ABSTRACT
The presence of pharmaceutical compounds in wastewater due to an increase in industrialization and urbanization is a serious health concern. The demand for diverse types of pharmaceutical compounds is expected to grow as there is continuous improvement in the global human health standards. Discharge of domestic pharmaceutical personal care products and hospital waste has aggravated the burden on wastewater management. Further, the pharmaceutical water is toxic not only to the aquatic organism but also to terrestrial animals coming in contact directly or indirectly. The pharmaceutical wastes can be removed by adsorption and/or degradation approach. Nanoparticles (NPs), such as 2D layers materials, metal-organic frameworks (MOFs), and carbonaceous nanomaterials are proven to be more efficient for adsorption and/or degradation of pharmaceutical waste. In addition, inclusion of NPs to form various composites leads to improvement in the waste treatment efficacy to a greater extent. Overall, carbonaceous nanocomposites have advantage in the form of being produced from renewable resources and the nanocomposite material is biodegradable either completely or to a great extent. A comprehensive literature survey on the recent advancement of pharmaceutical wastewater is the focus of the present article.
GRAPHICAL ABSTRACT
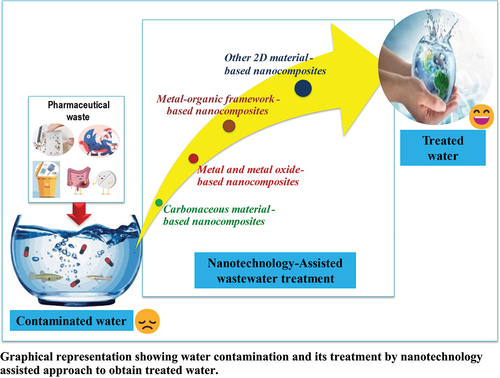
1. Introduction
The demand for food, and medicines is continuously increasing due to rapid increase in the global population [Citation1]. Every country is promoting industrialization and focusing on increased production to deal with this problem. It is polluting the air, water, soil, and food items thus affecting human health. Despite the benefits of use of textile, paper pulp, paint, and plastic materials for essential human needs, it causes water contamination through discharge of volatile organic compounds and or other pollutants during dyeing, finishing of products, and paper board formation [Citation2]. Pharma industry is helpful to cure diseases that save lives, but ultimately it also leads to environmental contamination [Citation3]. With an increase in the rate of drugs production, pharmaceutical waste contaminants are also increasing day-by-day. Pharmaceutical pollution is majorly caused by the release of pharmaceutical drugs and their metabolites in water bodies. Pharmaceutical contaminant is a class of biomedical waste that include discarded medicines, unused creams, drug containing animal waste from hospitals, and other drugs [Citation4]. Furthermore, in the last few years, antibiotics, anti-depressants, and anti-hypertensive drugs have been extensively used around the world due to the high prevalence rate of antiviral diseases [Citation5]. Our body uses only 20–30% of the drug; the rest is excreted through urine and contaminates the environment [Citation6]. Recently, it has been reported that the Cauvery River and its tributaries in India were contaminated with anti-hypertensives, anti-inflammatory, antibiotics, stimulants, and antidepressant drugs [Citation7,Citation8]. In this context, the drug contaminants recovered from water bodies were diclofenac, ibuprofen, isoprenaline, caffeine, carbamazepine, cetirizine, and ciprofloxacin. In addition, heavy metal ions, pesticides, plastics materials, and personal care products, were also found to be major water pollutants and their concentration in drinking water resources is increasing continuously [Citation9]. Different conventional methods such as coagulation, reverse osmosis, filtration, evaporation, and sedimentation are used to treat water contaminated with drugs. However, these methods are unable to eradicate them completely and have low sustainability, long processing time, and high operational cost. Various researchers try to explore nanotechnology to ensure the complete removal of pharmaceutical contaminants from wastewater [Citation10]. Nano-sorbents, nano-photocatalysts, and nano-sensors have been extensively used for the detection and removal of pharmaceutical contaminations [Citation11]. Nanocomposites such as two-dimensional (2D) layer nanomaterials; metal chalcogenides, graphene nanosheets, boron nitride, graphitic carbon nitride g-C3N4, metal oxide nanorods [Citation12], nanoflowers [Citation13], and nano-leaves [Citation14] have successfully been used for the adsorption of pharmaceutical pollutants [Citation15]. The adsorption capacity of nano-adsorbents depends upon their surface area, the charge on the surface, porous nature, and shape of the nanomaterials [Citation16]. Nano-photocatalysts use semiconductor nanomaterial for the UV or visible light mediated degradation of pharmaceuticals in contaminated water. Recently, the Z-scheme and dual z scheme are the most advanced photocatalytic techniques that are being explored for water treatment [Citation17]. The benefits of using Z-scheme photocatalysts such as low electron-hole recombination rate, complete degradation, cost-effective, and visible-light driven photocatalytic response makes them more efficient as compared to conventional photocatalyst. Bismuth-based BiOX (where X=Cl, Br, I), BiVO4, Bi2S3, Bi2O3, Bi2Se3, Bi2WO6/CeO2, Fe3O4/CeO2/BiOI, and BiOCl@CeO2 have been used for the degradation of various types of antibiotics. The advantage of using bismuth and graphitic carbon nitride (Energy band gap = 2.7) eV in photo-heterostructures is their visible light-tuneable energy bandgap, low toxicity, cost-effectiveness, and high storage stability. Various types of graphene-based composites have been used for the treatment of antibiotic contaminant water. TiO2–ZnO/CS–Gr nanocomposite has been used to achieve the light-mediated degradation of tetracycline [Citation6,Citation18,Citation19].
Nanocomposites have earlier been reported for the removal of more than one type of pharmaceutical molecules from wastewater. In such a study, maltodextrin/reduced graphene and maltodextrin/reduced graphene/copper oxide have been used for the adsorption-based removal of amoxicillin antibiotic as well as the anti-inflammatory and painkiller drug diclofenac [Citation20]. The degradation of pharmaceutical contaminants has also been achieved at large volume in a reactor using α-Fe2O3-TiO2 nanocomposite on exposure to sunlight [Citation21]. ZnFe2O4@UiO-66 nanocomposite has been used for the photo-catalytic degradation of metronidazole antibiotic [Citation22]. The nanocomposites with magnetic properties make the separation and reusability of photo catalysts and adsorbents easy. Various types of biopolymer-based composites have been used for the treatment of pharmaceutical waste as discussed in detail elsewhere [Citation23]. In this review, we have discussed treatments of pharmaceutical pollutants using degradation or adsorption techniques. We summarized the photocatalytic and adsorption characteristics, synthesis strategies and variation parameters of carbonaceous nanomaterials, metal organic frameworks (MOF’s) and 2D layer nanomaterials. Recently discovered carbonaceous nanomaterials such as carbon nanotubes, activated carbon, carbon quantum dots, graphene oxide and graphitic carbon nitride have been increasingly used for wastewater treatment due to their high thermal stability, low moisture sensitivity and highly tuneable surface properties [Citation12,Citation13]. Similarly, 2D layer nanomaterials (metal chalcogenides, graphene nanosheets, boron nitride, graphitic carbon nitride g-C3N4, metal oxide nanorods, nanoflowers, and nano-leaves) have been reviewed which are widely used in the manufacturing of visible light-assisted photocatalyst and nano adsorbents due to its high carrier mobilities, good thermal conductivity, mechanical flexibility, and high visible light absorption capacity. The novelty of this work is focused on the comparative discussion about the photocatalytic and adsorption capacity of the three most explored carbonaceous nanomaterials, metal organic frameworks (MOF’s) and 2D layer nanomaterials. It also highlights all the difficulties in handling, creating, and implementing nanocarriers for contaminated water treatment. This review article provides crucial information to address the pressing issue of pharmaceutical contamination.
2. Carbonaceous material-based nanocomposite for treatment of pharmaceutical wastewater
2.1. Carbonaceous material-based photocatalysts
Recently, attention has been focused on employing nanotechnology-based employing carbon nanocomposites because of low fabrication cost, high surface area, and environmental stability [Citation24–26]. Due to their alluring benefits, including their customizable electronic structure, high stability, and ease of synthesis, functionalized carbonaceous nanocomposites have many applications including the treatment of pollutants. Among these carbonaceous nanocomposites, 2D graphite carbon nitride (g-C3N4) is studied widely due to suitable band energy, better stability, and high surface area [Citation27–29]. Poor absorption of light, faster recombination of electron-hole pairs generated on exposure to light, and poor electric conductivity restricts the activity of g-C3N4 [Citation30]. It has been documented that the functionalization of graphite carbon nitride with other substances can enhance the capabilities of g-C3N4 for the treatment of pharmaceutical waste through better photocatalytic activity [Citation31]. Zhang et al. reported the synthesis of AgI/g-C3N4 composite for diclofenac treatment [Citation32]. The degradation of the composite was 43.2 and 12.5 times higher than pure g-C3N4 and AgI NPs, respectively.
Jiang et al. have developed a Z-scheme photocatalyst (NiCo2O4/g-C3N4-Nvac) that uses nickel cobaltate NPs embedded in nitrogen-defects containing graphitic carbon nitride nanosheets. The defects were responsible for the activation of key species peroxymonosulfate, •SO4− based oxidation and Z-scheme-charge-transfer thus leading to better tetracycline degradation in case of the composite than NiCo2O4/g-C3N4 and g-C3N4 [Citation33]. Likewise, Mo6+/Mo5+ oxidation-reductioncycle and PMS activation mediated degradation of tetracycline hydrochloride have been reported using MoO3/Bi2O3/g-C3N4 composites [Citation34].
Guo et al. [Citation35] reported the degradation of sulfamethoxazole using g-C3N4@PDA/BiOBr photocatalyst. The composite caused complete sulfamethoxazole degradation while other photocatalysts have shown lower photocatalytic abilities in order: g-C3N4/BiOBr (71%) > g-C3N4@ PDA + BiOBr (47%) > BiOBr (42%) > g-C3N4 (30%). The increased efficiency of the composite was attributed to the better electron transfer ability of polydopamine between g-C3N4 and BiOBr. It was established that h+ and •O2− were the main reactive species responsible for oxidizing sulfmethoxazole.
Quaternary nanohybrid photocatalyst of composition g-C3N4/NiO/ZnO/Fe3O4 has been used for the degradation of esomeprazole [Citation36]. Esomeprazole degrades up to 95.05 ± 1.72% which is related to the synergistic effects of the various moieties. Improved charge separation, increased production of hydroxyl free radicals from H2O2, as well as a Fenton-related process, were mainly responsible for the degradation of esomeprazole. Zhang et al. prepared a Fe3O4/CdS/g-C3N4 photocatalyst using the mono-dispersion approach with 81% degradation efficiency for ciprofloxacin [Citation37]. The separation of photogenerated carriers in photocatalytic reactions is promoted by efficient inhibition of carrier recombination. The addition of g-C3N4 broadens the optical response spectrum of CdS. With the aid of simulated solar light, the ternary catalyst CuO/CuFe2O4/g-C3N4, decomposed 99% of tetracycline hydrochloride and removed 74% of the chemical oxygen requirement. Both the values are reportedly greater than those of either CuO/CuFe2O4 and pure g-C3N4 [Citation38]. With the aid of simulated solar light, this composite has decomposed tetracycline hydrochloride to a 99% extent and removed 74% of the chemical oxygen requirement, both of which are reportedly greater than those of either CuO/CuFe2O4 and pure g-C3N4. The enhanced reaction sites are provided by g-C3N4 substrate and inhibition of recombination of photo-excited electrons and holes is due to the synergetic effect of individual moieties in composite. •O2−, h+, and •OH were the oxidative components involved in the breakdown. Hydrothermal approach is successfully used to fabricate Fe3O4/CeO2/g-C3N4 composites for the photocatalytic degradation of tetracycline hydrochloride [Citation39]. The composite having 1:0.75:0.75 mass ratio displayed an excellent degradation rate of 96.63% within 180 min. The Fenton-like reaction mechanism was followed for the degradation of tetracycline hydrochloride. g-C3N4 decreased the release of Fe from the catalyst surface and ensures proper interaction of tetracycline hydrochloride with •OH. The experiment to capture active species demonstrated that •OH was the main reactive species to carry out the photocatalytic degradation of the antibiotic molecule. Fe3O4/g-C3N4/MoO3 nanocomposite displayed 94% degradation efficiency for tetracycline degradation under visible light irradiation [Citation40]. Nanocomposite has shown 5–20 times higher photocatalytic activity than that of the individual components owing to •O2− generation and synergetic effect of components to form Z-scheme structure leading to separation of e/h+ pairs.
g-C3N4/NaBiO3 nanocomposites produced through the hydrothermal method have been reported for the degradation of tetracycline [Citation41]. The Z-scheme heterojunction-mediated electric field to induces charge separation and give carriers with high redox potential the opportunity to produce •O2− and •OH, which reduced tetracycline to 87% of its original strength. The ternary photocatalyst BiOI/g-C3N4/CeO2 prepared by calcination and hydrothermal treatment has been used for the degradation of tetracycline under visible-light irradiation [Citation42]. Tetracycline is decomposed with an efficiency of 91.6% when using a catalyst with 3 wt% BiOI/g-C3N4/CeO2. The photocatalytic activity was reported in order BiOI/g-C3N4/CeO2> BiOI/g-C3N4> BiOI > CeO2> g-C3N4. The nanocomposite possessed approximately 13 and 34 times higher degradation rate and rate constant as compared to g-C3N4, respectively. The better separation of produced electron-hole and dual charge transfer process between different components at ternary heterojunction were the main reasons behind the improved photocatalytic activity. Akbarzadeh et al. reported g-C3N4/Ag/AgCl/BiVO4-based composite for the photocatalytic degradation of ibuprofen. The hydrothermal method was used for the fabrication of g-C3N4/Ag/AgCl/BiVO4 composites and maximum 94.7% ibuprofen degradation was observed within 60 min of light exposure [Citation43]. Photogenerated holes (h+) were the major reactive species causing the photocatalytic effect. The composite has shown better photocatalytic activity than BiVO4, Ag/AgCl/BiVO4, and g-C3N4/BiVO4 due to higher absorption of visible light and availability of electron-hole pairs generated along the heterojunction for a longer time. A similar mechanism was followed in the case of g-C3N4/Ag/AgCl/BiVO4-induced photocatalytic degradation of tetracycline. Nanocomposite has shown the best photocatalytic activity as compared to individual components and it was found to decrease in order g-C3N4/Ag/AgCl/BiVO4, Ag/AgCl/BiVO4, g-C3N4/BiVO4, BiVO4, g-C3N4. Kang et al. reported g-C3N4/Na-BiVO4 heterojunction as a catalyst for tetracycline degradation on visible light exposure in the presence of peroxymonosulfate [Citation44]. About 98.20% of the tetracycline is degraded in 40 min, which is 3.13 times higher than the degradation rate by BiVO4. The increased tetracycline degradation over the composite is attributed to the creation of Z-scheme heterojunctions, and oxygen vacancies brought on by Na+ which make it easier for electron–hole couples to move apart and form free radicals as shown in .
Figure 1. Possible mechanism of peroxymonosulfate assisted degradation of tetracycline using g-C3N4/Na-BiVO4 nanocomposites on exposure to light [Citation44].
![Figure 1. Possible mechanism of peroxymonosulfate assisted degradation of tetracycline using g-C3N4/Na-BiVO4 nanocomposites on exposure to light [Citation44].](/cms/asset/bce2c0f0-fdb1-45f4-b43b-1fe06bdd3bae/kbie_a_2260919_f0001_oc.jpg)
AgSCN/Ag3PO4/C3N4 heterojunction has been used for the decomposition of ibuprofen under sunlight. The protection of Ag3PO4 and the effective separation of electron–hole pairs by AgSCN and C3N4 components in the composite has resulted in its better degradation efficiency. The composite followed Z-mechanism and has 1.5–3.3 times higher catalytic activity than AgSCN/Ag3PO4, and Ag3PO4 [Citation45]. Cellulose acetate (CA) membrane functionalized with Cu2(OH)2CO3/g-C3N4 heterojunction (Cu/CN) has been used for the degradation of tetracycline [Citation46]. The Cu/CN@CA composite membrane has 48.43 mg/g tetracycline adsorption efficiency. Adsorption was supported by electron generation and exchange between g-C3N4 and Cu2(OH)2CO3 components. During solar light stimulation, type II heterojunction transfer pathway increased the membrane oxidation thus producing many active species that leads to tetracycline degradation.
Graphene oxide (GO) is one more carbonaceous substance that is frequently used as a design template and support for many hybrid composites. The addition of GO to composite materials makes an excellent photo catalyst because it activates the fabricated composite under sunlight and maintains effective charge carrier separation because of its large surface area [Citation47–49]. These characteristics have led to its use or coupling with other materials to maximize its benefits. Moztahida et al. [Citation50] reported heterogeneous Fenton-like hybrid composites Fe3O4/rGO for the degradation of carbamazepine (). The integration of rGO into the composite has the following goals: to boost the adsorption capacity of hybrid composite, decrease the aggregation of Fe3O4 nanoparticles, and decrease the charge recombination of exposed reactive sites. Within 180 min, carbamazepine is degraded by 98.7% with 10 wt% rGO loading. The composites could maintain the catalytic activity for up to five cycles in an acidic environment as it is magnetically separable and simple to regenerate.
Figure 2. Schematic presentation of mechanism of oxidative species generation in case of rGO-loaded-magnetite composite of during the light-induced degradation process [Citation50].
![Figure 2. Schematic presentation of mechanism of oxidative species generation in case of rGO-loaded-magnetite composite of during the light-induced degradation process [Citation50].](/cms/asset/aa75c068-d465-46f8-9d36-aed110d6871a/kbie_a_2260919_f0002_oc.jpg)
Composite comprising 2D rGO nanosheet/3D Fe2O3/2D g-C3N4 nanosheet has been fabricated using hydrothermal process for the tetracycline and ciprofloxacin treatment [Citation51]. The distinct heterojunction helped to produce numerous channels that caused fast exchange of light produced charge carriers which in turn significantly reduced the recombination rate. The nanocomposite has shown about 22- and 16-times higher tetracycline and ciprofloxacin degradation as compared to pristine g-C3N4 nanosheets, respectively. Su et al. fabricated Fe3O4@SiO2@BiFeO3/rGO composite using a hydrothermal technique which shows 95.6% and 98.2% ciprofloxacin and tetracycline hydrochloride degradation, respectively [Citation52]. rGO has a crucial role in reducing the energy gap, and suppression of the recombination of charge carriers thus regulating overall photocatalytic activity. GO@Fe3O4/ZnO/SnO2 nanocomposite has been reported for azithromycin degradation with a maximum of 90% efficiency on exposure to UV-C [Citation53]. Azithromycin degradation was performed in a constant bed column, and it was dependent on variables including height of the bedding material, rate of azithromycin flow, and initial concentration of azithromycin.
Carbon quantum dots (CQDs) have been regarded as remarkable candidate for interfacial modulation because of their inherent up-conversion photoluminescence, superior electron-transfer capabilities, and favorable biocompatibility [Citation54,Citation55]. As a result, these zero-dimensional carbon-based nanomaterials are potential candidate for photocatalysis and are a promising solid-state electron mediator. N-CQDs/oxygen vacancies (OV)-BiOBr nanocomposite prepared through hydrothermal approach has been employed for tetracycline degradation [Citation56]. The photo response region is greatly expanded, and the charge flow is effectively directed by the synergetic action of doped QDs and OV. Additionally, oxygen vacancies and N-CQDs promoted two-electron oxygen reduction and the production of •O2− and H2O2 through a single electron, respectively, which resulted in direct oxidation and indirect photo-Fenton-like reaction of tetracycline. With the increase in the amount of N-CQDs in composite, the photocatalytic performance is greatly improved.
Likewise, CQDs included in the CQDs/BiOCOOH/ultrathin g-C3N4 nanosheets (uCN) composite has mediated the transfer of electrons between BiOCOOH and uCN to fasten photocatalytic degradation of sulfathiazole. The composite containing 50 wt% uCN leads to complete degradation after 90 min of LED lamp exposure due to quick transfer and separation of photo-generated charges. The degradation efficiency was reported to be 97.35% ± 1.57 after five cycles of reuse [Citation57]. CQDs synthesized from corncob biomass using photoinduced approach have been incorporated to form Fe3O4/BiOBr/CQDs composite. The composite has been used for the photo-catalytic degradation of carbamazepine. The photocatalytic effect was mainly caused by Z-scheme structures formation, photoluminescence of CQDs, the synergetic effect of CQDs and Fe3O4 and generation of •O2−, h+, and •OH [Citation58]. enlists details about different carbonaceous material-based nanocomposites that have been explored as photo-catalyst for the remediation of pharmaceuticals wastewater.
Table 1. Carbonaceous material-based nanocompositess as photo-catalyst for remediation of pharmaceuticals wastewater.
2.2. Carbonaceous material-based adsorbents
Adsorption offers greater benefits in terms of pollutant treatment strategies when compared to photo degradation and other membrane-based technologies, because it is an efficient, straightforward, ecologically beneficial, and simple procedure [Citation59]. The efficacy of adsorption for antibiotic removal depends up on the sorbent nature, such as specific surface area, porosity, and pore diameter. Graphene has a polarized surface that can interact with various type of hydrophobic organic contaminants with the help of π-π interaction. However, it is difficult to separate and extract graphene from a suspension after adsorption in large-scale environmental applications. The problem can be overcome by further functionalization of magnetic graphene oxide with compounds rich in amino and carboxyl groups, among other things [Citation60]. Li et al. documented the use of graphene oxide functionalized with diethylenetriamine-pentaacetic acid (DDMGO) for the adsorption of tetracycline and ciprofloxacin [Citation61]. The abundance of oxygen or nitrogenous groups on the surface, high surface area to volume ratio, and the ease with which it could be easily separated and recycled due to magnetic properties from aqueous solution are some of the factors that make nanocomposites a better option as compared to individual components. In binary systems, the coexisting competing antibiotics had a higher inhibitory effect on tetracycline sorption than ciprofloxacin. Tetracycline and ciprofloxacin were removed by DDMGO mostly through the amidation process, H-bonds, and π-π interaction (). Cation-π and electrostatic interaction could be used to explain tetracycline and ciprofloxacin uptake, respectively. DDMGO was documented to possess better capability for removal of tetracycline and ciprofloxacin adsorption capabilities than other reported adsorbents namely, graphene nanosheets, functionalized graphene oxide with magnetic properties, MWCNTs, and activated carbon, removal [Citation62–65].
Figure 3. Adsorption schematic diagram of tetracycline and ciprofloxacin on DDMGO [Citation61].
![Figure 3. Adsorption schematic diagram of tetracycline and ciprofloxacin on DDMGO [Citation61].](/cms/asset/4da5752e-6bdc-4116-b271-647b10de2037/kbie_a_2260919_f0003_oc.jpg)
rGO-Fe3O4 has been fabricated for phenazopyridine residue removal from waste-water samples [Citation66]. The magnetic nanocomposite has 14.064 mg/L sorption capacity at pH = 6 for phenazopyridine with maximum of 91.4% phenazopyridine adsorption efficiency. Phenazopyridine adsorption occurs due to accelerated charge transport at the interface of nanocomposite components and its interaction with aromatic rings and oxygen-terminated group on the nanocomposite surface.
NPs can be functionalized using enzyme to increase their adsorption efficiency. Chitosan-graphene oxide (CsGOn) composite functionalized with horseradish peroxidase (HRP) has been used for the removal of ofloxacin [Citation67,Citation68]. HRP forms a covalent bond with HRP-CsGOn thus increasing the mechanical strength of the enzyme leading to ofloxacin removal [Citation69]. This green nanocomposite has shown 378 mg/g maximum adsorption efficiency and can be recycled for at least five cycles. Fe3O4@SiO2-Chitosan/GO nanocomposite is explored for tetracycline elimination [Citation70]. The greatest tetracycline adsorption capacities on nanocomposite with and without Cu(II) were 183.47 and 67.57 mmol/kg, respectively. The electrostatic and π–π interactions between the tetracycline and Cu (II) components of the composite were the major factors involved in the adsorption process.
Fe3O4@ polyethyleneimine (PEI)-rGO nanocomposite synthesized using a self-assemble method has been documented for adsorption of polar non-steroidal anti-inflammatory medicines; ketoprofen, naproxen, diclofenac, and ibuprofen. This composite involved the synergetic effect of both PEI and rGO which demonstrated greater extraction efficiency as compared to individual components, Fe3O4@ PEI, Fe3O4-rGO, and Fe3O4@PEI-GO. The adsorption mechanism displayed the potential of Fe3O4@PEI-rGO as a polar and π-π type adsorbent [Citation71]. Similarly, magnetically Fe3O4@ graphene nanoplatelets composite prepared by solid-phase fabrication has been reported for the removal of these non-steroidal anti-inflammatory drugs. H-bonding, hydrophobic, π–π electrostatic, and n–π electron acceptor–donor interaction were responsible for adsorption of drugs on the surface of nanocomposite [Citation72].
Tetracycline elimination rate of 99.4% is observed by using MnO2/graphene nanocomposite [Citation73]. The incorporation of MnO2 nanorods has modified adsorption efficiency as it avoided stacking too many graphene sheets together throughout the adsorption process and gave additional chances to tetracycline molecules for contacting the adsorbents. The primary adsorption mechanism involved complex formation by Mn(IV) and π-π interactions involving benzene ring of graphene sheets with tetracycline. Nanocomposite containing 40% MnO2/graphene has shown 198 mg g−1 adsorption capacity that was better than free treated graphene sheets with 170 mg g−1 capacity. A triple-network composite hydrogels with composition carbon nanotubes/L-cysteine@graphene oxide/sodium alginate (CNTs/L-cys@GO/SA) has been reported to remove ciprofloxacin antibiotic from aqueous contaminated samples [Citation74]. The internal three-dimensional space of network structure has shown maximum adsorption of 181 mg/g for ciprofloxacin in a weak acidic environment.
The ability of graphitic carbon nitride material to selectively interact with the various type of pollutant has been extended to remove pharmaceutical molecules from contaminated waste. It offers other advantages like being a nonmetallic environmentally safe material with tuneable thermal, chemical, and physical properties [Citation75,Citation76]. Fe3O4-g-CN@PEI-β-CD nanocomposite removes tetracycline with an efficiency of 833.33 mg/g. Larger surface area, more porosity, and presence of function groups that form nanocomposite–drug complex were main factors responsible for tetracycline adsorption [Citation77].
The activated carbon (AC) at the microscale is frequently utilized as a sorbent to remove various contaminants because of its unique structure and strong adsorption ability [Citation78]. AC made from the biomass is extremely effective as an adsorbent for removing pharmaceutical molecules. This includes hormone, antibiotics, anti-inflammatory, and analgesics compound [Citation79–81]. Nanoscale AC due to its high surface area, affordable commercial availability, and variety of surface-active groups support the binding and hence, the removal of pollutant [Citation82]. Activated carbon-Fe3O4 (AC-Fe3O4) nanocomposite with magnetic properties has been fabricated for the removal of promazine which is a phenothiazine antipsychotic drug. Nanocomposites possess high adsorption efficiency, quick adsorption, and simple magnetic separation. Complete drug removal with 101.01 mg/g adsorption capacity was achieved within 6 min [Citation83]. Yeast-extracted carbon functionalized with magnetic NPs (NP-YC) prepared from ethanol industry waste has been used for the Ibuprofen removal. Ibuprofen was removed from sewage effluent with 51 mg/g capacity by NP-YC [Citation84]. Nanocomposite made of carbon obtained from plantain peel functionalized with zinc oxide NPs (PPAC-ZnO) has been used for the adsorption of chloroquine, a medication used to treat malaria and COVID-19 [Citation85]. Maximum of 78.89% chloroquine adsorption was achieved.
Spherical fullerene is one of the members of the carbon nanomaterials family that has zero-dimensional structure with exceptional electron withdrawing capabilities [Citation86]. These nanostructures have properties that are on the cusp of being in between nanomaterials and molecules [Citation87]. Yet, numerous initiatives from the field of materials science to a variety of applications on fullerene chemistry and nano-molecular chemistry resulted in the development of readily produced composites [Citation88]. Fullerene produces active oxygen that helps in wastewater treatment [Citation89]. Functionalized magnetic fullerene nanocomposite (FMFN) has been used for the adsorption of the antibiotic Ciprofloxacin. Nanocomposite is produced by the catalytic thermal degradation of recyclable poly(ethylene terephthalate) bottle wastes as resource using ferrocene as a catalyst with magnetite precursor [Citation90]. After five cycles, the adsorbent’s capacity for regeneration is reported to remain high. The reported description about carbonaceous material-based nanocomposites as adsorbent for remediation of pharmaceuticals wastewater is tabulated in .
Table 2. Carbonaceous material-based nanocomposites as adsorbent for remediation of pharmaceuticals wastewater.
3. Metal and metal oxide-based nanocomposites for treatment of pharmaceutical wastewater
3.1. Metal and metal oxide-based photocatalyst
Functionalization of metal/metal oxide nanocomposite for wastewater remediation is well documented. Functionalized metal/metal oxide nanocomposite has been reported to contain high photocatalytic activity, low band gap, better separation of charge carriers, and recovery of the catalyst. The details about metal and metal oxide-based nanocomposite for remediation of pharmaceutical wastewater are tabulated in . CuO/ZnO composite prepared using thermal process has been used for visible light driven ciprofloxacin degradation [Citation91]. Hydroxyl radical (•OH) and the oxidative hole (h+) generation by nanocomposite were mainly responsible for the ciprofloxacin photodegradation.
Table 3. Metal and metal oxide-based nanocomposite as photocatalyst for remediation of pharmaceutical wastewater.
A simple two-step synthesis approach has been used to fabricate ZnO/Ag2CO3/Ag2O for the photocatalytic degradation of ibuprofen. The presence of Ag2CO3 and Ag2O heterostructure over the ZnO surface led to 99% degradation of Ibuprofen that is 10-fold better degradation than the pure ZnO [Citation92]. By employing Ce@TiO2 nanocomposites under UV irradiation, diclofenac photocatalytic degradation of the drug is increased as reported [Citation95]. After being exposed to UV light for 80 min, the diclofenac underwent full degradation. Comparing the effectiveness of Ce@TiO2 nanocomposites to commercial TiO2, nano TiO2, and CeO2 revealed that these materials have increased activity. Until to the fifth cycle, the Ce@TiO2 nanocomposite remained stable. Payan et al. produced Cu-TiO2@functionalized single-walled carbon nanotubes for the catalytic degradation of sulfamethazine within 135 min [Citation96]. On the surface of the SWCNTs, Cu-doped TiO2 particles are reported to be distributed uniformly that help to uniformly capture light induced electrons and reduction of electron–hole recombination.
Polypyrrole (PPy)-ZnO composite fabricated using polymerization technique has been used for the diclofenac degradation. PPy-ZnO has demonstrated a higher photocatalytic rate constant which is about twice as compared to ZnO. The h+ was the primary oxidizing species in charge of the 81% degradation of diclofenac by PPy-ZnO in just 60 min [Citation98]. Fe3O4/Bi2S3/BiOBr nanocomposites prepared using Fe3O4 nanosphere, Bi2S3 nanorod, and BiOBr nanosheet demonstrated excellent photo catalytic performance for the removal of diclofenac and ibuprofen with removal efficiencies of 93.81% and 96.78%, respectively [Citation97]. The solvothermal method used to prepare nanocomposite result has been found to improve the crystal behavior that was mainly responsible for better photocatalytic degradation by nanocomposite as compared to the pure individual component. h+ and e− played the most important roles in the diclofenac and ibuprofen photo degradation as per active species trapping experiment. BiOBr/Fe3O4@SiO2 nanocomposite prepared via the solvothermal method has been reported for the photocatalytic degradation of ibuprofen [Citation94]. The nanocomposite was a mesoporous magnetic photocatalyst that can achieve almost complete mineralization within 360 min of visible light exposure. The two main pathways for degradation are e−CB-mediated processes and direct-hole oxidation. The magnetic property was useful in photocatalyst recycling and approximately 80% of the photocatalytic activity was maintained even after five consecutive cycles.
Model pharmaceutical pollutant has been used to study photocatalytic removal using TiO2@ ZnFe2O4/Pd nanocomposite. The nanocomposite has been used in batch and continuous systems to carry out diclofenac degradation using sunlight [Citation99]. Pd coated nanocomposite displayed better diclofenac photo degradation than ZnFe2O4 and TiO2@ZnFe2O4 owing to its magnetically properties nanocomposite was recycled up to five cycles of usage, although the maximum photo catalytic activity was dropped from 86.1% in the first cycle to 71.38% in the final cycle. p-type nanoanatase TiO2/Zinc ferrite NPs based photocatalyst has been used for the degradation of anti-inflammatory drug, etodolac [Citation100].
Superparamagnetic Ag/AgBr/ZnFe2O4 nanocomposite has been reported for photocatalyic degradation of carbamazepine [Citation93]. The photocatalyst can be easily separated using external magnetic field. The semiconductor conduction and valance bands levels were studied to reveal Z- scheme mechanism of heterostructure photocatalyst. The photocatalytic activity has been observed to increase upon the addition of persulfate and it decreases upon the addition of H2O2. Overall, •O2− and •SO4− were the main species involved in the photocatalytic process. Sodium dodecyl sulfate (SDS)/BiOBr-magnetic bentonite (MB) nanocomposites has been used for the catalytic degradation of tetracycline and ciprofloxacin [Citation101]. Nanocomposite has shown better tetracycline and ciprofloxacin degradation activity with maximum 85% and 95% efficiency, respectively. Further, an external magnet allowed for the rapid separation of the photocatalysts from the solution within 20 s. SDS/BiOBr-MB composite showed improved photocatalytic degradation as compared to BiOBr, and BiOBr-MB. The synergetic effect due to presence of SDS, the n–n heterojunction between BiOBr and Fe3O4, and the addition of bentonite, which created a platform for dispersing the growth of BiOBr. Overall, these factors were responsible for the improved photocatalytic efficacy of SDS/BiOBr-MB (). The photo-generated electron–hole pairs of SDS/BiOBr-MB are more effectively separated and transferred to the n-n heterojunction between BiOBr and Fe3O4. The capacity of the bentonite’s permanently negative charge to absorb photo-generated holes led to a decrease in the number of photo-generated carriers.
Figure 4. Schematic representation of the mechanism of enhanced photogradation of tetracycline and ciprofloxacin by SDS/BiOBr-MB [Citation101].
![Figure 4. Schematic representation of the mechanism of enhanced photogradation of tetracycline and ciprofloxacin by SDS/BiOBr-MB [Citation101].](/cms/asset/8e55a0ea-8fe9-4886-aedc-e04c62f29db8/kbie_a_2260919_f0004_oc.jpg)
Nasseh et al. prepared magnetic FeNi3@SiO2@ZnO nanocomposite to photo-catalytically remove tamoxifen from wastewater simulated light [Citation102]. The photocatalyst could also effectively complete five rounds of photodegradation in a row. In addition to this, sulfamethoxazole, trimethoprim, erythromycin, and roxithromycin are four persistent antibiotics that are being removed from surface waters using a Fe3O4/ZnO based nanocomposite [Citation103]. The accessible Fe in the nanocomposite has a synergistic impact that aided in the secondary reaction of antibiotic oxidation by photo-Fenton. The system increased Fe3O4/ZnO photonic efficiency, simplicity of magnetic separation and catalyst reusability that depends upon the reactor configuration. Antibiotic removal efficiency was higher using stirred system as the catalyst was dispersed more evenly thus increasing the surface to volume ratio. The solvothermal method is used to generate the ZnFe2O4@TiO2/Cu nanocomposite which is used as photocatalytic degradation of naproxen [Citation104]. In sunlight, the nanocomposite showed 80% naproxen photodegradation. This composite exhibited ferromagnetic property, stability and cyclability up to five cycles with maximum 72.31% removal efficiency. The nanocomposite showed superior photocatalytic activity compared to individual components. This is as a result of the high surface area of copper that increases the charge and energy transfer between metal semiconductors. Flower shaped TiO2/Fe2O3 core–shell artificial light driven catalysts have been reported for the degradation of paracetamol [Citation105]. An increase in the content of TiO2 in composite improved the rate of paracetamol photodegradation and mineralization. The photocatalyst has strong stability and was magnetically separable up to four cycles.
To create the Fe3O4/BiVO4/CdS heterojunction photocatalyst, pure Fe3O4 and BiVO4 surfaces are simultaneously decorated with CdS in a low-temperature water bath system [Citation106]. During visible light irradiation, FBC-3 (2 mmol of Cd(CH3COO)2·2 H2O) showed the highest rate of tetracycline degradation (87%). The main active groups involved in tetracycline degradation are h+ and •O2−. The Z-scheme transfer route as a means of enhancing photocatalytic activity is considered. Fe3O4@ SiO2@ Bi2O2CO3-sepiolite nanocomposite has been reported for ciprofloxacin and tetracycline hydrochloride photodegradation with 92% and 100%, respectively [Citation107]. Sepiolite can increase the surface area of composite and provided a strong interfacial effect between the sepiolite and rest of the components of composite. This circumstance led to high capacity of light harvesting, separation of charge carriers, and formation of many active reaction sites on photocatalyst.
4. Metal-organic framework-based nanocomposites for treatment of pharmaceutical wastewater
4.1. Metal-organic framework-based adsorbents
MOFs have a spatial topology with a periodic porous structure and are often created by the self-assembly of organic ligand, metal ions, or clusters of metal ion. Ultra-high porosity, large surface area, tuneable structure, and ease of preparation are some of the amazing characteristics of MOFs [Citation108]. These fascinating advantages make MOFs appealing for drug delivery [Citation109], photocatalysis, and gas storage/separation application [Citation110,Citation111]. MOFs and their composites have shown tremendous application promise for wastewater treatment due to their improved water stability, great flexibility, and tuneable physicochemical features [Citation112–115]. Some common examples that demonstrate this idea include graphene oxide/MOFs (GO/MOFs) and polydimethylsiloxane/MOFs (PDMS/MOFs) [Citation116,Citation117]. The incorporation of graphene oxide in GO/MOFs composites offers a hydrophobic environment that prevent water molecules from attacking the coordination bonds. PDMS/MOFs coating with PDMS can lead to the synthesis of composite with hygroscopic or water repellent properties. The various types of MOFs functionalized nanocomposites that have been explored as an absorbent for the remediation of pharmaceutical wastewater are tabulated in .
Table 4. Mofs based nanocomposites as absorbent for remediation of pharmaceutical wastewater.
Adsorption is a highly competitive technology to remove pharmaceutical waste from aqueous solutions due to its cost-effective nature, ease of use, and lack of chances of secondary contamination. MIL-101(Cr) with sodium alginate (MIL-101(Cr)/SA) and MIL-101(Cr) with chitosan (MIL-101(Cr)/CS) composite prepared by combining MIL-101(Cr) with SA and CS has been used as an adsorbent for adsorption of ibuprofen and ketoprofen [Citation117]. The composite beads exhibit higher adsorption capability than individual components. The electrostatic interactions between Cr center and functional groups of the composites with the functional groups of the pharmaceutical contaminant were responsible for the adsorption. Further, it was possible to improve the hydrophobic properties by integrating adequate water-resistant functional groups in the MOFs. Xiong et al. reported MWCNT loaded iron-MOF [MIL-53(Fe)] composite for adsorption of tetracycline hydrochloride, oxytetracycline hydrochloride, and chlortetracycline hydrochloride from aqueous solutions [Citation118]. The nanocomposite has 1.6, 1.2, and 1.4 times more pore volume, specific surface area, and pore diameter than MIL-53, respectively due to the presence of MWCNT. This contributed to enhancement in the maximum tetracycline hydrochloride, oxytetracycline hydrochloride, and chlortetracycline hydrochloride adsorption capacity of nanocomposite that was 1.25–8.28 times higher than that of MWCNT, respectively. Likewise, amino-functionalized MIL-53(Fe) containing MWCNT/NH2-MIL-53(Fe) composite has also been used to adsorb chlortetracycline and tetracycline hydrochloride [Citation119]. The improved mesoporosity of MWCNT/NH2-MIL-53(Fe), the π-π interaction and hydrogen bonding between amino functional groups of composites with hydroxyl functional groups on tetracycline hydrochloride/chlortetracycline hydrochloride were collectively accountable for the increased adsorption capacity.
The addition of graphene oxide (GO) to MOF adsorbents produced more functional substances with wide pore structure in order to increase the adsorption efficiency. Hence, highly porous MOF composites are shaped by fusing GO with MIL-101(Cr) [Citation120]. The linking of GO layers to the MOF framework led to enhancement thus producing more pore openings and hydrogen bonding due to the availability of diverse functional groups on the composites. Compared to pure MIL-101 and commercial activated carbon, MIL-101GO (3%) composites showed significantly better adsorption toward anti-inflammatory drugs like naproxen and ketoprofen in a aqueous solution. In a similar way, Wei et al. reported GO/MOFs using the solvothermal technique to remove tetracycline hydrochloride [Citation121]. Zr/Fe-MOFs/GO composite has shown good absorption efficiency for tetracycline hydrochloride as compared to GBCM350 activated carbon [Citation136], Fe3O4@SiO2-chitosan/GO [Citation70], ZIF-8 [Citation112], NH2-MIL-101(Cr) [Citation137], MIL-101(HCl) [Citation138], and UiO-66 [Citation139]. Further, the hydrothermal approach is used to fabricate GNP/UiO-66 nanocomposite derived from zirconium-based MOF (UiO-66) and graphene nanoplatelets [Citation122]. GNP/UiO-66 NPs have a higher BET surface area than GNP NPs (750–910.518 m2/g), which raised their maximum adsorption effectiveness to 99.02%. MrGO/ZIF composite is created for the adsorption of methamphetamine by attaching ZIF-67 on magnetic substrates [Citation123]. Some of the properties contributed by different components of nanocomposites were responsible for its drug adsorption performance that include large surface area to volume ratio, hierarchical pore structure, Van der Waals forces, electrostatic interactions, and H-bonding and unsaturated coordinate bonds formation. Additionally, aluminum-based MOF/GO (MIL-68(Al)/GO) composite has been reported for aqueous tetracycline adsorption with maximum 228 mg/g capacity [Citation124]. In addition to intricate hydrogen bonding, π–π stacking and Al-N covalent bond formation were prominent interactions that supported the adsorption process. MIL-101(Cr)@GO has also been investigated for the adsorption of three different sulfonamides, namely sulfadiazine, sulfadoxine, and sulfamethoxazole [Citation125]. It is found to be reusable upto five cycles with a maximum 101–135 mg/gadsorption capacity. Hydrophobic interaction, π−π interactions, hydrogen, and coordinate bond formation are driving forces for the adsorption of sulfonamides on nanocomposite. Zr(VI)-based MOF UiO-66-(COOH)2/GO nanocomposite have shown five times better tetracycline hydrochloride adsorption than that of UiO-66 [Citation126]. It has achieved increased adsorption active sites by incorporating carboxyl groups and GO. Weak electrostatic interaction, chemical coordination, and π–π interaction between nanocomposite and tetracycline hydrochloride accounted for the adsorption process.
The metal doping of MOFs changes the electronegativity of the composite surface which ultimately alters the electrostatic interactions between the nanocomposite and pharmaceuticals. Jin et al. [Citation127] reported the formation of CuCo/MIL-101 composite by loading bimetallic CuCo NPs onMIL-101 for the removal of tetracycline from aqueous solutions. Nanocomposites have 140% more tetracycline removal efficiency than pure MIL-101. Improvement in adsorption efficiency was attributed to electrostatic interactions between CuCo NPs and tetracycline molecules as illustrated in .
Figure 5. Schematic diagrams of the synthesis of CuCo/MIL-101 and the adsorption of tetracycline [Citation127].
![Figure 5. Schematic diagrams of the synthesis of CuCo/MIL-101 and the adsorption of tetracycline [Citation127].](/cms/asset/69d71126-30a7-4760-a40e-5530d5cee023/kbie_a_2260919_f0005_oc.jpg)
Porous carbon materials can be functionalized to acquire essential properties including structural stability, porosity, and functional groups so that they can be used as excellent adsorbents. MnO@C nanocomposite produced by pyrolysis of metals or metal oxides self-supported on porous carbon was used for the adsorption of antibiotic pollutants including tetracycline, ciprofloxacin, diclofenac, and chloramphenicol in aqueous medium [Citation128]. Adsorption of antibiotics occurs due to the presence of graphitic carbon, Mn-O and other bonds in the nanocomposite. Additionally, Cu/Cu2O/CuO@C were reported for the adsorption of ciprofloxacin and tetracycline [Citation129]. Nanocomposites have a porous hollow structure along with several surface chemical bonds on its surface leading to antibiotic adsorption. XPS spectroscopy revealed the involvement of Cu (I, II) oxidation states, hydrogen bonds, π–π, and n–π interactions for higher adsorption efficiency than individual components.
The interference observed during the practical application in term of recovery and regeneration of the adsorbent after use is difficult to manage at large scale. One way to accomplish the goal of recovering and reusing the adsorbent is by combining magnetic materials with MOFs. Based on this concept, Wu et al. developed a Fe3O4/HKUST-1 copper-based MOF composite as an efficient and recyclable adsorbent for the removal of ciprofloxacin and norfloxacin types of fluoroquinolone antibiotics [Citation113]. Due to its high adsorption rate, magnetic composite 513–538 mg/g antibiotics adsorption capacity was achieved in less than 30 min. Nanocomposite can be separated from the contaminated water using external magnets. The primary sorption process involved in the removal of ciprofloxacin and norfloxacin from water using Fe3O4/HKUST-1 included π–π interactions, hydrophobic interactions, electrostatic interactions, and hydrogen bonding. Fe3O4/HKUST-1 was effectively regenerated and found reusable up to more than 10 times. Naeimi and Faghihian prepared MIL-53(Fe)/Fe3O4 composite for the removal of 322 mg/g doxycycline from aqueous solutions [Citation130]. The super paramagnetic character of composite was used to recycle it for six regenerations. Minocycline was removed within 160 min using magnetic aluminum-based MOF composite. Fe3O4@MIL-68 (Al) has maximum 248.05 mg/g adsorption efficiency [Citation131]. The adsorption mechanism revealed the participation of intricate interactions, such as electrostatic adsorption, hydrogen bonding, covalent Al–N and Fe–N bonds and π–π stacking. Another magnetic composite, MIL-101/Fe3O4, has been reported for the adsorptive removal of ciprofloxacin [Citation132]. Temperature-dependent difference in adsorption capacity was obtained with maximum 63.28 mg/g at 298 K. By exploiting the advantage of easily phase separable magnetic sorbent over non-magnetic sorbent, Fe3O4@MIL-100(Fe) and Fe3O4@MOF-235(Fe) is scrutinized for ciprofloxacin removal [Citation133]. However, magnetic nanocomposite with Fe3O4@MIL-100 (Fe) composition has a comparatively higher 322.58 mg/g adsorption capacity. The adsorption occurred in the form of a monolayer over the nanocomposite surface. The presence of Fe3O4NPs was mainly responsible for better adsorption capacity due to an increase in the number of accessible sorption sites as compared to bare MOFs.
Zeolite imidazolate framework-8 (ZIF-8) containing MOFs are known for their exceptional chemical stability, porosity, thermal/water stability, and active sites endowed for various types of chemical and physical interactions with pharmaceutical molecules in aqueous phase [Citation120]. Ceftazidime was removed by adsorptive means using ZIF-8@SiO2@Fe3O4, which was produced through the self-assembly of ZIF-8 with SiO2@Fe3O4 [Citation134]. Due to hydrophobic and electrostatic interactions, as well as a high specific surface area of 1273.08 m2/g, it demonstrated a greater adsorption capacity of 96.84 mg/g. After five cycles, nanocomposite could be efficiently regenerated with over 90% efficiency. Additionally, a dual MOFs-based smart platform produced using ZIF-8 and 2D amino-functionalized Al-MOF (NH2-MIL-53(Al)) nanoplates for the adsorption and detection of doxycycline, tetracycline, oxytetracycline, and chlortetracycline [Citation135]. In particular, the pyridine N of ZIF-8 in combination with -NH2 group on surface of NH2-MIL-53(Al) displayed significant affinity toward these drugs due to hydrogen-bond formation, π−π stacking and chemisorption.
4.2. Metal-organic framework-based photocatalysts
Adsorption and degradation processes for pharmaceutical waste are the main water clean-up techniques. The adsorption process could only reduce the concentration of pharmaceutical waste, but it could not get rid of this pharmaceutical waste [Citation140]. Antibiotics are mostly degraded by electrochemical, Fenton and photo-catalytic techniques. Among these processes, photo-catalysis could save a significant amount of energy by fully utilizing solar light throughout the degradation process [Citation141]. The development of heterojunctions is very advantageous for raising photo-catalytic performance of MOFs. It enhances the amount of light absorption by other components of heterojunction which produce more free electrons, voids, and reaction sites along with facilitating the charge separation [Citation142]. A detailed description about MOFs derived nanocomposites as photocatalyst for remediation of pharmaceutical waste water is tabulated in .
Table 5. Mofs based nanocomposites as photocatalyst for remediation of pharmaceutical wastewater.
CoTiO3/UiO-66-NH2 binary p–nheterojunction has been reported for the degradation of norfloxacin by designing and evolving competent heterogeneous catalyst for harvesting solar energy [Citation143]. The inverted V-shaped Mott–Schottky plot supported this binary p–n heterojunction. The improved photocatalytic reaction followed Type-II p–n hetero junction charge transfer mechanism with 90.13% degradation. Subudhi et al. reported various advantages for this heterojunction: (i) an increase in the lifespan of photo-generated active species including a variety of active sites by heterojunction, (ii) strengthening of the hydrophilicity due to hetero atoms which provide suitable contact site between CoTiO3/UiO-66-NH2 and norfloxacin, (iii) greater ability to respond to solar light than a photocatalyst in its purest state and (iv) unlocking new surface pores to capture the suitable adsorbates. Hydrothermal approach has been used to synthesize the double Z-scheme CuWO4/Bi2S3/ZIF67 ternary heterostructure for the degradation of metronidazole and cephalexin under visible light illumination [Citation144]. Comparing this ternary heterostructure with CuWO4/Bi2S3 and pristine ZIF67, the photoactivity of the former showed a notable improvement. Based on the dual Z-scheme structure, the augmentation of CuWO4/Bi2S3/ZIF67 photoactivity is attributed to the higher surface area, photo-stability, and suppression of bandgap in addition to better charge separation. Metronidazole and cephalexin, the ternary heterostructure had maximum degradation efficiencies of 95.6% and 90.1%, respectively. The ternary heterostructure showed remarkable chemical stability and reusability after six cycles. The trapping experiments are used to investigate the governing active species and the results showed that the main oxidants are hydroxyl free radicals. Bi2O2CO3/porous g-C3N4 Z-scheme heterojunction is created by Wang et al. to remove sulfamethazine [Citation145]. The amount of intimate contact at the interface is greatly increased as Bi2O2CO3 NPs evenly disperse across surface, edge, and interlayer in g-C3N4 nanosheet. The mechanism analysis showed that Bi2O2CO3/g-C3N4 composites specifically encouraged molecular oxygen activation into singlet oxygen (1O2). This may be attributed to Bi-N bonds at the interface and the development of Z-scheme system which speeded up the oxidation of •O2− by holes. The composites outperformed the pristine g-C3N4 in the elimination of sulfamethazine after 90 min of visible light illumination and helped to selectively generate (1O2) in a targeted manner during molecular oxygen activation.
For the photocatalytic elimination of sulfamethoxazole, an in-situ growing approach is used to generate a visible light responsive composite photocatalyst AgI/UiO-66 composite [Citation146]. As compared to pure AgI, the AgI/UiO-66 had significantly improved photocatalytic performance. Superoxide radicals (•O2−) and hydroxyl radicals (•OH) are shown to be the predominant active species in the photocatalytic degradation of sulfamethoxazole as per the radical capture studies. Phenyl nitrification, isoxazole ring hydroxylation, and S-N bond cleavage are all components of the sulfamethoxazole degradation pathway. Ag2S NPs are coupled with MOFs material (MIL-53(Fe)) using an easy solvothermal method for tetracycline degradation to create Ag2S/MIL-53(Fe) heterojunction hybrids [Citation147]. Nano composites have shown higher photocatalytic activities as compared to bare individual components. The creation of heterojunction with close contact between interface improved charge transfer and separation, band gap reduction, and increased photo absorption which in turn was responsible for the increased catalytic activity. Moreover, the synergistic effects of light and Fenton catalysis allowed the addition of H2O2 which further improved the catalytic activity.
A series of mesoporous composites of UiO-67/CdS/rGO-x has been fabricated by incorporating visible-light responsive CdS NPs into MOF and encapsulating them in excellent conductor rGO thin sheets. In this composite, the simultaneous utilization of sunlight-harvesting and electron-relay-penetration is exploited [Citation148]. It led to a maximum 93.4% ofloxacin degradation within 30 min under the simulated photo exposure. This is credited to following synergistic aspects of extension of the light absorption range of MOF, band-gap matching, and maintenance stability and activity of catalytic site. Further, TiO2-based catalysts upon using a molybdenum disulfide/ZIF-8 composite photocatalyst have been reported for ciprofloxacin and tetracycline hydrochloride degradation [Citation149]. Nanocomposite has better photodegradation due to better conductivity of light generated electron and lowering the recombination rate.
MOFs can have structural defects and combining different capabilities because of heterogeneity as they are solid-state crystals. By carefully managing the structural heterogeneity and defects through defect engineering, it is possible to manipulate the desired characteristics of MOFs, such as the production of mesopores, reduction of network rigidity/density, enhancement of active sites, development of novel functionalities, and mechanical properties. In continuation to this aspect, Gao et al. reported the use of defect-engineered MOFs with multi-functionalities for the degradation of diclofenac [Citation150]. The level of defect affected the crystallite morphology, phase purity and properties of nanocomposite with a maximum 590 mg/g adsorption capacity. The formation of 1O2was observed that ultimately indicated the involvement of type II photosensitization reaction. The degradation of diclofenac is facilitated by both the energy transfer from TCPP to triplet oxygen and the electron transport from TCPP to Zr clusters. A dual-function platform with CuBi2O4@ZIF-8 composites has been documented to carry out sensing and degradation of tetracycline [Citation151]. Nanocomposite owing to the better formation of free radicals and charge separation was observed to cause maximum 75.2% degradation which was higher than 50–73% in case of individual components, ZIF-8 and CuBi2O4.
The extraordinary function that the heterostructure plays in charge separation made the development of heterostructures in photocatalysts an efficient way to increase their catalytic activity. Regarding this, a simple so no crystallization method is successful in producing a new type II photocatalyst ZnO/ZIF-9 heterojunction [Citation152]. ZnO/ZIF0.5 could attain 87.7% tetracycline photodegradation within 60 min. Type II heterojunction formation between ZnO and ZIF-9 is primarily responsible for the higher photocatalytic activity because it increases the conductance of light generated electrons to make it easier to separate charges from holes. Li et al. reported ZIF-8@TiO2NPs containing composite for the catalytic degradation of tetracycline [Citation153]. MOF with porous structure and superior adsorption capacity, electron transmission by ZIF-8 in the ZIF-8@TiO2 micron composite, reducing the charge carrier’s recombination rate, visible light absorption and narrow band gap are prominent factors for enhancing the catalytic ability of the composite photocatalyst.
MIL-101(Fe)/TiO2 composite with magnetic properties has been prepared to photodegrade tetracycline to take the advantage of easy separation of catalyst [Citation154]. Under sun light irradiation, 92.76% of the 20 mg/L tetracycline was degraded in 10 min. Further, TiO2 added to the composite has a significant effect on the catalytic process, which could be enhanced by light exposure to produce significant amounts of the radicals •O2− and •OH. The composite has high reusability as it is easy to recover the composite from tetracycline solution using a magnet. Using Fe3O4 as a metal precursor, Fe3O4@MIL-100(Fe) was created through microwave in 30 min and used to treat diclofenac sodium in water samples [Citation155]. Under visible light, H2O2-assisted Fe3O4@MIL-100(Fe) photocatalytic process 99.4% diclofenac sodium degradation was achieved. The diclofenac sodium degradation process followed Fenton-like reaction. Rasheed et al. combined MIL-100(Fe) with Fe3O4 and carbon aerogel to form a nanocomposite for the removal of tetracycline hydrochloride [Citation156]. The comparative analysis revealed 85% antibiotic degradation by MIL-100(Fe)@Fe3O4/CA nanocomposite that was higher than observed in case of individual components. The performance of MIL-100(Fe)@Fe3O4 increased 1.6 times by coupling carbon aerogel with it. This significantly speeded up the transfer of light produced charge carriers. Further, MIL-88A (Fe) particles were immobilized onto the porous block substrate and then calcined to produce the supported MIL-88A derivative Fe3O4@ porous block substrate catalyst to remove tetracycline hydrochloride [Citation157]. Maximum 97.5% tetracycline hydrochloride degradation was observed within 40 min of white light exposure. Even after 30 consecutive recycles, the nanocomposite could achieve very simple recycling and great reusability. According to Doan et al., a Cu2O/Fe3O4/MIL-101(Fe) nanocomposite degraded the antibiotic ciprofloxacin in an aqueous solution when exposed to visible light [Citation158]. The ciprofloxacin degradation process is considerably aided by the lowered band gap energy, e–/h+ recombination suppression, and production of hydroxyl and superoxide radicals. After five cycles, the ciprofloxacin degradation efficiency dropped by just 6%, which demonstrated the outstanding cyclability of Cu2O/Fe3O4/MIL-101(Fe) nanocomposites. Bagherzadeh et al. reported the best performance of MIL/CoFe2O4/(3%)GO as compared to MIL-101(Fe), CoFe2O4, novel binary (MIL-101(Fe)/CoFe2O4, MIL-101(Fe)/GO and CoFe2O4/GO) for visible light photocatalytic and photo-Fenton-like degradation of tetracycline hydrochloride [Citation159]. Besides effectively separating charge carriers and reducing their recombination, the inclusion of the optimal GO as a strong electron acceptor in MIL/CoFe2O4/(3%)GO significantly boosted the absorption of visible light. A magnetic photocatalyst Fe3O4@MIL-53(Fe) is fabricated via calcination treatment of pristine MIL-53(Fe) under visible light and composite demonstrated 99% photocatalytic removal of ibuprofen in the presence of H2O2 after 60 min [Citation160]. In the photo-degradation process, active species like h+, •OH, e−, and •O2− played crucial roles. g-C3N4@CoFe2O4/Fe2O3 composite is fabricated by taking advantage of the Z-scheme heterojunction upon combining g-C3N4 and MOF-derived CoFe2O4/Fe2O3 [Citation161]. A small quantity of MOF-derived CoFe2O4/Fe2O3 doping significantly improved the adsorption capacity of g-C3N4 to absorb visible light and decrease its band gap. As a high-efficient mediator, the generated hybrid photocatalysts were used to activate persulfate for the degradation of emerging pharmaceutical pollutants upon exposure to visible light. After 80 min, tetracycline (99.7%), sulamethoxazole (94.8%), diclofenac (97.0%), ibuprofen (96.1%), and ofloxacin (96.5%) all could be removed. The magnetic Fe3O4@MIL-100(Fe) hybrid composites can encourage photo-Fenton-like process which has been further exploited for levofloxacin degradation [Citation162]. The composite with a Fe3O4: MIL-100(Fe) mass ratio of 1:4 has a greater degrading efficiency (up to 93.4%) as compared to Fe3O4, MIL-100(Fe) and the other produced composites. This increased catalytic activity is attributed to the synergistic interaction between Fe3O4 and MIL-100(Fe) which favored both the effective reduction of Fe3+ to Fe2+ in the photo-Fenton reaction and the speedy separation of the hole-electron pair from MIL-100(Fe). Based on free radical quenching, electron paramagnetic resonance, and mass spectrometry research, a preliminary hypothesis is made on the potential degradation mechanism and intermediates of levofloxacin in the photo-Fenton reaction.
5. Other 2D material-based nanocomposites for treatment of pharmaceutical waste water
As potential adsorbents and photocatalyst for the efficient treatment of several environmental pollutants, functionalized two-dimensional 2D NPs/nanocomposites possess distinctive properties. The different parameters about other 2D-based nanocomposite as photocatalyst for remediation of pharmaceutical wastewater are in .
Table 6. 2D material-based nanocomposite as photocatalyst for remediation of pharmaceutical wastewater.
5.1. MXene nanocomposite
Several studies reported MXene as a photocatalyst for the removal of hazardous pollutants from air and water [Citation182]. MXenes have accessible metal sites and good electron conductivity thus making it a useful co-catalyst. The hydrophilic hydrogen and oxygen groups on MXenes surface makes its interaction with diverse pollutant easy [Citation183]. In 2D form MXenes creates Schottky barrier at the photocatalyst/MXene interface due to movement of charge from photocatalysts to the MXene. This process is helpful in inhibiting electron–hole recombination [Citation184]. The modification of MXenes with different moieties has earlier been explored to improve its photocatalytic abilities. Faster recombination of electron holes in TiO2has been reported to cause low quantum yield and poor photocatalytic efficiency [Citation185]. The combination of TiO2 with MXenes resulted into semiconductor heterojunctions with reduced band gap and an effective photocatalysis [Citation186]. Shahzad et al. produced an effective photocatalytic heterostructure 001-T/MX nanocomposite using a hydrothermal method [Citation163]. The nanocomposite has 98.67% carbamazepine degradation efficiency as compared to ~60% in case of pure Ti3C2Tx MXene. The presence of {0 0 1} facets of TiO2 inserted into Ti3C2Tx sheets was primarily responsible for the better photocatalytic activity of pure MXene. The superior photocatalytic activity was attributed to Schottky junctions and the additional e− and h+ produced by {0 0 1} facets of TiO2. Because of free radicals •OH, •O2– and the strong oxidation ability of 001-T/MX photocatalyst to breakdown carbamazepine into CO2 and H2O toward the end of the process are possible carbamazepine photocatalytic degradation mechanism. Despite good catalytic activity, the poor stability of MXenes makes them less useful. On the other hand, polymeric carbon nitride (C3N4) has good solubility being a metal-free photocatalyst, but it has disadvantages in the form of low conductivity and severe charge recombination. Keeping this in mind, delaminated-Ti3C2Tx/alkalized-C3N4 (TC-aCN), a strong heterostructure photocatalyst made by Yi et al., was embedded into alkalized C3N4 in a few layers without being oxidized [Citation164]. The combined effect of both these effects has made the process stable and has been used for the visible light assisted removal of tetracycline hydrochloride with 77% efficiency. The robust TC-aCN heterostructure in the form of composite having close contact between its components, Schottky junction formed between the alkalized C3N4 and Ti3C and holes generated in the in the VB of C3N4 on visible light exposure were mainly responsible for the catalytic breakdown of tetracycline hydrochloride. The photocatalyst demonstrated exceptional stability and reusability up to four cycles. In another study, g-C3N4/Ti3C2/TNTAs has been used as a visible-light-driven catalyst for the 85% degradation of tetracycline hydrochloride. g-C3N4 and Ti3C2 mixture had uniformly covered the TiO2 nanotube arrays that led to increase in surface area, better light absorption, and charge carrier migration. Hydroxylation, dimethyl amino loss, aromatic ring, double bond oxidation, and carboatomic ring cleavage were identified as five steps of the degradation process [Citation165].
A single step in-situ calcination process has been reported to prepare MXene-derived TiO2/g-C3N4. The nanocomposite demonstrated higher photocatalytic degradation of tetracycline and ciprofloxacin compared to TiO2/graphene, g-C3N4, and conventionalTiO2/g-C3N4. Better photocatalytic activity was attributed to improved visible light adsorption, carrier separation, and generation of •OH and •O2 – free radicals [Citation166].
Simple hydrothermal procedure has been used to produce Bi2WO6/Nb2CTx nanosheets [Citation167]. Nb2CTx nanosheets significantly increased the separation of carriers and transfer of electrons from conduction band of Bi2WO6 to Nb2CTx. The created at the Bi2WO6/Nb2CTx interface act as Schottky junction to prevent recombination of photogenerated electron-hole pairs. Bi2WO6/Nb2CTx hybrid nanosheet photocatalysts have shown superior photodegradation efficiency for tetracycline hydrochloride as compared to Bi2WO6.
Zero-valent iron NPs functionalized Ti3C2-based MXene (nZVI@Ti3C2) nanosheets has been used for the treatment of ranitidine [Citation168]. Nanosheets acted as Fenton like catalyst for the H2O2 dependent oxidation of ranitidine. Ti3C-based MXene prevented the agglomeration of and promoted the electron transmission between the magnetic NPs. Ranitidine molecules are primarily broken down by hydroxyl radical (•OH) attacks, particularly those that targeted surface-bound •OHads (.). Diluted hydrochloric acid treatment was effective to regenerate the catalyst for multiple times use. CuFe2O4/MXene heterojunction has been used for the photocatalytic degradation of sulfamethazine antibiotic [Citation169]. Ternary photocatalysts Au-GCN-MXene nanocomposites has been developed using Au plasmonic NPs incorporating graphitic carbon nitride and Ti3C2 MXene to degrade cefixime antibiotic. •OH produced by nanocomposite on exposure to light exposure of the helps in the breaking of the cefixime bonds. Ti3C2 ensured the prolonged availability of photoinduced carriers by preventing the reorganization of photogenerated electron holes [Citation170]. The synergetic effect posed by 2D GCN and 2D Ti3C2 MXene nanosheet is significant from photocatalytic activity point of view. 2D GCN nanosheets acted as semiconducting photocatalyst that effectively harvests energy from visible light and 2D Ti3C2 MXene due to their increased surface are acted as electron sink. Due to the establishment of interfacial contact with GCN nanosheets, the presence of Au NPs and MXene in the nanocomposite increased the number of active sites and prevented the interaction of charge carrier. Moreover, the Au NPs plasmonic impact enhances light absorption, improving the photocatalytic efficacy of the nanocomposite. The ternary heterojunction photocatalysts made of Ti3C2/TiO2/BiOCl has been reported for the treatment of tetracycline. The creation of heterostructures resulted into better generation of •O2− and •OH radicals and better separation efficiency of photoinduced carriers. Even after four cycles the heterojunctions exhibited good stability and activity [Citation187]. MXene-Ti3C2/MoS2 composites prepared through hydrothermal process have been documented for ranitidine photodegradation [Citation171]. The superior catalytic activity was due to the Schottky-like heterojunction of Ti3C2/MoS2 which improved charge carrier separation. The nanocomposite with 37.69% concentration of Ti3C2 demonstrated the best photocatalytic activity. Maximum 88.4% ranitidine degradation was achieved within 60 min.
Figure 6. (a) Schematic illustration of the mechanism of ranitidine degradation by nZVI@Ti3C2/H2O2 system. (b) possible type of reaction zone in this heterogeneous Fenton-like oxidation system [Citation168].
![Figure 6. (a) Schematic illustration of the mechanism of ranitidine degradation by nZVI@Ti3C2/H2O2 system. (b) possible type of reaction zone in this heterogeneous Fenton-like oxidation system [Citation168].](/cms/asset/e51c7e7e-4d06-41af-be31-5e6bbba39564/kbie_a_2260919_f0006_oc.jpg)
5.2. Boron nitride nanocomposite
Hexagonal boron nitride (h-BN) has a van der Waals heterojunction and 2D atomic crystal structure like graphene and hence, is referred as ‘white graphene.’ It is made up of an equal amount of nitrogen and boron atoms [Citation188]. h-BN are unique characteristics that suggest it has great potential for use in catalysis, adsorption, and energy storage [Citation189–192]. h-BN is a hydrophobic material with increased tendency to aggregate in water; thus, considerably decreasing its ability to treat organic contaminants [Citation193]. Grinding and surface modification of h-BN can alter its surface characteristics, increasing its sorption capacity [Citation194].
Du et al. developed an ultrathin h-BN/Bi2MoO6 heterojunction with good photocatalytic activity using a workable solvothermal method [Citation172]. The surface of h-BN is uniformly loaded with Bi2MoO6. The addition of h-BN boosted the photocatalytic ability through better absorb of light. Nanocomposites having 50 wt% h-BN/Bi2MoO6 had better degradation with maximum 99.19%, 95.28%, and 91.04% efficiency in case of tetracycline, oxytetracycline, respectively. h+ and •O2– were mainly responsible for photocatalytic activity. h-BN prevented photogenerated electron-hole pairs from recombining. TiO2-BN nanocomposites prepared and their potential as attractive photocatalysts for the treatment of ibuprofen in water is explored under visible light [Citation173]. The TiO2-BN composites appeared to have shorter band gaps as compared to pure TiO2 nanoscale fibers as per UV-vis absorption spectra. Higher photocurrents are reported for the TiO2-BN nanocomposites than for pure TiO2 which suggested that negative charge on the BN nanosheets encouraged the charge separation by transporting the holes to the TiO2 surface. The maximum photocatalytic activity for the treatment of ibuprofen under visible light is demonstrated by TiO2-10 wt% BN and kinetic rate constant is almost 10 times more for TiO2-10 wt% BN than pure TiO2 [Citation174]. The addition of boron nitride nanosheets to MIL-53(Fe) altered its morphology and giving it a distinctive prism shape for the improved persulfate assisted catalysis of ibuprofen. BNFe-3 Composites containing three percent boron nitride nanosheets exhibited maximum 99% ibuprofen degradation activity. Ibuprofen degradation was aided by the formation of •SO2 −, •OH and •O2–. Cai et al. produced MoSe2/ZnO/p-BN photocatalyst for the degradation of ofloxacin (OFL). Nanocomposite with 30 wt % of produced maximum 92.2% ofloxacin degradation under visible light irradiation. Synergetic effect of tight adsorption of ofloxacin by p-BN and better visible light absorption by flower-shape MoSe2 was responsible for improved catalytic activity. Active species quenching tests revealed that the degradation of antibiotic was largely mediated by e−, •O2−, h+, and •OH [Citation175]. Boron nitride quantum dots-functionalized reduced g-C3N4 (BNRU) nanocomposite has been produced for the ciprofloxacin, ofloxacin, enrofloxacin, norfloxacin, and lomefloxacin with 100%, 94.4%, 87.7%, 100%, and 94.6% efficiencies, respectively. BNRU had a 1.7-fold greater carrier density as compared to ultrathin g-C3N4. Synergistic effects of defect engineering and quantum dots loading were mainly responsible for better antibiotic degradation activity of BNRU [Citation176].
5.3. Metal chalcogenide nanocomposite
Metal chalcogenides due to their easy availability in a range of chemical composition, variable bandgap, light tuneable optical, electrical, and catalytic properties are in focus for treatment of pharmaceutical contaminant in water [Citation195]. Hence, metal chalcogenide has been used to form 2D/2D heterojunctions to prepare better photocatalyst through separation and transportation of charge carriers [Citation114,Citation196]. Che et al. reported the production of yeast-derived carbon (YC) functionalized Cu2WS4/g-C3N4 heterojunction to form 2D nanocomposite. The Cu2WS4/YC/g-C3N4 has been used for the degrading tetracycline under visible light [Citation177]. Cu2WS4 has a strong photo-catalytic activity and ease of crystal surface modulation. YC sphere acted as electron bridge which helped to prevent rapid electron recombination and significantly increased the ability to absorb visible light. The nanocomposite exhibited better catalytic activity than individual pure components. O2−, h+ and •OH all have a significant impact on the degradation of tetracycline. An immobilized Z-scheme CdS/Au/TiO2 nanobelts composite photocatalyst made of CdS, Au, and TiO2 nanobelts were produced to augment the norfloxacin degradation activity on exposure to solar light [Citation178]. The CdS NPs improved overall light harvesting, TiO2 NBs offered more absorption and reaction sites for the special designs and Au served as the electron transfer mediator, boosted the interfacial charge transfer and effective separation of electrons and holes. CdS/Au/TiO2 nanobelts showed the highest rate of degradation after being exposed to light for 60 min. The other photocatalysts degrade in the following order: CdS/Au/TiO2 NBs (64.67%) > CdS/TiO2 NBs (55.94%) > Au/TiO2 NBs (50.60%) > TiO2 NBs (42.04%). This trend is ascribed to increased light absorption and the separation of photogenerated electrons and holes result in mentioned composite. Superoxide radicals (•O2−) shown to be the most active species throughout the photocatalysis process in the radical trapping tests. Cu2WS4/NiTiO3 (CWS/NTO) composite prepared using an easy electrospinning/calcination approach along with hydrothermal procedure has been documented to show improved abilities to separate photo-generated charge carriers, endowing these composites with good and enduring photocatalytic performance for tetracycline. The degradation rate for pure NTO and CWS is just 21.5% and 43.9%, respectively within 60 min. The degradation efficiency for tetracycline exhibited a substantial improvement after the formation of CWS/NTO heterojunction under the same conditions, with degradation efficiency 88.6%, CWS/NTO composites (x = 0.5). Free radical species •O2− and h+ were mainly responsible for the degradation of tetracycline degradation are [Citation179]. Z-scheme ZnIn2S4/MoO3 is fabricated using hydrothermal method tailed by the impregnation for the efficient degradation of paracetamol as compared to pure MoO3 and ZnIn2S4 [Citation180]. The components of composite were tightly bound that is advantageous for the catalysis and ensured efficient charge carriers’ separation. ZnIn2S4/MoO3 has prolonged electron-hole pairs separation and the better visible light absorption owing to the direct Z-scheme system. Ganguly et al. reported ternary metal chalcogenide heterostructure nanocomposites photo-catalyst of titania via hybrid nanoarchitectures of AgInS2 with TiO2 using a modified sol-gel technique [Citation181]. In comparison to their pristine parent samples, nanocomposites exhibit better light absorption as well as charge carrier separation. 0.5 wt% AgInS2 loading exhibited more than 95% sunlight assisted degradation of doxycycline in 3 h. Recent studies on boron nitride (BN) have shown that it is an effective adsorbent for organic pollutants and heavy metal ions [Citation197–199]. The low density and sufficiently robust graphene nanoplatelet/Boron Nitride composite aerogels (GNP/BNA) have been used for the adsorption of ciprofloxacin with a capacity of 185 mg/g. Various groups on the surface of nanocomposite particularly –COO and –CO functional groups were mainly responsible for the adsorption of ciprofloxacin [Citation200]. Further, to remove 17-ethinylestradiol from a water solution by adsorption, Xu et al. reported a magnetic MXene composite made of Fe3O4@Ti3C2 [Citation201]. The quadratic model is used to optimize the process variables for the highest adsorption of 17-ethinylestradiol by Fe3O4@Ti3C2. Maximum 97% adsorption was achieved. Hydrogen bonding between -O, -F, and -OH functional groups on the MXene Ti3C2and aromatic ring/phenolic hydroxyl groups of 17-ethinylestradiol were mainly responsible for adsorption.
Worldwide nanotechnology market was approximately 7.73 billion in 2022 which is expected to grow up to more than $114 billion by 2030 [Citation202]. On a rough estimate, the cost to produce one gram of gold NPs is 1600 times more than cost to produce pure gold from raw material. The cost of gold NPs may be $0.08 per microgram [Citation203]. Industrial scale production of NPs is cheaper using bottom-up approach as compare to top-down approach. A tool CatCostTM has been used for the estimation of cost-effective precursor for the chemical synthesis of nickel catalyst. This was useful to lower nickel NPs production by 58% as estimated using the U.S. Bureau of Labor Statistics Chemical Producer Price Index [Citation204]. Further, green synthesis routes are cheaper than chemical synthesis approach [Citation205]. Every year more than three percent of global population is dying due to contaminated water. The problem is predicted to aggravate further with approximately more than 57% world population affected by the availability of clean drinking water. India and China account for more than 36% world population, but currently has access to only 10% of the drinking water. Countries are taking measures to clean the contaminated water. India pledged USD 46.5 billion to provide clean drinking water. Germany is one of the leading countries setting up approximately 3000 waste water treatment plants treating 920 million cubic meters of wastewater [Citation206].
6. Challenges and outlooks
Advancements in medical research have resulted in the modernization of healthcare facilities, subsequently leading to a higher level of production and usage of pharmaceuticals to sustain better quality of life [Citation207]. 26% of the world population lack clean drinking water in 2020. By 2050 half of the global urban population is expected to face clean water scarcity [Citation208].
The presence of pharmaceutical compounds has been reported in more than 1,052 sites of 258 rivers in more than half of the world’s countries. Water pollution by pharmaceutical products is a serious health concern. Pharmaceutical pollutant has been reported to induce severe genotoxic response upon long-term exposure to human. Sub-Saharan Africa, south Asia, and South America were the most contaminated areas with under developed and developing countries were having highest amount of pharmaceutical contaminant [Citation207,Citation209]. Increasing access to medicines in middle income countries has led to higher volume of pharmaceuticals in wastewater as compared to low-income countries because both countries have poor waste water management while middle income countries have comparatively more access to medicines [Citation207]. Efficient and cost-effective water treatment strategies are required by both low, as well as middle income countries. Pharmaceutical contamination of water can lead to development of antibiotic and drug resistant in microorganisms. Resistance is a natural phenomenon as these microorganisms must survive in the presence of such contaminated water. Study including bacterial antibiotic resistance for 23 pathogens and 88 pathogen-drug combinations in 204 countries and territories in 2019 covering 471 million individual records or isolates revealed that top seven microorganism accounted for four to seven hundred thousand deaths in 2019 due to antibiotic resistance. Among these methicillin resistant Staphylococcus aureus caused maximum one hundred thousand deaths in a year [Citation209]. The aquatic animals and other organisms present in the contaminated water experience toxicity leading to ecosystem disturbance. Further, the water may be consumed by terrestrial animals and consumption of such organisms by human along with contaminated soil grown vegetables and fruits may lead to serious health hazards [Citation210].
Among the various types of pharmaceutical wastewater treatment methods, the microorganism-based method is cost-effective and produces sludge with low toxicity to environment. However, sometimes microorganism may covert less toxic pharma waste to more toxic components. The conventional treatment methods suffer from high capital investment and require regular input to maintain efficiency. Microbial treatment methods are cost effective; generate less toxic secondary sludge and are eco-friendly [Citation211]. Adsorption of pharmaceutical pollutant is an effective and economical strategy that allows regeneration of adsorbents. Along with routine adsorbent, the use of nano adsorbent further improves the adsorption efficiency in terms of porosity, tunability, and surface area. Currently the application of nano adsorbent is costly as compared to routine adsorbent due to the cost of nanomaterial synthesis. However, with the advent of nanomaterials that has higher adsorption capacities and can be regenerated more easily for several generations as compare to routine adsorbent overall it may be compensated for the difference in the cost [Citation212]. The challenges with adsorbent as well as nano-adsorbent are the poor regeneration efficiency after few a cycle of usage. Different methods are different methods that are followed to regenerate the adsorbent and among them few use acid and base that ultimately pollute environment. In case of poor regeneration efficiency, the absorbent needs to be discarded and itself become a pollutant. To regenerate the nano adsorbent, the methods that have low input cost and do not use harmful chemicals to cause desorption of bound contaminant need to be thoroughly explored.
Membrane-based technologies are the most efficient available and reliable method to filter water for drinking purposes. This method is expensive and wastes more water than it filters. We still lack another reliable method that can work at large scale making it the most used method [Citation213]. Nanomaterial functionalization of membranes has been in focus to further enhance its efficiency. The advantageous properties of nanomaterials can be completely utilized if the concerns regarding nanomaterials are seriously addressed. Although attempts are made to have strategies to handle nanomaterials more carefully than routine contaminant, however, still there is no general method for safe disposal of nanomaterials [Citation214]. The type of nanomaterials used to prepare nano adsorbent needs to be carefully selected. Nanomaterial synthesis is challenging as the control over size, shape, and surface properties is difficult. Further, heavy metals and toxic metal-based materials should be avoided. Organic sources must be focused to prepare nanomaterials. Further the method followed to prepare organic nanomaterials must follow green chemistry principles. This would make the nano adsorbent eco-friendly itself to a great extend thus reducing the pollutant load.
Green synthesis of NPs gives better stability products however, green synthesis suffers from few drawbacks that needs to be sorted out before making it is a method of choice for large scale production [Citation215,Citation216]. Hence combination of environmental contamination and limited resource in case of nonrenewable resources makes recycling of nanoproducts and use of eco-friendly synthesis method essential. Grimaldi et al. designed a milli-continuous flow that has less manpower input cost by using better procedures that mainly include cleaning using mild detergent and less waste production as compare to batch synthesis method [Citation217]. Ross et al. designed a NanoParticle Flow Synthesis System (NPFloSS) for the synthesis of large volume of silver and gold NPs [Citation218]. Solution-based and substrate-confined nanoreactors has been used for the synthesis of various nanomaterials [Citation219].
7. Conclusion
The elevated concentration of pharmaceuticals in water have posed an alarming situation for human health and environmental sustainability. This review discusses the current developments in the adsorptive and photocatalytic techniques being used to remove pharmaceutical pollutants using nanocomposites decorated with diverse types of nanomaterials. This has enhanced the effectiveness of photocatalysis and adsorption process. In-depth review is conducted on the nanocomposites that contain metal organic frameworks, carbonaceous material, metal/metal oxide and 2D materials such as MXenes, boron nitride, and metal chalcogenides. Introduction of carbonaceous QDs to the photocatalyst formulation can enhance the photodegradation rate to almost double as a result of increasing the light absorption efficiency. The degradation rate of hybrid materials can be significantly improved by using metal oxides nanomaterials. Metal oxide NPs are non-biodegradable material and can be replaced with biodegradable carbonaceous materials. Carbonaceous materials (0D, 1D, 2D, and 3D) forms hybrid material with NPs to improve the absorption efficiency up to 10 folds due to their better surface area and active functional groups. The interaction of pharmaceuticals with nanocomposites is due to coordination with unsaturated sites, π-π stacking, hydrogen, and electrostatic bond formation. Various variables involved in enhancing the photocatalytic degradation of pharmaceutical water include pH, Fenton reagent, catalyst dose, ultra violet/visible light exposure, oxidation-reduction potential, number of free radicals, and charge carrier recombination rate. This review provides critical information regarding the creation of functionalized nanocomposite materials to obtain complete or near to complete pharmaceutical waste degradation.
Author contributions statement
Amandeep Saroa: conception, drafting of the paper,editing, final approval of manuscript. Amrit Singh: drafting of the paper, final approval of manuscript. Neha Jindal: drafting of the paper, final approval of manuscript. Raj Kumar: drafting of the paper, final approval of manuscript. Kulvinder Singh: drafting of the paper, final approval of manuscript. Praveen Guleria: drafting of the paper, final approval of manuscript. Raj Boopathy: conception, drafting of the paper, final approval of manuscript. Vineet Kumar: conception, drafting of the paper, editing, final approval of manuscript, revising it critically for intellectual content.All authors agree to be accountable for all aspects of the work and has final approval of the version submitted.
Acknowledgments
A.S. thanks Sri Guru Teg Bahadur Khalsa College, Sri Anandpur Sahib. NJthanks DAV College, Bathinda. R.K. thanks Maharaja Agrasen University. K.S. is grateful to DAV College, Sector 10, Chandigarh. P.G. sis thankful to DAVU management for continuous support to carry out research. RBthanks Nicholls State University. V.K. gratefully acknowledge Lovely Professional University, Jalandhar for their support.
Disclosure statement
No potential conflict of interest was reported by the authors.
Data availability statement
Data sharing is not applicable to this article as no new data were created or analyzed in this study as it is a review manuscript.
Additional information
Funding
References
- Farahani B, Firouzi F, Chang V, et al. Towards fog-driven IoT eHealth: promises and challenges of IoT in medicine and healthcare. Future Gener Comput Syst. 2018 Jan;78:659–38. doi: 10.1016/j.future.2017.04.036.
- Förstner U, Wittmann GTW. Metal pollution in the aquatic environment. Berlin, Heidelberg: Springer; 1981. doi: 10.1007/978-3-642-69385-4.
- Velusamy S, Roy A, Sundaram S, et al. A review on heavy metal ions and containing dyes removal through graphene oxide-based adsorption strategies for textile wastewater treatment. Chem Rec. 2021 Jul;21(7):1570–1610. doi: 10.1002/tcr.202000153.
- Bottoni P, Caroli S, Caracciolo AB. Pharmaceuticals as priority water contaminants. Toxicol Environ Chem. 2010 Mar;92(3):549–565. doi: 10.1080/02772241003614320.
- Nageswara Rao R, Nagaraju V. An overview of the recent trends in development of HPLC methods for determination of impurities in drugs. J Pharm Biomed Anal. 2003 Oct;33(3):335–377. doi: 10.1016/S0731-7085(03)00293-0.
- Gupta A, Kumar Verma M, Kumar R. A critical review for antibiotic removal from aqueous medium using ceria-based nanostructures. Mater Today Proc. 2023. doi: 10.1016/j.matpr.2023.02.206
- Renganathan J, S IUH, Ramakrishnan K, et al. Spatio-temporal distribution of pharmaceutically active compounds in the River Cauvery and its tributaries, South India. Sci Total Environ. 2021 Dec;800:149340. doi: 10.1016/j.scitotenv.2021.149340.
- Balakrishna K, Rath A, Praveenkumarreddy Y, et al. A review of the occurrence of pharmaceuticals and personal care products in Indian water bodies. Ecotoxicol Environ Saf. 2017 Mar;137:113–120. doi: 10.1016/j.ecoenv.2016.11.014.
- Margot J, Rossi L, Barry DA, et al. A review of the fate of micropollutants in wastewater treatment plants. WIREs Water. 2015 Sep;2(5):457–487. doi: 10.1002/wat2.1090.
- Colla V, Branca TA, Rosito F, et al. Sustainable reverse osmosis application for wastewater treatment in the steel industry. J Clean Prod. 2016 Sep;130:103–115. doi: 10.1016/j.jclepro.2015.09.025.
- Ahmed SF, Mofijur M, Ahmed B, et al. Nanomaterials as a sustainable choice for treating wastewater. Environ Res. 2022 Nov;214:113807.
- AbuKhadra MR, Basyouny MG, AlHammadi AA, et al. Enhanced decontamination of levofloxacin as toxic pharmaceutical residuals from water using CaO/MgO nanorods as a promising adsorbent. Sci Rep. 2020 Sep;10(1):14828. doi: 10.1038/s41598-020-71951-6.
- Yang X, Wen L, Huang H, et al. Hydrothermal synthesis of MoS2 nanoflowers and its rapid adsorption of tetracycline. Solid State Sci. 2022 Nov;133:107014. doi: 10.1016/j.solidstatesciences.2022.107014.
- Mansi K, Kumar R, Kaur J, et al. DL-Valine assisted fabrication of quercetin loaded CuO nanoleaves through microwave irradiation method: augmentation in its catalytic and antimicrobial efficiencies. Environ NanotechnolMonitmanag. 2020 Dec;14:100306.
- Garg A, Basu S, Shetti NP, et al. 2D materials and its heterostructured photocatalysts: synthesis, properties, functionalization and applications in environmental remediation. J Environ Chem Eng. 2021 Dec;9(6):106408. doi: 10.1016/j.jece.2021.106408.
- Othman A, Vargo P, Andreescu S. Recyclable adsorbents based on Ceria nanostructures on mesoporous silica beads for the removal and recovery of phosphate from eutrophic waters. ACS Appl Nano Mater. 2019 Nov;2(11):7008–7018. doi: 10.1021/acsanm.9b01512.
- Dutta V, Sharma S, Raizada P, et al. Recent progress on bismuth-based Z-scheme semiconductor photocatalysts for energy and environmental applications. J Environ Chem Eng. 2020 Dec;8(6):104505. doi: 10.1016/j.jece.2020.104505.
- Wang H, Liao B, Lu T, et al. Enhanced visible-light photocatalytic degradation of tetracycline by a novel hollow BiOCl@CeO2 heterostructured microspheres: structural characterization and reaction mechanism. J Hazard Mater. 2020 Mar;385:121552. doi: 10.1016/j.jhazmat.2019.121552.
- Senasu T, Youngme S, Hemavibool K, et al. Sunlight-driven photodegradation of oxytetracycline antibiotic by BiVO4 photocatalyst. J Solid State Chem. 2021 May;297:122088. doi: 10.1016/j.jssc.2021.122088.
- Kalambate PK, Noiphung J, Rodthongkum N, et al. Nanomaterials-based electrochemical sensors and biosensors for the detection of non-steroidal anti-inflammatory drugs. Trends Analyt Chem. 2021 Oct;143:116403.
- Umapathi R, Venkateswara Raju C, Majid Ghoreishian S, et al. Recent advances in the use of graphitic carbon nitride-based composites for the electrochemical detection of hazardous contaminants. Coord Chem Rev. 2022 Nov;470:214708.
- Muthusankar G, Sasikumar R, Chen S-M, et al. Electrochemical synthesis of nitrogen-doped carbon quantum dots decorated copper oxide for the sensitive and selective detection of non-steroidal anti-inflammatory drug in berries. J Colloid Interface Sci. 2018 Aug;523:191–200. doi: 10.1016/j.jcis.2018.03.095.
- Ansari S, Ansari MS, Devnani H, et al. CeO2/g-C3N4 nanocomposite: a perspective for electrochemical sensing of anti-depressant drug. Sens Actuators B Chem. 2018 Nov;273:1226–1236. doi: 10.1016/j.snb.2018.06.036.
- Selvaraj M, Hai A, Banat F, et al. Application and prospects of carbon nanostructured materials in water treatment: a review. Water Proc Eng. 2020 Feb. 1; 33. Elsevier Ltd. doi: 10.1016/j.jwpe.2019.100996
- Aziz T, Farid A, Haq F, et al. Role of silica-based porous cellulose nanocrystals in improving water absorption and mechanical properties. Environ Res. 2023 Apr;222:115253.
- Azhar U, Bashir MS, Babar M, et al. Template-based textural modifications of polymeric graphitic carbon nitrides towards waste water treatment. Chemosphere. 2022 Sep;302:134792.
- Mafa PJ, Kuvarega AT, Mamba BB, et al. Photoelectrocatalytic degradation of sulfamethoxazole on g-C3N4/BiOI/EG p-n heterojunction photoanode under visible light irradiation. Appl Surf Sci. 2019;483:506–520. doi: 10.1016/j.apsusc.2019.03.281.
- Bao Y, Chen K. Novel Z-scheme BiOBr/reduced graphene oxide/protonated g-C3N4 photocatalyst: synthesis, characterization, visible light photocatalytic activity and mechanism. Appl Surf Sci. 2018;437:51–61. doi: 10.1016/j.apsusc.2017.12.075.
- You Z, Shen Q, Su Y, et al. Construction of a Z-scheme core–shell g-C3N4/MCNTs/BiOI nanocomposite semiconductor with enhanced visible-light photocatalytic activity. New J Chem. 2018;42(1):489–496. doi: 10.1039/C7NJ03623J
- Yu Z, Li F, Yang Q, et al. Nature-mimic method to fabricate polydopamine/graphitic carbon nitride for enhancing photocatalytic degradation performance. ACS Sustain Chem Eng. 2017 Sep;5(9):7840–7850. doi: 10.1021/acssuschemeng.7b01313.
- Fu J, Yu J, Jiang C, et al. G-C3N4-based heterostructured photocatalysts. Adv Energy Mater. 2018 Jan;8(3):1701503. doi: 10.1002/aenm.201701503.
- Zhang W, Zhou L, Shi J, et al. Fabrication of novel visible-light-driven AgI/g-C3N4 composites with enhanced visible-light photocatalytic activity for diclofenac degradation. J Colloid Interface Sci. 2017 Jun;496:167–176. doi: 10.1016/j.jcis.2017.02.022.
- Jiang J, Wang X, Yue C, et al. Efficient photoactivation of peroxymonosulfate by Z-scheme nitrogen-defect-rich NiCo2O4/g-C3N4 for rapid emerging pollutants degradation. J Hazard Mater. 2021 Jul;414:125528.
- Alnaggar G, Hezam A, Drmosh QA, et al. Sunlight-driven activation of peroxymonosulfate by microwave synthesized ternary MoO3/Bi2O3/g-C3N4 heterostructures for boosting tetracycline hydrochloride degradation. Chemosphere. 2021 Jun;272. doi: 10.1016/j.chemosphere.2021.129807.
- Guo F, Chen J, Zhao J, et al. Z-scheme heterojunction g-C3N4@PDA/BiOBr with biomimetic polydopamine as electron transfer mediators for enhanced visible-light driven degradation of sulfamethoxazole. Chem Eng J. 2020 Apr;386:124014.
- Raha S, Ahmaruzzaman M. Enhanced performance of a novel superparamagnetic g-C3N4/NiO/ZnO/Fe3O4 nanohybrid photocatalyst for removal of esomeprazole: effects of reaction parameters, co-existing substances and water matrices. Chem Eng J. 2020 Sep;395. doi: 10.1016/j.cej.2020.124969.
- Zhang N, Li X, Wang Y, et al. Fabrication of magnetically recoverable Fe3O4/CdS/g-C3N4 photocatalysts for effective degradation of ciprofloxacin under visible light. Ceram Int. 2020 Sep;46(13):20974–20984. doi: 10.1016/j.ceramint.2020.05.158.
- Wang L, Ma X, Huang G, et al. Construction of ternary CuO/CuFe2O4/g-C3N4 composite and its enhanced photocatalytic degradation of tetracycline hydrochloride with persulfate under simulated sunlight. J Environ Sci. 2021 Feb;112:59–70.
- Wang S, Long J, Jiang T, et al. Magnetic Fe3O4/CeO2/g-C3N4 composites with a visible-light response as a high efficiency Fenton photocatalyst to synergistically degrade tetracycline. Sep Purif Technol. 2022 Jan;278:119609.
- He T, Wu Y, Jiang C, et al. Novel magnetic Fe3O4/g-C3N4/MoO3 nanocomposites with highly enhanced photocatalytic activities: visible-light-driven degradation of tetracycline from aqueous environment. PLoS One. 2020 Aug;15(8):e0237389. doi: 10.1371/journal.pone.0237389.
- Wu Y, Zhao X, Huang S, et al. Facile construction of 2D g-C3N4 supported nanoflower-like NaBiO3 with direct Z-scheme heterojunctions and insight into its photocatalytic degradation of tetracycline. J Hazard Mater. 2021 Jul;414:125547.
- Jiang X, Lai S, Xu W, et al. Novel ternary BiOI/g-C3N4/CeO2 catalysts for enhanced photocatalytic degradation of tetracycline under visible-light radiation via double charge transfer process. J Alloys Compd. 2019 Nov;809:151804.
- Akbarzadeh R, Fung CSL, Rather RA, et al. One-pot hydrothermal synthesis of g-C3N4/Ag/AgCl/BiVO4 micro-flower composite for the visible light degradation of ibuprofen. Chem Eng J. 2018 Jun;341:248–261. doi: 10.1016/j.cej.2018.02.042.
- Kang J, Tang Y, Wang M, et al. The enhanced peroxymonosulfate-assisted photocatalytic degradation of tetracycline under visible light by g-C3N4/Na-BiVO4heterojunction catalyst and its mechanism. J Environ Chem Eng. 2021 Aug;9(4):105524. doi: 10.1016/j.jece.2021.105524.
- Zhang J, Liu X, Liu Q, et al. Z-scheme AgSCN/Ag3PO4/C3N4 heterojunction with excellent photocatalytic degradation of ibuprofen. Ceram Int. 2020 Jan;46(1):106–113. doi: 10.1016/j.ceramint.2019.08.239.
- Kong L, Wang Q, Wang Y, et al. Sustainable Cu2(OH)2CO3/g-C3N4/cellulose acetate-derived porous composite membrane for Congo red and tetracycline removal with photocatalytic self-cleaning properties under natural solar irradiation. Sustain Horiz. 2023 Mar;5:100047. doi: 10.1016/j.horiz.2023.100047.
- Qianqian Z, Tang B, Guoxin H. High photoactive and visible-light responsive graphene/titanate nanotubes photocatalysts: preparation and characterization. J Hazard Mater. 2011;198:78–86. doi: 10.1016/j.jhazmat.2011.10.012.
- Lin L, Wang H, Jiang W, et al. Comparison study on photocatalytic oxidation of pharmaceuticals by TiO2-Fe and TiO2-reduced graphene oxide nanocomposites immobilized on optical fibers. J Hazard Mater. 2017;333:162–168. doi: 10.1016/j.jhazmat.2017.02.044.
- Boruah PK, Szunerits S, Boukherroub R, et al. Magnetic Fe3O4@V2O5/rGO nanocomposite as a recyclable photocatalyst for dye molecules degradation under direct sunlight irradiation. Chemosphere. 2018;191:503–513. doi: 10.1016/j.chemosphere.2017.10.075.
- Moztahida M, Jang J, Nawaz M, et al. Effect of rGO loading on Fe 3 O 4: a visible light assisted catalyst material for carbamazepine degradation. Sci Total Environ. 2019 Jun;667:741–750. doi: 10.1016/j.scitotenv.2019.02.376.
- Shanavas S, Mohana Roopan S, Priyadharsan A, et al. Computationally guided synthesis of (2D/3D/2D) rGo/fe2o3/g-C3N4 nanostructure with improved charge separation and transportation efficiency for degradation of pharmaceutical molecules. Appl Catal, B. 2019 Oct;255:117758.
- Su G, Liu L, Liu X, et al. Magnetic Fe3O4@SiO2@BiFeO3/rGO composite for the enhanced visible-light catalytic degradation activity of organic pollutants. Ceram Int. 2021 Feb;47(4):5374–5387. doi: 10.1016/j.ceramint.2020.10.118.
- Sayadi MH, Sobhani S, Shekari H. Photocatalytic degradation of azithromycin using GO@Fe3O4/ZnO/SnO2 nanocomposites. J Clean Prod. 2019 Sep;232:127–136. doi: 10.1016/j.jclepro.2019.05.338.
- Li J, Liu K, Xue J, et al. CQDS preluded carbon-incorporated 3D burger-like hybrid ZnO enhanced visible-light-driven photocatalytic activity and mechanism implication. J Catal. 2019;369:450–461. DOI:10.1016/j.jcat.2018.11.026
- Lim SY, Shen W, Gao Z. Carbon quantum dots and their applications. Chem Soc Rev. 2015;44(1):362–381. doi: 10.1039/C4CS00269E.
- Yu H, Huang J, Jiang L, et al. Enhanced photocatalytic tetracycline degradation using N-CQDs/OV-BiOBr composites: unraveling the complementary effects between N-CQDs and oxygen vacancy. Chem Eng J. 2020 Dec;402:126187.
- Hu Z, Xie X, Li S, et al. Rational construct CQDs/BiOCOOH/uCN photocatalyst with excellent photocatalytic performance for degradation of sulfathiazole. Chem Eng J. 2021 Jan;404:126541.
- Xie X, Li S, Qi K, et al. Photoinduced synthesis of green photocatalyst Fe3O4/BiOBr/CQDs derived from corncob biomass for carbamazepine degradation: the role of selectively more CQDs decoration and Z-scheme structure. Chem Eng J. 2021 Sep;420. doi: 10.1016/j.cej.2021.129705.
- Xiang Y, Xu Z, Wei Y, et al. Carbon-based materials as adsorbent for antibiotics removal: mechanisms and influencing factors. J Environ Manage. 2019;237:128–138. DOI:10.1016/j.jenvman.2019.02.068
- Smith SC, Ahmed F, Gutierrez KM, et al. A comparative study of lysozyme adsorption with graphene, graphene oxide, and single-walled carbon nanotubes: potential environmental applications. Chem Eng J. 2014;240:147–154. doi: 10.1016/j.cej.2013.11.030.
- Fang Li M, Liu Y-G, Liu S-B, et al. Performance of magnetic graphene oxide/diethylenetriaminepentaacetic acid nanocomposite for the tetracycline and ciprofloxacin adsorption in single and binary systems. J Colloid Interface Sci. 2018 Jul;521:150–159.
- Li M, Liu Y-G, Zeng G-M, et al. Tetracycline absorbed onto nitrilotriacetic acid-functionalized magnetic graphene oxide: influencing factors and uptake mechanism. J Colloid Interface Sci. 2017;485:269–279. DOI:10.1016/j.jcis.2016.09.037
- Zhang L, Song X, Liu X, et al. Studies on the removal of tetracycline by multi-walled carbon nanotubes. Chem Eng J. 2011;178:26–33. doi:10.1016/j.cej.2011.09.127
- Li M, Liu Y-G, Liu S-B, et al. Cu(ii)-influenced adsorption of ciprofloxacin from aqueous solutions by magnetic graphene oxide/nitrilotriacetic acid nanocomposite: competition and enhancement mechanisms. Chem Eng J. 2017;319:219–228. DOI:10.1016/j.cej.2017.03.016
- Zhu X, Tsang DCW, Chen F, et al. Ciprofloxacin adsorption on graphene and granular activated carbon: kinetics, isotherms, and effects of solution chemistry. Environ Technol. 2015 Dec;36(24):3094–3102. doi: 10.1080/09593330.2015.1054316.
- Karimi-Maleh H, Shafieizadeh M, Taher MA, et al. The role of magnetite/graphene oxide nano-composite as a high-efficiency adsorbent for removal of phenazopyridine residues from water samples, an experimental/theoretical investigation. J Mol Liq. 2020 Jan;298:112040.
- Monier M, Ayad DM, Wei Y, et al. Immobilization of horseradish peroxidase on modified chitosan beads. Int j biol macromol. 2010;46(3):324–330. doi: 10.1016/j.ijbiomac.2009.12.018.
- Zeraatkar Moghaddam A, Esmaeilkhanian E, Shakourian-Fard M. Immobilizing magnetic glutaraldehyde cross-linked chitosan on graphene oxide and nitrogen-doped graphene oxide as well-dispersible adsorbents for chromate removal from aqueous solutions. Int j biol macromol. 2019;128:61–73. doi: 10.1016/j.ijbiomac.2019.01.086.
- Suri A, Khandegar V, Kaur PJ. Ofloxacin exclusion using novel HRP immobilized chitosan cross-link with graphene-oxide nanocomposite. Groundw Sustain Dev. 2021 Feb;12:100515. doi: 10.1016/j.gsd.2020.100515.
- Huang B, Liu Y, Li B, et al. Effect of Cu(II) ions on the enhancement of tetracycline adsorption by Fe3O4@SiO2-chitosan/graphene oxide nanocomposite. Carbohydr Polym. 2017 Feb;157:576–585.
- Li N, Chen J, Shi YP. Magnetic polyethyleneimine functionalized reduced graphene oxide as a novel magnetic sorbent for the separation of polar non-steroidal anti-inflammatory drugs in waters. Talanta. 2019 Jan;191:526–534. doi: 10.1016/j.talanta.2018.09.006.
- Al-Khateeb LA, Hakami W, Abdel Salam M, et al. Solid phase-fabrication of magnetically separable Fe3O4@graphene nanoplatelets nanocomposite for efficient removal of NSAIDs from wastewater. Perception of adsorption kinetics, thermodynamics, and extra-thermodynamics. Anal Chim Acta. 2022 Aug;1223. doi: 10.1016/j.aca.2022.340158.
- Song Z, Ma YL, Li CE. The residual tetracycline in pharmaceutical wastewater was effectively removed by using MnO2/graphene nanocomposite. Sci Total Environ. 2019 Feb;651:580–590. doi: 10.1016/j.scitotenv.2018.09.240.
- Ma J, Jiang Z, Cao J, et al. Enhanced adsorption for the removal of antibiotics by carbon nanotubes/graphene oxide/sodium alginate triple-network nanocomposite hydrogels in aqueous solutions. Chemosphere. 2020 Mar;242. doi: 10.1016/j.chemosphere.2019.125188.
- Agha Beygli R, Mohaghegh N, Rahimi E. Metal ion adsorption from wastewater by g-C3N4 modified with hydroxyapatite: a case study from sarcheshmeh acid mine drainage. Res Chem Intermed. 2019;45(4):2255–2268. doi: 10.1007/s11164-018-03733-9.
- Tonda S, Jo W-K. Plasmonic Ag nanoparticles decorated NiAl-layered double hydroxide/graphitic carbon nitride nanocomposites for efficient visible-light-driven photocatalytic removal of aqueous organic pollutants. Catal Today. 2018;315:213–222. doi: 10.1016/j.cattod.2017.12.019.
- Foroughi M, Ahmadi Azqhandi MH, Kakhki S. Bio-inspired, high, and fast adsorption of tetracycline from aqueous media using Fe3O4-g-CN@PEI-β-CD nanocomposite: modeling by response surface methodology (RSM), boosted regression tree (BRT), and general regression neural network (GRNN). J Hazard Mater. 2020 Apr;388:121769. doi: 10.1016/j.jhazmat.2019.121769.
- Juang R-S, Yei Y-C, Liao C-S, et al. Synthesis of magnetic Fe3O4/activated carbon nanocomposites with high surface area as recoverable adsorbents. J Taiwan Inst Chem Eng. 2018;90:51–60. DOI:10.1016/j.jtice.2017.12.005
- Mestre AS, Hesse F, Freire C, et al. Chemically activated high grade nanoporous carbons from low density renewable biomass (agave sisalana) for the removal of pharmaceuticals. J Colloid Interface Sci. 2019;536:681–693. doi: 10.1016/j.jcis.2018.10.081.
- Modesto HR, Lemos SG, dos Santos MS, et al. Activated carbon production from industrial yeast residue to boost up circular bioeconomy. Environ Sci Pollut Res. 2021;28(19):24694–24705. doi: 10.1007/s11356-020-10458-z
- Viegas RMC, Mestre AS, Mesquita E, et al. Assessing the applicability of a new carob waste-derived powdered activated carbon to control pharmaceutical compounds in wastewater treatment. Sci Total Environ. 2020;743:140791. DOI:10.1016/j.scitotenv.2020.140791
- Hameed BH, Daud FBM. Adsorption studies of basic dye on activated carbon derived from agricultural waste: hevea brasiliensis seed coat. Chem Eng J. 2008;139(1):48–55. doi: 10.1016/j.cej.2007.07.089.
- D’Cruz B, Madkour M, Amin MO, et al. Efficient and recoverable magnetic AC-Fe3O4 nanocomposite for rapid removal of promazine from wastewater. Mater Chem Phys. 2020 Jan;240:122109. doi: 10.1016/j.matchemphys.2019.122109.
- Labuto G, Carvalho AP, Mestre AS, et al. Individual and competitive adsorption of ibuprofen and caffeine from primary sewage effluent by yeast-based activated carbon and magnetic carbon nanocomposite. Sustain Chem Pharm. 2022 Sep;28:100703.
- Dada AO, Inyinbor AA, Bello OS, et al. Novel plantain peel activated carbon–supported zinc oxide nanocomposites (PPAC-ZnO-NC) for adsorption of chloroquine synthetic pharmaceutical used for COVID-19 treatment. Biomass Convers Biorefin. 2021;13(10):9181–9193. doi: 10.1007/s13399-021-01828-9.
- Miura T, Tao R, Shibata S, et al. Geometries, electronic couplings, and hole dissociation dynamics of photoinduced electron–hole pairs in polyhexylthiophene–fullerene dyads rigidly linked by Oligophenylenes. J Am Chem Soc. 2016 May;138(18):5879–5885. doi: 10.1021/jacs.5b13414.
- Ali N, Chipara D, Lozano K, et al. Polyethylene oxide—fullerene nanocomposites. Appl Surf Sci. 2017;421:220–227. doi: 10.1016/j.apsusc.2016.11.166.
- Cano Ordaz J, Chigo Anota E, Salazar Villanueva M, et al. Possibility of a magnetic [BN fullerene: B6 cluster]− nanocomposite as a vehicle for the delivery of dapsone. New J Chem. 2017;41(16):8045–8052. doi: 10.1039/C7NJ01133D.
- Herreros-López A, Carini M, Da Ros T, et al. Nanocrystalline cellulose-fullerene: novel conjugates. Carbohydr Polym. 2017;164:92–101. DOI:10.1016/j.carbpol.2017.01.068
- Elessawy NA, Elnouby M, Gouda MH, et al. Ciprofloxacin removal using magnetic fullerene nanocomposite obtained from sustainable PET bottle wastes: adsorption process optimization, kinetics, isotherm, regeneration and recycling studies. Chemosphere. 2020 Jan;239:124728.
- Trakulmututa J, Chuaicham C, Shenoy S, et al. Effect of transformation temperature toward optical properties of derived CuO/ZnO composite from Cu–zn hydroxide nitrate for photocatalytic ciprofloxacin degradation. Opt Mater (Amst). 2022 Nov;133. doi: 10.1016/j.optmat.2022.112941.
- Rosman N, Salleh WNW, Mohamed MA, et al. Constructing a compact heterojunction structure of Ag2CO3/Ag2O in-situ intermediate phase transformation decorated on ZnO with superior photocatalytic degradation of ibuprofen. Sep Purif Technol. 2020 Nov;251:117391. doi: 10.1016/j.seppur.2020.117391.
- Yentür G, Dükkancı M. Fabrication of magnetically separable plasmonic composite photocatalyst of Ag/AgBr/ZnFe2O4 for visible light photocatalytic oxidation of carbamazepine. Appl Surf Sci. 2020 Apr;510. doi: 10.1016/j.apsusc.2020.145374.
- Khan M, Fung CSL, Kumar A, et al. Magnetically separable BiOBr/Fe3O4@SiO2 for visible-light-driven photocatalytic degradation of ibuprofen: mechanistic investigation and prototype development. J Hazard Mater. 2019 Mar;365:733–743. doi: 10.1016/j.jhazmat.2018.11.053.
- Thiruppathi M, Senthil Kumar P, Devendran P, et al. Ce@TiO2 nanocomposites: an efficient, stable and affordable photocatalyst for the photodegradation of diclofenac sodium. J Alloys Compd. 2018 Feb;735:728–734. doi: 10.1016/j.jallcom.2017.11.139.
- Payan A, Akbar Isari A, Gholizade N. Catalytic decomposition of sulfamethazine antibiotic and pharmaceutical wastewater using Cu-TiO2@functionalized SWCNT ternary porous nanocomposite: influential factors, mechanism, and pathway studies. Chem Eng J. 2019 Apr;361:1121–1141. doi: 10.1016/j.cej.2018.12.118.
- Li S, Wang Z, Zhang X, et al. Preparation of magnetic nanosphere/nanorod/nanosheet-like Fe3O4/Bi2S3/BiOBr with enhanced (0 0 1) and (1 1 0) facets to photodegrade diclofenac and ibuprofen under visible LED light irradiation. Chemical Engineering Journal. 2019 Dec;378:122169. doi: 10.1016/j.cej.2019.122169.
- Silvestri S, Ferreira CD, Oliveira V, et al. Synthesis of PPy-ZnO composite used as photocatalyst for the degradation of diclofenac under simulated solar irradiation. J Photochem Photobiol A Chem. 2019 Apr;375:261–269. doi: 10.1016/j.jphotochem.2019.02.034.
- Ahmadpour N, Sayadi MH, Sobhani S, et al. Photocatalytic degradation of model pharmaceutical pollutant by novel magnetic TiO2@ZnFe2O4/Pd nanocomposite with enhanced photocatalytic activity and stability under solar light irradiation. J Environ Manage. 2020 Oct;271. doi: 10.1016/j.jenvman.2020.110964.
- Mrotek E, Dudziak S, Malinowska I, et al. Improved degradation of etodolac in the presence of core-shell ZnFe2O4/SiO2/TiO2 magnetic photocatalyst. Sci Total Environ. 2020 Jul;724. doi: 10.1016/j.scitotenv.2020.138167.
- Liu K, Tong Z, Muhammad Y, et al. Synthesis of sodium dodecyl sulfate modified BiOBr/magnetic bentonite photocatalyst with three-dimensional parterre like structure for the enhanced photodegradation of tetracycline and ciprofloxacin. Chem Eng J. 2020 May;388:124374.
- Nasseh N, Al-Musawi TJ, Miri MR, et al. A comprehensive study on the application of FeNi3@SiO2@ZnO magnetic nanocomposites as a novel photo-catalyst for degradation of tamoxifen in the presence of simulated sunlight. Environ Pollut. 2020 Jun;261. doi: 10.1016/j.envpol.2020.114127.
- Fernández L, Gamallo M, González-Gómez MA, et al. Insight into antibiotics removal: exploring the photocatalytic performance of a Fe 3 O 4/ZnO nanocomposite in a novel magnetic sequential batch reactor. J Environ Manage. 2019 May;237:595–608.
- Ahmadpour N, Sayadi MH, Sobhani S, et al. A potential natural solar light active photocatalyst using magnetic ZnFe2O4 @ TiO2/Cu nanocomposite as a high performance and recyclable platform for degradation of naproxen from aqueous solution. J Clean Prod. 2020 Sep;268. doi: 10.1016/j.jclepro.2020.122023.
- Abdel-Wahab AM, Al-Shirbini AS, Mohamed O, et al. Photocatalytic degradation of paracetamol over magnetic flower-like TiO2/Fe2O3 core-shell nanostructures. J Photochem Photobiol A Chem. 2017 Oct;347:186–198. doi: 10.1016/j.jphotochem.2017.07.030.
- Xu G, Du M, Li T, et al. Facile synthesis of magnetically retrievable Fe3O4/BiVO4/CdS heterojunction composite for enhanced photocatalytic degradation of tetracycline under visible light. Sep Purif Technol. 2021 Nov;275. doi: 10.1016/j.seppur.2021.119157.
- Liu L, Su G, Liu X, et al. Fabrication of magnetic core–shell Fe3O4@SiO2@Bi2O2CO3–sepiolite microspheres for the high-efficiency visible light catalytic degradation of antibiotic wastewater. Environ Technol Innov. 2021 May;22:101436.
- Wang B, Lv X-L, Feng D, et al. Highly stable Zr(IV)-based metal–organic frameworks for the detection and removal of antibiotics and organic explosives in water. J Am Chem Soc. 2016 May;138(19):6204–6216. doi: 10.1021/jacs.6b01663.
- Wu M-X, Yang Y-W. Metal–organic framework (MOF)-based drug/cargo delivery and cancer therapy. Adv Mater. 2017;29(23):1606134. doi: 10.1002/adma.201606134.
- Dhakshinamoorthy A, Li Z, Garcia H. Catalysis and photocatalysis by metal organic frameworks. Chem Soc Rev. 2018;47(22):8134–8172. doi: 10.1039/C8CS00256H.
- Keskin S, Sholl DS. Screening metal−Organic framework materials for membrane-based methane/carbon dioxide separations. J Phys Chem C. 2007;111(38):14055–14059. doi: 10.1021/jp075290l.
- Wu C, Xiong Z, Li C, et al. Zeolitic imidazolate metal organic framework ZIF-8 with ultra-high adsorption capacity bound tetracycline in aqueous solution. RSC Adv. 2015;5(100):82127–82137. doi: 10.1039/C5RA15497A.
- Wu G, Ma J, Li S, et al. Magnetic copper-based metal organic framework as an effective and recyclable adsorbent for removal of two fluoroquinolone antibiotics from aqueous solutions. J Colloid Interface Sci. 2018;528:360–371. DOI:10.1016/j.jcis.2018.05.105
- Yan J, Chen Z, Ji H, et al. Construction of a 2D graphene-like MoS2/C3N4 heterojunction with enhanced visible-light photocatalytic activity and photoelectrochemical activity. Chem Eur J. 2016 Mar;22(14):4764–4773. doi: 10.1002/chem.201503660.
- Kobielska PA, Howarth AJ, Farha OK, et al. Metal–organic frameworks for heavy metal removal from water. Coord Chem Rev. 2018;358:92–107. doi: 10.1016/j.ccr.2017.12.010.
- Zhang W, Hu Y, Ge J, et al. A Facile and general coating approach to moisture/Water-resistant metal–organic frameworks with intact porosity. J Am Chem Soc. 2014 Dec;136(49):16978–16981. doi: 10.1021/ja509960n.
- Zhuo N, Lan Y, Yang W, et al. Adsorption of three selected pharmaceuticals and personal care products (PPCPs) onto MIL-101(Cr)/natural polymer composite beads. Sep Purif Technol. 2017;177:272–280. DOI:10.1016/j.seppur.2016.12.041
- Xiong W, Zeng G, Yang Z, et al. Adsorption of tetracycline antibiotics from aqueous solutions on nanocomposite multi-walled carbon nanotube functionalized MIL-53(Fe) as new adsorbent. Sci Total Environ. 2018 Jun;627:235–244.
- Xiong W, Zeng Z, Li X, et al. Multi-walled carbon nanotube/amino-functionalized MIL-53(Fe) composites: remarkable adsorptive removal of antibiotics from aqueous solutions. Chemosphere. 2018 Nov;210:1061–1069.
- Sarker M, Song JY, Jhung SH. Adsorptive removal of anti-inflammatory drugs from water using graphene oxide/metal-organic framework composites. Chem Eng J. 2018 Mar;335:74–81. doi: 10.1016/j.cej.2017.10.138.
- Wei F, Zhang H, Ren Q, et al. Removal of organic contaminants from wastewater with GO/MOFs composites. PLoS One. 2021 Jul;16(7):e0253500. doi: 10.1371/journal.pone.0253500.
- Bayazit ŞS, Kurtulbaş E, Bilgin M, et al. Decontamination of endocrine disruptors from water by graphene nanoplatelet/UiO-66 nanocomposites. Environ NanotechnolMonitmanag. 2022 Dec;18. doi: 10.1016/j.enmm.2022.100733.
- Song F, Cao S, Liu Z, et al. Different decorated ZIF-67 adsorption performance towards methamphetamine revealed by theoretical and experimental investigations. J Mol Liq. 2022 Oct;364. doi: 10.1016/j.molliq.2022.119950.
- Ling Yu L, Cao W, Chuan Wu S, et al. Removal of tetracycline from aqueous solution by MOF/graphite oxide pellets: preparation, characteristic, adsorption performance and mechanism. Ecotoxicol Environ Saf. 2018 Nov;164:289–296. doi: 10.1016/j.ecoenv.2018.07.110.
- Jia X, Li S, Wang Y, et al. Adsorption behavior and mechanism of sulfonamide antibiotics in aqueous solution on a novel MIL-101(Cr)@GO composite. J Chem Eng Data. 2019 Mar;64(3):1265–1274. doi: 10.1021/acs.jced.8b01152.
- Wang K, Wu J, Zhu M, et al. Highly effective pH-universal removal of tetracycline hydrochloride antibiotics by UiO-66-(COOH)2/GO metal–organic framework composites. J Solid State Chem. 2020 Apr;284. doi: 10.1016/j.jssc.2020.121200.
- Jin J, Yang Z, Xiong W, et al. Cu and Co nanoparticles co-doped MIL-101 as a novel adsorbent for efficient removal of tetracycline from aqueous solutions. Sci Total Environ. 2019 Feb;650:408–418.
- Van Tran T, Nguyen DTC, Nguyen TT, et al. High performance of Mn2(BDC)2(DMF)2-derived MnO@C nanocomposite as superior remediator for a series of emergent antibiotics. J Mol Liq. 2020 Jun;308:113038. doi: 10.1016/j.molliq.2020.113038.
- Van Tran T, Nguyen DTC, Nguyen TT, et al. Metal-organic framework HKUST-1-based Cu/Cu2O/CuO@C porous composite: rapid synthesis and uptake application in antibiotics remediation. Water Proc Eng. 2020 Aug;36:101319. doi: 10.1016/j.jwpe.2020.101319.
- Naeimi S, Faghihian H. Application of novel metal organic framework, MIL-53(Fe) and its magnetic hybrid: for removal of pharmaceutical pollutant, doxycycline from aqueous solutions. Environ Toxicol Pharmacol. 2017 Jul;53:121–132. doi: 10.1016/j.etap.2017.05.007.
- Ying Zhang Y, Liu Q, Yang C, et al. Magnetic aluminum-based metal organic framework as a novel magnetic adsorbent for the effective removal of minocycline from aqueous solutions. Environ Pollut. 2019 Dec;255. doi: 10.1016/j.envpol.2019.113226.
- Bayazit ŞS, Danalıoğlu ST, Abdel Salam M, et al. Preparation of magnetic MIL-101 (Cr) for efficient removal of ciprofloxacin. Environ Sci Pollut Res. 2017 Nov;24(32):25452–25461. doi: 10.1007/s11356-017-0121-0.
- Moradi SE, Haji Shabani AM, Dadfarnia S, et al. Effective removal of ciprofloxacin from aqueous solutions using magnetic metal–organic framework sorbents: mechanisms, isotherms and kinetics. J Iran Chem Soc. 2016 Sep;13(9):1617–1627. doi: 10.1007/s13738-016-0878-y.
- Duan H, Hu X, Sun Z. Magnetic zeolite imidazole framework material-8 as an effective and recyclable adsorbent for removal of ceftazidime from aqueous solution. J Hazard Mater. 2020 Feb;384. doi: 10.1016/j.jhazmat.2019.121406.
- Li C, Zhang X, Wen S, et al. Interface engineering of zeolite imidazolate framework−8 on two-dimensional al−metal−organic framework nanoplates enhancing performance for simultaneous capture and sensing tetracyclines. J Hazard Mater. 2020 Aug;395:122615.
- Álvarez-Torrellas S, Ribeiro RS, Gomes HT, et al. Removal of antibiotic compounds by adsorption using glycerol-based carbon materials. Chem Eng J. 2016;296:277–288. doi: 10.1016/j.cej.2016.03.112.
- Tian N, Jia Q, Su H, et al. The synthesis of mesostructured NH2-MIL-101(Cr) and kinetic and thermodynamic study in tetracycline aqueous solutions. J Porous Mater. 2016;23(5):1269–1278. doi: 10.1007/s10934-016-0186-z
- Hu T, Lv H, Shan S, et al. Porous structured MIL-101 synthesized with different mineralizers for adsorptive removal of oxytetracycline from aqueous solution. RSC Adv. 2016;6(77):73741–73747. doi: 10.1039/C6RA11684A
- Chen C, Chen D, Xie S, et al. Adsorption behaviors of organic micropollutants on zirconium metal–organic framework UiO-66: analysis of surface interactions. ACS Appl Mater Interfaces. 2017 Nov;9(46):41043–41054. doi: 10.1021/acsami.7b13443.
- Choi K-J, Kim S-G, Kim S-H. Removal of antibiotics by coagulation and granular activated carbon filtration. J Hazard Mater. 2008;151(1):38–43. doi: 10.1016/j.jhazmat.2007.05.059.
- Ahmed MB, Zhou JL, Ngo HH, et al. Progress in the biological and chemical treatment technologies for emerging contaminant removal from wastewater: a critical review. J Hazard Mater. 2017;323:274–298. doi: 10.1016/j.jhazmat.2016.04.045.
- Lai C, Zhang M, Li B, et al. Fabrication of CuS/BiVO4 (0 4 0) binary heterojunction photocatalysts with enhanced photocatalytic activity for ciprofloxacin degradation and mechanism insight. Chem Eng J. 2019;358:891–902. DOI:10.1016/j.cej.2018.10.072
- Subudhi S, Paramanik L, Sultana S, et al. A type-II interband alignment heterojunction architecture of cobalt titanate integrated UiO-66-NH2: a visible light mediated photocatalytic approach directed towards norfloxacin degradation and green energy (hydrogen) evolution. J Colloid Interface Sci. 2020 May;568:89–105. doi: 10.1016/j.jcis.2020.02.043.
- Askari N, Beheshti M, Mowla D, et al. Fabrication of CuWO4/Bi2S3/ZIF67 MOF: a novel double Z-scheme ternary heterostructure for boosting visible-light photodegradation of antibiotics. Chemosphere. 2020 Jul;251. doi: 10.1016/j.chemosphere.2020.126453.
- Wang Z, Wang H, Zeng Z, et al. Metal-organic frameworks derived Bi2O2CO3/porous carbon nitride: a nanosized Z-scheme systems with enhanced photocatalytic activity. Appl Catal, B. 2020 Jun;267:118700.
- Wang C, Xue Y, Wang P, et al. Effects of water environmental factors on the photocatalytic degradation of sulfamethoxazole by AgI/UiO-66 composite under visible light irradiation. J Alloys Compd. 2018 Jun;748:314–322. doi: 10.1016/j.jallcom.2018.03.129.
- Deng L, Yin D, Khaing KK, et al. The facile boosting sunlight-driven photocatalytic performance of a metal–organic-framework through coupling with Ag 2 S nanoparticles. New J Chem. 2020 Aug;44(29):12568–12578. doi: 10.1039/d0nj02030c.
- Zhang S, Wang Y, Cao Z, et al. Simultaneous enhancements of light-harvesting and charge transfer in UiO-67/CdS/rGO composites toward ofloxacin photo-degradation. Chem Eng J. 2020 Feb;381:122771.
- Chen WQ, Li L-Y, Li L, et al. MoS2/ZIF-8 hybrid materials for environmental catalysis: solar-driven antibiotic-degradation engineering. Eng. 2019 Aug;5(4):755–767. doi: 10.1016/j.eng.2019.02.003.
- Gao Y, Lu J, Xia J, et al. In situ synthesis of defect-engineered MOFs as a photoregenerable catalytic adsorbent: understanding the effect of LML, adsorption behavior, and photoreaction process. ACS Appl Mater Interfaces. 2020 Mar;12(11):12706–12716. doi: 10.1021/acsami.9b21122.
- Gao Y, Wu J, Wang J, et al. A novel multifunctional p-type semiconductor@MOFs nanoporous platform for simultaneous sensing and photodegradation of tetracycline. ACS Appl Mater Interfaces. 2020 Mar;12(9):11036–11044. doi: 10.1021/acsami.9b23314.
- Liu N, Shang Q, Gao K, et al. Construction of ZnO/ZIF-9 heterojunction photocatalyst: enhanced photocatalytic performance and mechanistic insight. New J Chem. 2020 Apr;44(16):6384–6393. doi: 10.1039/d0nj00510j.
- Li R, Li W, Jin C, et al. Fabrication of ZIF-8@TiO2 micron composite via hydrothermal method with enhanced absorption and photocatalytic activities in tetracycline degradation. J Alloys Compd. 2020 Jun;825:154008. doi: 10.1016/j.jallcom.2020.154008.
- He L, Dong Y, Zheng Y, et al. A novel magnetic MIL-101(Fe)/TiO2 composite for photo degradation of tetracycline under solar light. J Hazard Mater. 2019 Jan;361:85–94. doi: 10.1016/j.jhazmat.2018.08.079.
- Li S, Cui J, Wu X, et al. Rapid in situ microwave synthesis of Fe3O4@MIL-100(Fe) for aqueous diclofenac sodium removal through integrated adsorption and photodegradation. J Hazard Mater. 2019 Jul;373:408–416. doi: 10.1016/j.jhazmat.2019.03.102.
- Rasheed HU, Lv X, Zhang S, et al. Ternary MIL-100(Fe)@Fe3O4/CA magnetic nanophotocatalysts (MNPCs): magnetically separable and Fenton-like degradation of tetracycline hydrochloride. Adv Powder Technol. 2018 Dec;29(12):3305–3314. doi: 10.1016/j.apt.2018.09.011.
- Zhang XW, Wang F, Wang CC, et al. Photocatalysis activation of peroxodisulfate over the supported Fe3O4 catalyst derived from MIL-88A(Fe) for efficient tetracycline hydrochloride degradation. Chem Eng J. 2021 Dec;426: doi: 10.1016/j.cej.2021.131927
- Doan VD, Huynh BA, Le Pham HA, et al. Cu2O/Fe3O4/MIL-101(Fe) nanocomposite as a highly efficient and recyclable visible-light-driven catalyst for degradation of ciprofloxacin. Environ Res. 2021 Oct;201:111593. doi: 10.1016/j.envres.2021.111593.
- Bagherzadeh SB, Kazemeini M, Mahmoodi NM. Preparation of novel and highly active magnetic ternary structures (metal-organic framework/cobalt ferrite/graphene oxide) for effective visible-light-driven photocatalytic and photo-Fenton-like degradation of organic contaminants. J Colloid Interface Sci. 2021 Nov;602:73–94. doi: 10.1016/j.jcis.2021.05.181.
- Liu N, Wang J, Wu J, et al. Magnetic Fe3O4@MIL-53(Fe) nanocomposites derived from MIL-53(Fe) for the photocatalytic degradation of ibuprofen under visible light irradiation. Mater Res Bull. 2020 Dec;132:111000.
- Lv SW, Liu J-M, Zhao N, et al. MOF-derived CoFe2O4/Fe2O3 embedded in g-C3N4 as high-efficient Z-scheme photocatalysts for enhanced degradation of emerging organic pollutants in the presence of persulfate. Sep Purif Technol. 2020 Dec;253:117413.
- He W, Li Z, Lv S, et al. Facile synthesis of Fe3O4@MIL-100(Fe) towards enhancing photo-Fenton like degradation of levofloxacin via a synergistic effect between Fe3O4 and MIL-100(Fe). Chem Eng J. 2021 Apr;409:128274.
- Shahzad A, Rasool K, Nawaz M, et al. Heterostructural TiO2/Ti3C2Tx (MXene) for photocatalytic degradation of antiepileptic drug carbamazepine. Chem Eng J. 2018 Oct;349:748–755.
- Yi X, Yuan J, Tang H, et al. Embedding few-layer Ti3C2Tx into alkalized g-C3N4 nanosheets for efficient photocatalytic degradation. J Colloid Interface Sci. 2020 Jul;571:297–306.
- Diao Y, Yan M, Li X, et al. In-situ grown of g-C3N4/Ti3C2/TiO2 nanotube arrays on Ti meshes for efficient degradation of organic pollutants under visible light irradiation. Colloids Surf A Physicochem Eng Asp. 2020 Jun;594:124511.
- Wu Z, Liang Y, Yuan X, et al. Mxene Ti3C2 derived Z–scheme photocatalyst of graphene layers anchored TiO2/g–C3N4 for visible light photocatalytic degradation of refractory organic pollutants. Chem Eng J. 2020 Aug;394:124921.
- Cui C, Guo R, Xiao H, et al. Bi2WO6/Nb2CTx MXene hybrid nanosheets with enhanced visible-light-driven photocatalytic activity for organic pollutants degradation. Appl Surf Sci. 2020 Mar;505:144595.
- Ma Y, Lv X, Xiong D, et al. Catalytic degradation of ranitidine using novel magnetic Ti3C2-based MXene nanosheets modified with nanoscale zero-valent iron particles. Appl Catal, B. 2021 May;284. doi: 10.1016/j.apcatb.2020.119720.
- Cao Y, Fang Y, Lei X, et al. Fabrication of novel CuFe2O4/MXene hierarchical heterostructures for enhanced photocatalytic degradation of sulfonamides under visible light. J Hazard Mater. 2020 Apr;387:122021.
- Kumar A, Majithia P, Choudhary P, et al. Mxene coupled graphitic carbon nitride nanosheets based plasmonic photocatalysts for removal of pharmaceutical pollutant. Chemosphere. 2022 Dec;308:136297.
- Zou X, Zhao X, Zhang J, et al. Photocatalytic degradation of ranitidine and reduction of nitrosamine dimethylamine formation potential over MXene–Ti3C2/MoS2 under visible light irradiation. J Hazard Mater. 2021 Jul;413:125424. doi: 10.1016/j.jhazmat.2021.125424.
- Du Z, Feng L, Guo Z, et al. Ultrathin h-BN/Bi2MoO6 heterojunction with synergetic effect for visible-light photocatalytic tetracycline degradation. J Colloid Interface Sci. 2021 May;589:545–555.
- Lin L, Jiang W, Nasr M, et al. Enhanced visible light photocatalysis by TiO2–BN enabled electrospinning of nanofibers for pharmaceutical degradation and wastewater treatment. Photochem Photobiol Sci. 2019;18(12):2921–2930. doi: 10.1039/c9pp00304e.
- Liu N, Wang J, Tian M, et al. Boron nitride nanosheets decorated MIL-53(Fe) for efficient synergistic ibuprofen photocatalytic degradation by persulfate activation. J Colloid Interface Sci. 2021 Dec;603:270–281.
- Cai H, Wang J, Du Z, et al. Construction of novel ternary MoSe2/ZnO/p-BN photocatalyst for efficient ofloxacin degradation under visible light. Colloids Surf A Physicochem Eng Asp. 2023 Apr;663:131050.
- Wu Y, Jin X, Liu H, et al. Synergistic effects of boron nitride quantum dots and reduced ultrathin g-C3N4: dual-channel carrier transfer and band structure regulation boost the photodegradation of fluoroquinolone. Sep Purif Technol. 2022 Dec;303. doi: 10.1016/j.seppur.2022.122185.
- Che H, Che G, Zhou P, et al. Yeast-derived carbon sphere as a bridge of charge carriers towards to enhanced photocatalytic activity of 2D/2D Cu 2 WS 4/g-C 3 N 4 heterojunction. J Colloid Interface Sci. 2019 Jun;546:262–275. doi: 10.1016/j.jcis.2019.03.080.
- Li J, Xia Z, Ma D, et al. Improving photocatalytic activity by construction of immobilized Z-scheme CdS/Au/TiO2 nanobelt photocatalyst for eliminating norfloxacin from water. J Colloid Interface Sci. 2021 Mar;586:243–256.
- Peng D, Wang Y, Shi H, et al. Fabrication of novel Cu2WS4/NiTiO3 heterostructures for efficient visible-light photocatalytic hydrogen evolution and pollutant degradation. J Colloid Interface Sci. 2022 May;613:194–206.
- Khan A, Danish M, Alam U, et al. Facile synthesis of a Z-Scheme ZnIn2S4/MoO3 heterojunction with enhanced photocatalytic activity under visible light irradiation. ACS Omega. 2020 Apr;5(14):8188–8199. doi: 10.1021/acsomega.0c00446.
- Ganguly P, Mathew S, Clarizia L, et al. Ternary metal chalcogenide heterostructure (AgIns 2 –TiO 2) nanocomposites for visible light photocatalytic applications. ACS Omega. 2020 Jan;5(1):406–421. doi: 10.1021/acsomega.9b02907.
- Raheem I, Mubarak NM, Karri RR, et al. Rapid growth of MXene-based membranes for sustainable environmental pollution remediation. Chemosphere. 2023;311:137056. DOI:10.1016/j.chemosphere.2022.137056
- Cao S, Shen B, Tong T, et al. 2D/2D heterojunction of ultrathin MXene/Bi2WO6 nanosheets for improved photocatalytic CO2 reduction. Adv Funct Mater. 2018 May;28(21):1800136. doi: 10.1002/adfm.201800136.
- Wang H, Zhang L, Chen Z, et al. Semiconductor heterojunction photocatalysts: design, construction, and photocatalytic performances. Chem Soc Rev. 2014;43(15):5234–5244. doi: 10.1039/C4CS00126E
- Beydoun D, Amal R, Low G, et al. Role of nanoparticles in photocatalysis. J Nanopart Res. 1999;1(4):439–458. doi: 10.1023/A:1010044830871.
- Bagheri S, TermehYousefi A, Do T-O. Photocatalytic pathway toward degradation of environmental pharmaceutical pollutants: structure, kinetics and mechanism approach. Catal Sci Technol. 2017;7(20):4548–4569. doi: 10.1039/C7CY00468K.
- Liu H, Yang C, Jin X, et al. One-pot hydrothermal synthesis of MXene Ti3C2/TiO2/BiOCl ternary heterojunctions with improved separation of photoactivated carries and photocatalytic behavior toward elimination of contaminants. Colloids Surf A Physicochem Eng Asp. 2020 Oct;603. doi: 10.1016/j.colsurfa.2020.125239.
- Zhang Z, Penev ES, Yakobson BI. Two-dimensional boron: structures, properties and applications. Chem Soc Rev. 2017;46(22):6746–6763. doi: 10.1039/C7CS00261K.
- Auwärter W. Hexagonal boron nitride monolayers on metal supports: versatile templates for atoms, molecules and nanostructures. Surf Sci Rep. 2019;74(1):1–95. doi: 10.1016/j.surfrep.2018.10.001.
- Chen L, Zhou M, Luo Z, et al. Template-free synthesis of carbon-doped boron nitride nanosheets for enhanced photocatalytic hydrogen evolution. Appl Catal, B. 2019;241:246–255. doi: 10.1016/j.apcatb.2018.09.034.
- Lei W, Liu D, Chen Y. Highly crumpled boron nitride nanosheets as adsorbents: scalable solvent-less production. Adv Mater Interfaces. 2015 Feb;2(3):1400529. doi: 10.1002/admi.201400529.
- Han R, Liu F, Wang X, et al. Functionalised hexagonal boron nitride for energy conversion and storage. J Mater Chem A Mater. 2020;8(29):14384–14399. doi: 10.1039/D0TA05008C.
- Gonzalez Ortiz D, Pochat-Bohatier C, Cambedouzou J, et al. Inverse pickering emulsion stabilized by exfoliated hexagonal-boron nitride (h-BN). Langmuir. 2017 Nov;33(46):13394–13400. doi: 10.1021/acs.langmuir.7b03324.
- Yu S, Wang X, Pang H, et al. Boron nitride-based materials for the removal of pollutants from aqueous solutions: a review. Chem Eng J. 2018;333:343–360. doi:10.1016/j.cej.2017.09.163
- Ejeromedoghene O, Nnyia M, Okoye C, et al. Environmental decontamination using transition metal dichalcogenides based materials: a review. J Mater & Environ Sustain Res. 2022 Mar;2(1):1–18. doi: 10.55455/jmesr.2022.001.
- Jing D, Liu M, Chen Q, et al. Efficient photocatalytic hydrogen production under visible light over a novel W-based ternary chalcogenide photocatalyst prepared by a hydrothermal process. Int J Hydrogen Energy. 2010;35(16):8521–8527. doi: 10.1016/j.ijhydene.2010.04.170.
- Goyal A, Aggarwal D, Kapoor S, et al. A comprehensive experimental and theoretical study on BN nanosheets for the adsorption of pharmaceutical drugs. New J Chem. 2020 Mar;44(10):3985–3997. doi: 10.1039/c9nj06029d.
- Li S, Liu F, Su Y, et al. Luffa sponge-derived hierarchical meso/macroporous boron nitride fibers as superior sorbents for heavy metal sequestration. J Hazard Mater. 2019;378:120669. doi:10.1016/j.jhazmat.2019.05.062
- Song Q, Fang Y, Liu Z, et al. The performance of porous hexagonal BN in high adsorption capacity towards antibiotics pollutants from aqueous solution. Chem Eng J. 2017;325:71–79. doi:10.1016/j.cej.2017.05.057
- Han L, Khalil AME, Wang J, et al. Graphene-boron nitride composite aerogel: a high efficiency adsorbent for ciprofloxacin removal from water. Sep Purif Technol. 2022 Jan;278:119605.
- Xu M, Huang C, Lu J, et al. Optimizing adsorption of 17α-ethinylestradiol from water by magnetic mxene using response surface methodology and adsorption kinetics, isotherm, and thermodynamics studies. Molecules. 2021 Jun;26(11):3150. doi: 10.3390/molecules26113150.
- Data Bridge Market Research. Global nanotechnology market-industry trends and forecast to 2030. March 2023. 350. [cited 2023 May 14].
- Perkins R Here’s a Way To Produce Nanomaterials On a Larger Scale. University of Southern California news. February 24, 2016. [cited 2023 May 10].
- Petel BE, Van Allsburg KM, Baddour FG. Cost‐responsive optimization of nickel nanoparticle synthesis. Adv Sustainable Syst. 2023;2300030. doi: 10.1002/adsu.202300030
- Pandey G, Jain P. Assessing the nanotechnology on the grounds of costs, benefits, and risks. Beni-Suef Univ J Basic Appl Sci. 2020;9(1):1–10. doi: 10.1186/s43088-020-00085-5.
- Mordor Intelligence Research & Advisory. Nanomaterials Market Size & Share Analysis - Growth Trends & Forecasts (2023 - 2028). Mordor Intelligence. 2023 [cited 2023 Sept 23]. Available from: https://www.mordorintelligence.com/industry-reports/nanomaterials-market
- Wilkinson JL, Boxall AB, Kolpin DW, et al. Pharmaceutical pollution of the world’s rivers. Proc Nat Acad Sci. 2022;119(8):e2113947119. doi: 10.1073/pnas.2113947119.
- UN World Water Development Report. UNESCO. Accessed on 30 April 2023. [cited 2023 May 10]. Available from: https://www.unesco.org/reports/wwdr/2023/en
- Murray CJ, Ikuta KS, Sharara F, et al. Global burden of bacterial antimicrobial resistance in 2019: a systematic analysis. Lancet. 2022;399(10325):629–655. doi: 10.1016/S0140-6736(21)02724-0.
- Larsson DJ, Flach CF. Antibiotic resistance in the environment. Nature Rev Microbiol. 2022;20(5):257–269. doi: 10.1038/s41579-021-00649-x.
- Malik S, Dhasmana A, Preetam S, et al. Exploring microbial-based green nanobiotechnology for wastewater remediation: a sustainable strategy. Nanomaterials. 2022;12(23):4187. doi: 10.3390/nano12234187.
- Neha R, Adithya S, Jayaraman RS, et al. Nano-adsorbents an effective candidate for removal of toxic pharmaceutical compounds from aqueous environment: a critical review on emerging trends. Chemosphere. 2021;272:129852. doi: 10.1016/j.chemosphere.2021.129852.
- Poornima S, Manikandan S, Karthik V, et al. Emerging nanotechnology based advanced techniques for wastewater treatment. Chemosphere. 2022;303:135050. doi: 10.1016/j.chemosphere.2022.135050.
- Jain K, Patel AS, Pardhi VP, et al. Nanotechnology in wastewatermanagement: a new paradigm towards wastewater treatment. Molecules. 2021;26:1797. doi: 10.3390/molecules26061797.
- Ying S, Guan Z, Ofoegbu PC, et al. Green synthesis of nanoparticles: Current developments and limitations. Environ Technol Innov. 2022;26:102336. doi: 10.1016/j.eti.2022.102336.
- Gautam A, Sharma P, Ashokhan S, et al. Magnesium oxide nanoparticles improved vegetative growth and enhanced productivity, biochemical potency and storage stability of harvested mustard seeds. Environ Res. 2023;29:116023. doi: 10.1016/j.envres.2023.116023.
- Grimaldi F, Pucciarelli M, Gavriilidis A, et al. Anticipatory life cycle assessment of gold nanoparticles production: comparison of milli-continuous flow and batch synthesis. J Clean Prod. 2020;269:122335. doi: 10.1016/j.jclepro.2020.122335.
- Ross A, Muñoz M, Rotstein BH, et al. A low cost and open access system for rapid synthesis of large volumes of gold and silver nanoparticles. Sci Rep. 2021;11(1):1–10. doi: 10.1038/s41598-021-84896-1.
- Swisher JH, Jibril L, Petrosko SH, et al. Nanoreactors for particle synthesis. Nature Rev Mater. 2022;7(6):428–448. doi: 10.1038/s41578-021-00402-z.