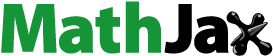
ABSTRACT
The main objective of this study is to choose appropriate solid waste disposal sites based on environmentally friendly, cost-effective, and socially responsible factors using geospatial technology. To achieve the study’s objectives, factor maps such as slope, settlement, road, stream, land use/land cover, geology, borehole, protected area, and soil maps were used and prepared using GIS. The most appropriate solid waste disposal sites were identified and categorized as highly suitable, suitable, less suitable, or unsuitable using a weighted linear combination (WLC) analysis. Through ArcMap overlay analysis, the final suitability map was created. The AHP pairwise comparison module helped establish the weight of factor parameters. The findings reveal that out of the total area, 51.32 hectares are highly suitable, 54.86 hectares are suitable, 34.99 hectares are less suitable, and 3171.22 hectares are unsuitable for solid waste disposal. The use of GIS and remote sensing data has allowed for a comprehensive evaluation of multiple factors and their weighted importance, resulting in a final suitability map that can guide decision-makers in selecting appropriate sites for solid waste disposal. In conclusion, the study provides a practical solution to the problem of solid waste disposal by using geospatial technology.
1. Introduction
Solid waste has become a major global environmental and health concern in both developed and developing nations in the modern era (UN, Citation2017). Along with associated social, economic, and land availability issues, these environmental challenges give rise to concerns regarding land management and evaluation practices (Coban et al., Citation2018). Waste, a byproduct of several human activities, causes serious problems in human environments. Globally, the production of solid waste has increased as a result of poor habits, population growth, and fast economic expansion (Hering, Citation2012). The deteriorating state of the environment was the most noteworthy development for the world community throughout the second half of the 20th century. The anthropogenic environmental degradation resulting from urbanization, rising consumption, population growth, and increased industrial and agricultural output is undoubtedly putting the world’s civilization in jeopardy of collapsing (Shkileva, Citation2021). Waste has been a problem for humanity from the start, and it is a growing issue that affects every country on the globe (Berisa and Birhanu, Citation2015). Solid waste management is a global issue that both developing and less developed countries must deal with. Solid waste generation is accelerating globally due to rising community living standards, rapid economic growth, and population growth (Elmira et al., Citation2011). Solid waste creation has evolved into a widespread environmental and health challenge in the modern world, affecting both rich and developing nations. Growing urban and industrial activity, rapid economic growth, rising living standards, and a growing population all contribute to an increase in the production of solid waste (Ebistu & Minale, Citation2013).
Since urbanization started in the 1960s in developing nations like Africa, waste output has increased, and land availability in rural regions has become scarcer. The practice of discarding waste into open pits exacerbates this problem and is especially common in the impoverished areas of most African towns (Hammer, Citation2003). In the past, the governments of several African states allowed the unrestricted disposal of waste at deserted quarry sites without hygienic landfills, which resulted in major health issues (Martin & Williams, Citation1992). The majority of solid waste disposal sites in Ethiopian cities and towns are found close to settlements, fault lines, water sources, and the sides of roads. The improper disposal of solid waste results in various negative effects on the environment and human health, including contaminated surface and groundwater, soil contamination from direct waste associates, greenhouse gas emissions, pollution of the environment, harm to people and property, and a decrease in business and tourism (EGSSAA, Citation2009). Because of this, the bulk of illnesses in the country – including cholera, diarrhea, dysentery, and other infections – are associated with poor environmental sanitation, contaminated water, a dearth of suitable disposal sites for waste, public latrines, and inadequate waste disposal management systems in the vicinity, all of which can affect daily activities in the neighborhood (Mekuria, Citation2006).
Depending on the local conditions, a number of important criteria must be established in order to carry out the parametric selection process. The physical, social, and land use components of the criterion may be processed, analyzed, and comprehended through the use of geospatial technologies (Habtamu, Citation2001). Furthermore, the environmental state of the places is mentioned as a determining factor for crucial aspects derived from the physical, demographic, economic, policy, and environmental disciplines. Slope, drainage, schools, roads, urban centers, groundwater wells, soil, population, land use, and land cover were all taken into consideration as research factors, depending on the specifics of the location in question (Collins et al., Citation2001).
Solid waste dumping is a crucial component of the waste management system that requires careful study to minimize its negative environmental and social impacts and hence lessen environmental pollution and health concerns (Gbanie et al., Citation2013). Unmanageable methods of garbage disposal, which are ultimately a result of inadequate planning and implementation, are to blame for the current global trends in waste management issues (Abbas et al., Citation2011). High rates of urbanization and population growth, together with ineffective and underfunded administrations, obstruct effective garbage management. The disposal of municipal solid trash has been a major issue in developing nations. To find the best solutions for the management of urban solid waste, waste management concerns should be challenged in more generalized systems (Mohammedshum et al., Citation2014). Each day, thousands of tons of solid waste are produced across various parts of Africa. The majority of this debris is dumped in wetlands and open landfills, causing significant health risks by poisoning surfaces and groundwater (EGSSAA, Citation2009). The rate of garbage generation ranges from approximately 0.5 kilograms per person per day to as high as 0.8 kilograms per person per day in a few cities and regions (EGSSAA, Citation2009).
In Ethiopia, most solid waste disposal facilities are located outside of cities and towns, near water sources, farms, villages, highways, and crop fields. Such improper disposal of solid waste causes significant environmental pollution, contaminates surface groundwater and soil, emits greenhouse gases and other air pollutants, harms ecosystems, and causes health problems for people and damage to property while deterring business and tourism (EGSSAA, Citation2009). The largest challenge with managing solid waste is finding proper locations for their disposal far from populated areas, natural resources, and settlements.
The increasing population of Shone Town due to rapid urbanization and migration from rural regions has led to solid waste management issues and increased waste generation. The majority of solid waste created in the town is not collected and is haphazardly disposed of in open areas (Lemma & Tekilu, Citation2014). Thus, finding appropriate sites for solid waste disposal away from populated areas, natural resources, and settlements is the primary concern for solid waste management. Computerized systems, such as geographic information systems and remote sensing, can be integrated to acquire ideal solutions for effective and efficient solid waste management. This tool enables users to analyze spatial information, edit data and maps, and provide results of spatial and non-spatial analysis (Mohammedshum et al., Citation2014).
This study aims to use GIS and RS techniques to find feasible sites for solid waste disposal in the study area while preserving environmental pollution, preventing public health risks, and considering other socioeconomic issues. Since waste disposal site location is one of the most important determinants of sound waste management, it is crucial to integrate environmental, social, and economic factors. Therefore, if a suitable site for solid waste landfilling is selected using GIS and RS technologies while considering environmental and socio-economic criteria, solid waste disposal practices and management may improve.
The primary goal of this research is to contribute to the improvement of solid waste management practices in Shone Town, Ethiopia, as well as other similar regions. By utilizing GIS and RS technologies and taking into account environmental and socio-economic factors, this study endeavors to locate optimal solid waste disposal sites, providing a balance between effective waste management and the preservation of public health and sustainability. These findings have the potential to influence waste management policies and practices, leading to widespread efforts aimed at reducing the damaging effects of waste on both the environment and human health. Specifically, this study has four main objectives: Firstly, to utilize GIS and RS technologies to pinpoint suitable sites for solid waste disposal while considering key environmental factors such as slope, land usage, and proximity to water sources. Secondly, to analyze social factors, including population density and proximity to residential areas and transportation systems, in order to ensure the chosen location is both accessible and secure. Thirdly, to estimate the economic feasibility of the proposed site and assess the costs of waste collection and management; and finally, to use the results of this study to recommend effective solid waste management practices and develop an inclusive management plan that includes sustainable and environmentally friendly methods for waste reduction, recycling, treatment, and disposal. Additionally, this study seeks to raise awareness within the community of the significance of waste management, the negative impact it can have on the environment, and methods for proper waste segregation and disposal. Finally, the study intends to establish a monitoring and evaluation system to ensure effective implementation of the waste management plan, measuring its impact on both the environment and community.
2. Study area and methods description
2.1. The study area’s description
2.1.1. Geographical location
The town of Shone is found in the Badawacho district, Hadiya zone, central Ethiopian regional state, Ethiopia, 345 kilometers south of the country’s capital, Addis Ababa. Moreover, Shone town is situated 97 kilometers south of Hosana, the regional capital, and the exact coordinates are 7°6’45.46“N to 7°11’45.63“N latitudes and 37°55’53.48“E to 37°58’28“E longitudes (see ). Shone, a thriving community with a rich cultural past and stunning vistas, offers a peaceful escape from city life, offering residents and visitors a serene retreat between lush forests and mountains. The town is also well-known for its traditional markets, where residents congregate to exchange and sell a variety of commodities while promoting the region’s wide range of agricultural products. Shone Town’s location on the route that connects Addis Ababa and Arba Minch makes it an important center for transportation in the area. Its central location also facilitates easy access from neighboring cities like Hosana and Hawasa, adding to its significance on the social and economic fronts in the region.
2.1.2. Topography
The relief of the town is categorized into plain and undulating surfaces and hilly terrain. As one travels from the center towards the south, the altitude slightly decreases. On the contrary, altitude increases as one moves away from the central part of the town towards the north and east directions, where Shone Mountain is located. The elevation of Shone town in this study was prepared from the Digital Elevation Model (DEM) of the town using the Spatial Analyst tool of the Surface extension. The elevation of the town ranges from 1910 meters above sea level in the southeastern part to about 2084 meters above sea level in the eastern part, where Shone Mountain is found, as shown in . The town is located on average 1450 meters above sea level. From a topographic perspective, the town appears to be the most suitable for habitation overall. The difficult process of finding an appropriate location for the landfilling of solid waste necessitates the careful analysis and manipulation of numerous geographical data points obtained from various sources.
2.1.3. Slope
Slope, the downward or upward slope of the ground, is a crucial factor in determining the most suitable landfill sites (). A more suitable option is a gentle slope, while a steep slope is considered more unsuitable. An area with a steeper slope contributes to erosion and increases construction costs. Therefore, when selecting a landfill site, it is important to consider the slope of the land to ensure its suitability for waste disposal. A gentle slope is preferred, as it reduces the risk of erosion and allows for easier construction of the landfill. On the other hand, a steep slope is unfavorable, as it increases the risk of erosion and may result in higher construction costs. Thus, slope is an important factor to consider in ensuring the long-term stability and effectiveness of a landfill site. Additionally, a steep slope can also lead to the potential for landslides, which pose significant risks to nearby communities and the environment. Furthermore, a gentle slope allows for better drainage and reduces the likelihood of water pooling at the landfill site, which can lead to contamination of the surrounding soil and groundwater.
2.1.4. Trends in temperature and rainfall in the study area
Temperature and precipitation are meteorological variables of great importance for the water supply and crop productivity of countries that heavily rely on rainfed agriculture (Abebe, Citation2013). The pattern of rain in Ethiopia presents considerable spatial and temporal variability. In previous times, the country suffered from unpredictable rainfall in some years and from complete failure of seasonal rainfall in others, associated with climate variability (Ministry of Foreign Affairs of the Netherlands, 2018). Ethiopian farmers heavily depend on rainfed agriculture, making this variability a significant challenge. Droughts, especially, can cause catastrophic effects on crop yield, food security, and economic growth. Furthermore, the increase in temperature due to climate change is expected to worsen water scarcity in the country, compounding the difficulties of crop cultivation. A recent investigation disclosed that by 2050, the agricultural productivity of Ethiopia may diminish by as much as 30% due to climate change (International Food Policy Research Institute, 2015). Ethiopia has implemented several adaptive measures to mitigate the impact of climate variability and change on agriculture, such as the construction of water storage facilities, the use of drought-tolerant crop varieties and irrigation techniques, and the promotion of agroforestry and soil conservation practices (Ministry of Foreign Affairs of the Netherlands, 2018).
Despite these efforts, there is still much to be accomplished to enhance the country’s resilience and adaptive capacity to climate change. This involves joint efforts and collaboration amongst government agencies, development partners, and local communities, targeting the establishment of climate-resilient agricultural systems and the improvement of farmers’ livelihoods.
The annual total rainfall from 2002 to 2022 showed high inter-annual variability and ranged from 1361.7 mm to 589.7 mm (). The study obtained seasonal rainfall variation, i.e. summer season, the main rainy season from June to August; spring, a smaller rainy season that falls from March to May; winter (Bega’) is the driest season (December to February); and autumn (today) rainfall plays a significant role in agricultural production. An analysis of the bar-graph trends of annual rainfall indicates variability trends in the study area. The amount of rainfall showed a yearly fluctuation between 2002 and 2022. This variability has also been indicated as a major problem for crop production and livestock rearing at the rural household level in the study area. The driest years were 2007, 2008, 2011, 2012, and 2021, which had the minimum rainfall of all years. Thus, the study area has suffered from a shortage of rainfall in these six years, while the remaining years demonstrated heavy and erratic rainfall. As displayed in the bar graph above, the trend of annual rainfall distribution in the study area was found to be slightly negative, as Y = −21.078× + 1065.8. However, the regression value of 0.368, which is below 0.5, has significant variability. The general annual mean rainfall distribution of the decades indicates a decreasing trend analysis of the rainfall distribution in the study area.
The annual distribution of temperature, trends, and variability are discussed. In terms of the distribution of the annual average temperature in the study area, the minimum, maximum, and mean annual temperatures were 12°C, 23.5°C, and 18°C, respectively. Long-term temperature data from 2002–2022 showed an increasing trend in temperature in the study area. The variability of mean, maximum, and minimum temperatures has been examined on an inter-annual time scale for the aforementioned period. The results showed an increasing trend for both maximum and minimum temperatures. The annual mean temperature in the study area was 18°C. The R-value for the trend linear equation is nearly 0.64, 0.52, and 0.013 for the maximum, mean, and minimum annual temperature, respectively (). It can be concluded that the maximum and mean temperatures indicated significant variability, as their respective R-values are greater than or equal to 0.5. This suggests a strong positive correlation between the maximum and mean temperatures over the study period. However, the minimum temperature showed a weak positive correlation, indicating less variability compared to the other two variables.
2.1.5. Population
According to CSA forecasts, there were 65,851 residents living in the Shone town administration in 2015, of whom 28,877 were males and 36,974 were females, based on the 2007 Census of Population. The population of Shone town administration has been steadily increasing since the 2007 Census of Population. It would be interesting to see how these numbers have changed in recent years. The majority of the town’s inhabitants are laborers, public servants, and business owners (CSA, Citation2013). The diverse occupations of the town’s inhabitants contribute to its economic growth and development. Additionally, understanding the current population trends can help inform future infrastructure and resource allocation decisions for the Shone town administration.
2.1.6. Drainage
There is the Gere Stream, but there are also irrigation dams such as the Artificial Lake in Lalo Gerbe Kebele and swampy areas like Damsite near Shone Town. According to the Southern Nation Nationalities People Regional State Water and Mining Construction Service Bureau (2015), there are two main sources of the water system for the community: surface water and groundwater. These systems are used for a variety of household purposes. Surface water is found near the town in the form of a reservoir, while groundwater is pumped from the aquifer to the earth’s surface for use. Thus, inhabitants may take water from either a private well or the community water system. Nevertheless, the Shone area faces several challenges with water supply. The current supply falls short of satisfying the surging demand, and during the dry spell, long commutes to fetch water are necessary for several households. Furthermore, contamination poses a major threat to the quality of the water. The absence of appropriate waste management systems intensifies this problem, with the cumulative effect being health risks for the community and an impediment to the growth and development of the town.
To tackle these issues, regional governing bodies and NGOs are collaborating to improve the water supply situation in the area. Projects focused on constructing water catchments and distribution systems and the establishment of water treatment plants have been implemented. The government has facilitated the drilling of boreholes to increase the groundwater supply and has assisted communities in installing water purification systems. While these steps have been taken, they have not sufficed, particularly in rural areas where the provisions are still lacking. The government needs to put an increased emphasis on the enhancement of water infrastructure. This would entail developing dams, reservoirs, and pipelines specifically aimed at rural communities. Furthermore, the government needs to work together with these communities to encourage conservation practices and promote the sustainable use of water resources.
In conclusion, access to clean and safe water is a fundamental requirement for the well-being and advancement of societies. In the Shone area, the government must give priority to the adequate supply of water to fulfill the surging demand and address quality concerns. Through continued investment in water infrastructure as well as community participation, the town can achieve a sustainable water supply and contribute towards the growth and progress of the Southern Nation Nationalities People Regional State.
2.2. Sources of data
The study utilized both primary and secondary data sources to gather the necessary information.
2.2.1. Primary data sources
The primary sources in this study comprise knowledgeable elders, women, and men who act as informants. The local authorities of both sexes, Kebele administrators, were also a significant source of information. During the field survey, GPS was used to collect points that identified community facilities such as schools and health centers, as well as protected areas like churches and mosques. Sample points were collected from different land use types, which helped to update the current land use type of the study area. To showcase the existing landfill site, photographs and field observations were utilized. Satellite images were also of significant importance in displaying the character of land use in this research. To gather further information, interviews were conducted with experts, such as environmental protection officers and residents living near the existing landfill. The results from these interviews helped identify the problems associated with the existing landfill in the study area. Other primary sources included data from both government and non-governmental organizations that have worked to address environmental issues related to landfill management in the study area. Reports, maps, and statistics associated with waste generation, transportation, and disposal practices were also collected to gain a comprehensive understanding of the current waste management practices in the area. Additionally, interviews were conducted with waste management experts and professionals to gather insights on best practices and potential solutions for the existing landfill issues. Furthermore, field observations were carried out to assess the physical conditions of the landfill site and its surrounding areas, providing valuable firsthand information on the environmental impact of current waste management practices.
2.2.2. Secondary data sources
Secondary sources of data were acquired through a review of literature, research reports, and published and unpublished papers, among others. Various maps were used in the process of choosing suitable landfill sites: the road network map of Shone town; the soil map of the study area; the land use and land cover map of the study area; the Digital Elevation Model (DEM) of Shone town with a resolution of 30 meters (which helped determine the drainage and slope); the geological map of the study area derived from the Sentinel 2A 2023 image at a resolution of 10 meters; and the study area boundary map (). These maps are crucial for further analysis when selecting suitable landfill sites. The road network map helps identify potential areas that are easily accessible by waste collection vehicles. The soil map provides information on the type of soil in the study area and helps determine the suitability of the soil for landfill construction. The land use and land cover maps help identify areas that are not suitable for landfill construction, such as residential areas, agriculture, and forest lands. The DEM map helps identify areas with steep slopes and poor drainage conditions that are not ideal for landfill construction. The geological map provides information on the geology and rock formations in the study area, which can help determine the stability of the landfill site. Finally, the boundary map helps identify the study area and determine the available area for landfill construction. Additionally, the soil analysis report provides detailed information on the composition and characteristics of the soil, helping to determine its suitability for landfill construction. Furthermore, environmental impact assessments are conducted to evaluate the potential effects of landfill construction on surrounding ecosystems and communities, ensuring that any negative impacts are minimized or mitigated.
Table 1. Sources and types of data.
Overall, the secondary sources of data and factor maps used in site selection provide vital information that is crucial in determining the most appropriate location for landfill construction. By taking into consideration these factors, the potential negative impacts on the environment and human health can be minimized. Waste management authorities can also utilize this information to make well-informed decisions about the construction and selection of landfill sites, which will ultimately result in more environmentally friendly waste management procedures.
2.3. Software used
ArcGIS 10.8 was utilized to prepare the evaluation factor data for a thematic suitability map, topographically related analysis, proximity analysis, and weighted overlay analysis. These analyses were crucial in determining the most suitable areas for the proposed project based on various factors such as land use, slope, distance to infrastructure, and other relevant criteria. The use of ArcGIS 10.8 allowed for efficient data processing and visualization, enabling informed decision-making during the project planning phase. The MCDA technique in the Excel sheet was employed to derive the weight for each factor and to assess the consistency ratio. Additionally, Mendeley Desktop software was applied for referencing purposes, Excel was leveraged for GPS point data preconditioning, a digital camera was used to support the qualitative analysis of observations, and Google Earth was utilized for output result validation purposes. Collectively, the GIS software played a pivotal role in data preparation and analysis. Excel and MCDA techniques facilitated determining the weight of each factor. The use of Mendeley Desktop software ensured the accuracy and reliability of the reference sources. The digital camera captured visual observations that supported the qualitative analysis of the data. Google Earth was instrumental in validating the output results. These tools and techniques, in tandem, produced a comprehensive and precise assessment of the thematic suitability map and land use analysis. The integration of these various tools and techniques allowed for a robust and comprehensive evaluation of the thematic suitability map and land use analysis. By utilizing GIS software, Excel, MCDA techniques, Mendeley Desktop software, a digital camera, and Google Earth, the assessment was able to incorporate both quantitative and qualitative data, resulting in accurate and reliable results. The GIS software provided a platform for spatial analysis and data visualization, allowing for the identification of patterns and relationships between different land use variables. Excel was used to organize and analyze the collected data, facilitating statistical analysis and generating graphs and charts for easy interpretation. Additionally, the MCDA techniques helped in prioritizing different land use options based on multiple criteria, ensuring a systematic and objective evaluation process. The integration of Mendeley Desktop software allowed for efficient management of research articles and references, ensuring credibility and validity.
2.4. Method for data analysis and presentation
There are different methods for GIS operation used in suitability analysis. In this study, methods such as buffering, overlay, digitizing, spatial analysis, and AHP were the main techniques employed to select suitable landfill sites. These methods were chosen based on their ability to analyze various factors, such as proximity to residential areas, topography, soil type, and accessibility. The combination of these techniques allowed for a comprehensive evaluation of potential landfill sites and ensured that the final selection was based on a holistic assessment of suitability.
2.4.1. Buffering
Buffer operation refers to the creation of a zone of a predetermined width around a point, line, or polygon area. It is also known as an area of a specified distance around coverage features. It is used to produce regions of a given distance around specific criteria that are used to identify appropriate solid waste disposal sites. In this study, the following features were buffered: roads, settlements, surface waterways, schools, medical facilities, churches, and mosques. Buffering these features can help identify areas that are suitable or unsuitable for solid waste disposal. For example, buffer zones can be used to ensure that disposal sites are not located too close to settlements or schools where people live or gather. Buffer zones around surface waterways can help protect water quality by preventing waste from being deposited too close to the water source. Buffer zones around medical facilities can help ensure that waste does not pose a health risk to patients and staff. Additionally, buffer zones around mosques and churches may be considered to respect cultural and religious requirements regarding waste management. Overall, buffer operation is a useful tool for identifying suitable locations for solid waste disposal and ensuring that waste is managed in a way that minimizes potential negative impacts on human health and the environment.
2.4.2. Reclassify
Using the GIS spatial analysis tool, the Euclidean distance function categorized the variables (slope, soil, geology, and borehole). Thereafter, the distances were reassessed and ranked according to their suitability for landfill placement, based on specific requirements like proximity to residential neighborhoods, water bodies, and delicate ecological components. The regions with higher suitability ratings were given priority for more detailed analysis and siting consideration. The GIS spatial analysis, thus, facilitated a thorough evaluation of various factors and their correlation, culminating in a more judicious and informed approach toward landfill siting. This approach allowed for a comprehensive understanding of the spatial relationship between the variables and their impact on landfill placement. Additionally, the use of GIS technology provided a visual representation of the data, aiding in the identification of potential conflicts or constraints that may arise during siting.
2.4.3. Pairwise comparison and factor weighting
The municipality expert’s rating of factors on a 1–9 continuous scale using AHP techniques was used as an input for subsequent weight derivation. The main objective is to plan mathematical tools to support the subjective evaluation of a finite number of decision alternatives under a finite number of criteria to find the best choice while avoiding bias (Osman et al., Citation2010). Fagbohun and Aladejana (Citation2016) further support this notion, saying that AHP is one of the GIS-based MCDMs that perfectly organizes and converts spatial data (input) into decisions (output). The pairwise comparison technique involves three steps:
Creation of a pairwise comparison matrix.
The weights are computed in the following phases: Summarize the values in each column. The total of each matrix element’s columns is used to divide it. The matrix’s values are all normalized in this manner. The average of each row’s elements in the normalized matrix is calculated by dividing the sum of the normalized values for each row by the total number of criteria. The averages give a good idea of how much each criterion is weighted relative to the others.
The consistency ratio (CR): This is done to ascertain whether or not the comparisons are reliable. According to Malczewski (2011), the actions listed below are taken to get a consistency ratio (CR):
To create the weighted sum vector, multiply the weight associated with the first criterion by the first column of the original pairwise comparison matrix, then multiply the second weight by the second column, the third criterion by the third column, and finally, sum these values across the rows.
Calculate the consistency vector by subtracting the weighted sum vector from the previously calculated criterion weights.
Calculate lambda (), the consistency vector’s average value, and the consistency index (CI), a measure of deviation from consistency, using the formula: CI= (max-n)/(n-1), where n is the number of criteria and is the consistency vector’s average value.
The consistency ratio (CR), which has the following definition: CR = CI/RI, Where, depending on the number of components being compared, RI is the random index. When CR is less than 0.10, the ratio shows that there is a decent amount of consistency in the pairwise comparison; when CR is greater than 0.10, the ratio values show inconsistent judgments. According to Saaty (Citation1980), the appropriate random index (RI) for a 9 by 9 matrix is 1.45 in the case of the current study. The consistency ratio obtained using a pair-wise matrix was 0.05 (), which was 0.1, and it was acceptable to adopt the experts’ appropriateness factors relative to rating judgment.
2.4.4. Analysis of weighted overlay
It is a requirement of the multicriteria technique that all criteria be expressed on the same scale. Therefore, a common assessment scale was set from 1 to 4 with increments of 1 before adding the reclassified input raster to the weighted overlay tool. A specific location’s suitability is assessed using this standard measuring scale, which allows for meaningful comparisons between criteria.
Higher pixel values, such as 4, denote more suitable sites, while lower pixel values, such as 1, denote less suitable locations. It is not possible to compare layers with distinct measurement units, as separate input factor maps have different measurement units. For instance, the degree of slope, the length of a road, and the type of land use and land cover were all measured. Therefore, all reclassified datasets were translated into the common unit of measurement, a scale of 1 to 4, in a weighted overlay to make all study locations comparable.
The identified suitability was represented with a numerical score value ranging from 0 (restricted) to 4 (highly suitable) in this study. Nine (9) evaluation factors for landfill site selection were used to achieve the stated objectives. Each reclassified dataset was added to the ArcGIS Weighted Overlay tool with its respective weights derived from the pairwise comparison matrix earlier.
2.4.5. Analysis’s conceptual framework
The overall process of this study involves four main steps: selecting the factors to be used to determine the best location for the landfill site and producing maps for each; allocating weight to the chosen factors; overlaying and creating a map of the landfill site’s suitability; and, finally, selecting appropriate areas for the landfill site. illustrates the conceptual framework in detail. The conceptual framework in provides a visual representation of how the four main steps of the study are interconnected. It helps researchers understand the flow of the process and highlights the importance of each step in determining the best location for the landfill site. Additionally, the framework serves as a guide for future studies or decision-making processes related to landfill site selection.
3. Data analysis, results, and discussions
The study employed the GIS capability and the AHP method’s weighting product to determine suitable sites for Shone Town’s solid waste disposal. Solid waste disposal sites must adhere to fundamental requirements to mitigate surface or groundwater pollution and safeguard other resources. The GIS capability allowed for the analysis of various factors, such as topography, land use, and proximity to water bodies, which are crucial in identifying suitable sites. The AHP method’s weighting product further prioritized these factors based on their importance, ensuring a comprehensive evaluation of potential sites for solid waste disposal in Shone Town.
Moreover, to minimize public health repercussions, solid waste disposal sites must be located far from population centers. Simultaneously, waste disposal sites should not be too distant from existing roads to save on costs associated with transportation, collection, and road development. Additionally, topographically challenging or steep terrains are unfit for holding solid waste disposal. This study incorporated nine suitability criteria (distance from roads, protected areas, settlement, road network, groundwater, surface water, soil type, geology, urban land use type, and slope). Individual maps were produced for each criterion, and the final composite map was constructed by overlaying these maps. Tables were utilized to specify the layers, buffer zones used, rankings, and layer weights. To increase the appropriateness of the sites, the weights were assessed while considering the possibility of modifying the natural conditions.
3.1. Solid waste management in Shone town
The primary objective of this study was to evaluate the current waste management practices in Shone Town. This was accomplished through onsite observations and interviews with professionals in the environmental protection department of the town. It was discovered that the municipal authorities and the land administration office selected and prepared the present open dumping site based solely on its distance from the town center. The current solid waste disposal system was deemed unsatisfactory as the existing site does not consider important environmental and health factors such as proximity to water bodies, agricultural fields, and settlements. The inhabitants near the present open dump site are afflicted with air pollution, and their use of the nearby stream for animal feeding and irrigation is prohibited due to contamination. Waste collectors employ donkey carts and handcarts to collect waste from hotels, houses, and other institutions and transport it to the dumping site. In many Ethiopian towns, there are temporary waste sites at various distances where solid wastes are stored post-collection from different sources. This poses a significant hazard to human health, particularly since many of these sites are located near residential areas. However, in the case of Shone Town, solid waste is dumped immediately after collection at the current site.
Currently, solid waste management is a topic of considerable attention in many Ethiopian towns, particularly in the study area, where solid wastes originating from abattoirs are a major concern. Proper handling and management of solid wastes that are generated in most Ethiopian towns remain an issue. Nevertheless, these issues can be addressed through the planning and implementation of different municipal solid waste management components. While surface water is the core difficulty in Shone Town, the negative effects of solid waste disposal can be reduced through the use of a geographic information system and remote sensing techniques to select suitable sites that minimize environmental impact.
Overall, the present waste management practices in Shone Town are unsatisfactory, and immediate measures are required to address the environmental and health problems. The choice and preparation of the dumping site must be reconsidered based on its distance from water bodies, agricultural fields, and settlements. Appropriate transport and disposal methods must be implemented, and suitable sites must be identified using GIS and RS techniques that minimize potential environmental impacts. Proper planning and implementation can lessen the negative effects of solid waste disposal and prioritize the health and well-being of inhabitants.
3.2. Open solid waste dumping in the study area
In the town, the majority of the solid waste that is collected from hotels, residences, institutions, asphalt roads, the abattoir, as well as the business district, is dumped in an open field that is quite close to the mainstream, crop field, and major road. Due to runoff and wind, solid waste dumped in an open field close to a stream significantly contaminates the waterway as the slope towards the stream is steep (see , and ). This contamination poses a serious threat to the health and safety of the community and the environment. The decomposing waste emits foul odors and attracts vermin like rats and flies, which contribute to the spread of disease. Additionally, the waste can leach harmful chemicals and bacteria into the groundwater, affecting not only the stream but also the crops and drinking water sources in the area.
Dumping solid waste in an open field is a violation of environmental laws and regulations. It is the responsibility of the local government to ensure that proper waste management practices are in place to protect both the community and the environment. Adopting more sustainable waste management practices, such as source reduction, recycling, and composting, can greatly reduce the amount of waste that needs to be dumped in landfills or open fields.
It is essential to take appropriate and urgent action to address this issue. The local government should enforce strict regulations on proper waste disposal and encourage waste reduction and recycling to prevent the accumulation of waste. Additionally, they should work to clean up and remediate the contaminated area and invest in proper waste management infrastructure to prevent future instances of illegal dumping. It is crucial to prioritize the health and well-being of the community and the environment by implementing sustainable waste management practices.
The repercussions of insufficient management of solid waste can be grave and can lead to environmental deterioration, health risks, and financial setbacks. The indiscriminate disposal of refuse can contaminate nearby water bodies and soil, engender air pollution due to the incineration of waste, and pose a peril to the well-being of humans and animals by facilitating the transmission of diseases. Furthermore, the accumulation of refuse can attract pests and vermin, thereby exacerbating health hazards.
Additionally, inadequate solid waste management can exert a detrimental influence on economic activities, as it can deter tourists from visiting the region and diminish the productivity of agricultural land. In the long term, addressing the issue of waste management belatedly might incur a higher cost for remediation than if a proper waste management system were to be implemented from the outset.
Hence, the municipality of Shone Town ought to undertake remedial measures to enhance the infrastructure for solid waste management. This could involve investing in a designated landfill site, implementing a structured waste collection system, and involving stakeholders in initiatives aimed at waste reduction and recycling. By adopting these measures, the municipality can alleviate environmental degradation, safeguard the well-being of humans and animals, and enhance its economic prospects.
3.3. Landfill site selection criteria
The initial step in the landfill siting procedure is to determine the site’s needs or criteria. Since choosing an appropriate landfill site is the primary goal of this study, the selection criteria should have minimal negative effects on the local ecology, economy, and society. To achieve this, a thorough assessment of the potential environmental impacts, such as soil and water contamination, air pollution, and habitat disruption, should be conducted. Additionally, economic and social factors, including proximity to residential areas, transportation infrastructure, and public opinion, must also be taken into consideration. By carefully evaluating these criteria, a suitable landfill site can be identified that minimizes adverse effects on the surrounding environment and community.
3.3.1. Suitability of the slope class
Slope is a crucial criterion that must be considered when selecting a solid waste disposal site. Using the Arc-GIS spatial analyst extension of the surface tool, the slope of the research region was generated from the DEM. The slope affects the amount of water in the soil, the risk of erosion, surface runoff, and groundwater pollution. In addition, the slope is critical for the construction of landfill sites, as a higher slope causes higher construction costs due to the vast number of materials, technology, and labor required. Four types of slope percentages were added to the slope raster, with 4 being the most suitable and 1 being the least suitable for site selection. The slope raster analysis provided valuable information for the site selection of the waste disposal site. It allowed for the identification of areas with suitable slope percentages, which are crucial for minimizing water-related issues and construction costs. This data can greatly assist in making informed decisions regarding the most appropriate location for the landfill site. Additionally, the slope raster analysis helps in assessing the potential risks of erosion and landslide occurrences in different areas, ensuring the long-term stability and safety of the waste disposal site. Moreover, by considering slope percentages, the analysis aids in optimizing construction efforts and reducing expenses associated with earthwork and grading activities.
In this study, land with a lower slope was considered more appropriate than land with a higher slope. According to many studies, sites with steep slopes have a higher risk of pollution and may not be the best places to dump waste (Ravinder J., Citation2014). The location should be regarded as being least suitable as the slope becomes steeper. The type of slope for a solid waste dumping site should be gentle, less than 10%, according to Ebistu and Minale (Citation2013). The majority of the research area, or 68.85% of it, falls within the slope class of 2–6%, making it ideal for the disposal of solid waste (see and ). As a result, most of the property can be used as a site for the dumping of solid waste. Slope classes 6–10, 10–13, and > 13%, on the other hand, cover 25.70%, 3.80%, and 1.75%, respectively, of the research area. This demonstrates that choosing a solid waste dumping site in Shone Town does not present a substantial slope-related challenge. Thus, the town’s topography can be considered to be quite smooth. As to the findings of Ebistu and Minale (Citation2013) study, 68.85% of Shone Town’s research area has a slope class of 2–4 percent. This implies that a sizable chunk of the town’s land is appropriate for the dumping of solid waste. Furthermore, only a small portion of the region – more precisely, 25.70%, 3.80%, and 1.75% for slope classes 6–10—is affected.
Table 2. Slope suitability class.
The slope suitability map was generated based on the slope classes identified in the study area. These slope classes were determined through a combination of field surveys and remote sensing data analysis. The map provides valuable information for land use planning and natural resource management in the study area. The green areas on the map indicate the most suitable locations for solid waste dumping, while the yellow areas are less suitable. The red areas, which correspond to slope classes greater than 13%, are not recommended for waste disposal. Overall, the map shows that the majority of the study area is suitable for solid waste dumping, except for a few steep slopes. Waste management authorities can use this information to determine which locations in Shone Town are suitable for disposing of waste. By selecting areas with gentle slopes and avoiding steep slopes, the risk of pollution and environmental degradation can be minimized. It is important to note that other factors, such as proximity to residential areas and groundwater resources, must also be taken into account when selecting a waste disposal site. Nevertheless, the slope suitability map provides a valuable starting point for waste management planning in Shone Town. The slope suitability map can serve as a useful tool for waste management authorities to prioritize and allocate resources for waste disposal in Shone Town. Additionally, it can help in identifying potential areas where erosion and soil instability may occur, allowing for proactive measures to be taken to prevent any negative environmental impacts.
3.3.2. Accessibility
Road networks and accessibility are significant parameters given due consideration in locating a suitable site for a landfill. The road network offers a linkage between settlements, factories, and industrial areas that are all sources of waste production to the remotely located waste disposal site. Road networks have a major impact on the cost of transportation as well as the efficacy and effectiveness of the waste management system (Valentina, Citation2011). According to Valentina, a suitable landfill should be situated at a minimum distance of 60 meters from the main roads, where areas located within a buffer distance of 60 meters are considered unsuitable and labeled as constraints for landfill site selection. Areas beyond 60 meters are generally deemed appropriate for a landfill. On the contrary, Nas et al. (Citation2010) stated that a landfill situated within a distance of 200 meters is unsuitable. ‘Landfills shall not be sited within 200 meters of any major highways or town streets. On the other hand, the landfill site should not be located too far away from existing road networks to avoid the expensive cost of constructing connecting roads’ (Nas et al., Citation2010).
According to Berisa and Birhanu (Citation2015), local research output argues that landfill sites must not be located within a distance of 100 meters due to environmental concerns and a bad smell affecting the surroundings. This buffer distance is known in this thesis work based on similar environmental and topographic similarities. An interval of 400 meters from the buffer zone is elected as less suitable for a disposal site in the town of Shone. Landfill sites should not be sited too far away from roads because that will increase costs significantly. They also should not be near roads that might cause odor and environmental pollution. To prevent public health concerns and lessen the cost of transportation, the EPA (Citation2007) used 500 meters, and Allen et al. (Citation2010) used 60–600 meters. Therefore, in this study, a 200-meter buffer distance is considered a limited distance. However, based on the outcome of waste transportation and public health, the area was classified into four buffer zone classes: 0–200 meters, 200–400 meters, 400–600 meters, and 600–800 meters. The road and the area within 200 meters are considered unsuitable (restricted), and the remaining area is classified according to its suitability. Generally, for areas < 200 meters and > 800 meters, suitability decreases with very close proximity and increased distance, which may result in pollution, increased transportation costs, and inaccessibility of the area, respectively.
In this study, existing roads were established from the structural plan of the town accessed from Shone town municipality in a vector format. To locate landfills that are suitable for the existing roads, buffer zones were classified into four levels and standardized as very close and very far places from roads that are not suitable for landfills because of the pollution impact and additional transport and construction costs, respectively. The distance classification of roads is demonstrated in and . The buffer zones were classified based on their proximity to the existing roads, with ‘very close’ referring to areas that are within a certain distance from the roads and ‘very far’ indicating areas that are located farther away. This classification was necessary to identify suitable locations for landfills that minimize pollution impacts and avoid additional transportation and construction expenses. The distance classification of roads can be found in and , providing a visual representation of the different levels of proximity.
Table 3. Road suitability class.
3.3.3. Surface water
Most of the surface waters in the study area are rivers and lakes. The solid waste dumping place must not be close to surface water bodies like streams, rivers, and lakes. This is because as the distance between the dumping place and water bodies narrows, the probability of contaminating the water increases. Pollution in water resources causes severe problems in the environment, public health, and economy. In this study, the streams and rivers were prepared from the DEM of Shone Town using the spatial analyst hydrology extension. Furthermore, the farther the distance from rivers and streams, the more preferable the area for landfills (Gizachew, Citation2010). The waste disposal areas should not be in the vicinity of rivers, lakes, or swamps. This criterion has a direct relationship with the suitability of land for being used as a landfill. The farther the landfill site is from streams and river banks, the more suitable it is. In some literature reviews, the researchers have suggested a distance of up to 500 m away from freshwater bodies (Elahi & Samadyar, Citation2014). Consequently, the Multiple Ring Buffer from Analysis Tools was used to prepare multiple buffers for distances: 0–1500, 1500–3000, 3000–4500, and > 4500, and ranks were assigned for each buffer, 1, 2, 3, and 4. To lessen the effect of landfill leachate on surface water pollution, the 0–1500 m buffer areas were excluded from the sitting process. The rest of the areas were analyzed based on the distance from the streams and rivers. A summary of the suitability level for their respective areas is shown in . and . The suitability level for the remaining buffer areas was determined based on their distance from streams and rivers. This information is summarized in and illustrated in . The analysis aimed to minimize the impact of landfill leachate on surface water pollution by excluding areas within a 0–1500 m buffer from the sitting process.
Table 4. Stream suitability class.
3.3.4. Soil types of the study area
The soil characteristics of the study area, such as texture and depth, should also be considered for landfill site selection within the study area. This is due to the availability of lining material and permeability, depending on the depth and texture of the soil, respectively. Clay-textured soil is preferred for the landfill as it is impermeable to leachate and is also used for lining the base of a sanitary landfill. Moreover, areas with deep soil are preferred, as they provide the soil for covering solid waste after each disposal to minimize air pollution from the landfill. The capacity to hold water and the rate of water infiltration fluctuate depending on the kind of soil. The permeability and porosity of the soil control both the vertical and horizontal flow of pollutants (Mekuria, Citation2006). It is recommended that the soil at the selected site have a good nature of impermeability to reduce the possibility of aquifer contamination. The three dominant soil types in the study area were identified as highly suitable, suitable, and less suitable based on their dominant texture suitability for landfills. Eutric Nitisols were identified as highly suitable, Pellic Nitisols were identified as suitable, and Dystric Nitisols were identified as less suitable (FAO, Citation2014). These soil types were assessed based on their permeability and porosity, which are key factors in controlling the flow of pollutants. The identification of highly suitable soil types indicates that they have a good nature of impermeability, reducing the risk of aquifer contamination.
In the study area, three dominant soil types have been identified: eutric nitisols, pellic nitisols, and dystric nitisols.
3.3.4.1. Eutric Nitisols
These soil types are highly suitable for landfill sites due to their impermeability and ability to hold water. They have a high clay content, which makes them less susceptible to leachate infiltration and minimizes the risk of aquifer contamination. Eutric Nitisols are commonly used for lining the bases of sanitary landfills.
3.3.4.2. Pellic Nitisols
These soil types are suitable for landfill sites as they also have a good nature of impermeability. They provide a suitable balance between clay and silt content, which helps reduce the vertical and horizontal flow of pollutants. Although not as impermeable as eutric nitisols, pellic nitisols still offer a level of protection against leachate movement.
3.3.4.3. Dystric Nitisols
These soil types are identified as less suitable for landfill sites due to their lower impermeability compared to the other two dominant soil types. They have a higher sand content, which makes them more prone to leachate infiltration and potential aquifer contamination.
However, with proper management practices and additional lining materials, Dystric Nitisols can still be considered for landfill sites. In summary, the soil types in the study area vary in their suitability for landfill sites. Eutric nitisols are highly suitable; pellic nitisols are suitable; and dystric nitisols are less suitable. The impermeability of the soil is a key characteristic to consider, as it determines the potential for leachate movement and aquifer contamination.
shows that it’s evident that dystric nitisols, which make up 62.18% of the research area and are mostly dispersed in the north-central region (see ), cover the majority of the area. Pellic Vertisols and Eutric Nitisols hold the second and third places in area share, comprising about 24.37% and 13.45% of the study region, respectively, and are distributed from the southeast to the southwest. Meanwhile, only 13.45% of the study area is underlain by eutric nitisols, distributed from the southwest to the north.
Table 5. Soil suitability class.
The suitability of soil types for landfill siting was assessed based on their permeability and porosity to the movement of pollutants, as well as their susceptibility to erosion. The soil should be sufficiently low in permeability to ensure the very slow movement of leachate from the landfill site towards groundwater. For the present study, after reviewing the above-discussed literature, eutric nitisols were reclassified as highly suitable for landfill siting, and pellic vertisols were reclassified as the second-best option, following eutric nitisols. On the other hand, dystric nitisols were reclassified as less suitable due to their high rate of permeability. Additionally, the soil’s porosity is an important factor to consider, as it affects the ability of pollutants to infiltrate and contaminate groundwater. Therefore, soils with low porosity are preferred for landfill siting to minimize the risk of pollution. It is also worth noting that the susceptibility to erosion should be taken into account when selecting a suitable soil type for landfill siting, as highly erodible soils may lead to the exposure and spread of pollutants.
3.3.5. Protected areas
The protected area in this study comprises Shone Town’s churches, mosques, schools, and health centers. To avoid potential interventions and risks to human health, a dumping area should be located at a distance greater than 300 meters from built-up areas in general and social services in particular (Berisa & Birhanu, Citation2016). Therefore, a 300-meter buffer distance from a landfill is considered suitable to identify areas not suitable for landfill sites. Accordingly, four different zones were specified: areas far from protected areas are more appropriate, while areas near protected areas are less suitable for landfill sitting. The study considered the reclassified distance as unsuitable from 0 to 600 meters, less suitable from 600 meters to 1200 meters, suitable from 1200 meters to 1800 meters, and highly suitable above 1800 meters. A summary of suitability levels with their corresponding areas is shown in and . The study found that areas within a 300-meter buffer distance from a landfill are considered unsuitable for landfill sites. In contrast, areas that are further away from protected areas are deemed more appropriate for landfill siting. The reclassification of distances helped categorize areas as unsuitable, less suitable, suitable, or highly suitable based on their proximity to the landfill. and provide a summary of these suitability levels and their corresponding areas.
Table 6. Protected area suitability class.
3.3.6. Settlement
The waste disposal sites should not be located in or near populated areas. The range of residential areas was derived from reclassification, and distances of 1000 m and above are considered suitable, while those below 500 m were deemed unsuitable. Above 1350 m, areas are highly suitable. Thus, the suitability of landfills increases with the increasing distance from residential areas. Solid waste disposal sites should not be situated near commercial areas, governmental or private institutions, schools, health clinics, religious institutions, educational institutions, residential areas, and other social services areas since they might cause different types of pollution. Khalek et al. (Citation2011) set a built-up distance of 500 m as the minimum buffer distance (Hasan et al., Citation2009). To control the effects of public health and the aesthetic value of the land, the study area was categorized into four buffer zones: 0-550 m, 550-900 m, 900-1350 m, and >1350 m. The built-up area below 550 m is considered unsuitable (restricted), and the remaining area is categorized and ranked according to its suitability with the help of a literature review. The distance classification of the built-up area is displayed in and . The study found that the closer the built-up area was to the pollution sources, the higher the risk of negative impacts on public health and the aesthetic value of the land. Therefore, it was crucial to establish buffer zones and restrict any further development within 550 meters of these sources. The categorization and ranking of the remaining areas helped in identifying areas that were more suitable for development while minimizing potential pollution effects.
Table 7. Settlement suitability class.
3.3.7. Hydro-geologic characteristics
In the water sector of Shone town, seven boreholes were drilled, and GPS point data was collected for each. Based on this data, a borehole layer was created in the ArcGIS environment. To prevent groundwater contamination by leachate from landfill sites, a buffer distance was specified for the borehole layer. Groundwater circulation and pollutant flow through rocks and soils depend on hydrogeological conditions, specifically hydraulic properties such as porosity and permeability. Thus, the boreholes were divided into groups based on permeability levels, ranging from high to very low. As previously mentioned, less permeable materials are suitable for landfill areas because the leachate from solid waste cannot spread out as easily. Therefore, the red-shaded area is highly suitable for landfill sites, and areas shaded in light green, blue, and dark green are moderately suitable, less suitable, and unsuitable, respectively ( and ).
Table 8. Borehole suitability class.
Wells and reservoir locations are sensitive sites that must be considered when determining waste disposal systems. To ensure the protection of wells from runoff and leaching from landfills, proximity to wells is a vital criterion for accessing landfill sites. Accordingly, a 300-m buffer was specified around all wells using GIS software. This buffer function was used to generate the buffer. As stated in the literature by Taye (Citation2018), this buffer distance is recommended to protect wells from landfill-related contamination. Similarly, Shamshiry et al. (Citation2011) suggested locating dumping sites at least 300 meters away from wells and reservoirs. Thus, this study accepts a minimum distance of 300 m from wells and reservoirs for landfill sites. This minimum distance of 300 m is crucial in preventing the potential contamination of wells and reservoirs by leachate from landfills. It ensures that the water sources remain safe and free from any landfill-related pollutants, safeguarding public health and the environment.
3.3.8. Suitability analysis of geological factors
The geological formation of the site determines the degree of weathering and fracture of the underlying rocks. Hence, a geological factor map analysis was employed for landfill site selection. Impermeable strata and consolidated material are suitable for landfill sites as they do not allow the movement of leachate and hence minimize the risk of groundwater contamination from landfill leachate (Simsek & Gunduz, Citation2006). According to Ersoy and Bulut (Citation2009), unconsolidated lithology should not be used as a site for landfills. Four geological classes were determined for the research area based on geological data: hydrogenous sediment (93.28%), lacustrine sediment (4.40%), igneous rock (1.59), and pegmatite (0.72). According to Peter (Citation2015), areas with poor geological conditions were given lower scores, which is an indication of their poor suitability for landfill siting, while those that are best were given higher scores. This scoring system allows for a comprehensive evaluation of the geological conditions in the research area and helps to identify areas that are most suitable for landfill siting. By considering factors such as lithology and sediment type, the risk of groundwater contamination can be minimized, ensuring the protection of local water resources.
Geological surveys, reports, academic research papers, local geological agencies, and expert opinions were used to analyze the suitability of geological formations for landfill siting. These sources provide detailed information on rock formations, lithology, and sedimentary deposits, as well as insights into groundwater contamination. Local government agencies and geologists provide guidelines for landfill siting, identifying sub-criteria for selecting the best geological formations for landfill construction. For the present study, through a review of the above-discussed literature, Igneous was reclassified as highly suitable for landfill siting. Lacustrine sediment was reclassified as the second most moderately suitable, following igneous. Meanwhile, pegmatite and hydrogenous sediment were reclassified as less and unsuitable, respectively, due to their high rate of permeability. Generally, the suitability of a geologic type for landfill siting decreases as its permeability increases, and vice versa. A summary of suitability levels with their respective areas is shown in , and provides a comprehensive summary of the suitability levels for landfill siting based on the reclassification of various geologic types. The current study shows that igneous rocks are very appropriate and that lacustrine sediment is only somewhat appropriate. However, pegmatite and hydrogenous sediment are considered less and unsuitable, respectively, due to their high permeability rates. This finding suggests that the suitability of a geologic type for landfill siting is inversely proportional to its permeance.
Table 9. Geological suitability class.
3.3.9. Urban land use
The land cover of the urban area was analyzed using Sentinel-2A data acquired in 2023. The image was georeferenced to the Adindan datum, the UTM Z 37N Grid, and the Universal Transverse Mercator projection. Classified pixels were grouped into six general categories: water bodies, settlements, vegetation, forests, agriculture, and barren land. Accordingly, the land use types were ranked based on their importance to evaluate suitable sites for finding landfill sites. These are: barren land is classified as highly suitable; forests and urban agriculture as moderately suitable; vegetation areas as less suitable; and areas near streams and urban settlements as unsuitable. The classification of land use types based on their suitability for landfill sites is crucial for effective site selection. By ranking the land use types according to their importance, decision-makers can prioritize areas that are highly suitable, moderately suitable, less suitable, and unsuitable. This approach ensures that potential landfill sites are evaluated thoroughly and in accordance with their compatibility with the surrounding environment and human settlements.
An analysis of land use and land cover data was conducted to determine the best location for a landfill based on its direct and indirect effects on the environment, society, and economic value of the property. The natural and anthropological landscapes that are at risk from landfill proximity are those with varying land uses and covers. It is ideal to use bare land for the disposal of solid waste (Ebistu & Minale, Citation2013). Based on this, from the available land use and land cover classes ( and ), bare lands with an area coverage of 7.51% were reclassified as very highly suitable for solid waste landfilling because the economic value of the land is low. This reclassification of bare lands as very highly suitable for solid waste landfilling takes into consideration the low economic value of the land. Additionally, by using bare land for landfill purposes, the potential risks to natural and anthropological landscapes can be minimized,
Table 10. Urban land use suitability class.
lists the land use and land cover classes that were restricted by reviewing the LU/LC suitability for landfill siting, to the extent of the study. These classes include the settlement area and the water body. These land uses and land covers were restricted because of their social values; fixing solid waste landfill sites in these cited LU and LC areas is unsuitable. The settlement area is restricted due to its high population density and potential for adverse health impacts. Water bodies are also restricted to protect aquatic ecosystems and prevent contamination. The exclusion of these land use and land cover classes ensures that landfill siting is done in areas that minimize negative impacts on both human communities and the environment.
3.3.10. Evaluation of accuracy
A confusion or error matrix was used in the accuracy assessment technique. The confusion matrix outlines the existing and anticipated classifications of a classification system. The classified image pixel was compared with the corresponding location in the field. The results of an accuracy assessment provide users with information on the overall correctness of the map and the accuracy of each class on the map. The following formula was used to determine the percentage of total accuracy:
A similar formula was utilized to determine the classification accuracy of each class as well as the overall accuracy. Two techniques are used: user accuracy and producer accuracy. The producer’s accuracy is calculated by dividing the number of accurate pixels in one class by the overall number of pixels taken from reference data (). This determines how accurately a particular place has been classified in this study. It also includes the error of omission, which is the percentage of observed ground features that are not labeled on the map. The user’s accuracy is calculated by dividing the proportion of correctly classified pixels in each category by the total number of pixels that were classified in that category.
Table 11. Error matrix showing classification accuracy of the true land cover.
Accuracy in general is calculated by dividing the total number of correctly identified pixels (i.e. the sum of the major diagonal’s members) by the total number of reference pixels. The result of the total error matrix is displayed in , in which the study achieved a total accuracy of 85% in 2023. According to Anderson et al. (Citation1976), the minimum overall accuracy value obtained from an error matrix for a viable land cover categorization is 85%. Consequently, based on Anderson’s criteria, the overall accuracy of the research’s land use/cover map was above the minimum of 85%. The 2023 supervised classification was completed with a Kappa coefficient (Khat) of 0.85 and an overall accuracy of 85%, denoting a high degree of agreement. Sometimes the Kappa coefficient is multiplied by 100 to represent the classification accuracy as a percentage. In this case, the Kappa coefficient of 0.85 indicates a strong level of agreement between the classified land use/cover map and the reference data. This suggests that the classification results are reliable and can be used with confidence for further analysis or decision-making purposes.
Table 12. Confusion matrix accuracy technique between the land cover dataset and ground truth data.
3.4. Calculating factor weights and overlaying identified suitable sites
Assigning weights to the criteria for each factor map is one of the steps in the GIS-based Multi-Criteria Evaluation (MCE) technique. Weighting serves to convey each factor’s preference or priority with other factors that have an impact on landfill sitting when choosing a landfill location. The literature on multi-criteria decision-making has suggested several criterion-weighting techniques depending on the decision-makers assessments. The techniques comprise ranking, rating, pair-wise comparison, and trade-off analysis. They vary from one another in terms of accuracy, degree of usability and comprehension on the part of decision-makers, and theoretical underpinning (Hasan et al., Citation2009). As a result, one of the most promising pair-wise comparison methods is the Analytical Hierarchical Process (AHP), which was developed in the context of a decision-making process. The weights must add up to 100% in MCE when employing a weighted linear combination. The primary eigenvector of a square reciprocal matrix of pair-wise comparisons between the criteria in AHP can be used to determine weight. Comparisons are made about the relative weight given to each of the two factors considered separately when determining fit for the stated goal. To create a pair-wise comparison matrix, which the module uses to produce a set of weights and consistency ratios, all feasible combinations of two factors were compared using expert judgment. This ratio is crucial because it highlights any discrepancies that might have emerged throughout the pairwise comparison procedure (Saaty Katz, Citation2008). The scale shows the intensity of the importance of two factors at a time: 1=equal importance, 2=equal to moderately important, 3 = moderately important, 4 = moderately to strongly important, 5 = strongly important, 6=strongly to very strongly important, 7=very strongly important, 8=very to extremely important, and 9=extremely important.
Analyzing the consistency ratio can be used to assess how consistent the comparisons are. Multiple steps are used in the procedure. The weight for the first criterion times the first column of the original pairwise comparison matrix will yield the weighted sum vector. The second weight times the second column, the third criterion times the third column of the original matrix, and finally the sum of these values over the rows will yield the weighted sum vector. Next, calculate the consistency vector by dividing the weighted sum vector by the previously established criterion weights. Third, calculate the consistency vector’s average value, lambda (), and the consistency index (CI), which offers a measure of deviation from consistency and has the formula below: The consistency ratio (CR), which is calculated last, is calculated as follows: CR = CI/RI, where the number of elements being compared determines the random index (RI). The random index (RI) is determined based on the number of elements being compared. The consistency ratio (CR) is then calculated by adding the consistency index (CI) and the random index (RI).
As a result, if the consistency ratio is less than or equal to 0.1, the reciprocal matrix is considered acceptable (Dano, Citation2011; Saaty, Citation1980). According to the study’s consistency ratio of 0.05, there was a respectable amount of consistency in the paired comparison. As a result, it is acknowledged as realistic, and the weights assigned to each criterion were fair. The standardization of each data set to a common scale of 1, 2, 3, and 4 (value 1 = unsuitable, value 2 = less suitable, value 3 = suitable, and value 4 = highly suitable) was carried out to merge all the layers to process overlay analysis. Overlay analysis is a spatial analysis technique that combines multiple layers of data to identify areas that meet specific criteria. By standardizing each data set to a common scale, the overlay analysis can effectively merge all the layers and provide valuable insights for decision-making. This process allows for a comprehensive evaluation of suitability and helps in identifying areas that are highly suitable for the desired outcome (, and ). Overlay analysis is commonly used in various fields, such as urban planning, environmental management, and natural resource assessment. It helps in understanding the spatial relationships between different factors and can assist in making informed decisions regarding land use, conservation efforts, and infrastructure development. Additionally, the results of overlay analysis can be visually represented through maps or spatial models, enabling stakeholders to easily interpret and communicate the findings.
Table 13. Provides a random index for the number of elements and criteria.
Table 14. Pair-wise comparison on seven-point continuous scales.
Table 15. Weight of consideration for choosing a suitable solid waste disposal site.
Four classes are present in the final map (). About 1.55% (51.32 ha) of the town’s total area is classified as a highly suitable region for a landfill site. The appropriate area (3) covers 54.86 hectares, or 1.66% of the total area. The region that makes up 1.06% (34.99 ha) falls into the less suitable category, while the remaining 95.74% (3171.22 ha) falls into the unsuitability category and has a value of 1 (). By using the aforementioned criteria, suggested places that are acceptable for solid waste disposal sites are found to be in the south and southwest directions of the town. These suggested places in the south and southwest directions of the town have a combined area of approximately 2.61% (86.18 ha) and are considered suitable for solid waste disposal sites according to the criteria mentioned above. Additionally, it is important to note that further analysis and assessment should be conducted to ensure the environmental impact and feasibility of establishing landfill sites in these areas.
Table 16. Landfill suitability.
Choosing and evaluating landfill locations is important because the size of the area chosen for the landfill defines how long it will be used as a waste disposal site. Landfill size is one of the determining factors for sustainable solid waste management. Larger parcels of land that will be used for at least 10 years are better from a sustainability and economic standpoint than smaller ones (Sulaiman et al., Citation2012). This is because choosing a large-scale landfill can reduce the cost of site selection, design, and closure procedures that must be carried out when the landfill reaches the end of its useful life. As a result, the analysis’s findings indicate that four landfill candidate sites meet these area requirements and have been chosen for further assessment. A landfill site with an area of 51.32 ha falls under the category of highly suitable and is located in the northwestern and southeastern parts of the town. Other landfill sites with an area of 54.86 ha are classified as suitable, 34.99 ha are classified as less suitable, and 3171.22 ha are classified as unsuitable. Concerning settlements, protected areas, rivers, and road networks, depicts the two categories of landfill appropriateness. The south and south-western outskirts of the town are where you’ll find locations that are ideal for landfills, as this image demonstrates. Based on the identified parameter analysis, the allocated area is an ideal location for solid waste disposal. Because the location does not belong to private farmers in the area, it is flat, which makes excavation easy, has little vegetation coverage, no nearby surface water, and a good access road, and the distance from the town is also comparable. Additionally, the allocated area is located away from any protected areas, rivers, and road networks, ensuring minimal environmental impact. Moreover, the south and south-western outskirts of the town provide a suitable distance from residential areas, reducing potential health hazards for nearby residents.
4. Conclusions and recommendations
4.1. Conclusions
Solid waste is a significant source of pollution in urban areas and can contribute to the spread of diseases in developing countries. Inadequate waste management systems and improper disposal practices can exacerbate the problem, leading to contaminated water sources and the proliferation of disease-carrying vectors such as mosquitoes. Moreover, the accumulation of solid waste in urban areas can also have detrimental effects on air quality, further compromising the health and well-being of residents. This study identified paper and cartons, plastics/bags/bottles, food waste, and tins/cans as the primary types of solid waste that pollute the environment. These types of solid waste not only contribute to pollution but also pose a threat to wildlife and marine ecosystems. Additionally, the improper management of solid waste can result in the release of harmful greenhouse gases, contributing to climate change and its associated impacts on the environment.
The selection of a solid waste disposal site is critical to any waste management system and needs to be properly planned. A well-planned solid waste disposal site should be located away from residential areas and sensitive ecosystems to minimize the potential negative impacts on human health and biodiversity. Furthermore, implementing effective waste management practices, such as recycling and composting, can significantly reduce the amount of solid waste that ends up in landfills, promoting a more sustainable and environmentally friendly approach to waste disposal. The open dumping system in Shone Town creates environmental and social problems that result from the dumping site. These problems include air and water pollution, as well as the spread of diseases due to the improper disposal of waste. Additionally, the open dumping system can lead to a decline in property values and quality of life for residents in Shone Town, further highlighting the need for a more sustainable waste management solution. Solid wastes from households, hotels, markets, service areas, and commercial areas are all dumped in an open dumping site, which contains toxic compounds that are harmful to the environment and human health. These toxic compounds can seep into the soil and contaminate groundwater, posing a serious threat to both the ecosystem and the local community. Moreover, the lack of proper waste management infrastructure in Shone Town exacerbates the problem, making it crucial to implement effective waste disposal methods that prioritize environmental protection and public health.
The study used GIS and AHP methodologies to analyze decision support criteria and identify the best location for a solid waste dumping site. The results concluded that the southeast side of the research area is the most appropriate site for solid waste disposal. The final suitability map showed that 51.32 ha of the research area is highly suitable, 54.86 ha is suitable, 34.99 ha is less suitable, and 3,171.22 ha of the study area overall is unsuitable. The study also considered factors such as proximity to residential areas, water bodies, and sensitive ecosystems to ensure minimal impact on public health and the environment. Additionally, the researchers recommended implementing proper waste management practices and regular monitoring to mitigate any potential risks associated with the solid waste disposal site.
Proper solid waste management and advanced technologies such as GIS and AHP are necessary for identifying suitable waste disposal sites to protect the environment and promote public health. These technologies can help in analyzing various factors, such as proximity to residential areas, water bodies, and sensitive ecosystems, ensuring that the chosen waste disposal sites have minimal impact on these important aspects. Moreover, regular monitoring of these sites is crucial to detecting any potential risks or environmental hazards that may arise from the solid waste disposal process. Governments and municipalities should prioritize improving waste disposal and incorporating advanced technologies. By doing so, we can reduce the adverse effects of solid waste on the environment and public health. Further research could focus on optimizing waste management systems to reduce waste generation, improve recycling rates, and enhance sustainability. This research could also explore the potential for implementing more sustainable waste-to-energy technologies, such as anaerobic digestion or gasification, which can help generate renewable energy while minimizing the environmental impact of solid waste disposal. To further create comprehensive waste management policies that suit the unique demands and difficulties of various locations, coordination between governments, companies, and communities is crucial. Moreover, effective waste management programs that take into account the particular requirements and difficulties of various regions require collaboration between governmental entities, businesses, and communities. To further reduce the amount of garbage produced, it is critical to educate the public on correct waste disposal procedures and encourage responsible consumption. To further create comprehensive waste management policies that address the unique demands of each region, coordination between many stakeholders, including companies, communities, and environmental organizations, is crucial.
In conclusion, improving solid waste management is essential for creating a healthier and more sustainable future for us all. By implementing effective waste management strategies, we can reduce the negative impact of garbage on our environment and human health. This includes promoting recycling, composting, and the use of sustainable materials to minimize waste generation. Additionally, investing in research and development for innovative waste management technologies can help us find more efficient and eco-friendly solutions for handling our waste. Governments need to take a proactive approach to this issue and prioritize waste management and recycling strategies to protect the environment and public health. By implementing strict regulations and policies, governments can ensure that industries and businesses adhere to sustainable waste management practices. Furthermore, educating the public about the importance of reducing, reusing, and recycling can encourage individuals to make conscious choices that contribute to a cleaner and healthier environment.
The use of advanced technologies could play a significant role in optimizing waste management systems, reducing waste generation, improving recycling rates, and enhancing sustainability. For example, implementing waste-to-energy technologies can help convert non-recyclable waste into renewable energy sources, reducing the reliance on fossil fuels. Additionally, incentivizing companies to invest in research and development for eco-friendly packaging and production methods can further promote sustainable waste management practices. It is critical to involve the community in this process to foster environmental awareness and promote sustainable living. A collective effort towards solid waste management would lead to a more sustainable and healthier future for all. By implementing effective waste management strategies, such as recycling and composting programs, we can divert a significant amount of waste from landfills. This not only helps to reduce greenhouse gas emissions but also conserves valuable resources that can be reused or repurposed. Moreover, educating individuals about the importance of reducing waste and providing them with accessible resources for proper disposal can empower communities to actively participate in sustainable waste management practices. Communities can also save money on waste disposal costs and create new job opportunities in the recycling and composting industries. Additionally, adopting a circular economy approach, where products are designed to be easily recyclable or biodegradable, can further enhance waste management efforts and promote a more sustainable future.
Overall, this study highlights the importance of responsible waste disposal and the use of advanced technologies such as GIS and AHP to identify suitable waste disposal sites. The findings of this study can be used as a guide for policymakers, environmentalists, and waste management professionals to make informed decisions about waste disposal in urban areas. It is crucial to prioritize sustainability and public health in the management of solid waste to ensure a healthier and cleaner environment for future generations. By implementing responsible waste disposal practices, we can minimize the negative impacts on ecosystems and human health. GIS and AHP technologies provide valuable tools to analyze various factors, such as proximity to residential areas, water sources, and ecologically sensitive zones, ensuring the selection of appropriate waste disposal sites that mitigate potential risks. Additionally, involving stakeholders in decision-making processes can foster a sense of ownership and promote sustainable waste management practices within communities.
4.2. Recommendations
Based on the findings of the study, the following recommendations are suggested to improve the situation: Firstly, it is recommended to implement educational programs that raise awareness about the importance of environmental conservation and sustainable practices. Additionally, policymakers should consider implementing stricter regulations and incentives to encourage businesses and individuals to adopt eco-friendly practices.
The government and municipalities should focus on efficient waste management practices like recycling and composting to reduce landfill waste. Investing in advanced technologies and infrastructure, educating the public about waste segregation, and implementing policies encouraging businesses to adopt sustainable practices can contribute to a more sustainable future.
GIS and AHP technologies are essential for identifying suitable waste disposal sites that minimize public health and environmental impacts. These tools help decision-makers make informed choices based on factors like land availability, transportation infrastructure, and future expansion. By considering these factors, waste management facilities can be strategically placed to minimize environmental impact and optimize efficiency. Engaging with local communities and conducting thorough environmental impact assessments can also help address concerns and conflicts, promoting responsible waste management practices that prioritize public health and environmental sustainability.
Regular monitoring of waste disposal sites is essential for detecting potential environmental hazards and implementing corrective measures. This proactive approach not only protects the environment but also promotes responsible waste management practices among individuals and industries. By closely monitoring sites, authorities can identify violations or non-compliance with regulations, leading to appropriate enforcement actions and penalties. Monitoring also helps gather data on waste types and quantities, which can inform future waste management strategies and initiatives aimed at reducing waste generation and promoting recycling and reuse. Overall, effective monitoring plays a vital role in promoting responsible waste management practices and fostering a more sustainable future.
Local communities should be encouraged to practice responsible consumption and waste disposal through awareness-raising campaigns, educational programs, and collaboration with local businesses. Government regulations and incentives can encourage responsible consumption habits and encourage investors to invest in innovative waste disposal technologies. Raising awareness through public outreach programs fosters a culture of environmental consciousness. Governments can collaborate with businesses to develop recycling infrastructure and promote renewable resources. Supporting research and development in waste management technologies can drive innovation and create economic opportunities. These measures, combined with strict enforcement of environmental regulations, can lead to a more sustainable future.
Governments, businesses, and communities should collaborate to develop comprehensive waste management policies that address regional demands. This can incentivize sustainable practices and reduce waste generation. Clear communication, education, and support from governments can help promote environmentally friendly habits. Governments can also offer incentives for businesses to adopt sustainable practices, enforce strict regulations, and foster a culture of responsibility towards waste management. Collaboration between sectors like businesses, non-profit organizations, and educational institutions can facilitate knowledge sharing and innovative solutions.
Governments can encourage businesses to invest in sustainable waste management practices by providing tax incentives or grants, collaborating with industry experts to develop certification programs, and investing in innovative recycling technologies. These measures can educate consumers about environmentally friendly products and reduce the environmental impact of packaging materials.
Waste-to-energy technologies convert non-recyclable waste into renewable energy, reducing dependence on fossil fuels. They create new job opportunities, contribute to a greener economy, and address landfill overcrowding. They also reduce greenhouse gas emissions, as burning waste produces less carbon dioxide than burning fossil fuels. Promoting waste-to-energy technologies can mitigate environmental pollution and health hazards.
The circular economy approach promotes a sustainable future by reducing waste generation, minimizing waste-to-energy plants, and encouraging reuse and repurposing of materials. It stimulates innovation, creates new business opportunities, and encourages renewable resources. This reduces waste sent to landfills, greenhouse gas emissions, and addresses limited landfill space. Transitioning to a circular economy can lead to a more sustainable and greener future for generations to come.
The summary emphasizes the importance of responsible waste disposal and sustainable waste management practices in fostering a healthier and cleaner environment for future generations. Implementing efficient waste management practices, identifying suitable disposal sites, and educating individuals about waste reduction can minimize its negative impacts on ecosystems and human health. Regular monitoring, stakeholder coordination, and eco-friendly technologies can further advance sustainable practices. Governments and municipalities must prioritize waste management and recycling strategies to protect the environment and public health. Engaging communities in decision-making and providing resources encourages sustainable practices. Future research should focus on optimizing waste management systems and exploring eco-friendly technologies. The public should be educated about the environmental and health risks of improper waste disposal through awareness campaigns, workshops, and educational programs. Collaboration between government agencies, non-profits, and the private sector is crucial for developing comprehensive waste management strategies and incentivizing sustainable practices.
5. Future research perspectives
Future research should focus on optimizing waste management systems, developing sustainable waste-to-energy technologies, exploring circular economy strategies, and understanding the social and economic impacts of waste management. These strategies can help reduce waste generation, improve recycling rates, and reduce the environmental impact of waste disposal. Advanced technologies like artificial intelligence and machine learning can also be explored to streamline waste collection and sorting processes.
Circular economy strategies can be implemented to reduce waste generation and promote the reuse and repurposing of materials. Digital technologies can facilitate these strategies, and understanding the social and economic implications of transitioning to a circular economy can provide valuable insights for policymakers and businesses. Understanding regional differences in solid waste management is crucial for developing effective and sustainable waste management policies. Region-specific strategies can be developed to address specific challenges and maximize resource recovery. Climate change impacts on waste management can also be studied, as they can affect waste management infrastructure and lead to adaptation and mitigation strategies. Collaboration between stakeholders, such as industry leaders, environmental organizations, and local communities, is essential for developing innovative solutions and initiatives that address the challenges of waste management on a larger scale.
Acknowledgments
The authors thank the administrative authorities of the town of Shone and the municipal authorities, the city beautification office, and the municipal administrators of Shone town for their cooperation and for providing permission to conduct this study. The authors also express gratitude to the participants in this study who took the time to provide valuable insights and information. Their willingness to share their experiences and perspectives has significantly contributed to the research findings. Additionally, the author acknowledges the support and guidance provided by academic advisors and colleagues who offered valuable feedback and insights throughout the research process. Finally, the author acknowledges the encouragement and support provided by friends and family, whose unwavering support has been a source of motivation to complete this study.
Disclosure statement
No potential conflict of interest was reported by the author(s).
Data availability statement
The data supporting the results of this study are available at reasonable request from the corresponding author.
Additional information
Funding
Notes on contributors
Ajitesh Singh Chandel
Ajitesh Singh Chandel played a crucial role in a research project by providing guidance, supervision, and insightful insights. His extensive knowledge and ability to communicate complex concepts facilitated the research process. His critical thinking and problem-solving skills were invaluable in overcoming challenges. His collaborative approach fostered a positive research environment, and his strong communication skills helped disseminate the study’s findings. His adaptability and persuasive writing style increased the study’s visibility and impact, leading to further advancements in the field.
Asrat Ermias Weto
Asrat Ermias Weto contributed to the study’s conceptualization, drafted the manuscript, formulated methodology, conducted data analysis, and conducted field investigation. He collaborated with fellow scholars to ensure the precision and reliability of his findings.
Degu Bekele
Degu Bekele’s meticulous manuscript refinement, data collection, and statistical analysis significantly enhanced the study’s clarity, reliability, and validity. His meticulous approach ensured accurate recording and organization of information, while his analytical skills allowed for comprehensive interpretation of data. His expertise in statistical analysis provided a solid foundation for drawing meaningful conclusions, fostering a productive research environment, and revealing significant patterns and trends for future research.
References
- Abbas, I., Naiya, R., & Arigbede, Y. (2011). Use of remote sensing and Gis in effective and efficient solid wast e management planning (a case study of Samaru, Zaria, Nigeria). Journal of Environmental Science, Toxicology and Food Technology, 1(December), 46–47.
- Abebe, M. (2013). Development of a Solid Waste Management Strategy for Hossana Town, Environmental Planning and Landscape Design. MSc thesis.
- Allen, R. W., Davies, H., Cohen, M. A., Mallach, G., Kaufman, J. D., Adar, S. D. & Hricko, A.(2010). The spatial relationship between traffic-generated air pollution and noise in 2 US cities. Environmental Research, 110(1), 89–93.
- Anderson, J. R., Hardy, E. E., Roach, J. T. & Witmer, R. E.(1976). A land use and land cover classification system for use with remote sensor data. Geological survey professional Paper 964, United States Government Printing Office.
- Berisa, G., & Birhanu, Y. (2015). Municipal Solid Waste Disposal Site Selection of Jigjiga Town using GIS and remote sensing techniques, Ethiopia. International Journal of Scientific and Research Publications, 5(1), 2250–3153.
- Birhanu, Y. (2015). Assessment of Solid Waste Management Practices and the Role of Public Participation in Jigjiga Town, Somali Regional State, Ethiopia. International Journal of Environmental Protection and Policy, 3(5), 153. https://doi.org/10.11648/j.ijepp.20150305.16
- Central Statistical Agency (CSA). (2013). Population projection of Ethiopia for all regions at the woreda level from 2014–2017.
- Coban, A., Ertis, I. F., & Cavdaroglu, N. A. (2018). Municipal Solid Waste Management via Multi-Criteria decision-making methods: Acase study in Istanbul. Journal of Environmental Management, 213, 457–468.
- Collins, M. G., Steiner, F. R., & Rushman, M. J. (2001). Land-use suitability analysis in the United States: Historical development and promising technological achievements. Environmental Management, 28(5), 611–621. https://doi.org/10.1007/s002670010247
- Dano, U. L. (2011). A Geographic Information System and Multi-Criteria Decision Analysis in A Geographic Information System and Multi-Criteria Decision Analysis in Proposing New Recreational Park Sites Universiti Teknologi https://doi.org/10.5539/mas.v5n3p39
- Ebistu, T. A., & Minale, A. S. (2013). Solid waste dumping site suitability analysis using geographic information systems (GIS) and remote sensing for Bahir Dar Town, North Western Ethiopia. African Journal of Environmental Science, 7(November), 976–989. https://doi.org/10.5897/AJEST2013.1589
- EGSSAA. (2009). Solid waste: Generation, handling, treatment, and disposal. Environmental guidelines for small-scale activities in Africa (EGSSAA) (Vol. 11)
- Elahi, A., & Samadyar, H. (2014). Municipal Solid Waste Landfill Site Selection Using Analytic Hierarchy Process Method for Tafresh Town. Annals of Surgical Oncology, 22(4), 1294–1300. https://doi.org/10.5829/idosi.mejsr.2014.22.09.21545
- Elmira N. B. , Bin Mokhtar, M., Komoo, I., & Saadiah Hashim, H. (2011). Urban solid waste management is based on geoinformatics technology. Journal of Public Health and Epidemiology, 3(2), 54–60.
- EPA. (2007). National ambient air quality standards for particulate Matter; final rule. Federal register, 72(25), 6114–6229.
- Ersoy, H., & Bulut, F. (2009). An overview of waste management and research. Waste Management & Research, 27(4), 303–315. https://doi.org/10.1177/0734242X08098430
- Fagbohun, B. J., & Aladejana, O. (2016). Integrating knowledge-based multi-criteria evaluation techniques with GIS for landfill site selection: A case study using AHP povezavanaznanjuutemeljenomnogokriterijskometodoocenjevanja s system GIS zaizbirolokacije odlagališča padkos. Materials and Geoenvironment, 63(3), 169–182. https://doi.org/10.1515/rmzmag-2016-0016
- FAO. (2014). Soil suitability rating for land capabilities. Food and Agriculture Organization of the United Nations.
- Gbanie, S. P., Peter, S., Bobby, P., Samuel, J., & Medo, J. (2013). Modeling landfill location using Geographic Information Systems (GIS) and Multi-Criteria Decision Analysis (MCDA): Case study bo, Southern Sierra Leone modeling land fill location using Geographic Information Systems (GIS) and May 2016. https://doi.org/10.1016/j.apgeog.2012.06.013
- Gizachew, T.(2010). Evaluation of landfill site selection using GIS and multicriteria decision analysis. International Journal of Environmental Science and Engineering Research, 1(3), 53–64.
- Habtamu, A. (2001). GIS and remote sensing-based solid waste landfill site selection: Acase of Addis Ababa City. Waste Management & Research, 19(6), 564–568.
- Hammer, G. (2003). Solid waste treatment and disposal: Effects on public health and environmental safety. Biotechnology Advances, 22(1–2), 71–79. https://doi.org/10.1016/j.biotechadv.2003.08.007
- Hasan, M. R., Tetsuo, K., & Islam, S. A. (2009). Landfill demand and allocation for municipal solid waste disposal in Dhaka city—an assessment in a GIS environment. 37(2), 133–149.
- Hering, J. G. (2012). An end to waste? Science, 337(6095), 1623. https://doi.org/10.1126/science.1227092
- Khalek, I. A., Bougher, T. L., Merritt, P. M., Zielinska, B., Khalek, I. A., Bougher, T. L., Merritt, P. M., Zielinska, B., Khalek, I. A., Bougher, T. L., & Merritt, P. M. (2011). Regulated and unregulated emissions from highway heavy-duty diesel engines complying with U.S. Environmental Protection Agency 2007 emissions standards regulated and unregulated emissions from highway heavy-duty diesel engines complying with U.S. En. 2247, https://doi.org/10.3155/1047-3289.61.4.427
- Lemma, E., & Tekilu, H. (2014). Characterization and Disposal of Municipal Solid Waste, Case Study, Hosanna Town. American Journal of Environmental Engineering, 4(6), 162–168. https://doi.org/10.5923/j.ajee.20140406.04
- Martin, D., & Williams, H. (1992). Market-Area analysis and accessibility to primary health-care centres. Environment & Planning A: Economy & Space, 24(7), 1009–1019. https://doi.org/10.1068/a241009
- Mekuria, T. (2006). A Multi-Criteria Analysis for Solid Waste Disposal Site Selection Using Remote Sensing and GIS Department of Earth Sciences, Tsegaye Mekuria Addis Ababa University.
- Mohammedshum, A. A., Gebresilassie, M. A., Rulinda, C. M., Kahsay, G. H., & Tesfay, M. S. (2014). Application of geographic information systems and remote sensing to ineffective solid waste disposal site selection in Wukro Town, Tigray, Ethiopia. International Archives of the Photogrammetry, Remote Sensing, and Spatial Information Sciences (ISPRS Archives), 40(2), 115–119. https://doi.org/10.5194/isprsarchives-XL-2-115-2014
- Nas, S. Dalhatu, A. & Zubair, S. (2010). Effects of proximity to a landfill site on surrounding soil and groundwater quality: A case study of Maiduguri Municipal Dumpsite, Nigeria. International Journal of Environmental Monitoring and Analysis, 1(5), 258–264.
- Osman, K., Bojcetic, N., & Marjanovic, D. (2010). Multi-criteria decision-making in product platform development and evaluation. Applied Mechanics and Materials, 4, 265–272.
- Peter, J. B. (2015). Patterns and correlates of solid waste disposal practices in Dar es Salaam city, Tanzania. African Journal of Environmental Science and Technology, 9(6), 545–556. https://doi.org/10.5897/ajest2014.1831
- Ravinder, J.(2014). Impact of Slope on Soil Erodibility and pollution potential: A review. International Journal of Recent Scientific Research, 5(1), 1–7.
- Saaty, T. L. (1980). The Analytic Hierarchy Process: Planning, Priority Setting, Resource Allocation. New York: McGraw-Hill.
- Saaty Katz, T. L. (2008). Decision-making with the analytic hierarchy process. International Journal of Services Sciences, 1(1), 83. https://doi.org/10.1504/IJSSCI.2008.017590
- Shamshiry, E., Nadi, B., Bin Mokhtar, M., Komoo, I., Saadiah Hashim, H., & Yahaya, N. (2011). Integrated models for solid waste management in tourism regions: Langkawi Island, Malaysia. Journal of Environmental and Public Health, 2011, 1–5. https://doi.org/10.1155/2011/709549
- Shkileva, A. (2021). Implementation of a degassing system at the MSW landfill. Civil Engineering Journal, 7(6), 1008–1014. https://doi.org/10.28991/cej-2021-03091706
- Simsek, C., & Gunduz, O. (2006). A solid waste disposal site selection procedure based on groundwater vulnerability mapping. https://doi.org/10.1007/s00254-005-0111-2
- Sulaiman, H., Kabite, G., & Suryabhagavan, K. V. (2012). GIS-Based solid waste landfill site selection in Addis Ababa, Ethiopia. International Journal of Ecology and Environmental Sciences, 38(3), 59–72.
- Taye, Z. H. (2018, May). GIS and remote sensing applications in solid waste management and optimal site suitability assessment for landfills: The case of Shashemene City, Ethiopia. Norwegian University of Science and Technology.
- United Nations. (2017). World population prospects: 2017 revision population database. https://population.un.org/wpp/.
- Valentina, E. S.(2011). Evaluating site suitability for solid waste landfill siting using geospatial techniques in the Greater Accra Metropolitan Area, Ghana. Geoinformatics, 6(3), 52–69.