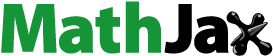
ABSTRACT
Deep eutectic solvent pretreatment with different temperatures and durations was applied to corncob to increase hydrogen yield via photo-fermentation. The correlation of composition, enzymatic hydrolysis, and hydrogen production in pretreated corncobs, as well as energy conversion was evaluated. Deep eutectic solvent pretreatment effectively dissolved lignin, retained cellulose, and enhanced both enzymatic hydrolysis and hydrogen production. The maximum cumulative hydrogen yield obtained under a pretreatment condition of 50°C and 12 h was 677.45 mL; this was 2.72 times higher than that of untreated corncob, and the corresponding lignin removal and enzymatic reduction of sugar concentration were 79.15% and 49.83 g/L, respectively; the highest energy conversion efficiency was 12.08%. The hydrogen production delay period was shortened, and the maximum shortening time was 18.9 h. Moreover, the cellulose content in pretreated corncob was positively correlated with both reducing sugar concentration and hydrogen yield and had the strongest influence on hydrogen production.
GRAPHICAL ABSTRACT
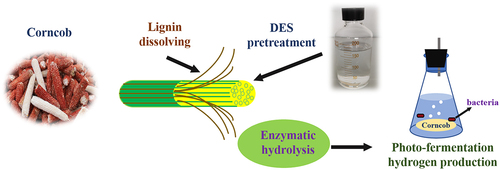
1. Introduction
The sustained and massive consumption of fossil fuels has caused serious environmental pollution and ecological damage; thus, both the development and utilization of renewable energy resources are attracting growing interest worldwide [Citation1]. Hydrogen has been considered as a promising alternative to traditional fossil fuels owing to its renewability, lack of harmful emissions and high energy density [Citation2]. With the increasing attention that is directed to hydrogen, China has included ‘hydrogen energy and hydrogen fuel cell technology innovation’ into its ‘Energy Technology Revolution Innovation Action Plan (2016–2030),’ marking the entry of hydrogen into China’s energy strategy. Furthermore, ‘The Blue Book of China’s Hydrogen Energy Industry Infrastructure Development’ outlines the development plan and technology development route of the hydrogen industry infrastructure, stipulating the direction for the application and industrial development of hydrogen energy. In addition, the worldwide carbon neutrality strategy places increasing importance on the utilization of hydrogen. The Chinese government actively promotes the development of the whole chain of hydrogen production, storage, transportation, sale, and utilization, and encourages the production of green hydrogen from renewable energy. Green hydrogen production technologies include hydrogen production based on solar power generation and wind power generation, as well as biological fermentation. Among these green methods for producing hydrogen, biological hydrogen production is an important measure to achieve emission reduction and the low carbon goal, as well as to build a beautiful countryside in China. Photo-fermentation hydrogen production (PFHP) can utilize various organic wastes as substrates, and offers the advantages of environmental friendliness, mild reaction conditions, and high substrate conversion efficiency [Citation3,Citation4]. Because of these advantages, PFHP has attracted substantial attention in the field of biological hydrogen production. PFHP from agricultural residues (such as corn stover, wheat stover, and corncob) [Citation5] has the dual benefits of alleviating environmental stress caused by conventional disposal (via open burning or landfill) and achieving energy production. Corncob, which is rich in cellulose and hemicellulose, is the main by-product of corn grain. Corncob can be enzymatically converted into sugar that is used by photosynthetic bacteria to produce hydrogen; thus, corncob is regarded as a promising substrate for PFHP. However, the cellulose, hemicellulose, and lignin contained in corncob are intertwined and present in the form of a cellulose-hemicellulose-lignin network. The complex structure of this network restricts the enzymolysis of corncob, and therefore, only a small amount of available substances can be acquired by hydrogen producing bacteria in the subsequent hydrogen production process [Citation5]. To overcome this limitation, it is necessary to find a relatively cheap, eco-friendly, and efficient pretreatment method that can break the network structure of corncob, thus improving its enzymatic hydrolysis ability and providing sufficient substances for PFHP [Citation6,Citation7].
A deep eutectic solvent (DES) is a stable, biodegradable, and recyclable eutectic mixture [Citation8,Citation9]. DES has been used in various industrial applications because of its environmental friendliness, low cost, and low sensitivity to the environment compared with an ionic liquid [Citation10]. It is synthesized by mixing a hydrogen bond donor and a hydrogen bond acceptor [Citation11]. Usually, choline chloride (ChCl) is the most commonly used hydrogen bond acceptor [Citation12]; hydrogen bond donors for the synthesis of DES mainly include acid, polyol, and amine [Citation13,Citation14] because of their low price and phase catalytic transfer properties. It has been reported that DES can selectively separate lignin and cellulose by breaking the ether bonds in the carbohydrate complex to efficiently deconstruct plant cell walls, which is vital for the selective utilization of cellulose in lignocellulosic biomass [Citation15]. As a green solvent with promising potential, DES has been widely applied for lignocellulose pretreatment [Citation16–18]. After the pretreatment of DES (formed by ChCl and lactic acid), 88% of lignin was removed from oil palm empty fruit bunch [Citation19]. The enzymatic hydrolysis efficiency of eucalyptus pretreated by a DES of ChCl-lactic acid reached up to 94% [Citation20], and that of sugarcane with ChCl-glycerol pretreatment reached up to 95.8% [Citation21]. Moreover, the temperature and duration of pretreatment determine lignin removal and cellulose reservation of the biomass source, and most research has optimized these variables to obtain the optimal DES pretreatment performance [Citation22,Citation23]. Totals of 78% of lignin and 70% of hemicelluloses could be removed from poplar wood by ChCl-lactic acid pretreatment at 145°C for 6 h [Citation24]. DES (ChCl-glycerol) by coordinating FeCl3 was mainly attributed to 78.9% of lignin removal and 93.6% of hemicellulose dissolution of Hybrid Pennisetum under 140°C for 9 h [Citation18]. The main pretreatment methods of corncob include microwave irradiation, acid and alkali pretreatments, and thermochemical pretreatment [Citation25–27]. However, few studies focused on hydrogen production from corncob enhanced by DES pretreatment. Therefore, it is necessary to study the influence of DES pretreatment (both temperature and duration) on the composition, enzymatic hydrolysis, and hydrogen production of corncob.
Based on the above-presented considerations, the effects of DES pretreatment under different temperatures and durations on the composition, enzymatic hydrolysis, and kinetics of hydrogen production from corncob were comprehensively examined. In addition, the correlation of composition, enzymatic hydrolysis, and hydrogen yield of pretreated corncob, as well as the energy conversion efficiency of PFHP from corncob was analyzed.
2. Materials and methods
2.1. Substrate and microorganisms
Corncob, the substrate for PFHP, was collected from the experimental field of Henan Agricultural University (Zhengzhou, China). After natural air drying, corncob was crushed into powder (60-mesh) using a 2500Y (Zhejiang Yongkang Baoo Hardware Products Co., Ltd., China) grinder and this powder was stored in transparent polyethylene bags until use.
Mixed photosynthetic bacteria HAU-M1, provided by Henan Agricultural University, were used as inoculum. This mixture mainly included Rhodospirillum rubrum, Rhodopseudomonas capsulate, and Rhodopseudomonas palustris [Citation28]. Before inoculation, a mix of photosynthetic bacteria and growth medium was added to 500 mL transparent plastic bottles, and mixed photosynthetic bacteria achieved 30% (v/v). The growth medium contained 1 g/L NH4Cl, 2 g/L NaHCO3, 0.2 g/L K2HPO4, 4 g/L CH3COONa, 0.2 g/L MgSO4, and 2 g/L NaCl. The transparent plastic bottles for the bacterial culture were placed in an incubator under constant conditions (temperature of 30°C and light intensity of 3000 Lux) for 48 h. The experiments were conducted at the Key Laboratory of New Materials and Facilities for Rural Renewable Energy, Ministry of Agriculture, Henan Agricultural University, China.
2.2. DES Pretreatment of corncob
DES preparation: After vacuum drying, ChCl and ethanolamine (analytical purity 98%) were added to a 500 mL beaker at a molar ratio of 1:6; the mixture was stirred continuously at 60°C and 800 rpm until uniform and transparent DES formed [Citation13]. The prepared DES was then cooled to room temperature and stored in a vacuum dryer prior to corncob pretreatment.
Prepared DES (400 g) and corncob (20 g) were added to a conical flask at a liquid-solid ratio of 20:1 (w/w) [Citation29], then, the conical flask was placed into an electric blast drying oven (101-2A, China) to start the corncob pretreatment. When the influence of DES pretreatment temperature on the composition of corncob and PFHP was studied, the pretreatment temperature was set to 30, 50, 70, 90, and 110°C, while the pretreatment duration remained fixed at 9 h. After the optimal pretreatment temperature was identified, pretreatment duration was set to 3, 6, 9, 12, and 15 h under the optimal pretreatment temperature to study the effect of different DES pretreatment durations on the PFHP of corncob. After pretreatment, DES and corncob were separated by centrifugation (under 10,000 rpm for 5 min), and the separated corncob was repeatedly rinsed with distilled water and ethanol until the filtrate was neutral, and then dried at 50°C. The experiments were carried out in triplicate. The control group used untreated corncob as substrate.
2.3. Enzymatic hydrolysis of corncob pretreated by DES
DES-pretreated corncob was used for subsequent enzymatic saccharification and hydrogen production. Corncob powder (5 g; either pretreated or untreated) was added to a 250 mL conical flask along with 0.6 mL of cellulase (Ctec 2, enzyme activity of 51 FPU/mL; Novozymes, Bagsværd, Denmark) and 100 mL of pH 4.8 citric acid buffer. Enzymolysis experiments were conducted in an oscillator that was operated for 48 h at 50°C and 150 rpm to obtain hydrolyzate for PFHP. Each experiment was replicated three times.
2.4. PFHP using DES pretreated corncob
Hydrogen production medium (40 mL) and HAU-M1 (60 mL) were added to each hydrolyzate obtained by the enzymatic hydrolysis of corncob, and the pH value of the solution was adjusted to 7.0. Then, before reactors were sealed with rubber plugs, the top of reactors was filled with nitrogen gas to ensure an anaerobic environment for hydrogen production. Hydrogen production experiments were conducted in an incubator at a temperature of 30°C and a light intensity of 3000 Lux. The generated hydrogen was collected using gas collection bags.
2.5. Analytical methods
The cellulose, xylan, and lignin contents of DES-pretreated corncob were analyzed using the two-step acid hydrolysis method developed by the National Renewable Energy Laboratory [Citation30]. The cellulose reservation (%), xylan loss (%), and lignin removal (%) were calculated as follows:
Where CC, XC and LC is cellulose content, xylan content, and lignin content (%), respectively; RR is the residue recovery (%).
The modified Gompertz equation was used to analyze the kinetics of PFHP to predict the potential hydrogen production; at the same time, the kinetic equation of the hydrogen production rate was also be obtained, and both are presented as Eq. (4) and (5) [Citation31].
Where, H(t) is cumulative hydrogen yield (mL); Hmax is maximum potential of hydrogen production (mL); rm is maximum hydrogen production rate (mL/h); λ is the delay period (h); is hydrogen production rate (mL/h); e is Euler number (2.72), and t is fermentation time (h).
The ECE of PFHP from corncob was determined by the Eq. (6):
Where, is hydrogen yield (mL); m is the corncob weight (g);
and
are the heating value of hydrogen (12.86 J/mL) and corncob (untreated = 15690.00 J/g, pretreated = 14428.55 J/g) [Citation32].
The sugar concentration in hydrolyzate, generated by enzymatic hydrolysis of corncob was determined using a high-performance liquid chromatography (Aminex HPX-87P, Bio-Rad, Inc., USA) [Citation33]. Hydrogen content and light intensity were measured by a gas chromatograph (6820 GC-14B, Agilent Technologies, USA) and a digital photometer (TES-1330, Shanghai, China), respectively [Citation34,Citation35]. The pH value was measured using a pH meter (PHS-3E, Shanghai, China). The heating value was determined by a microcomputer calorimeter (FRL-100, Jiangsu Taizhou Shuangcheng Analytic Instrument Co., Ltd., China). Statistical analysis was carried out with SPSS software. Canonical correlation analysis was performed using the R programming language and Origin [Citation36].
3. Results and discussion
3.1. Composition of DES pretreated corncob
shows the composition of both DES-pretreated and untreated corncob. The results show that DES pretreatment had a significant effect on the composition of corncob. The recovery of corncob residues gradually decreased (from 76.02% to 56.56%) with increasing DES pretreatment temperature. A cellulose reservation of 76.67–87.01% was observed during the DES pretreatment with different temperatures (). DES pretreatment contributed to a large amount of lignin removal (47.41–95.93%) and a small amount of xylan loss (25.64–59.22%). After DES pretreatment (temperature range of 30–110°C), the cellulose content of the recovered residue increased from 32.10% (untreated) to 43.51%, the xylan content decreased from 28.54% (untreated) to 20.58%, and the lignin content decreased from 13.91% (untreated) to 1.00%. In other words, the cellulose content of pretreated corncob increased significantly, which is favorable for enzymatic hydrolysis fermentation. Simultaneously, the lowest lignin content (1.00%) was obtained at a pretreatment temperature of 110°C, which achieved the highest lignin removal (95.93%). The potential reason is that increasing temperatures lead to weaker interactions between the ChCl cation and the corresponding hydrogen bond donor in the DES system, resulting in a lower viscosity of the DES solution, thus increasing the separability of lignin [Citation37,Citation38]. Although a DES pretreatment temperature of 110°C had a significant lignin removal effect on corncob, residue recovery and cellulose reservation under this condition were minimal at 56.56% and 76.67%, respectively. When corncob was pretreated at 50°C, the lignin content and cellulose content of recovered residue were 5.07% and 38.79%, respectively. Compared with corncob pretreated at 110°C, corncob pretreated at 50°C achieved lower lignin removal and cellulose content. However, both the recovery and cellulose reservation of residues from corncob pretreated at 50°C were higher than that of corncob pretreated at 110°C. This may be because softening combined with DES during high temperature pretreatment (110°C) severely damaged the fiber structure, which resulted in a significant loss of corncob. Hence, considering factors of lignin removal, cellulose reservation, and residue recovery, a pretreatment temperature of 50°C can be considered to be the optimal pretreatment temperature for corncob. The main composition of corncob pretreated at this temperature was 38.79% of cellulose, 26.41% of xylan, and 5.07% of lignin.
Table 1. Effect of DES pretreatment temperature and duration on composition of corncob.
Pretreatment duration is another major factor affecting the DES-pretreated biomass [Citation18]. also displays the influence of different DES pretreatment durations (3–15 h) on corncob composition with a fixed temperature of 50°C. With increasing pretreatment duration, lignin removal of corncob increased from 53.95% to 82.83% (p < 0.05), confirming that the pretreatment duration was positively correlated with the dissolution of polymer molecules (lignin) [Citation39]. The anions and ChCl cations of the DES system formed hydrogen bond complexes with hydrogen and oxygen atoms in the hydroxyl group, which promote the ionization of the phenolic group of lignin and lead to the dissolution of lignin in aqueous solution [Citation19]. Xylan loss also increased from 22.37% to 50.59%. Moreover, cellulose reservation maintained a relatively high range of 82.58–89.17% (p < 0.05) (). The above results can be explained by the fact that the lengthening of pretreatment duration allowed DES to fully react with the substrate, leading to the destruction of ether bonds in lignin and the rupture of ester bonds between lignin and hemicellulose [Citation40]. However, compared to untreated corncob, the composition of pretreated corncob residues changed greatly after DES pretreatment, as the cellulose content of corncob pretreated for 15 h increased by 34.52% and the lignin content decreased by 73.83%. These results indicate that DES pretreatment could effectively dissolve lignin in corncob. After DES-pretreatment of corncob for 12 h, its composition changed and a cellulose content of 40.74%, a xylan content of 22.66%, and a lignin content of 4.13% were obtained (). As the main factors determining the enzymatic hydrolysis effect of biomass, lignin and cellulose contents of corncob pretreated for 12 h and 15 h were not significantly different (differences of 0.49% and 2.44%). Therefore, from the perspective of energy consumption, the most appropriate DES pretreatment duration for corncob was 12 h. Comparison of the composition of corncob change showed that more liberated electrons with stretching groups can attack and interact with corncob components in the DES pretreatment system [Citation19]. This enhances the mass transfer between DES and corncob, thus promoting pretreatment efficiency.
3.2. Enzymolysis ability of pretreated corncob
The PFHP process of biomass includes biomass pretreatment, enzymatic hydrolysis, and hydrogen generation, during which pretreatment provides the precondition for hydrogen production, and enzymatic saccharification links pretreatment and hydrogen productivity. The factors affecting the enzymatic hydrolysis efficiency are the type of the raw material, the methods and conditions of pretreatment, the temperature and time of enzymatic hydrolysis, and the solid: liquid ratio (raw material: enzymolysis solution) [Citation29,Citation41]. Pretreatment methods and conditions exert a stronger influence on the enzymatic hydrolysis for the same raw material. The sugar yield of corncob with different DES pretreatment methods during the enzymatic hydrolysis is presented in . The reducing sugar concentration obtained from DES-pretreated corncob exceeded 33.06 g/L under different pretreatment temperatures, which was much higher than that of untreated corncob (7.92 g/L). The highest total reducing sugar concentration of 46.80 g/L was found at a pretreatment temperature of 50°C, which represented an increase of 5.91 times compared to untreated corncob. This was because the pretreatment effectively removed lignin and increased the accessibility of enzymes to cellulose and hemicellulose, thereby promoting the release of reducing sugar [Citation30]. As the main products of the enzymatic hydrolysis of corncob, glucose and xylose concentrations increased to 27.30 g/L and 19.50 g/L, respectively, as the pretreatment temperature increased from 30°C to 50°C. However, as the pretreatment temperature further increased from 50°C to 110°C, the concentrations of both glucose and xylose decreased. This result was consistent with the result reported by Procentese et al., where the increased removal of xylan at higher pretreatment temperature resulted in lower concentrations of glucose and xylose [Citation10]. These results showed that the lignin removal rate increased with increasing pretreatment temperature, while glucose and xylose concentrations tended to increase and then decrease with increasing pretreatment temperature. This result indicated that an appropriate increase of the pretreatment temperature effectively improved the enzymatic hydrolysis of corncob while also removing lignin.
The results of the influence of DES pretreatments using different durations on enzymatic hydrolysis are shown in . The pretreatment temperature was set to 50°C. The total reducing sugar concentration of pretreated corncob increased from 34.35 g/L (3 h) to 49.83 g/L (12 h) with prolonged pretreatment duration. This was likely because the longer the pretreatment duration, the larger the surface area of cellulose, which promoted the hydrolysis of cellulose to glucose and increased the total concentration of reducing sugar. The maximum reducing sugar concentration of 49.83 g/L was achieved at a pretreatment duration of 12 h, which was 6.29 times higher than that of untreated corncob. Moreover, the 49.83 g/L (996.60 mg/g) of reducing sugar from DES-pretreated corncob was higher than that obtained from corncob with ultrafine grinding pretreatment (752.22 mg/g) for 2 h and a ball: powder weight of 20:1 [Citation42]. The total reducing sugar concentration of corncob pretreated for 15 h was 49.39 g/L, which was only 0.44 g/L less than that of corncob pretreated for 12 h. The reason may be that cellulose reservation and lignin removal were almost the same when corncob was pretreated for 12 h and 15 h (); therefore, the upper limit of cellulase adsorption sites was reached and the reaction tended to be stable in both cases. Compared with aqueous ammonia pretreatment at 71.3°C for 8.8 h with a corncob loading of 20.0% (reducing sugar of 822.31 mg/g) [Citation43], acid steam-explosion pretreatment (0.5% H2SO4) for corncob exposed to 1.21 MPa steam for 20 min (reducing sugar of 720 mg/g), and alkali soaking pretreatment (0.5% NaOH soaked for 1 h) (reducing sugar of 670 mg/g) [Citation44], DES pretreatment of corncob was more effective. Glucose and xylose, from both cellulose and hemicellulose, respectively, were released in the biomass by enzymatic hydrolysis [Citation45]. In the process of enzymatic saccharification of corncob, by extending the pretreatment duration from 3 h to 12 h, the glucose concentration increased from 22.15 g/L to 31.25 g/L, and the xylose concentration increased from 12.20 g/L to 19.36 g/L. The above results showed that corncob pretreatment increased the cellulose content because of lignin dissolution; cellulose was decomposed into glucose by cellulase, which improved the enzymatic degradation of corncob.
3.3. Effects of DES pretreatment on PFHP from corncob
DES-pretreated corncob was enzymolized and converted into available reducing sugar (glucose, C6H12O6), which was then decomposed by photosynthetic bacteria to produce hydrogen. PFHP by HAU-M1 is a complex biochemical metabolic activity. The hydrogen yield is closely related to the metabolic pathway of photosynthetic bacteria. Hydrogen is mainly generated from metabolic pathways of acetic acid (C2H4O2) and butyric acid (C4H8O2) (see Eqs. (7) and (8)). Acetic acid and butyric acid produced by the bacterial metabolism can also be used to produce hydrogen under substrate insufficiency (Eqs. (9) and (10)). In terms of Gibbs free energy, butyric acid type (ΔG0 = −254 KJ) was more favorable than acetic acid type (ΔG0 = −206 KJ) for the hydrogen production metabolic pathway. Therefore, under the same fermentation conditions, hydrogen production by a butyric acid type metabolism is more likely fermenting glucose into hydrogen and acid.
The hydrogen production data of pretreated and untreated corncob were fitted by a modified Gompertz model. As shown in , the cumulative hydrogen yields of corncob pretreated under different temperatures and durations were higher than that of the control group (248.62 mL). At a pretreatment temperature of 50°C, the cumulative hydrogen yield was 558.51 mL, which was 2.25 times higher than that of untreated corncob. However, when the pretreatment temperature increased further, the cumulative hydrogen yield decreased gradually.
The variation law of the hydrogen production rate of pretreated corncob was consistent, showing a first increasing and then a decreasing trend, and the hydrogen production rate at a DES pretreatment temperature of 50°C was as high as 9.71 mL/h (). These results were consistent with the change of reducing sugar concentration shown in , as the decrease in reducing sugar concentration at higher temperatures led to a reduction of available substrates for photosynthetic bacteria, which reduced the hydrogen yield. When the pretreatment temperature was fixed at 50°C, the cumulative hydrogen yield increased gradually with the prolongation of the pretreatment duration from 3 h to 12 h. However, the cumulative hydrogen yield of corncob pretreated for 15 h was 42.15 mL lower than that of corncob pretreated for 12 h. The maximum cumulative hydrogen yield was 677.45 mL (136 mL/g), obtained at a pretreatment duration of 12 h, which represents an increase of 2.72 times compared to untreated corncob. With increasing pretreatment duration from 3 h to 12 h, the lignin removal and the enzymatic hydrolysis efficiency increased significantly, thereby enhancing the hydrogen production from corncob [Citation28]. Therefore, an appropriate increase in DES pretreatment temperature and pretreatment duration (50°C and 12 h, respectively) can increase the cellulose content through lignin removal and promote enzymatic digestion of corncob. Thus, these pretreatment conditions increased the hydrogen production rate from corncob photo-fermentation.
The kinetic parameters of the modified Gompertz model that were obtained by fitting the hydrogen production data are shown in . The R2 of each condition was greater than 0.99, indicating that this model achieved a good fit of the experimental data. The maximum hydrogen production potential of 715.08 mL (143 mL/g) was obtained under the pretreatment condition of 50°C and 12 h, and the corresponding hydrogen production rate was 10.52 mL/h ( and ). Moreover, biohydrogen production from various sources was compared, and the results are shown in . Hydrogen yield from DES-pretreated corncob was much higher than that of giant reed pretreated by ionic liquids [Citation39], sugarcane bagasse pretreated by hydrochloric acid [Citation50], wheat straw pretreated by hydrothermal alkali [Citation49], and corncob pretreated by acid steam-explosion [Citation44,Citation46–48]. The hydrogen yields of corn stalk pretreated by 2% NaOH [Citation5] and corncob pretreated thermochemically by 2% H2SO4 [Citation25] were similar to the yields obtained in the present study. However, the best hydrogen yield from corncob was achieved with ultrafine grinding pretreatment [Citation42] was best. The delay period of hydrogen production reflected the efficiency of the PFHP system [Citation51].
Table 2. Kinetic parameters of hydrogen production by modified Gompertz model.
Table 3. Comparison of biohydrogen production under different pretreatment methods.
The pretreatment time was set to 9 h when studying the effect of DES pretreatment temperature on hydrogen production results, while the pretreatment temperature was set to 50°C when studying the effect of pretreatment time on hydrogen production results.
Compared with untreated corncob (delay period of 33.30 h), the pretreatment conditions shortened the hydrogen production delay period of corncob significantly, and the maximum shortening time was 18.90 h (the shortest delay period of 14.40 h). The reason may be that after DES pretreatment, corncob had an increases cellulose content; thus, the large amount of available reducing sugars obtained during enzymatic hydrolysis promoted the hydrogen production by photosynthetic bacteria [Citation45].
3.4. Correlation analysis
The correlation between features including composition (contents of cellulose, xylan, and lignin), enzymolysis ability (concentrations of reducing sugar, glucose, and xylose), and hydrogen production (hydrogen yield) of DES-pretreated corncob was analyzed using a scatter matrix (). The cellulose content of DES-pretreated corncob was positively related with reducing sugar concentration, glucose concentration obtained from enzymatic hydrolysis, and hydrogen yield (Pearson’s r values were positive) (). At a DES pretreatment temperature of 50°C and a varying pretreatment duration from 3 to 15 h, the cellulose content of pretreated corncob had the maximum effect on hydrogen production (Pearson’s r = 0.97; ). This result confirmed that the cellulose reservation during DES pretreatment process had a significant impact on the behavior of PFHP. However, the lignin content of pretreated corncob was negatively correlated with the concentrations of reducing sugar and glucose as well as with hydrogen production (Pearson’s r < 0; ). In addition, a complete negative correlation was found between reducing sugar concentration and lignin content of pretreated corncob (Pearson’s r = −1), indicating that lignin in corncob did not contribute to the enzymatic saccharification for hydrogen production.
Figure 3. Correlation analysis of corncob composition, enzymatic hydrolysis and hydrogen production.

Red color indicates a negative correlation between reducing sugar concentration and lignin content of pretreated corn kernels, green color indicates a positive correlation between cellulose content and reducing sugar concentration, glucose concentration and hydrogen production of pretreated corn kernels, while the darker color indicates a stronger correlation.
3.5. Energy conversion efficiency of PFHP
The energy conversion efficiency of DES-pretreated corncob in the PFHP process was calculated according to EquationEq. (6)(6)
(6) . The energy conversion efficiency of pretreated corncob increased from 4.75% (30°C) to 9.96% (50°C), and then gradually decreased with further increasing pretreatment temperature (). Moreover, the energy conversion efficiency of corncob increased when DES pretreatment duration was varied from 3 h to 12 h, and then decreased when the pretreatment duration was further extended to 15 h (). The significant variation was caused by the comprehensive effect of DES pretreatment temperature and duration on enzymatic hydrolysis and hydrogen production. Corncob pretreated at 50°C for 12 h had the best enzymatic hydrolysis effect and hydrogen production yield. The highest energy conversion efficiency of 12.08% was also obtained from corncob pretreated at 50°C for 12 h (), and this efficiency was 1.96 times higher than that of untreated corncob (4.08%). The energy conversion efficiency was also higher than the values of 10.12% and 6.45% obtained in a prior study, where corn stover was ground by a superfine pulverizer and taken as feed stock for biohydrogen production by both photo-fermentation and dark-photo co-fermentation [Citation52].
DES mainly used for applications in metal finishing, pulping, alkaloid purification, nanomaterial preparation, and CO2 capture from the air. In recent years, DES has emerged as a lignocellulosic pretreatment method. The limited research has highlighted the outstanding dissolution ability of DES for lignin and has proved the potential of DES pretreatment for biohydrogen production. However, DES pretreatment of lignocellulose biomass was conducted in small-scale batches, and only a narrow range of DES has been utilized (including in this paper). Thus far, the detailed mechanism of action of DES on lignin remains unclear, and the loss of xylan during the pretreatment process lowered the hydrogen production. Therefore, it is worthwhile to further study the use of DES for the conversion of lignocellulose, including the expansion of DES types, application of continuous fermentation, DES recycling, process integration, and techno-economical assessment.
4. Conclusions
DES pretreatment can effectively dissolve lignin and enhance enzymatic saccharification, thereby improving the hydrogen production from corncob. The maximum cumulative hydrogen yield of 677.45 mL was obtained at a pretreatment condition of 50°C for 12 h, and this yield was 2.72 times higher than that of untreated corncob; the corresponding lignin removal and cellulose reservation rates were 79.15% and 89.17%, respectively. The cellulose content was positively correlated with reducing sugar concentration and hydrogen yield, and the highest energy conversion efficiency in the hydrogen production process was 12.08%. This study shows that DES pretreatment is an effective method for promoting hydrogen production from corncob.
Disclosure statement
No potential conflict of interest was reported by the author(s).
Data availability statement
The data that support the findings of this study are available on request from the corresponding author, upon reasonable request.
Additional information
Funding
References
- Xing XK, Yan YM, Zhang HR, et al. Optimal design of distributed energy systems for industrial parks under gas shortage based on augmented epsilon-constraint method. J Clean Prod. 2019;218:782–481. doi: 10.1016/j.jclepro.2019.02.052
- Zhang T, Jiang DP, Li YM, et al. Study of the interrelationship between nano-TiO2 addition and photo-fermentative bio-hydrogen production of corn straw. Biores Technol. 2021;338:338. doi: 10.1016/j.biortech.2021.125549
- Androga DD, Ozgur E, Eroglu I, et al. Significance of carbon to nitrogen ratio on the long-term stability of continuous photofermentative hydrogen production. Int J Hydrogen Energy. 2011;36(24):15583–15594. doi: 10.1016/j.ijhydene.2011.09.043
- Lu CY, Li WZ, Zhang QG, et al. Enhancing photo-fermentation biohydrogen production by strengthening the beneficial metabolic products with catalysts. J Clean Prod. 2021;317:317. doi: 10.1016/j.jclepro.2021.128437
- Zhang T, Jiang DP, Zhang H, et al. Effects of different pretreatment methods on the structural characteristics, enzymatic saccharification and photo-fermentative bio-hydrogen production performance of corn straw. Biores Technol. 2020;304:304. doi: 10.1016/j.biortech.2020.122999
- Soccol CR, Vandenberghe LPD, Medeiros ABP, et al. Bioethanol from lignocelluloses: Status and perspectives in Brazil. Biores Technol. 2010;101(13):4820–4825. doi: 10.1016/j.biortech.2009.11.067
- Sun D, Lv ZW, Rao J, et al. Effects of hydrothermal pretreatment on the dissolution and structural evolution of hemicelluloses and lignin: A review. Carbohydr Polym. 2022;281:281. doi: 10.1016/j.carbpol.2021.119050
- El-Hallag I, Elsharkawy S, Hammad S. Electrodeposition of Ni nanoparticles from deep eutectic solvent and aqueous solution as electrocatalyst for methanol oxidation in acidic media. Int J Hydrogen Energy. 2021;46(29):15442–15453. doi: 10.1016/j.ijhydene.2021.02.049
- Protsenko VS, Bogdanov DA, Korniy SA, et al. Application of a deep eutectic solvent to prepare nanocrystalline Ni and Ni/TiO2 coatings as electrocatalysts for the hydrogen evolution reaction. Int J Hydrogen Energy. 2019;44(45):24604–24616. doi: 10.1016/j.ijhydene.2019.07.188
- Procentese A, Johnson E, Orr V, et al. Deep eutectic solvent pretreatment and subsequent saccharification of corncob. Biores Technol. 2015;192:31–36. doi: 10.1016/j.biortech.2015.05.053
- Vijayakumar J, Mohan S, Kumar SA, et al. Electrodeposition of Ni-Co-Sn alloy from choline chloride-based deep eutectic solvent and characterization as cathode for hydrogen evolution in alkaline solution. Int J Hydrogen Energy. 2013;38:10208–10214. doi: 10.1016/j.ijhydene.2013.06.068
- Hong S, Yuan Y, Liu CZ, et al. A stretchable and compressible ion gel based on a deep eutectic solvent applied as a strain sensor and electrolyte for supercapacitors. J Mater Chem C. 2020;8(2):550–560. doi: 10.1039/c9tc05913j
- Mjalli FS, Murshid G, Al-Zakwani S, et al. Monoethanolamine-based deep eutectic solvents, their synthesis and characterization. Fluid Phase Equilibria. 2017;448:30–40. doi: 10.1016/j.fluid.2017.03.008
- Sarmad S, Xie YJ, Mikkola JP, et al. Screening of deep eutectic solvents (DESs) as green CO2 sorbents: from solubility to viscosity. New J Chem. 2017;41(1):290–301. doi: 10.1039/c6nj03140d
- Francisco M, van den Bruinhorst A, Kroon MC. New natural and renewable low transition temperature mixtures (LTTMs): screening as solvents for lignocellulosic biomass processing. Green Chem. 2012;14(8):2153–2157. doi: 10.1039/c2gc35660k
- Chen Z, Reznicek WD, Wan CX. Deep eutectic solvent pretreatment enabling full utilization of switchgrass. Biores Technol. 2018;263:40–48. doi: 10.1016/j.biortech.2018.04.058
- Kumar AK, Parikh BS, Pravakar M. Natural deep eutectic solvent mediated pretreatment of rice straw: bioanalytical characterization of lignin extract and enzymatic hydrolysis of pretreated biomass residue. Environ Sci Pollut Res. 2016;23(10):9265–9275. doi: 10.1007/s11356-015-4780-4
- Wang ZK, Li HY, Lin XC, et al. Novel recyclable deep eutectic solvent boost biomass pretreatment for enzymatic hydrolysis. Biores Technol. 2020;307:307. doi: 10.1016/j.biortech.2020.123237
- Tan YT, Ngoh GC, Chua ASM. Evaluation of fractionation and delignification efficiencies of deep eutectic solvents on oil palm empty fruit bunch. Ind Crop Prod. 2018;123:271–277. doi: 10.1016/j.indcrop.2018.06.091
- Shen XJ, Wen JL, Mei QQ, et al. Facile fractionation of lignocelluloses by biomass-derived deep eutectic solvent (DES) pretreatment for cellulose enzymatic hydrolysis and lignin valorization. Green Chem. 2019;21(2):275–283. doi: 10.1039/c8gc03064b
- Chourasia VR, Pandey A, Pant KK, et al. Improving enzymatic digestibility of sugarcane bagasse from different varieties of sugarcane using deep eutectic solvent pretreatment. Biores Technol. 2021;337:337. doi: 10.1016/j.biortech.2021.125480
- Guo ZW, Zhang QL, You TT, et al. Short-time deep eutectic solvent pretreatment for enhanced enzymatic saccharification and lignin valorization. Green Chem. 2019;21(11):3099–3108. doi: 10.1039/c9gc00704k
- Yang JY, Zhang WJ, Wang Y, et al. Novel, recyclable Bronsted acidic deep eutectic solvent for mild fractionation of hemicelluloses. Carbohydr Polym. 2022;278. doi: 10.1016/j.carbpol.2021.118992
- Alvarez-Vasco C, Ma RS, Quintero M, et al. Unique low-molecular-weight lignin with high purity extracted from wood by deep eutectic solvents (DES): a source of lignin for valorization. Green Chem. 2016;18(19):5133–5141. doi: 10.1039/c6gc01007e
- Medina-Morales MA, De la Cruz-Andrade LE, Paredes-Peña LA, et al. Biohydrogen production from thermochemically pretreated corncob using a mixed culture bioaugmented with Clostridium acetobutylicum. Int J Hydrogen Energy. 2021;46(51):25974–25984. doi: 10.1016/j.ijhydene.2021.04.046
- Cai X, Hu CH, Wang J, et al. Efficient high-solids enzymatic hydrolysis of corncobs by an acidic pretreatment and a fed-batch feeding mode. Biores Technol. 2021;326:326. doi: 10.1016/j.biortech.2021.124768
- Zhang ZP, Fan XN, Li YM, et al. Photo-fermentative biohydrogen production from corncob treated by microwave irradiation. Biores Technol. 2021;340:340. doi: 10.1016/j.biortech.2021.125460
- Jing Y, Li F, Li Y, et al. Biohydrogen production by deep eutectic solvent delignification-driven enzymatic hydrolysis and photo-fermentation: Effect of liquid-solid ratio. Bioresour Technol. 2022;349:126867. doi: 10.1016/j.biortech.2022.126867
- Hou XD, Li AL, Lin KP, et al. Insight into the structure-function relationships of deep eutectic solvents during rice straw pretreatment. Biores Technol. 2018;249:261–267. doi: 10.1016/j.biortech.2017.10.019
- Sluiter A, Hames B, Ruiz R, et al. Determination of structural carbohydrates and lignin in biomass. Lab Anal Proced. 2008;1617:1–16.
- Zhu SN, Zhang ZP, Zhang H, et al. Rheological properties of corn stover hydrolysate and photo-fermentation bio-hydrogen producing capacity under intermittent stirring. Int J Hydrogen Energy. 2020;45(6):3721–3728. doi: 10.1016/j.ijhydene.2019.08.069
- Zhang XT, Jiang DP, Zhang H, et al. Enhancement of the biohydrogen production performance from mixed substrate by photo-fermentation: Effects of initial pH and inoculation volume ratio. Biores Technol. 2021;319:319. doi: 10.1016/j.biortech.2020.124153
- Jiang DP, Ge XM, Zhang T, et al. Effect of alkaline pretreatment on photo-fermentative hydrogen production from giant reed: Comparison of NaOH and Ca(OH)(2). Biores Technol. 2020;33:304.
- Jiang DP, Ge XM, Zhang T, et al. Photo-fermentative hydrogen production from enzymatic hydrolysate of corn stalk pith with a photosynthetic consortium. Int J Hydrogen Energy. 2016;41(38):16778–16785. doi: 10.1016/j.ijhydene.2016.07.129
- Lu CY, Zhang ZP, Ge XM, et al. Bio-hydrogen production from apple waste by photosynthetic bacteria HAU-M1. Int J Hydrogen Energy. 2016;41(31):13399–13407. doi: 10.1016/j.ijhydene.2016.06.101
- Jiang DP, Zhang XT, Jing YY, et al. Towards high light conversion efficiency from photo-fermentative hydrogen production of Arundo donax L. by light–dark duration alternation strategy. Biores Technol. 2022;344:344. doi: 10.1016/j.biortech.2021.126302
- Cheng J, Su HB, Zhou JH, et al. Microwave-assisted alkali pretreatment of rice straw to promote enzymatic hydrolysis and hydrogen production in dark- and photo-fermentation. Int J Hydrogen Energy. 2011;36(3):2093–2101. doi: 10.1016/j.ijhydene.2010.11.021
- Hou XD, Li N, Zong MH. Significantly enhancing enzymatic hydrolysis of rice straw after pretreatment using renewable ionic liquid-water mixtures. Biores Technol. 2013;136:469–474. doi: 10.1016/j.biortech.2013.02.118
- Chen Z, Jiang DP, Zhang T, et al. Comparison of three ionic liquids pretreatment of Arundo donax L. for enhanced photo-fermentative hydrogen production. Biores Technol. 2022;343:343. doi: 10.1016/j.biortech.2021.126088
- Li AL, Hou XD, Lin KP, et al. Rice straw pretreatment using deep eutectic solvents with different constituents molar ratios: Biomass fractionation, polysaccharides enzymatic digestion and solvent reuse. J Biosci Bioeng. 2018;126(3):346–354. doi: 10.1016/j.jbiosc.2018.03.011
- Zhang Y, Zhang H, Lee DJ, et al. Effect of enzymolysis time on biohydrogen production from photo-fermentation by using various energy grasses as substrates. Biores Technol. 2020;305:305. doi: 10.1016/j.biortech.2020.123062
- Li YM, Zhang ZP, Jing YY, et al. Forecasting of reducing sugar yield from corncob after ultrafine grinding pretreatment based on GM(1,N) method and evaluation of biohydrogen production potential. Biores Technol. 2022;348:348. doi: 10.1016/j.biortech.2022.126836
- Zhang H, Wu JH. Statistical optimization of aqueous ammonia pretreatment and enzymatic hydrolysis of corn cob powder for enhancing sugars production. Biochem Eng J. 2021;174:174. doi: 10.1016/j.bej.2021.108106
- XX T, NQ R, JF X. Evaluation of hydrogen production from corn cob with the mesophilic bacterium clostridium hydrogeniproducens HR-1. Int J Hydrogen Energy. 2013;38(22):9104–9110. doi: 10.1016/j.ijhydene.2013.05.066
- Wang JL, Yin YA. Fermentative hydrogen production using various biomass-based materials as feedstock. Renew Sust Energ Rev. 2018;92:284–306. doi: 10.1016/j.rser.2018.04.033
- Li YM, Fan XN, Zhang HR, et al. Pretreatment of corn stover by torrefaction for improving reducing sugar and biohydrogen production. Biores Technol. 2022;351:351. doi: 10.1016/j.biortech.2022.126905
- Asadi N, Zilouei H. Optimization of organosolv pretreatment of rice straw for enhanced biohydrogen production using enterobacter aerogenes. Biores Technol. 2017;227:335–344. doi: 10.1016/j.biortech.2016.12.073
- Dong LL, Cao GL, Zhao L, et al. Alkali/Urea pretreatment of rice straw at low temperature for enhanced biological hydrogen production. Biores Technol. 2018;267:71–76. doi: 10.1016/j.biortech.2018.05.055
- Zhu J, Song W, Chen X, et al. Integrated process to produce biohydrogen from wheat straw by enzymatic saccharification and dark fermentation. Int J Hydrogen Energy. 2023;48(30):11153–11161. doi: 10.1016/j.ijhydene.2022.05.056
- Lorencini P, Siqueira MR, Maniglia BC, et al. Biohydrogen production from liquid and solid fractions of sugarcane bagasse After optimized pretreatment with hydrochloric acid. Waste Biomass Valorization. 2016;7(5):1017–1029. doi: 10.1007/s12649-016-9494-4
- Lu CY, Jing YY, Zhang H, et al. Biohydrogen production through active saccharification and photo-fermentation from alfalfa. Biores Technol. 2020;304:304. doi: 10.1016/j.biortech.2020.123007
- Zhang T, Jiang DP, Zhang H, et al. Comparative study on bio-hydrogen production from corn stover: Photo-fermentation, dark-fermentation and dark-photo co-fermentation. Int J Hydrogen Energy. 2020;45(6):3807–3814. doi: 10.1016/j.ijhydene.2019.04.170