsible for the rise in slump values. The low specific gravity of C-RAP than the virgin coarse aggregate was accountable for the reduction in density. More the asphalt content in C-RAP, further increase in slump values and decrease in density was noticed. Admixtures used for increasing slump can be avoided if RAP is used in concrete.
RAP concrete properties in its hardened state: a) The hardened properties of RAP concrete decreased as compared to control mix with the increase in the fraction of C-RAP content. Besides, for the same fraction of C-RAP, further decrease in values of the properties was observed with an increase in the asphalt content in it. In this study, the proportion of C-RAP comprised four asphalt content (4.12, 3.05, 2.12, and 1.24%) was replaced with NCA. Properties of RAP concrete were found less for the same fraction of C-RAP, having higher asphalt content. The poor bonding between the asphalt coated aggregates and cement resulted in reducing the strength. The reason behind the decrease in properties for the higher asphalt content was asphalt film thickness. It means the more the asphalt in RAP, the thickness of the film is more, which further reduces the bonding, thereby decreasing the strength of material. b) The minimum suggested compressive and flexural strength values for PQC mixes are 40 Mpa and 4.5 Mpa, respectively, which were achieved by replacing 100 and 75% of NCA with C-RAP having 1.24 and 2.12% asphalt, respectively. Generally, aged and oxidized RAP contains low asphalt in the order of 2% is a well-suited alternative to NCA. If the bitumen content is more than 2% in the C-RAP, it is advantageous using C-RAP for the preparation of bituminous mixes. However, if it is proposed to use C-RAP containing asphalt content greater than 2% in the preparation of concrete mixes, minimize the replacement of NCA with C-RAP, and add cementitious/supplementary material to achieve the required strength.
Empirical Models: The empirical models developed in the current study had a good correlation and were statistically significant, supported by
. These models can be used to predict RAP concrete hardened properties (fb,fe, and Ec) for the known percentage of C-RAP, percentage of asphalt in C-RAP, and its compressive strength. Models validation showed the accuracy of the estimates as the R2 value was high.
Disclosure statement
No potential conflict of interest was reported by the author(s).
References
- Abed, A., Thom, N., & Lo Presti, D. (2018). Design considerations of high RAP-content asphalt produced at reduced temperatures. Materials Structural, 51(4), 91. https://doi.org/10.1617/s11527-018-1220-1
- ACI-318. (2014). Building code requirements for structural concrete: (ACI 318-14). Farmington, MI: American Concrete Institute.
- Al-Oraimi, S., Hassan, H. F., & Hago, A. (2009). Recycling of reclaimed asphalt pavement in Portland cement concrete. Journal of Engineering Research, 6(1), 37–27. https://doi.org/10.24200/tjer.vol6iss1pp37-45
- ASTM. (2013). Standard test method for determining potential resistance to degradation of pervious concrete by impact and Abrasion. ASTM C1747/C1747M.
- Bentsen, R. A., Vavrik, W. A., Roesler, J. R., & Gillen, S. L. (2013). Ternary blend concrete with reclaimed asphalt pavement as an aggregate in two-lift concrete pavement. Proc., 2013 Int. Concrete Sustainability Conf (pp. 1–13). Silver Spring, MD, USA: National Ready Mixed Concrete Association. https://intrans.iastate.edu/app/uploads/sites/7/2018/08/1-Ternary-Blend-Concrete-with-Reclaimed-Ashpalt.pdf
- Berry, M., Kappes, B., & Kappes, L. (2015). Optimization of concrete mixtures containing reclaimed asphalt pavement. ACI Materials Journal, 112 723–733. https://doi.org/10.14359/51687854
- BIS. (1988b). Indian standard methods of physical tests for hydraulic cement: Determination of compressive strength of hydraulic cement other than masonry cement, IS. 4031 - Part 6.
- BIS. (1988c). Indian standard methods of physical tests for hydraulic cement: Determination of consistency of standard cement paste, IS:4031 - Part 4, BIS, Reaffirmed 2005. Bureau of Indian Standards.
- BIS. (1988d). Indian standard methods of physical tests for hydraulic cement: Determination of soundness, IS:4031 - Part 3, BIS, Reaffirmed 2005. Bureau of Indian Standards.
- BIS. (1999). Indian standard methods of physical tests for hydraulic cement: Determination of fineness by blaine air permeability method, IS:4031 - Part 2.
- BIS. (2000). Plain and reinforced concrete code of practice. In 4th Revision, IS 456, BIS. Bureau of Indian Standards.
- BIS. (2013). Ordinary portland cement, 53 Grade — Specification, IS 12269.
- BIS. (2018a). Fresh concrete – methods of sampling and testing: Determination of consistency of fresh concrete, IS 1199 – Part 2.
- BIS. (2018c). hardened concrete - methods of test: Ultrasonic pulse velocity testing. IS:516 – Part 5/Sec 1.
- BIS. (2020). Hardened concrete - methods of test: Determination of modulus of elasticity of concrete. IS:516 – Part 8.
- BIS. (2021). Hardened concrete - methods of test: Testing of compressive, flexural, and split tensile strength hardened concrete. IS:516 – Part 1.
- BIS (Bureau of Indian Standards). (1963). Methods of test for aggregates for concrete: Specific gravity, density, voids, absorption and bulking, IS:2386-PartIII.
- BIS (Bureau of Indian Standards). (1988a). indian standard methods of physical tests for hydraulic cement: Determination of initial and final setting times, IS:4031 - Part 5, BIS, Reaffirmed 2005. Bureau of Indian Standards.
- BIS (Bureau of Indian Standards). (2018b). Fresh concrete – methods of sampling and testing: Determination of density of fresh concrete, IS 1199 – Part 3.
- Brand, A. S., Amirkhanian, A. N., & Roesler, J. R. (2014). Flexural capacity of full-depth and two-lift concrete slabs with recycled aggregates, Transp. Research Record, 2456(1), 64–72. https://doi.org/10.3141/2456-07
- Brand, A. S., & Roesler, J. R. (2015). Ternary concrete with fractionated reclaimed asphalt pavement. ACI Materials Journal, 112(1). https://doi.org/10.14359/51687176
- Brand, A. S., & Roesler, J. R. (2017a). Bonding in cementitious materials with asphalt-coated particles: Part I – The interfacial transition zone. Construction and Building Materials, 130, 171–181. https://doi.org/10.1016/j.conbuildmat.2016.10.019
- Brand, A. S., & Roesler, J. R. (2017b). Bonding in cementitious materials with asphalt-coated particles: Part II – Cement-asphalt chemical interactions. Construction and Building Materials, 130, 182–192. https://doi.org/10.1016/j.conbuildmat.2016.10.013
- CSA. (2004). Design of Concrete Structures CAN/CSA-A23.3-04”. Standards Council of Canada.
- Debbarma, S., Ransinchung, R. N., D, G., & Singh, S. (2019). Feasibility of roller-compacted concrete pavement containing different fractions of reclaimed asphalt pavement. Construction and Building Materials, 199, 508–525. https://doi.org/10.1016/j.conbuildmat.2018.12.047
- Debbarma, S., Selvam, M., & Singh, S. (2020). Can flexible pavements waste (RAP) be utilized in cement concrete pavements? - A critical review. Construction and Building Materials, 259, 1–20. https://doi.org/10.1016/j.conbuildmat.2020.120417
- ECS. (2004). Eurocode 2: design of concrete structures: General rules and rules for buildings, EN 1992-1-1”. CEN.
- Erdem, S., & Blankson, M. A. (2014). Environmental performance and mechanical analysis of concrete containing recycled asphalt pavement (RAP) and waste precast concrete as aggregate. Journal of Hazardous Materials, 264, 403–410. https://doi.org/10.1016/j.jhazmat.2013.11.040
- Fakhri, M., & Amoosoltani, E. (2017). The effect of reclaimed asphalt pavement and crumb rubber on mechanical properties of roller compacted concrete pavement, Constr. Building Materials, 137(2017), 470–484. https://doi.org/10.1016/j.conbuildmat.2017.01.136
- Ferrebee, E. C., Brand, A. S., Kachwalla, A. S., Roesler, J. R., Gancarz, D. J., & Pforr, J. E. (2014). Fracture properties of roller-compacted concrete with virgin and recycled aggregates. Transportation Research Record: Journal of the Transportation Research Board, 2441(1), 128–134. https://doi.org/10.3141/2441-17
- García, A., Castro-Fresno, D., Polanco, J. A., & Thomas, C. (2012). Abrasive wear evolution in concrete pavements. Road Materials and Pavement Design, 13(3), 534–548. https://doi.org/10.1080/14680629.2012.694094
- Guthrie, W. S., Brown, A. V., & Eggett, D. L. (2007). Cement stabilization of aggregate base material blended with reclaimed asphalt pavement. Transportation Research Record: Journal of the Transportation Research Board, 2026(1), 47–53. https://doi.org/10.3141/2026-06
- Hmoud, R. H. (2011). Evaluation of VMA and film thickness requirements in hot-Mix Asphalt. Modern Applied Science, 5(4). https://doi.org/10.5539/mas.v5n4p166
- Hossiney, N., Tia, M., & Bergin, M. J. (2010). Concrete containing RAP for use in concrete pavement. International Journal of Pavement Research and Technology, 3, 251–258.
- Hoyos, L. R., Puppala, A. J., & Ordonez, C. A. (2011). Characterization of cement-fiber-treated reclaimed asphalt pavement aggregates: Preliminary investigation. Journal of Materials in Civil Engineering, 23(7), 977–989. https://doi.org/10.1061/(asce)mt.1943-5533.0000267
- Huang, B., Li, G., Vukosavljevic, D., Shu, X., & Egan, B. K. (2005). Laboratory investigation of mixing Hot-Mix Asphalt with reclaimed asphalt pavement. Transportation Research Record: Journal of the Transportation Research Board, 1929(1), 37–45. https://doi.org/10.1177/0361198105192900105
- Huang, B., Shu, X., & Burdette, E. G. (2006). Mechanical properties of concrete containing recycled asphalt pavements. Magazine of Concrete Research, 58(5), 313–320. https://doi.org/10.1680/macr.2006.58.5.312
- Ibrahim, A., Mahmoud, E., Khodair, Y., & Patibandla, V. C. (2014). Fresh, mechanical, and durability characteristics of self-consolidating concrete incorporating recycled asphalt pavements. Journal of Materials in Civil Engineering, 26(4), 668–675. https://doi.org/10.1061/(asce)mt.1943-5533.0000832
- IRC. (2017). Tentative guidelines for cement concrete mix design for pavements. IRC:44.
- IRC (Indian Road Congress). (2015). Guidelines for the design of plain jointed rigid pavements for highways. IRC:58.
- Katsakou, M., & Kolias, S. (2007). Mechanical properties of cement-bound recycled pavements, Proc. ICE Constr. Mater. 160 171–179. https://doi.org/10.1680/coma.2007.160.4.171.
- Li, X., Williams, R. C., Marasteanu, M. O., Clyne, T. R., & Johnson, E. (2009). Investigation of in-place asphalt film thickness and performance of Hot-Mix Asphalt Mixtures. Journal of Materials in Civil Engineering, 21(6), 262–270. https://doi.org/10.1061/(ASCE)0899-1561(2009)21:6(262)
- Mahmoud, E., Ibrahim, A., El-Chabib, H., & Patibandla, V. C. (2013). Self-Consolidating concrete incorporating high volume of fly ash, slag, and recycled asphalt pavement. International Journal of Concrete Structures and Materials, 7(2), 155–163. https://doi.org/10.1007/s40069-013-0044-1
- Mansourkhaki, A., Ameri, M., Habibpour, M., & Shane Underwood, B. (2020). Chemical composition and rheological characteristics of binders containing RAP and rejuvenator. Journal of Materials in Civil Engineering, 32(4), 04020026. https://doi.org/10.1061/(ASME)MT.1943-5533.0003061
- Mathias, V., Sedran, T., & de Larrard, F. (2009). Modelling of mechanical properties of cement concrete incorporating reclaimed asphalt pavement. Road Materials and Pavement Design, 10(1), 63–82. https://doi.org/10.1080/14680629.2009.9690182
- Moaveni, M., Cetin, S., Brand, A. S., Dahal, S., Roesler, J. R., & Tutumluer, E. (2016). Machine vision-based characterization of particle shape and asphalt coating in Reclaimed Asphalt Pavement. Transportation Geotechnics, 6, 26–37. https://doi.org/10.1061/j.trgeo.2016.01.001
- Modarres, A., & Hosseini, Z. (2014). Mechanical properties of roller-compacted concrete containing rice husk ash with original and recycled asphalt pavement material, Mater. Design, 64(2014), 227–236. https://doi.org/10.1016/j.matdes.2014.07.072
- Mohammadafzali, M., Ali, H., Sholar, G. A., Rilko, W. A., & Baqersad, M. (2018). Effects of rejuvenation and aging on binder homogeneity of recycled asphalt mixtures. Journal of Transportation Engineering Part B. Pavements, 145(1), 4018066. https://doi.org/10.1061/JPEODX.0000089
- Naik, T. R., Singh, S. S., & Hossain, M. M. (1994). Abrasion resistance of concrete as influenced by the inclusion of fly ash. International Journal of Cement and Concrete Research, 24(2), 301–312. https://doi.org/10.1016/0008-8846(94)90056-6
- Noferini, L., Simone, A., Sangiorgi, C., & Mazzotta, F. (2017). Investigation on performances of asphalt mixtures made with Reclaimed Asphalt Pavement: Effects of interaction between virgin and RAP asphalt. International Journal of Pavement Research and Technology, 10(4), 322–332. https://doi.org/10.1016/j.ijprt.2017.03.011
- NZS (New Zealand Standard). (2006). Concrete structures standard: Part 1 - The Design of Concrete Structures, NZS 3101-1, New Zealand, the trading arm of the Standards Council, Private Bag 2439. NZS.
- Puppala, A. J., Hoyos, L. R., & Potturi, A. K. (2011). Resilient moduli response of moderately cement-treated reclaimed asphalt pavement aggregates. Journal of Materials in Civil Engineering, 23(7), 990–998. https://doi.org/10.1061/(asce)mt.1943-5533.0000268
- Sachet, T., Albuquerque, M. C. F., Balbo, J. T., & Sansone, C. E. (2011). Investigation of resistance and fracture parameters for compacted concrete with incorporation of reclaimed asphalt pavement. International Journal of Pavements, 10(1–3), 83–93.
- Saghafi, M., Tabatabaee, N., & Nazarian, S. (2019). Performance evaluation of slurry seals containing reclaimed asphalt pavement. Transportation Research Record, 2673(1), 358–368. https://doi.org/10.1177/0361198118821908
- Sengoz, B., & Agar, E. (2007). Effect of asphalt film thickness on the moisture sensitivity characteristics of hot-mix asphalt. Building and Environment, 42(10), 3621–3628. https://doi.org/10.1016/j.buildenv.2006.10.006
- Settari, C., Debieb, F., Kadri, E. H., & Boukendakdji, O. (2015). Assessing the effects of recycled asphalt pavement materials on the performance of roller-compacted concrete. Construction and Building Materials, 101, 617–621. https://doi.org/10.1016/j.conbuildmat.2015.10.039
- Shi, X., Mukhopadhyay, A., & Liu, K. W. (2017). Mix design formulation and evaluation of Portland cement concrete paving mixtures containing reclaimed asphalt pavement. Construction and Building Materials, 152(Oct), 756–768. https://doi.org/10.1016/j.conbuildmat.2017.06.174
- Shi, X., Mukhopadhyay, A., & Zollinger, D. (2018). Sustainability assessment for Portland cement concrete pavement containing reclaimed asphalt pavement aggregates. Journal of Cleaner Production, 192, 569–581. https://doi.org/10.1016/j.jclepro.2018.05.004
- Singh, S., Ransinchung, R. N., D, G., & Kumar, P. (2018a). Performance evaluation of RAP concrete in aggressive environment. Journal of Materials in Civil Engineering, 30(10), 04018231. https://doi.org/10.1061/(asce)mt.1943-5533.0002316
- Singh, S., Ransinchung, G. D., & Kumar, P. (2017). Feasibility study of RAP aggregates in cement concrete pavements. Road Materials Pavement Des, 1–20. https://doi.org/10.1080/14680629.2017.1380071
- Singh, S., Ransinchung, G. D. R. N., Monu, K., & Kumar, P. (2018b). Laboratory investigation of RAP aggregates for dry lean concrete mixes. Construction and Building Materials, 166, 808–816. https://doi.org/10.1016/j.conbuildmat.2018.01.131
- Singh, S., Ransinchung, G. D. R. N., Monu, K., & Kumar, P. (2019). Sustainable lean concrete mixes containing wastes originating from roads and industries. Construction and Building Materials, 209(Jun), 619–630. https://doi.org/10.1016/j.conbuildmat.2019.03.122
- Su, Y. M., Hossiney, N., Tia, M., & Bergin, M. (2014). Mechanical properties assessment of concrete containing reclaimed asphalt pavement using the Superpave indirect tensile strength test. Journal of Testing and Evaluation, 42(2014), 1–9. http://dx.doi.org/10.1520/JTE20130093
- Taha, R. (2003). Evaluation of cement kiln dust-stabilized reclaimed asphalt pavement aggregate systems in road bases, transportation research record. (1819), 11–17. https://doi.org/10.3141/1819b-02
- Topcu, I. B., & Isikdag, B. (2009). Effects of crushed rap on free and restrained shrinkage of mortars. International Journal of Concrete Structures and Materials, 3(2), 91–95. https://doi.org/10.4334/IJCSM.2009.3.2.091
- Visintine, B., Khosla, N. P., & Tayebali, A. (2013). Effects of higher percentage of recycled asphalt pavement on pavement performance. Road Materials and Pavement Design, 14(2), 432–437. https://doi.org/10.1080/14680629.2013.779310
- Xiao, F., Amirkhanian, S., & Juang, C. H. (2007). Rutting resistance of rubberized asphalt concrete pavements containing reclaimed asphalt pavement mixtures. Journal of Materials in Civil Engineering, 19(6), 475–483. https://doi.org/10.1061/(ASCE)0899-1561(2007)19:6(475)
- Yu, X., Dong, F., Xu, B., Ding, G., & Ding, P. (2017). RAP binder influences on the rheological characteristics of foamed warm-mix recycled asphalt. Journal of Materials in Civil Engineering, 29(9), 04017145. https://doi.org/10.1061/(ASCE)mt.1943-5533.0001993
- Zhang, H., Harvey, J., Jiao, L., Li, H., & Elkashef, M. (2020). Study on binder film thickness distribution of recycled asphalt pavements. Journal of Testing and Evaluation, 48(3), 2474–2493. https://doi.org/10.1520/JTE20190624