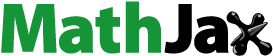
ABSTRACT
Biochar has shown large potential in water treatment because of its low cost, good textural properties, and high reusability. In this study, two porous biochars were developed from the Melia azedarach seeds via direct pyrolysis process (B-700) and through hydrothermal carbonization followed with pyrolysis (HB-700). They were characterized by morphology, structural characteristics, and surface features and used to adsorb the crystal violet (CV) dye in water environment. Results of the isotherm approaches demonstrated that the removal capacity of these biochars reached 119.4 mg/g for B-700, and 209 mg/g for HB-700 (at 45°C). Also, the Avrami model best fitted the kinetic data. The electrostatic attraction was regarded as one of the adsorptions mechanisms of CV dye. The regeneration tests reveal that both B-700 and HB-700 are good reusable adsorbents. Finally, findings of the study showed that the hydrothermal carbonization method that precede the pyrolysis process can improve significantly the adsorption capacity of the produced biochar.
1. Introduction
Nowadays, achieving environmental protection goals from polluted waters requires more effort in developing new cost-effective remediation technologies in conjunction with the excessive increase in human activities. Color effluents generated by several industries (i.e. textile, food, leather, paper, paint, cosmetics, pharmaceutical, etc.) produce various kinds of synthetic dyes with complex aromatic structure, thereby decolorizing them seems difficult and costly [Citation1]. Out of the various dyes, crystal violet (CV) is a triarylmethane cation dye. It has a broad range of applications with unique properties that make it useful in different fields. CV dye has widespread application in the field of animal and veterinary medicine as a biological stain. It is commonly employed for the purpose of protein-dye staining, allowing the identification of bloody fingerprints [Citation2]. Additionally, it is used as a histological and bacterial stain in microbiology, enabling the visualization and identification of various cellular structures and organisms. Also, in medicine, CV is known as gentian violet and utilized as an antifungal agent, a potential anticancer agent, and a dye for medical devices and wound dressing materials [Citation3].
However, the existence of this kind of non-biodegradable dye in high concentrations in water not exclusively makes it carcinogenic to human beings but also interrupts the light leading to damage the aquatic plants by slowing the photosynthesis operation [Citation4]. Addressing this issue can help to safeguard water resources and promote sustainable applications in different industries.
Therefore, several techniques such as photocatalytic degradation, electrocoagulation, membrane filtration, cation exchange membranes, oxidative degradation, electrochemical oxidation, biochemical degradation, and adsorption have been employed to eliminate hazardous dyes (i.e. CV dye) [Citation5].
Over the years, the adsorption method broke some limitations of the other techniques and had been considered the best choice to treat contaminated water for many reasons, such as the low quantity of chemical generation and waste, ease and simplicity of operation, high efficiency, and low cost [Citation6]. These considerable advantages could be proposed as a techno-economical solution for field-scale applications in removing toxic dyes from water. On the other hand, the efficiency of the designed adsorption operation depends on critical criteria, including the choice of the adsorbent and its properties, abundance and effectiveness [Citation7].
Based on the cost, abundance, and various textural properties of adsorbent (chemical stability, porosity, pore volume, and high surface areas), many carbonaceous materials (biochar or hydrochar) prepared from waste biomass were usually used as an alternative adsorbent to activated carbon in adsorbing CV dye molecules from solutions such as palm petioles biochar [Citation8] and hydrochar from straw mushroom [Citation9]. However, research is increasing daily to discover more adsorbents that may be able to tackle contaminated water problems.
In the present study, bio-based biochars from Melia azedarach seeds were prepared by two different methods: (1) biochar prepared via a direct pyrolysis of feedstock under limited O2 conditions at 700°C (named B-700), (2) biochar produced by hydrothermal carbonization followed by pyrolysis (named HB-700). FTIR technique, XRD method, SEM images, BET analyses and pHPZC measurements were done to characterize the samples. After studying their properties, the adsorbents were evaluated for their application potential in removing CV dye as contaminant from water. The adsorption experiments involved studying the influence of pH, time of contact, concentration of dye, adsorbent dose, and temperature. The adsorption capacity and mechanism were also discussed herein. The regeneration and reusability of materials were identified by determining the number of cycles and applications related to the removal rate.
2. Materials and methods
2.1. Preparation of B-700 and HB-700
Melia azedarach seeds (MAS) were collected from a local location in eastern Algeria and utilized as the initial feedstock. The seeds underwent a comprehensive washing process, initially with tap water until achieving clarity, followed by a rinse with distilled water to eliminate any impurities. Subsequently, they were dried under ambient conditions (24 ± 1°C) and further dehydrated in a laboratory oven at 75°C for a duration of 24 hours. After drying, the seeds were crushed and sieved to obtain fine particles ranging in size from 90 μm to 250 μm.
Direct pyrolysis method of the final MAS materials was performed at 700°C to produce B-700 biochar with a heating rate of 10°C/min for 4 hours using a muffle furnace (DAIHAN Scientific) under limited oxygen. The choice of the direct pyrolysis method, along with the specific temperature of 700°C, was based on existing literature [Citation10,Citation11]. This body of research indicates that elevated temperatures facilitate the degradation of organic compounds, leading to the formation of a material consisting primarily of carbon.
For the hydrochar preparation, the MAS powder was treated according to the hydrothermal technique by boiling in distilled water for 2 hours at 190°C, filtering and drying. Afterwards, the treated powder was subjected to the same process used for the previous biochar to obtain HB-700. After the pyrolysis stage, the obtained biochars were soaked in a 0.1 mol/L HCl to eliminate free acids and washed many times with distilled water till the filtrate phase reached pH between 6 and 7. Eventually, the products were dried and stored away from humidity for the subsequent experiments.
2.2. Characterization of biochars
The functional moieties responsible for the uptake of dye on the surface of adsorbents were examined and identified using infrared spectroscopy (Shimadzu model 8400S). The spectra were recorded under range of 4000 to 400 cm−1, utilizing KBr as a background. The data on the crystallographic structure of the two biochars were studied using X-ray diffractometry (Bruker D8 Advance, Germany). The XRD analysis was conducted in the 2θ range from 2° to 80° with a scanning rate of 10°/min. Scanning electron microscopy (EVO15-ZEISS device) was used to explore the morphological properties of B-700 and HB-700 samples. The textural parameters of the B-700 and HB-700 materials were obtained by analyzing nitrogen gas adsorption isotherms measured at 77 K, using ASAP 2020 equipment. The pH point zero charges (pHPZC) of the samples were determined according to the drift method as described in earlier researches [Citation11]. The solutions of 0.01 mol/L NaCl were adjusted by NaOH or HCl to initial pH values ranging from 2 to 11. Then, each sample of 25 mL NaCl was added to 25 mg of the biochar and shaken mechanically for 6 h (at 15 ± 1°C), then the final pH was recorded. The results were plotted and the pHPZC value was given from the intersection point of the curves ΔpH and the initial pH.
2.3. Preparation of CV dye solutions
The CV dye is cationic, also known as Basic Violet 3, and has a chemical formula of C25H30ClN3, MW of 408 g/mol, density of 1.19 g/cm3, solubility in water as 16 g/L at 25°C, and a color index number of 42,555. It was obtained from BIOCHEM Chemopharma in Montreal, Quebec. The chemical structure of the CV dye is depicted in . For the adsorption experiments, standard solutions were prepared by diluting the 1 g/L dye stock solution to the desired concentrations.
2.4. Batch adsorption experiments
Experiments of adsorption were performed in closed flasks containing 25 mL of CV solutions at the desired concentration. The dye solution and biochar were stirred at a constant temperature and different contact times, with a stirring speed of 250 rpm. Subsequently, the mixture was filtered through a 45 μm filter, and the remaining content of CV dye was measured using a spectrophotometric method (SP-3000 Plus, Japan) at the maximum wavelength (λ max = 581 nm).
The final concentrations of CV are then deduced via Beer-Lambert method. The effects of stirring time, concentration of dye (5–300 mg/L), temperature (15, 30, and 45°C), pH (3–11) and biochar dosage (12.5 mg, 25 mg and 50 mg/25 mL) were investigated to determinate the optimum conditions for the maximum adsorption of CV dye. The amount of dye fixed onto adsorbent at the desired time (qt) and equilibrium (qe) in (mg/g) and the efficiency of adsorption (R%) were determined from EquationEquation (1–3)(1)
(1) :
where, Co, Ct and Ce are concentration of dye (mg/L) at the beginning, desired time, and at equilibrium, respectively. V (L) is the volume of the solution of dye, and m (g) is the amount of biochar.
The better fit of the isotherm and kinetic models for the experimental data was selected on the base of statistical values of adjusted determination coefficient R2adj, and the root mean square error (RMSE). The values of the error functions were evaluated using EquationEquation (4, 5)(4)
(4) .
where qe,exp and qe,model (mg/g) are the CV dye adsorption capacity at equilibrium of the experimental and model, respectively. R2 adj is the adjusted coefficient of determination, n represents the number of points in the experiment and p is the number of independent variables in the equation.
3. Results and discussions
3.1. Adsorbents characterization
3.1.1. FT-IR spectra analysis of B-700 and HB-700 samples
The FTIR analysis was used to investigate qualitatively the chemical structure of biochars. displays the FTIR spectra of the prepared adsorbents B-700 and HB-700 which indicated various surface functional groups. Results show a strong broadband between 3160 and 3400 cm−1 corresponding to the O-H stretching frequency vibration which might be due to the absorption of water [Citation12]. The peaks of stretching frequency at 1620 and 1597 cm−1 are related to C=C group [Citation13]. The strong band at 1395 cm−1 is could belong to the C – C and C – N stretching [Citation14]. The characteristic peak located at lower intensity 1110 cm−1 is assigned to C – O–C stretching vibrations [Citation15]. Meanwhile, the small peak observed at 1073 cm−1 is attributed to the C-O stretching of organic compounds such as esters [Citation11,Citation16]. Finally, the narrow peak observed at 740 cm−1 represents the distinctive vibrational patterns of C-H bonds found specifically in aromatic rings [Citation11].
3.1.2. X – ray diffraction analysis (XRD) of B-700 and HB-700
The recorded XRD patterns of B-700 and HB-700 shown in are similar. From the results, it can be observed that the samples exhibited two peaks, where the bands intensity of HB-700 are higher than B-700. The broad band observed in the 20°-30° range is a result of the crystal plane index (002), which is related to the parallel and azimuthal alignment of the aromatic and carbonized structure. Additionally, the high symmetry of the (002) peaks suggests the absence of γ-bands linked to amorphous and aliphatic structures [Citation17,Citation18]. Another broad peak is evident at 44°, which is attributed to (100) diffractions of graphitic and hexagonal carbons that reflect the size of the aromatic lamina [Citation19,Citation20]
3.1.3. SEM images of B-700 and HB-700
depicts the SEM micrograph of B-700 and HB-700, revealing a heterogeneous structure with tunnel-shaped pores in the analyzed samples. Both samples exhibit notable porosity, which arises from the pyrolysis-induced degradation of lignin and cellulose within the biochar structure [Citation21]. This development holds the potential to render the subjected adsorbents highly effective for the removal of CV molecules from water media.
3.1.4. Textural properties of samples
illustrates the N2 adsorption and desorption isotherms of B-700 and HB-700 biochar samples. Both isotherms exhibit Type II behavior with H3-type hysteresis loops, indicating the presence of mesopores in the materials [Citation22]. The pore widths of the biochars are within the mesopore range of 2–50 nm, with B-700 having a central pore width of approximately 3.41 nm () and HB-700 having a central pore width of 3.42 nm (). These findings confirm that the materials possess a mesoporous structure. Moreover, the specific surface area (SBET) and total pore volume (VTotal) values of B-700 (405.1 m2/g and 0.225 cm3/g, respectively) closely resemble those of HB-700 (389.74 m2/g and 0.223 cm3/g), indicating that the two biochars exhibit similar surface characteristics.
3.2. Adsorption study
3.2.1. pH and ionic strength
The values of pHPZC were equal to 6.7 and 7.1 for the B-700 and HB-700, respectively (). The pHPZC confirmed that the surface of the biochar is negatively charged at pH > pHPZC, which promotes the uptake of CV dye in solution. Conversely, for pH < pHPZC, the surface of the two adsorbents becomes positive and therefore adsorption of cationic dye (such CV dye) is not favorable [Citation23].
Figure 6. (a) pHPZC of biochars, (b) Effect of the pH change in CV uptake (Co = 20 mg/L, 15 ± 1°C, t = 240 min and m/V = 1 g/L), and (c) Effect of ions strength on the adsorption of the dye onto biochars.

The influence of the initial pH of the dye solutions on the adsorption was studied in the interval from pH 3 to 11. The variation in the amount of adsorbed dye as a function of pH value is shown in . The amounts of dye retained by the adsorbent from different solutions have been found to be closely related to the value of the pH of solution. The retention of the CV dye by B-700 and HB-700 increases when the pH of the medium becomes basic, where it is appreciable between pH values ranging from 4 to 11. As the dye used is basic, its dissolution in water releases ions colored with a positive charge, which can explain the increase in dye retention rates by the increase in the negatively charged surface of the two biochars and promotes their uptake. Thus, the electrostatic attraction mechanism is present in the actual adsorption process. This mechanism is mainly controlled by the pH of the dye solution, which explains the variations in the removal efficiency of CV molecules onto the two adsorbents [Citation24]. However, the rate of retention of CV dye began to increase at pH = 4 and reaches its maximum at pH up to 9 and 6 for B-700 and HB-700 respectively.
On the other hand, the colored wastewater may contain other elements in the form of salts and various metal ions leading to ionic strength which can affect the adsorption efficiency. The influence of sodium chloride dosage on the elimination of CV dye by the two biochars was investigated with different doses of NaCl solution. According to , the increase in salt concentration from 0.05 to 1 M decreased the adsorption capacity by about 11.51% for B-700 and 07.21% for HB-700 respectively. This might attribute to the fact that the Na+ ions occupy the surface-active sites available on the biochars and reduce the negative charge of these sites which leads to reducing the electrostatic attraction forces between the dye and negative active sites. As result, increasing the ionic strength has an unfavorable effect on the sorption efficiency. Similar findings have been found by other scholars [Citation25,Citation26].
3.2.2. Effect of contact time and adsorption kinetic
The determination of accurate time is important or estimating the cost of adsorption operation. The variation in the quantity of adsorbed dye onto biochar versus contact time (5–300 minutes) is presented in . Results showed that the adsorption of dye occurred in two phases, where in the initial stage it goes up quickly. This is attributed to the high number of available free sites for adsorbing the dye molecules [Citation27]. Within 30 minutes, about 52.15% (10.43 mg/g) of the total dye concentration (20 mg/L) was removed by B-700 and 64.35% (12.87 mg/g) by HB-700. The capacity of adsorption rises with stirring time and attains equilibrium at 120 minutes for the booth biochars.
Figure 7. Effect of stirring time on CV adsorption onto biochar (Co = 20 mg/L, 15 ± 1°C, and m/V = 1 g/L).

Through these results, we note that HB-700 showed more effectiveness in the adsorption process than B-700. After 120 minutes a gradual decrease in the speed of the adsorption process is noted, where CV ions slowly occupy the remaining active sites due to the saturation adsorbent surface [Citation28]. This may also indicates that the intraparticle diffusion is involved in the adsorption process [Citation29]. At 240 minutes, the colorant removal percentage increased to 67.6% (13.52 mg/g) and 99.5% (19.90 mg/g) for the two biochars B-700 and HB-700, respectively.
To define the mechanisms controlled the adsorption interaction between CV dye molecules and biochar, the kinetic data were treated with four models: Pseudo-first-order (PFO), Pseudo-second-order (PSO), Avrami and Intra-particle diffusion (IPD) as shown in and their adsorption parameters data are listed in .
Table 1. Constants of kinetic models and the corresponding values.
The kinetic data modeling using PSO model demonstrated a high level of agreement between the calculated qe values and the corresponding experimental results. Specifically, for B-700, the calculated qe,cal value (qe,cal B-700 = 12.80 mg/g) closely matched the experimental value (qe,exp B-700 = 13.52 mg/g). Similarly, for HB-700, the calculated qe value (qe,cal HB-700 = 19.80 mg/g) exhibited a close resemblance to the experimental value (qe,exp HB-700 = 19.90 mg/g). These findings highlight the superior accuracy of the PSO models compared to the PFO model in predicting the adsorption capacities of the materials under investigation.
However, the adsorption kinetic of both biochars is perfectly followed the adsorption kinetic model of Avrami based on the greater adjusted determination coefficient R2adj and the smaller RMSE values. The Avrami fractional kinetic model, also known as the thermal decomposition kinetic model, is a widely utilized empirical approach for comprehending the kinetics of thermal decomposition reactions [Citation30]. The Avrami kinetic constant was found to be 10−7 and 0.007 min−1 for the B-700 and HB-700 samples, respectively.
In fact, the adsorption process can be influenced by various factors, diffusion of adsorbate through the liquid film surrounded the adsorbent particles, pore diffusion of adsorbate, and adsorbate-adsorbent interactions. The adsorption of CV dye onto biochars is governed by three concurrent steps. The first phase portrays the process of film diffusion, in which the dye molecules rapidly permeate the solid surface. The two subsequent segments are attributed to the intraparticle diffusion process and the attainment of equilibrium, respectively. Thus, intraparticle diffusion involves the attraction of positively charged CV molecules in the solution to deprotonated B-700 (with relatively lower attraction) and HB-700 (with significantly higher attraction) active sites at higher pH levels [Citation8,Citation24]. This attraction is driven by electrostatic forces and it is supported by the findings in sub-section 3.2.1 and other published papers [Citation31].
3.2.3. Effect of adsorbent dose
shows the influence of biochar dose on CV adsorption capacity and removal efficiency. The results showed that, regardless of the initial concentration of the colorant, as the dose of added adsorbent increased, the quantity of dye adsorbed per unit mass decreased, while the adsorption removal efficiency increased with the adsorbent dose. This phenomenon can be mainly attributed to the increased amount of adsorbents, resulting in an increase in the availability of active sites on the surface of each biochar. However, these adsorption sites remained unsaturated during the adsorption reaction. Furthermore, an increase in adsorbent mass may lead to particle agglomeration and aggregation, which can have a negative impact on the adsorption process [Citation32]. Similar results have been previously reported in other studies that investigated the influence of variations in the adsorbent dose on the adsorption of crystal violet [Citation33,Citation34].
3.2.4. Effect of dye concentration and isotherm behavior
Initial dye concentration has a significant effect on the elimination of dye. According to the results illustrated in . When the CV dye content rises from 20 to 300 (mg/L), the quantity of dye adsorbed increases from 13.53 to 37.66 (mg/g) for B-700 and from 19.90 to 92.45 (mg/g) for HB-700, and HB-700 shows better efficiency in eliminating CV dye compared to B-700. The increase in the content of dye molecules causes an increase in the driving force, which weakens all the resistances of the dye between the aqueous and solid phases, resulting in an increase in adsorption [Citation35]. The decrease in removal efficiency is probably due to the increase in the number of CV dye molecules in solution for the same quantity of biochar [Citation36]. Furthermore, we note that for low initial concentrations, almost the whole amount of CV dye was adsorbed at the beginning; this is explained by the availability of vacant sites on the biochar surface. Above 20 mg/L, the adsorbed quantity of CV dye decreased which is due to the progressive saturation of adsorbent [Citation25,Citation37].
Adsorption isotherms refer to mathematical equations that establish the correlation between the quantity of adsorbate assimilated onto an adsorbent and the concentration of adsorbate in the solution at a constant temperature, once equilibrium has been attained. However, the adsorption isotherms of CV dye by B-700 and HB-700 were studied at different temperatures of 15, 30 and 45°C. The data from the isotherm experiments are displayed in . The Langmuir, Freundlich, and Liu isotherm models were applied and fitted to obtain more information about the adsorption mechanism and adsorbent capacity under the specific conditions.
The Langmuir model suggests the homogeneity of adsorbent surface without any interaction between the adsorbed molecules [Citation38]. According to this model, the saturation can attain when the adsorption process can no longer occur at equilibrium. The adsorbate molecules occupy specific sites surface of adsorbent, and once a dye molecule takes up a site, no further absorption can take place [Citation23].
The Freundlich model assumes that sorption occurs on a heterogeneous surface of adsorbent [Citation39]. This model suggests that the adsorbed amount increases with the rises of adsorbate content [Citation23].
The Liu isotherm model is a mathematical model that characterizes the adsorption process of a solute onto a solid surface. The Liu isotherm model assumes that the amount of adsorbed solute on the solid surface is dependent on both the solute concentration and the adsorbent’s heterogeneity [Citation40].
The suitability of the studied models was assessed based on the values of R2 adj (adjusted R-squared) and RMSE (root mean square error) (). According to the high values of R2 adj, and low values of RMSE, it can be concluded that the Freundlich isotherm model is the most appropriate for characterizing the adsorption process of CV dye onto the two biochars. This indicates the heterogeneity of adsorbent surface, and thus the Freundlich adsorption isotherm model should be used to describe the adsorption process. At a temperature of 15°C, the adsorption capacity of B-700 and HB-700 is 39.6 mg/g and 97.1 mg/g, respectively. The capacity for a substance to be adsorbed is influenced by both the characteristics of the adsorbent material being used and the initial concentration of the substance that is being adsorbed. presents a comparison of the maximum adsorption capacities (Qmax) of B-700 and HB-700 for crystal violet with the corresponding Qmax values with other biochars in literature. The values of n > 1 (From Freundlich equation) for both biochars indicate favorable adsorption conditions [Citation48], which is consistent with the findings of previous studies [Citation1,Citation37].
Table 2. Constants of isotherm models and the corresponding values.
Table 3. Values of maximum adsorption capacities of similar biochars for crystal violet dye removal.
An intriguing observation from the equilibrium data was the response of the biochars to changes in temperature, as depicted in . The Qmax values for both B-700 and HB-700 exhibited a significant increase with the rise in temperature. For B-700, the values escalated from 39.6 mg/g (at 15°C) to 119.4 mg/g (at 45°C). Similarly, for HB-700, the values raised from 97.1 mg/g (at 15°C) to 209 mg/g (at 45°C).
3.2.5. Thermodynamic study
The impact of temperature on the adsorption process of CV dye using B-700 and HB-700 was investigated across a range of temperatures (15, 30, and 45°C). The corresponding results are shown in . It can be observed that the removal of CV dye using B-700 exhibited a slight increase with the rise in temperature. However, a notable improvement in the removal of CV dye was observed when the temperature of the solution was increased.
The observed phenomenon can be attributed to the rapid diffusion of CV dye molecules onto the adsorbent surface at higher temperatures. As the temperature increases, the collisions between the dye molecules and the adsorption sites become more frequent, resulting in enhanced adsorption efficiency. Additionally, the rise in temperature may lead to an increase in the porosity and overall pore volume of the adsorbent materials, further facilitating the adsorption process [Citation49,Citation50].
On the other hand, when the initial temperature of the dye solution was raised from 15 to 45°C with HB-700, a decline in the adsorption capacity of CV dye was observed. This decrease in adsorption may be attributed to the heightened solubility of the dye particles, which results in a stronger interaction force between the dye and the solvent than between the dye and HB-700 [Citation51]. Moreover, this could be attributed to the disruption of the binding forces that exist on the surface of HB-700 when exposed to elevated temperatures [Citation52]. In essence, the findings indicate that variations in temperature directly affected the adsorption capacity, resulting in a decrease in Qmax value. This suggests the presence of a weak force, specifically the Van der Waals force, playing a role in the adsorption process and mechanism [Citation11].
Furthermore, the difference between the observed increase in adsorption capacity of CV dye with B-700, which underwent only one step of treatment, and the decrease in adsorption capacity of HB-700, which underwent a two-step treatment, can be attributed to the fact that elevated temperatures can lead to an enlargement of the pore size and surface area of B-700, thus enhancing its adsorption capacity [Citation53]. However, the disparity in CV dye adsorption between B-700 and HB-700 at higher temperatures may be due to the stable nature of the adsorbent materials under such conditions [Citation54].
In order to identify the mechanisms of adsorption, it is crucial to conduct a thermodynamic investigation. This involves determining various parameters such as the standard Gibbs free energy change (ΔG°), standard enthalpy change (ΔH°) which reflects the total heat content of the system, and standard entropy change (ΔS°). These parameters can be calculated using the equations shown below [Citation55]:
The equations mentioned above involve the ideal gas constant R in units of kJ.mol−1K−1, temperature T measured in kelvin (K), and the dimensionless thermodynamic equilibrium constant Ke° [Citation55].
The equilibrium constant Ke° (Equation 8) was determined using the Langmuir energy constant KL (L/mg), crystal violet mass molar M (mg/mol), and a standard reference solution C° (1 mol/L) to resolve the issue of units, which has been previously discussed in the literature [Citation55,Citation56]. The right dimensionless constant can be achieved using the following equation [Citation57,Citation58]:
The thermodynamic behavior of the adsorption process of CV dye onto B-700 and HB-700 was investigated by estimating the values of thermodynamic parameters, which are listed in .
Table 4. Thermodynamics parameters of biochars.
The negative value of ΔG° indicates that the adsorption process was spontaneous and possible [Citation59]. Furthermore, the ΔG° values for B-700 and HB-700, demonstrate that physisorption mechanism might be the dominant process for CV adsorption in this study [Citation60,Citation61]. The magnitude in the value of enthalpy (ΔH°) lies in the range of − 2.1 to − 20.9 and − 80 to −200 kJ.mol−1 for physical and chemical adsorptions, respectively [Citation61]. Hence, the negative values of ΔH° of − 47.74 and − 59.58 for B-700 and HB-700 respectively, reveal that the removal mechanism of CV dye from solution using the two biochars is an exothermic reaction [Citation62], involve a combination of physisorption and physiochemical forces, in which electrostatic forces and other sorption mechanisms like hydrogen bonding, π-π interaction, and cation-π interaction have a share in the sorption process. Moreover, the negative (ΔS°) parameter value shows that the adsorption process leads to a reduction in random collisions and irregularities at the adsorbent surface. These results are consistent with earlier studies [Citation59,Citation63,Citation64].
3.2.6. Reusability of adsorbents
The recycling process has various advantages; it provides economic and environmental benefits. In order to understand the regeneration cycle, the process of adsorption and desorption were studied in this work for up to five cycles. Firstly, 1 g of biochar was stirred with 1000 mL of 20 mg/L dye solution for 240 minutes. The data of removal efficiency on regenerated biochars are shown in . The results reflected that after the fifth cycle, the efficiency of removal goes down from 68.02% to 27.95% for B-700, and from 98.76% to 37.73% for HB-700. Where the adsorption efficiency of the first three cycles and four cycles for B-700 and HB-700 respectively are up to 50%. This result, confirming the better reuse performance of biochars.
Figure 10. (a) Adsorption cycles of biochars and (b) the reuse test of the regenerated biochars dye (Co = 20 mg/L for (a), Co = 10–300 mg/L for (b), t = 240 min, 15 ± 1°C, and m/V = 1 g/L).

After that, 0.1 M HCl solution was used for desorption of dye from the saturated adsorbent, and the mixture of biochar/HCl solution was stirred for 4 h according to the previous method reported in the literature [Citation11]. The biochar was then filtered, washed with distilled water, and oven dried at 105°C for 24 h. Finally, the dried biochars were used again for dye removal. The efficiency of regenerated adsorbents to remove CV dye are presented in .
From the results, it was found that after regeneration, HB-700 still retained good depigmentation performance. While we note, the efficiency of CV removal by regenerated B-700 increased in comparison with the initial B-700. This may be due to the liberation of more of active sites on the surface of the biochar under the action of the various factors undergoing during the use and regeneration of the adsorbent (i.e. acid washing, washing with distilled water, and drying).
It is worth noting that, the adsorbents used in this study is a vegetable material which has good combustion properties. At the end of the adsorption cycle and when the biochar has been completely degraded, it can be used as fuel in specialized incinerators. This proposal is part of the circular economy concept and the preservation of the environment.
4. Conclusion
Melia azedarach seeds were used to produce B-700 biochar and HB-700 hydrochar as effective adsorbents to recover crystal violet dye from water. HB-700 demonstrated the highest adsorption performance with the Langmuir adsorption capacity of 209 mg/g at 45°C. The adsorption process was determined to be spontaneous and exothermic. The mechanisms underlying the adsorption process were identified to involve mainly pore filling, Van der Waals forces, and electrostatic attraction. The prepared adsorbents exhibited excellent reusability, retaining their effectiveness for up to four cycles without requiring additional treatment. This study emphasizes the potential of utilizing various types of biochar derived from Melia azedarach seeds as cost-effective and efficient materials for the remediation of colored effluents in an environmentally friendly manner.
Statements and declarations
Asma Nouioua: Writing – original draft. Dhirar Ben Salem: Conceptualization, writing – review & editing. Abdelkader Ouakouak: Conceptualization, Writing – review & editing. Noureddine Rouahna: Writing – review & editing. Omirserik Baigenzhenov: Conceptualization, writing – review & editing. Ahmad Hosseini-Bandegharaei: Conceptualization, writing – review & editing.
Acknowledgments
This work was supported by the LARHYSS laboratory, University of Biskra and the DGRSDT-Algeria.
Disclosure statement
No potential conflict of interest was reported by the author(s).
Data availability statement
The data that support the findings of this study are available from the corresponding author, [Nouioua A.], upon reasonable request http://dx.doi.org/10.1080/21655979.2023.2236843.
Additional information
Funding
References
- Mittal A, Mittal J, Malviya A, et al. Adsorption of hazardous dye crystal violet from wastewater by waste materials. J Colloid Interface Sci. 2010;343(2):463–473. doi: 10.1016/j.jcis.2009.11.060
- Saeed A, Sharif M, Iqbal M. Application potential of grapefruit peel as dye sorbent: Kinetics, equilibrium and mechanism of crystal violet adsorption. J Hazard Mater. 2010;179(1–3):564–572. doi: 10.1016/j.jhazmat.2010.03.041.
- Maley AM, Arbiser JL. Gentian violet: A 19th century drug re-emerges in the 21st century. Exp Dermatol. 2013;22(12):775–780. doi: 10.1111/exd.12257
- Abu Elella MH, Sabaa MW, ElHafeez EA, et al. Crystal violet dye removal using crosslinked grafted xanthan gum. Int j biol macromol. 2019;137:1086–1101. doi: 10.1016/j.ijbiomac.2019.06.243
- Tran HN, Wang YF, You SJ, et al. Insights into the mechanism of cationic dye adsorption on activated charcoal: The importance of Π–Π interactions. Process SafEnviron Prot. 2017;107:168–180. doi: 10.1016/j.psep.2017.02.010
- Tran HN, Tomul F, Thi Hoang Ha N, et al. Innovative spherical biochar for pharmaceutical removal from water: Insight into adsorption mechanism. J Hazard Mater. 2020;394(January):122255. doi: 10.1016/j.jhazmat.2020.122255
- Aichour A, Zaghouane-Boudiaf H, Mohamed Zuki FB, et al. Low-cost, biodegradable and highly effective adsorbents for batch and column fixed bed adsorption processes of methylene blue. J Environ Chem Eng. 2019;7(5):103409. doi: 10.1016/j.jece.2019.103409
- Chahinez H-O, Abdelkader O, Leila Y, et al. One-stage preparation of palm petiole-derived biochar: Characterization and application for adsorption of crystal violet dye in water. Environ Technol Innov. 2020;19:100872. doi: 10.1016/j.eti.2020.100872
- Zulfajri M, Kao YT, Huang GG. Retrieve of residual waste of carbon dots derived from straw mushroom as a hydrochar for the removal of organic dyes from aqueous solutions. Sustainable Chem Pharm. 2021;22(March):100469. doi: 10.1016/j.scp.2021.100469.
- Hadj-Otmane C, Ouakouak A, Touahra F, et al. Date palm petiole–derived biochar: effect of pyrolysis temperature and adsorption properties of hazardous cationic dye from water. Biomass Convers Biorefin. 2022. doi:10.1007/s13399-022-03127-3.
- Salem DB, Ouakouak A, Touahra F, et al. Easy separable, floatable, and recyclable magnetic-biochar/alginate bead as super-adsorbent for adsorbing copper ions in water media. Biores Technol. 2023;383:129225. doi: 10.1016/j.biortech.2023.129225
- Fazlzadeh M, Khosravi R, Zarei A. Green synthesis of zinc oxide nanoparticles using Peganum harmala seed extract, and loaded on Peganum harmala seed powdered activated carbon as new adsorbent for removal of Cr(VI) from aqueous solution. Ecol Eng. 2017;103:180–190. doi: 10.1016/j.ecoleng.2017.02.052
- Nazir R, Khan M, Rehman RU, et al. Adsorption of selected azo dyes from an aqueous solution by activated carbon derived from Monotheca buxifolia waste seeds. Soil Water Res. 2020;15(3):166–172. doi: 10.17221/59/2019-SWR
- Giraldo S, Robles I, Godínez LA, et al. Experimental and theoretical insights on methylene blue removal from wastewater using an adsorbent obtained from the residues of the orange industry. Molecules. 2021;26(15):4555. doi: 10.3390/molecules26154555
- Wang H, Lai X, Zhao W, et al. Efficient removal of crystal violet dye using EDTA/graphene oxide functionalized corncob: A novel low cost adsorbent. RSC Adv. 2019;9(38):21996–22003. doi: 10.1039/c9ra04003j
- Verma A, Kumar P, Rastogi V, et al. Preparation and evaluation of polymeric beads composed of Chitosan–Gellan Gum–Gum Ghatti/-Gum Karaya polyelectrolyte complexes as polymeric carrier for enteric sustained delivery of Diclofenac sodium. Future J Pharm Sci. 2021;7(1). doi: 10.1186/s43094-021-00343-y
- Huang Y, Yin X, Wu C, et al. Effects of metal catalysts on CO2 gasification reactivity of biomass char. Biotechnol Adv. 2009;27(5):568–572. doi: 10.1016/j.biotechadv.2009.04.013.
- Yan Y, Manickam S, Lester E, et al. Synthesis of graphene oxide and graphene quantum dots from miscanthus via ultrasound-assisted mechano-chemical cracking method. Ultrason Sonochem. 2021;73:105519. doi: 10.1016/j.ultsonch.2021.105519
- Lu L, Kong C, Sahajwalla V, et al. Char structural ordering during pyrolysis and combustion and its influence on char reactivity. Fuel. 2002;81(9):1215–1225. doi: 10.1016/S0016-2361(02)00035-2
- Mohan D, Abhishek K, Sarswat A, et al. Biochar production and applications in soil fertility and carbon sequestration – a sustainable solution to crop-residue burning in India. RSC Adv. 2018;8(1):508–520. doi: 10.1039/c7ra10353k
- Rouahna N, Salem DB, Bouchareb I, et al. Reduction of crystal violet dye from water by pomegranate peel–derived efficient biochar: influencing factors and adsorption behaviour. Water Air Soil Pollut. 2023;234(5):324. doi: 10.1007/s11270-023-06338-0
- Thommes M, Kaneko K, Neimark AV, et al. Physisorption of gases, with special reference to the evaluation of surface area and pore size distribution (IUPAC Technical Report). Pure Appl Chem. 2015;87(9–10):1051–1069. doi: 10.1515/pac-2014-1117
- Abbas M, Harrache Z, Trari M. Removal of gentian violet in aqueous solution by activated carbon equilibrium, kinetics, and thermodynamic study. Adsorpt Sci Technol. 2019;37(7–8):566–589. doi: 10.1177/0263617419864504.
- Jabar JM, Adebayo MA, Owokotomo IA, et al. Synthesis of high surface area mesoporous ZnCl2–activated cocoa (Theobroma cacao L) leaves biochar derived via pyrolysis for crystal violet dye removal. Heliyon. 2022;8(10):e10873. doi: 10.1016/j.heliyon.2022.e10873.
- Moharm AE, El Naeem GA, Soliman HMA, et al. Fabrication and characterization of effective biochar biosorbent derived from agricultural waste to remove cationic dyes from wastewater. Polymers. 2022;14(13):2587. doi: 10.3390/polym14132587
- Salah Omer A, El Naeem GA, Abd-Elhamid AI, et al. Adsorption of crystal violet and methylene blue dyes using a cellulose-based adsorbent from sugercane bagasse: characterization, kinetic and isotherm studies. J Mater Res Technol. 2022;19:3241–3254. doi: 10.1016/j.jmrt.2022.06.045
- Smith JA, Jaffé PR, Chiou CT. Effect of Ten quaternary ammonium cations on tetrachloromethane sorption to clay from water. Environ Sci Technol. 1990;24(8):1167–1172. doi: 10.1021/es00078a003.
- Elsherif K, El-Dali A, Alkarewi AA, et al. Adsorption of crystal violet dye onto olive leaves powder: Equilibrium and kinetic studies. Chem Int. 2021;7(2):79–89. https://papers.ssrn.com/sol3/papers.cfm?abstract_id=3865960
- Manzoor Q, Nadeem R, Iqbal M, et al. Organic acids pretreatment effect on Rosa bourbonia phyto-biomass for removal of Pb(II) and Cu(II) from aqueous media. Biores Technol. 2013;132:446–452. doi: 10.1016/j.biortech.2013.01.156
- Adebayo MA, Areo FI. Removal of phenol and 4-nitrophenol from wastewater using a composite prepared from clay and cocos nucifera shell: kinetic, equilibrium and thermodynamic studies. Resour Environ Sustainability. 2021;3:100020. doi: 10.1016/j.resenv.2021.100020
- Jabar JM, Adebayo MA, Odusote YA, et al. Valorization of microwave-assisted H3PO4-activated plantain (Musa paradisiacal L) leaf biochar for malachite green sequestration: models and mechanism of adsorption. Results Eng. 2023;18:101129. doi: 10.1016/j.rineng.2023.101129
- Mosoarca G, Vancea C, Popa S, et al. Crystal violet adsorption on eco-friendly lignocellulosic material obtained from motherwort (Leonurus cardiaca L.) Biomass. Polymers. 2022;14(18):3825. doi: 10.3390/polym14183825
- Gemici BT, Ucun Ozel H, Ozel HB. Adsorption behaviors of crystal violet from aqueous solution using Anatolian black pine (Pinus nigra Arnold.): kinetic and equilibrium studies. Sep Sci Technol (Philadelphia). 2020;55(3):406–414. doi: 10.1080/01496395.2019.1577268.
- Nieva AD, Avena LGS, Pascual MAM, et al. Characterization of powdered pineapple (Ananas comosus) crown leaves as adsorbent for crystal violet in aqueous solutions. IOP Conf Ser Earth Environ Sci. 2020;563(1):012010. doi: 10.1088/1755-1315/563/1/012010
- Zamouche M, Habib A, Saaidia K, et al. Batch mode for adsorption of crystal violet by cedar cone forest waste. SN Appl Sci. 2020;2(2). doi: https://doi.org/10.1007/s42452-020-1976-0
- Banerjee S, Chattopadhyaya MC. Adsorption characteristics for the removal of a toxic dye, tartrazine from aqueous solutions by a low cost agricultural by-product. Arabian J Chem. 2017;10:S1629–S1638. doi: 10.1016/j.arabjc.2013.06.005
- Imran MS, Javed T, Areej I, et al. Sequestration of crystal violet dye from wastewater using low-cost coconut husk as a potential adsorbent. Water Sci Technol. 2022;85(8):2295–2317. doi: 10.2166/wst.2022.124
- Fu J, Zhu J, Wang Z, et al. Highly-efficient and selective adsorption of anionic dyes onto hollow polymer microcapsules having a high surface-density of amino groups: Isotherms, kinetics, thermodynamics and mechanism. J Colloid Interface Sci. 2019;542:123–135. doi: 10.1016/j.jcis.2019.01.131
- Oloo CM, Onyari JM, Wanyonyi WC, et al. Adsorptive removal of hazardous crystal violet dye form aqueous solution using Rhizophora mucronata stem-barks: equilibrium and kinetics studies. Environ Chemi Ecotoxicol. 2020;2:64–72. doi: 10.1016/j.enceco.2020.05.001
- Romano NC, Tentler Prola LD, Passig FH, et al. Thermal treated sugarcane bagasse for acetylsalicylic acid removal: dynamic and equilibrium studies, cycles of reuse and mechanisms. Int J Environ Anal Chem. 2022:1–17. doi: 10.1080/03067319.2022.2130283
- Wathukarage A, Herath I, Iqbal MCMM, et al. Mechanistic understanding of crystal violet dye sorption by woody biochar: implications for wastewater treatment. Environ Geochem Health. 2019;41(4):1647–1661. doi: https://doi.org/10.1007/s10653-017-0013-8
- Kyi PP, Quansah JO, Lee C, et al. The removal of crystal violet from textile wastewater using palm kernel shell-derived biochar. Appl Sci. 2020;10(7):2251. doi: 10.3390/app10072251
- Ahmad Khan F, Ahad A, Shah SS, et al. Adsorption of crystal violet dye using Platanus orientalis (Chinar tree) leaf powder and its biochar: equilibrium, kinetics and thermodynamics study. Int J Environ Anal Chem. 2021:1–21. doi: 10.1080/03067319.2021.1931854
- Amin MT, Alazba AA, Shafiq M. Successful application of eucalyptus camdulensis biochar in the batch adsorption of crystal violet and methylene blue dyes from aqueous solution. Sustainability (Switzerland). 2021;13(7):3600. doi: 10.3390/su13073600
- DARAMA SE, Uçar Ö, Çoruh S. Crystal violet removal study with natural and biochar prina from aqueous solutions. Bull Biotechnol. 2021;2(1):1–5. doi: 10.51539/biotech.734889
- Sewu DD, Jung H, Kim SS, et al. Decolorization of cationic and anionic dye-laden wastewater by steam-activated biochar produced at an industrial-scale from spent mushroom substrate. Biores Technol. 2019 November 2018;277:77–86. doi: 10.1016/j.biortech.2019.01.034
- Nithyalakshmi B, Saraswathi R. Removal of colorants from wastewater using biochar derived from leaf waste. Biomass Convers Biorefin. 2023;13(2):1311–1327. doi: 10.1007/s13399-021-01776-4.
- Poots AVJP, Mckay G, Healy JJ, et al. All use subject to JSTOR terms and conditions removal from using of effluent wood basic as an dye adsorbent. J Water Pollut Control Fed. 1978;50(5):926–935. http://www.jstor.org/stable/25039656
- Dwiyaniti M, Elang Barruna AG, Muhamad Naufal R, et al. Extremely high surface area of activated carbon originated from sugarcane bagasse. IOP Conf Ser Mater Sci Eng. 2020;909(1):012018. doi: 10.1088/1757-899X/909/1/012018
- Wu J, Zhang T, Chen C, et al. Spent substrate of Ganodorma lucidum as a new bio-adsorbent for adsorption of three typical dyes. Biores Technol. 2018;266:134–138. doi: 10.1016/j.biortech.2018.06.078
- Dotto GL, Lima EC, Pinto LAA. Biosorption of food dyes onto Spirulina platensis nanoparticles: Equilibrium isotherm and thermodynamic analysis. Biores Technol. 2012;103(1):123–130. doi: 10.1016/j.biortech.2011.10.038.
- Huff MD, Lee JW. Biochar-surface oxygenation with hydrogen peroxide. J Environ Manage. 2016;165:17–21. doi: 10.1016/j.jenvman.2015.08.046
- Liu J, Wang Y, Fang Y, et al. Removal of crystal violet and methylene blue from aqueous solutions using the fly ash-based adsorbent material-supported zero-valent iron. J Mol Liq. 2018;250:468–476. doi: 10.1016/j.molliq.2017.12.003
- Shoukat S, Bhatti HN, Iqbal M, et al. Mango stone biocomposite preparation and application for crystal violet adsorption: A mechanistic study. Microporous Mesoporous Mater. 2017;239:180–189. doi: 10.1016/j.micromeso.2016.10.004
- Lima EC, Hosseini-Bandegharaei A, Moreno-Piraján JC, et al. A critical review of the estimation of the thermodynamic parameters on adsorption equilibria. wrong use of equilibrium constant in the Van’t Hoof equation for calculation of thermodynamic parameters of adsorption. J Mol Liq. 2019;273:425–434. doi: 10.1016/j.molliq.2018.10.048
- Rout DR, Jena HM. Removal of phenol from aqueous solution using reduced graphene oxide as adsorbent: isotherm, kinetic, and thermodynamic studies. Environ Sci Pollut Res. 2022;29(21):32105–32119. doi: 10.1007/s11356-021-17944-y.
- Peiravi-Rivash O, Mashreghi M, Baigenzhenov O, et al. Producing bacterial nano-cellulose and keratin from wastes to synthesize keratin/cellulose nanobiocomposite for removal of dyes and heavy metal ions from waters and wastewaters. Colloids Surf A Physicochem Eng Asp. 2023;656:130355. doi: 10.1016/j.colsurfa.2022.130355
- Rahmani-Sani A, Singh P, Raizada P, et al. Use of chicken feather and eggshell to synthesize a novel magnetized activated carbon for sorption of heavy metal ions. Biores Technol. 2020;297:122452. doi: 10.1016/j.biortech.2019.122452
- Foroutan R, Peighambardoust SJ, Peighambardoust SH, et al. Adsorption of crystal violet dye using activated carbon of lemon wood and activated carbon/fe3 o4 magnetic nanocomposite from aqueous solutions: A kinetic, equilibrium and thermodynamic study. Molecules. 2021;26(8):1–19. doi: 10.3390/molecules26082241.
- El Haddad M, Slimani R, Mamouni R, et al. Removal of two textile dyes from aqueous solutions onto calcined bones. J Assoc Arab Univ Basic Appl Sci. 2013;14(1):51–59. doi: 10.1016/j.jaubas.2013.03.002.
- Húmpola PD, Odetti HS, Fertitta AE, et al. Thermodynamic analysis of adsorption models of phenol in liquid phase on different activated carbons. J Chil Chem Soc. 2013;58(1):1541–1544. doi: https://doi.org/10.4067/S0717-97072013000100009
- Monte Blanco SPD, Scheufele FB, Módenes AN, et al. Kinetic, equilibrium and thermodynamic phenomenological modeling of reactive dye adsorption onto polymeric adsorbent. Chem Eng J. 2017;307:466–475. doi: 10.1016/j.cej.2016.08.104
- Li Y, Wang S, Shen Z, et al. Gradient adsorption of methylene blue and crystal violet onto compound microporous silica from aqueous medium. ACS Omega. 2020;5(43):28382–28392. doi: 10.1021/acsomega.0c04437
- Zazycki MA, Borba PA, Silva RNF, et al. Chitin derived biochar as an alternative adsorbent to treat colored effluents containing methyl violet dye. Adv Powder Technol. 2019;30(8):1494–1503. doi: 10.1016/j.apt.2019.04.026