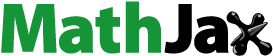
ABSTRACT
The versatility of a well-known fibrous crop, Hibiscus cannabinus (kenaf) is still relatively new to many. Kenaf’s potential applications, which can be extended even into critical industries such as pharmaceutical and food industries, have always been overshadowed by its traditionally grown fiber. Therefore, this study aimed to venture into the biotechnological approach in reaping the benefits of kenaf through plant cell suspension culture to maximize the production of kenaf callus biomass (KCB) and exopolysaccharide (EPS), which is deemed to be more sustainable. A growth curve was established which indicates that cultivating kenaf callus in suspension culture for 22 days gives the highest KCB (9.09 ± 1.2 g/L) and EPS (1.1 ± 0.02 g/L). Using response surface methodology (RSM), it was found that sucrose concentration, agitation speed, and naphthalene acetic acid (NAA) concentration can affect the production of KCB and EPS significantly (p < 0.05) while 2,4-dichlorophenoxy acetic acid (2,4-D) was deemed insignificant. To maximize the final yield of KCB and EPS, the final optimized variables are 50 g/L sucrose, 147.02 rpm, and 2 mg/L of NAA. To conclude, the optimized parameters for the cell suspension culture of kenaf callus serve as the blueprint for any sustainable large-scale production in the future and provide an alternative cultivating method to kenaf traditional farming.
GRAPHICAL ABSTRACT

Highlights
The optimized cell cultivation for plant kenaf callus is 22 days
Sucrose, agitation and NAA concentration stimulates the production of KCB and EPS
Highest KCB and EPS was generated at 13.41 g/L and 1.86 g/L, respectively
Maximum production blueprint for KCB and EPS require 50 g/L sucrose, 2 mg/L of NAA and 147.02 rpm
1. Introduction
Hibiscus cannabinus or kenaf is an annual dicotyledonous crop that is typically cultivated in areas blessed with high rainfall and abundant solar radiation such as in China, Malaysia, Thailand, and India mainly for its good and dependable natural fiber source [Citation1–3]. The natural multipurpose kenaf fiber can be retrieved within 4–5 months of the cultivation period directly from its dry stem material [Citation4]. Traditionally, kenaf is being widely used in various industries such as textiles, paper and pulp, absorption materials, and bio-composites [Citation5].
Recent findings have suggested that the applications of kenaf can be extended beyond its traditional uses. Extracts from kenaf leaves, seeds, stem, and flowers were proven to have therapeutic activities such as anticancer, antimicrobial, antifungal, anti-hyperpigmentation, antioxidant, antihypertensive, and antithrombotic [Citation6–13]. The manifestation of the reported therapeutic activities of kenaf extracts is highly attributed to the abundant content of its own phytochemical constituents such as phenols, flavonoids, tocopherol, tocotrienol, citric acid, siloxane, and terpene [Citation14]. Interestingly, another compound of kenaf that is tend to be overshadowed by the other phytochemicals is its polysaccharide. Polysaccharide retrieved from kenaf leave extract was proven to possess antioxidant and antimicrobial activities [Citation8]. This indicates the kenaf polysaccharide is an area that should be explored more, so that the vast potential of this promising compound will not be undermined.
However, despite kenaf’s encouraging performance within the therapeutic field, most of the reported activities are still heavily dependent on harvesting the physical crop itself which indirectly translates into our total reliance on traditional farming to retrieve kenaf valuable extracts. In the long run, this practice is deemed to be unsustainable as the end-products quality and efficiency could vary due to inconsistent farming practices, unpredictable climatic conditions, and pest infestation [Citation15–17]. Besides, land availability will become an issue as well for the mass production of kenaf solely for its therapeutic potential as the farmers may have to compete with other crop growers, especially for food and staple crops [Citation18]. This will eventually hamper the progress and our effort in fulfilling the true potential of kenaf. Looking at the bigger picture, the continuous practice of traditional farming will lead to more deforestation activities which prevents us from achieving the targeted Sustainable Development Goals (SDG), specifically SGD 13: Climate Action, and SDG 15: Life on Land.
Thus, an alternative method for the production of kenaf’s therapeutic compound should be opted for. Cultivation of the desired plant through cell suspension cultures is one of the plant tissue culture techniques that is widely used to produce the desired plant compounds. Essentially, this technique takes advantage of the totipotency trait of the dedifferentiated plant cell (callus), which is the ability to form one whole plant that is identical to its mother plant [Citation19]. A plant cell culture is a closed and sterile system that contains an unorganized mass of dedifferentiated cells (calli) in an agitating liquid media supplemented with the necessary nutrients for growth [Citation20]. The desired compounds are either extracted from the callus or the resulting cultivated media. Some of the benefits of implying this method are that it allows a homogenous plant cell population as the cultured cells are allowed to have direct contact with the supplied media containing nutrients, vitamins, plant growth hormones, and carbon sources. The gentle shear force due to the agitation will also break the callus into smaller clumps or pieces which increases their surface area for better nutrient absorption and gas exchange activities. These will eventually allow the plant cells to grow at a faster rate [Citation19,Citation20].
Plant cell suspension culture method has been used to produce a variety of phytochemicals from different types of plants such as betalain (dragon fruit), phenolics and flavonoids (box-thorn), free amino acids (bamboo), and extracellular polysaccharides (wheat and rice) [Citation15,Citation16,Citation21–23]. Since this technique is independent of raising and maintaining crops, the production of the targeted compounds from the plant of interest can be done regardless of season, land, and plant availability [Citation16]. But even with the production of kenaf’s therapeutic compound through cell suspension culture, it is very crucial for the final yield to be high enough to cater for the need of the industries, so that its therapeutic potential can eventually reach the end consumers [Citation24]. To the best of our knowledge, no studies have ever been conducted on the optimization of the cultivating conditions of kenaf callus through cell suspension culture.
Hence, in this study, the optimization of the culturing conditions; sucrose (g/L), agitation (rpm), naphthalene acetic acid (NAA, mg/L), and 2,4-dichlorophenoxy acetic acid (2,4-D, mg/L) for the maximum production of kenaf callus biomass (KCB, g/L) and kenaf exopolysaccharide (EPS, g/L) was conducted. The factors were chosen based on their importance in influencing the liquid cultivation of plant cells whereby each factor can greatly affect the outcome of the process [Citation19,Citation20]. Besides, the carbon source (sucrose), the agitation speed which requires the usage of an orbital shaker along with the inclusion of plant growth regulators (NAA and 2,4-D) are the essential factors in the case of plant cell suspension culture. The effects of each tested variable are explained in depth in the discussion section. If the amount of any of the aforementioned factors can be minimized, this will in return save the cost of production while maximizing the output of the targeted product which are the biomass and exopolysaccharide. Thus, this justifies the reason behind the selected factors for this study. The optimization of the kenaf cell suspension culture was done using response surface methodology (RSM). This study serves as the perfect foundation for any intended upscaling processes for kenaf cell suspension culture in the future which could include the usage of bioreactors from lab to pilot scale.
2. Materials and method
2.1. Plant material and seed germination
Kenaf seeds (variety V36) were purchased from the Lembaga Kenaf dan Tembakau Negara (LKTN), Kuala Lumpur, Malaysia. The seeds retrieved have been treated with fungicide. The seeds were sterilized and germinated according to Odahara, Horii [Citation25] and Ibrahim, Kayat [Citation26] with slight alterations. The seeds were thoroughly rinsed with tap water for 2 h before being briefly washed with a commercial antibacterial disinfectant (Dettol). Then, the seeds were sterilized using 70% (v/v) ethanol for 20 s followed by 5% (v/v) sodium hypochlorite (Clorox®) for 15 to 20 min before being properly and repeatedly rinsed with 2 L of sterilized distilled water. The seeds were left to air-dry inside the laminar air flow. The seeds were placed on seed germinating medium supplemented with Murashige-Skoog (MS) medium, 3% sucrose and 0.3% gelrite, pH 5.7 – pH 5.8. The seeds were cultivated at 25°C under 16 h photoperiod in an incubation room (Agro-Biotechnology Institute, Serdang, Selangor, Malaysia).
2.2. Callus induction and proliferation
The induction of kenaf callus and its proliferation was conducted according to Odahara, Horii [Citation25] with some modifications. The upper stem of the 2-week-old kenaf seedlings () was used to initiate callus formation. The stem was aseptically cut into smaller fragments (3–5 mm) and placed onto callus inducing medium; MS media with 3% sucrose, 0.3% gelrite, 0.2 mg/L naphthalene acetic acid (NAA), and 0.2 mg/L 2,4-dichlorophenoxy acetic acid (2,4-D). The cut stem was incubated and grown under the same condition as the seed germination. Then, the visible and friable 2-week-old grown callus on the stem () was subsequently subcultured onto a new media by scrapping the callus gently to obtain an axenic kenaf callus culture. The axenic culture () was allowed to grow for another 4 weeks.
2.3. Kenaf cell suspension culture
The 6 weeks old grown axenic kenaf callus was subjected to cell suspension culture () according to Kumar, Manoj [Citation27], Mustafa, Winter [Citation20] and Klaus and Wan-Mohtar [Citation28] with slight adjustments. The grown callus (1% inoculum of the working volume) was broken up into pieces and homogenized gently before being transferred into a shake flask containing liquid medium that contains the same medium composition as the callus induction medium except for gelrite. The kenaf cell suspension culture was cultivated and agitated at 25°C under 16 h photoperiod for 22 days.
2.4. Kenaf callus biomass (KCB) separation and dry weight estimation
KCB () was separated from the liquid culture using a Buchner filter system with the help of an air pump. The filtered sample was dried at 40°C where the dry weight of the KCB was determined using a pre-dried filter paper once the weight is constant based on an improved method [Citation29].
2.5. Kenaf extracellular polysaccharide (EPS) separation, extraction, and dry weight estimation
The cultured broth (filtrate) was separated from KCB with the help of a Buchner filter system. The filtrate was added with 99% (v/v) cold ethanol at a ratio of 1:4 to precipitate EPS before being stored at 4°C for 3 days using improvised method [Citation30,Citation31]. Then, the precipitated EPS was isolated by centrifugation (10000 rpm, 10 min, 25°C), where the formed pellet () will be dried and the weight is taken once it is constant [Citation32].
2.6. Optimisation of kenaf cell suspension culture parameters using response surface methodology (RSM)
The optimization of kenaf cell suspension culture using RSM was performed accordingly. The variables that are kept constant in this study are MS medium concentration (4.41 g/L), initial pH (5.7–5.8) and culturing temperature (25°C). To maximize the production of the kenaf cell suspension outcomes; KCB and EPS (g/L), and to optimize its culturing conditions; sucrose concentration (10–30 g/L), agitation (50–150 rpm), and NAA and 2,4-D concentrations (0–2 mg/L), a complete factorial central composite design (CCD) for response surface methodology (RSM) are utilized using Design Expert 13.0 software. In , the levels and the range of the variables or factors are shown for this study.
Table 1. Experimental range and levels of independent variables.
The influence of the variables upon the responses was done through an empirical model that is based on a second quadratic-order equation as follows:
Where; Y = predicted response, = the constant coefficient,
= the linear coefficient,
= the quadratic coefficient,
= the interaction coefficient, and
= the coded values [Citation30,Citation33,Citation34].
2.7. Statistical analysis
All experiments were conducted in triplicates where the corresponding mean and standard deviation were measured using the GraphPad Prism 9 software (version 9.0.0). If the error bar is absent, it indicates that it is shorter than the size of the symbol. Meanwhile, the analysis of variance (ANOVA) for the CCD of the RSM was done using the Design Expert 13.0 software (StatEase®). The p-values (p < 0.05) were used to show the statistical significance levels for the tested coefficient models.
2.8. Residual sucrose determination
A modified 3,5-Dinitrosalicylic acid (DNS) method was used to determine residual sucrose concentration in the cultured media. 40 μL of concentrated hydrochloric acid (HCL) was added to 2 mL of the cultured media which was then allowed for hydrolysis at 90°C for 5 min. The solution was neutralized with 5 M NaOH. Then, the media was added with DNS reagent (1 mL), thoroughly mixed, and boiled for 5 min before being left to cool down to room temperature. The sample absorbance was read at 540 nm. The residual sucrose concentration was determined against a sucrose standard curve (R2 = 0.8885)
2.9. Kinetics calculation
The kinetics performance and parameters of the kenaf callus in cell suspension culture were estimated as follows [Citation33]:
Where KCBmax and EPSO are the maximum amount of KCB and EPS concentration at 22nd day of cultivation, respectively. Meanwhile, KCBO and EPSO are the initial KCB and EPS concentration at the start of the cultivation, respectively.
2.10. Fourier-transform infrared spectroscopy (FTIR) of EPS
FTIR analysis for EPS was performed according to [Citation30,Citation35] using Agilent Cary 630 that is equipped with diamond ATR (Attenuated Total Reflectance). The data generated will be collected and analyzed using MicroLab software.
3. Results and discussion
3.1. Kenaf callus suspension culture growth curve
The kenaf callus suspension culture was conducted for 30 days whereby the produced KCB and EPS were collected every 2 days in triplicates. Typically, the harvesting time of a suspension culture occurs during the end of the log phase right before the culture enters the stationary phase [Citation20]. Based on , the cultivation of kenaf callus through cell suspension culture was slow during the first 18 days which indicated the occurrence of the extended lag phase. This can be clearly seen by the low and stagnant production of the biomass and EPS. Then, the kenaf callus experienced an exponential growth from Day 18 to Day 22 whereby both of its KCB and EPS showed an increasing production trend, and this can be categorized as the log phase. However, after Day 22, the growth of kenaf callus and the production of its EPS decreased, an indication of the transition phase which is then followed by a stationary phase which can be depicted by the plateauing of the plot in the figure above. In terms of the highest amount of biomass produced, it occurred during Day 30 (9.09 g/L ±3.19) of the cultivation. Meanwhile, for EPS, the amount secreted on Day 22 of the cultivation was (1.10 g/L ±0.02). Usually, the maximum quantity of EPS can be observed happening during the earlier growth phases and during the end of log phase or initial stationary phase. This conforms with the outcome of the study as Day 22 of kenaf callus suspension culture manifested the criteria of the end of log phase, which should be taken as the harvesting time of the cultivation.
3.2. Kenaf callus biomass (KCB) production optimization
For the optimization of KCB and EPS production, 30 distinct sets of cultivating conditions were tested using RSM (). The influence of sucrose concentration, agitation speed, NAA, and 2,4-D concentrations on the production of KCB and EPS of Hibiscus cannabinus was evaluated over 22 days for each experimental design, where each pre-determined condition was conducted in triplicates.
Table 2. RSM with CCD matrix and the outcomes of the KCB and EPS production.
presents the ANOVA for the KCB production. The results showed that the model was significant as the value of ‘Prob>F’ was <0.0001 (p < 0.05). The coefficient of determination (R2 = 0.9828) indicates that the model can describe 98.28% of the response or the outcome variations. The result of the adjusted coefficient of determination (Adj. R2 = 0.9667) implies the significance of the model that is in reasonable agreement with the predicted coefficient of determination (Predict. R2 = 0.9201) with a difference of less than 0.2. The model can be expressed in terms of the actual experimental variables for KCB production using the equation below.
Table 3. The results of analysis of variance (ANOVA) generated from the CCD quadratic model in RSM for KCB.
Where; Suc = sucrose, Agi = agitation, NAA = naphthalene acetic acid, 2,4-D = 2,4-dichlorophenoxy acetic acid
Based on the results obtained (), sucrose concentration (A), agitation (B), and NAA (C) can greatly influence the outcome of KCB production (p < 0.05). Besides, the interactions between sucrose and agitation (AB), sucrose and NAA (AC), sucrose and 2,4-D (AD), agitation and NAA (BC), agitation and 2,4-D (BD), NAA and 2,4-D (CD) along with the quadratic terms of sucrose (A2), agitation (B2) and 2,4-D (D2) also do have a significant (p < 0.05) impact on the production of KCB through this model. However, 2,4-D concentration (D) and the quadratic term of NAA (C2) have negative effects on the model. Based on the model, maximizing the KCB production will require the usage of 40.03 g/L of sucrose, 1.87 mg/L of NAA, and 0.04 mg/L of 2,4-D with an agitation speed of 129.59 rpm.
shows the combined effects and interactions between all the experimental variables on the production of KCB. Based on , it is safe to say that all variables are dependent upon each other when it comes to the production of KCB. For instance, the influence of 2,4-D on KCB production with sucrose (), and with agitation () is minimal. But the contribution of 2,4-D becomes higher upon its interaction with NAA as the increase of both hormones will lead to an increase in the KCB production (). The same phenomena can be observed for the KCB outcome in the interaction between NAA with sucrose, agitation, and 2,4-D (). This indicates that the variables’ interdependency among each other is important in maximizing the production of KCB of Hibiscus cannabinus through cell suspension culture.
Figure 3. Three-dimensional (3D) response surface plot of KCB production of Hibiscus cannabinus showing the interaction between; (a) sucrose and agitation, (b) sucrose and NAA, (c) sucrose and 2,4-D, (d) agitation and NAA, (e) agitation and 2,4-D and (f) NAA and 2,4-D.

Carbon sources for cell suspension culture come in various forms such as glucose, sucrose, and fructose. These sugars act as the energy source for cultured plants to grow and multiply through cell division and are also used for other metabolic pathways such as cell enlargement, elongation, and even in the production of secondary metabolites [Citation20]. Since the culture involves the usage of undifferentiated cells (calli) in liquid culture, the presence of these carbon sources is crucial in ensuring its growth. When sucrose is used, it will be converted into glucose and fructose where the former will be utilized first for metabolic processes followed by the latter [Citation19].
The plant growth regulators (PGR) chosen for this study were both in the form of auxin; NAA and 2,4-D. PGR are synthetic compounds that can imitate the effects of naturally produced plant hormones, and they are typically required in a very minute quantity [Citation20]. Auxins are crucial for the initiation of cell division, cell elongation, and the formation of callus. This class of PGR also tend to inhibit the production of shoots [Citation19]. The usage of NAA and 2,4-D was found to be effective in the callus formation of a Malvaceae-family plant, Helicteres isora L. [Citation36] which is in accordance with this study.
Besides, agitation is another crucial factor in plant cell suspension culture which is primarily for generating a gentle pressure to break the cultured cell aggregates into smaller pieces. This gives a larger total surface area of the cells for better nutrient exchange with the supplied medium. The agitation also helps the culture in achieving a homogenous mixture by maintaining a uniform distribution of the cells and nutrients, and provides good gas exchange activity in the cultivation system [Citation19,Citation20]. Taken together, a good agitation rate that will not cause negative effects on the cultured plant cells will promote the growth of the callus in liquid.
3.3. Kenaf extracellular polysaccharide production optimization
Meanwhile, shows the ANOVA for the EPS production. The results stated that the model was significant because the value of ‘Prob>F’ was <0.0001 (p < 0.05). The R2 of 0.9176 shows that the model can explain 91.76% of the response or the outcome variations for EPS. The result of the Adj. R2 (0.8406) implies the significance of the model that is in reasonable agreement with the Predict. R2 (0.6833) with a difference of less than 0.2. The model can be expressed in terms of the actual experimental variables for EPS production using the equation below.
Table 4. The results of analysis of variance (ANOVA) generated from the CCD quadratic model in RSM for EPS.
Where; Suc = sucrose, Agi = agitation, NAA = naphthalene acetic acid, 2,4-D = 2,4-dichlorophenoxy acetic acid
Based on the results in , sucrose concentration (A), agitation (B), and NAA concentration (C) can significantly affect the outcome of EPS production with p values of less than 0.05. Furthermore, the interaction between the variables especially between sucrose and agitation (AB), and agitation and NAA (BC) along with the quadratic terms of sucrose (A2) and NAA (C2) also turned out to be significant in producing EPS through this model (p < 0.05). But, other interactions and quadratic terms; AC, AD, BD, CD, B2, and D2 with p values of above 0.05 will negatively affect the EPS production of kenaf cell suspension culture using this model. According to the model, the maximum amount of EPS can be generated by agitating the culture at 134.25 rpm with media supplemented with 49.99 g/L of sucrose, 1.34 mg/L of NAA, and 1.983 mg/L of 2,4-D.
shows the significant interactions (p < 0.05) between experimental variables (sucrose, agitation, and NAA) on influencing the amount of EPS produced. Based on , the EPS produced will increase with the increase of both sucrose concentration and agitation. Meanwhile, the interaction between agitation rate and NAA concentration will also have a huge effect on the final EPS generated.
Figure 4. Three-dimensional (3D) response surface plot of EPS production of Hibiscus cannabinus showing the interaction between; (a) sucrose and agitation, and (b) agitation and NAA.

Based on the optimized variables for the production of KCB and EPS using kenaf callus in cell suspension cultures (), the experimental factors can be manipulated for a variety of purposes. Maximizing KCB output would require the usage of 30 g/L sucrose, 1.87 mg/L NAA, 0.04 mg/L 2,4-D and agitated at 129.59 rpm. To increase the EPS production, an agitation rate at 134.25 rpm and medium composition supplemented with 49.99 g/L sucrose, 1.34 mg/L NAA, and 1.983 mg/L 2,4-D will be favored. But if the cultivation requires the maximum outputs of both KCB and EPS, the usage of media with 50 g/L, 2 mg/L NAA and an agitation speed at 147.02 would be employed.
Table 5. Optimized conditions for kenaf callus cell suspension culture.
The production of EPS through plant cell suspension culture that can be retrieved from the growth medium is common [Citation15,Citation21,Citation37,Citation38]. The EPS produced can be categorized based on their major molecular structures such as glucans, galacturonan, and xyloglucan. The EPS secreted into the liquid medium is of the same nature as the type of polysaccharides that made up the cell wall of the cultured plant cells [Citation37,Citation39]. The production of EPS occurs during cell wall expansion whereby the in situ enzymes of the cells will initiate the process of hydrolysis that breaks down the glycosidic linkages of the polysaccharide matrix. This loosens the cell wall and causes it to expand where the product of the process which is the EPS is secreted into the cultivating medium concurrently [Citation37].
3.4. Verification of the optimized conditions
also shows the cultivating conditions to optimize the production of KCB and EPS which were executed to verify the statistical model. 250 mL shake flasks were used to validate the model. Conditions for maximizing KCB and EPS production produced 11.97 g/L of KCB and 0.73 g/L of EPS which are 87% and 40% of the predicted values, respectively. Meanwhile, 89% and 56% of the predicted values were achieved with 11.87 g/L of KCB and 0.91 g/L of EPS produced using 50 g/L of sucrose and 2 mg/L of NAA at 147.02 rpm. The EPS produced deviates from the predicted responses. This could be due to the age and condition of the callus. Callus that is old (more than 4 weeks old) and has shown the signs browning was found to have a poor performance in the production of EPS into the liquid medium.
3.5. Kinetics parameters of kenaf callus in cell suspension culture
At the end of the growth profile of kenaf callus in cell suspension culture (), the productivity of KCB and EPS are 0.37 ± 1.2 and 0.05 ± 0.02 g L−1 day−1. Meanwhile, the specific production rate of EPS was 0.01 ± 0.02 g g−1 day−1. For KCB optimization (), the KCBmax and KCBProductivity increased by 1.3 fold compared to kenaf callus grown in condition A (). However, for EPS optimization (), both EPSmax and EPSProductivity are lower than the EPS produced in condition A which is due to the age and condition of the callus. For condition D (), which is to maximize the production of KCB and EPS, the KCBmax and KCBProductivity recorded a 1.3 fold of increase as well compared to condition A.
Table 6. Kinetic parameters of kenaf callus in cell suspension culture.
A: 30 g/L sucrose, 0.2 mg/L NAA, and 0.2 mg/L 2,4-D at 100 rpm, 25°C, and 16 h photoperiod. B: 40.03 g/L sucrose, 1.87 mg/L NAA, and 0.04 mg/L 2,4-D at 129.59 rpm, 25°C, and 16 h photoperiod. C: 49.99 g/L sucrose, 1.34 mg/L NAA, and 1.98 mg/L 2,4-D at 134.25 rpm, 25°C, and 16 h photoperiod. D: 50 g/L sucrose and 2.00 mg/L NAA at 100 rpm, 25°C, and 16 h photoperiod.
3.6. Characterisation of kenaf EPS using Fourier-transform infrared (FTIR)
The extracted kenaf callus exopolysaccharide was subjected to FTIR () spectroscopy to study the interaction between its chemical bonds and infrared radiation which helps in characterizing its functional groups within its carbon ring in comparison to the standard glucan laminarin. The FTIR analysis was performed according to Pavia, Lampman [Citation40] and Hong, Yin [Citation41]. The single-broad absorption peak in the region between 3000 cm−1 and 3500 cm−1 suggested the presence of hydroxyl group (−OH) in the exopolysaccharide structure. A low absorption peak is observed at 2933 cm−1 which indicated the stretching vibration of C-H in the sugar ring. Besides, the peak manifested (1628 cm−1) in between 1600 cm−1 and 1650 cm−1 confirmed the stretching vibration of carbonyl group (C=O). The strong absorption peak at 1000 cm−1 − 1300 cm−1 designated the C-O-C vibration in the pyran structure of kenaf callus exopolysaccharide. The resulted peak at 865 cm−1 showed the presence of the α-linked glycosidic linkages in the sugar ring [Citation42]. These structural confirmations suggested that the extracted kenaf callus exopolysaccharide are glucans in the form of α-configuration (α-glucan). Interestingly, several studies have reported the bioactivities of α-glucan such as anti-inflammatory, antibacterial, and antioxidant [Citation43,Citation44]. This shows that the application of exopolysaccharide extracted from the callus of kenaf through cell suspension culture can be further explored in various industries such as pharmaceutical, cosmeceutical, and even food [Citation3].
4. Comparison with other literature
A comparison of the total callus biomass and extracellular polysaccharide produced through cell suspension culture between different species of plants is shown . To the best of our knowledge, this study is the first to ever report on the production of KCB and EPS of Hibiscus cannabinus. Other literature in Table 4.1 focused on the production of the callus biomass and other metabolites of interest, where none reported the production of its extracellular polysaccharide. The inconsistency in terms of the experimental parameters proved the need for the optimization study to produce KCB and EPS of Hibiscus cannabinus efficiently.
Table 7. Comparison of the total biomass and extracellular polysaccharide produced with other literature.
5. Challenges, limitations, and future improvement of kenaf callus in cell suspension culture
5.1. Cultivation technique
Cultivation of kenaf callus in cell suspension culture requires massive attention and must be done aseptically. Concerns about the homogeneity of the culture would arise if the callus used as the inoculum is not aseptically homogenized. Clumps of callus in the agitating liquid are not favorable as the distribution and the nutrient exchange rate between the growing callus and the media are not uniform. Even the total surface area of the clumped callus is lower compared to the dispersed callus. This will lead to a non-uniform growth of callus which would result in irreproducible outcomes [Citation20]. This condition is not acceptable if kenaf callus were to be brought into the industry as the production rate must be constant and equally high to meet the demands. Thus, it would be best to homogenize the callus gently before transferring it into the liquid media for better growth activity.
5.2. Solubility of the extracted EPS
The precipitated EPS after the cultivation is insoluble in water. This situation makes it difficult for it to go for any bioassay activities, which could reveal its hidden pharmacological properties. Certain polysaccharides such as pullulan and amylopectin are naturally soluble in water. But there are polysaccharides with poor water solubility such as xylan and mannan [Citation47], and the newly produced EPS belongs in this category as well. Thus, additional steps must be taken to dissolve the EPS which can be done through either biological modifications (enzymatic degradation), physical modifications (sonication, irradiation, autoclaving, heating, etc.) or chemical modifications (sulfation, carboxymethylation, phosphorylation, methylation, etc.) [Citation48].
5.3. Mass-scale kenaf callus liquid cultivation
This study serves as the perfect foundation for any upscaling activities in the future. Industrial-scale production of KCB and EPS would eventually require the usage of bioreactors [Citation49]. The experimental parameters or variables determined in this study to maximize the output of KCB and EPS are applicable to bioreactors’ applications. The production using bioreactors is expected to outperform the cultivation in shake flasks as more parameters are controllable such as aeration rate (L/min), the concentration of dissolved oxygen and the pH of the media. But, the impellers used in the bioreactor system must be gentle enough to not break and cause any damage to the cultivated callus and the marine-type impeller () is known for its reduced shear force on the cultures [Citation50]. Thus, it is highly suggested to use bioreactors for the mass production of KCB and EPS, with considerations of the optimized parameters and growth conditions.
5.4. The future of KCB
The produced KCB should be further screened for its useful extracts such as phenolic, flavonoid, polysaccharides (intracellular polysaccharides) and other secondary metabolites that are useful not only in the pharmaceutical industry but in food and cosmeceutical industries as well. It is suggested that the callus of plant can be the source for recovery of higher quantities and qualities of phytochemicals [Citation36]. With this, the burden or our dependency on the physical crop can be reduced through a greener approach. But, the yield of the extracted secondary metabolites from the produced callus should be enough to cater for the needs of the industries [Citation24]. So, other optimization studies that specifically target to maximize the content of the metabolites of interest can be explored as well with the intentions to apply and realize the idea of landless farming, and sustainable and circular agricultural systems [Citation17,Citation51,Citation52]. If the metabolites content in the callus can be increased more than the extracts from the in-tact plant, the need for land for plant cultivation can be alleviated greatly which in return leads to more preservation of the under threat-forests.
6. Conclusion
The true potential of kenaf is yet to be fully uncovered. Many are still focusing on the traditional method of cultivating and harvesting kenaf for different purposes. Looking into the Sustainable Development Goals, limiting the usage of physical land for farming practices would eventually turn the dreams of Goal No. 13 (Climate Action) and Goal No. 15 (Life on Land) into a reality. The first step could be achieved by looking into biotechnological approaches such as plant cell suspension culture in addressing the demand of the industries. To make this sustainable practice more industrial-friendly, the intended product’s final yield must be maximized. For the kenaf callus suspension culture, the manipulation of experimental variables through RSM; sucrose concentration (50 g/L), agitation (147.02 rpm), and NAA concentration (2 mg/L) will maximize the production of KCB and EPS on the 22nd day of the culture. Sucrose and NAA concentrations along with the agitation speed of the culture will significantly (p < 0.05) affect the outcome of KCB and EPS. Looking forward, this study provides insight into the possible upscaling production of KCB and EPS using bioreactors along with its potential applications in various industries. Further studies are suggested to evaluate the pharmaceutical activities from both EPS and the extracts of KCB which will vindicate this biotechnological approach in developing a sustainable production line.
Authors’ contributions
Conceptualization, D.‘A.N., P.L-S, N.M.S, Z.I and W.A.A.Q.I.W.-M.; methodology, S.R.A.U. and D.A.G.V.; software, N.M.S., D.A.G.V and M.F.I.; validation, N.M.S., M.F.I, Z.I., P.L-S and W.A.A.Q.I.W.-M.; formal analysis, D.‘A.N. and D.A.G.V.; investigation, D.‘A.N. and N.M.S.; resources, Z.I. and W.A.A.Q.I.W.-M.; data curation, S.R.A.U., M.F.I. and N.M.S.; writing – original draft preparation, D.‘A.N, S.R.A.U, D.A.G.V. and W.A.A.Q.I.W.-M.; writing – review and editing, D.‘A.N. and M.F.I.; visualization, D.‘A.N.; supervision, P.L-S. Z.I. and W.A.A.Q.I.W.-M.; project administration, N.M.S., Z.I. and W.A.A.Q.I.W.-M.; funding acquisition, N.M.S., S.R.A.U, P.L-S. and W.A.A.Q.I.W.-M. All authors have read and agreed to the published version of the manuscript.
Ethical approval
No ethical approval is required as no animals and related ethical materials were used in this study
Disclosure statement
No potential conflict of interest was reported by the author(s).
Data availability statement
The authors confirm that the data supporting the findings of this study are available within the article.
Additional information
Funding
References
- Mohd H, Arifin A, Nasima J, et al. Journey of kenaf in Malaysia: a review. Sci Res Essays. 2014;9(11):458–17. doi: 10.5897/SRE12.471
- Kamal IB. Kenaf for biocomposite: an overview. J Sci Technol. 2014;6(2):41–66.
- Norhisham DA, Saad NM, Ahmad Usuldin SR, et al. Bioactivities of kenaf biomass extracts: a review. Processes. 2023;11(4):1178. doi: 10.3390/pr11041178
- Ab Razak AF, Abidin MZ, OsmanMS, et al. The Effect of Storage Conditions on Pasteurised Kenaf Seeds Milk Mh8234 Physiochemical Properties. Math. Stat. Eng. Appl. 2022;71(3):1685–1698.
- Ayadi R, Hanana M, Mzid R, et al. Hibiscus cannabinus L. – « kenaf »: a review paper. J Nat Fibers. 2017;14(4):1–19. doi: 10.1080/15440478.2016.1240639
- Adnan M, Oh KK, Azad MOK, et al. Kenaf (Hibiscus cannabinus L.) leaves and seed as a potential source of the bioactive compounds: effects of various extraction solvents on biological properties. Life. 2020;10(10):1–16. doi: 10.3390/life10100223
- Arulrajah B, Qoms MS, Muhialdin BJ, et al. Antibacterial and antifungal activity of kenaf seed peptides and their effect on microbiological safety and physicochemical properties of some food models. Food Control. 2022;140(December 2021):109119. doi: 10.1016/j.foodcont.2022.109119
- Birhanie ZM, Xiao A, Yang D, et al. Polysaccharides, total phenolic, and flavonoid content from different kenaf (Hibiscus cannabinus l.) genotypes and their antioxidants and antibacterial properties. Plants. 2021;10(9):1900. doi: 10.3390/plants10091900
- Kobaisy M, Tellez MR, Webber CL, et al. Phytotoxic and fungitoxic activities of the essential oil of kenaf (Hibiscus cannabinus L.) leaves and its composition. J Agric Food Chem. 2001;49(8):3768–3771. doi: 10.1021/jf0101455
- Sim YY, Tan CP, Cheong LZ, et al. Hibiscus cannabinus L. leaf and seed in cosmetic formulation: an integrated approach as antioxidant and melanogenesis inhibitor. Sustainable Mater Technol. 2022;33(1):e00457. doi: 10.1016/j.susmat.2022.e00457
- Hanumegowda S, Srinivasa C, Shivaiah A, et al. Protein extract of kenaf seed exhibits anticoagulant, antiplatelet and antioxidant activities. Asian Pac J Tropical Biomedi. 2022;12(2):47–58. doi: 10.4103/2221-1691.335693
- Ryu J, Kwon S-J, Ahn J-W, et al. Phytochemicals and antioxidant activity in the kenaf plant (Hibiscus cannabinus L.). J Plant Biotechnol. 2017;44(2):191–202. doi: 10.5010/JPB.2017.44.2.191
- Zaharuddin ND, Hanafi MA, Chay SY, et al. Multifunctional hydrolysates from kenaf (Hibiscus cannabinus L.) seed protein with high antihypertensive activity in vitro and in vivo. J Food Meas Charact. 2020;15(1):652–663. doi: 10.1007/s11694-020-00663-2
- Sim YY, Nyam KL. Hibiscus cannabinus L. (kenaf) studies: nutritional composition, phytochemistry, pharmacology, and potential applications. Food Chem. 2021;344(1):128582. doi: 10.1016/j.foodchem.2020.128582
- Murtazina A, Ruiz Alcala G, Jimenez-Martinez Y, et al. Anti-cancerous potential of polysaccharides derived from wheat cell culture. Pharmaceutics. 2022;14(5. doi: 10.3390/pharmaceutics14051100
- Winson KWS, Chew BL, Sathasivam K, et al. The establishment of callus and cell suspension cultures of hylocereus costaricensis for the production of betalain pigments with antioxidant potential. Ind Crops Prod. 2020;155(4):112750. doi: 10.1016/j.indcrop.2020.112750
- Rahmann G, Azim K, Brányiková I, et al. Innovative, sustainable, and circular agricultural systems for the future. Org Agr. 2021;11(2):179–185. doi: 10.1007/s13165-021-00356-0
- Wong YH, Tan WY, Tan CP, et al. Cytotoxic activity of kenaf (Hibiscus cannabinus L.) seed extract and oil against human cancer cell lines. Asian Pac. J. Trop. Biomed. 2014;4:S510–S515.
- Chawla HS. Introduction to plant Biotechnology. Enfield, NH, USA: Science Publisher, Inc; 2002.
- Mustafa NR, de Winter W, van Iren F, et al. Initiation, growth and cryopreservation of plant cell suspension cultures. Nat Protoc. 2011;6(6):715–742. doi: 10.1038/nprot.2010.144
- Sims IM, Middleton K, Lane AG, et al. Characterisation of extracellular polysaccharides from suspension cultures of members of the poaceae. Planta. 2000;210(2):261–268. doi: 10.1007/PL00008133
- Ogita S. Callus and cell suspension culture of bamboo plant, phyllostachys nigra. Plant Biotechnol. 2005;22(2):119–125. doi: 10.5511/plantbiotechnology.22.119
- Mamdouh D, Smetanska I. Optimization of callus and cell suspension cultures of lycium schweinfurthii for improved production of phenolics, flavonoids, and antioxidant activity. Horticulturae. 2022;8(5). doi: 10.3390/horticulturae8050394
- Ghafar SAA, Ismail M, Saiful Yazan L, et al. Cytotoxic activity of kenaf seed oils from supercritical carbon dioxide fluid extraction towards human colorectal cancer (HT29) cell lines. evidence-based complementary and alternative medicine, 2013. Evid Based Complement Alternat Med. 2013;2013:1–8. doi: 10.1155/2013/549705
- Odahara M, Horii Y, Kimura M, et al. Efficient callus induction and a temperature condition for flowering and seed setting in kenaf Hibiscus cannabinus. Plant Biotechnol. 2020;37(1):9–14. doi: 10.5511/plantbiotechnology.19.1120a
- Ibrahim AM, Kayat FB, Susanto D, et al. Callus induction from ovules of kenaf (Hibiscus cannabinus L.). Biotechnology. 2015;14(2):72–78. doi: 10.3923/biotech.2015.72.78
- Kumar SS, Manoj P, Giridhar P. Optimization of an in vitro protocol for the production of ascorbic acid in Hibiscus cannabinus leaf-derived normal root cultures. EurAsian J BioSci. 2015;9(5):38–45.
- Klaus A, Wan-Mohtar WAAQI. Cultivation strategies of edible and medicinal mushrooms. In: Dhull SB, et al., editors. Wild mushrooms. Boca Raton: CRC Press; 2022. pp. 23–65. doi: 10.1201/9781003152583-3.
- Wan-Mohtar WAAQI, Taufek NM, Yerima G, et al. Effect of bioreactor-grown biomass from Ganoderma lucidum mycelium on growth performance and physiological response of red hybrid tilapia (Oreochromis sp.) for sustainable aquaculture. Org Agr. 2021;11(2):327–335. doi: 10.1007/s13165-020-00303-5
- Balamurugan JP, Supramani S, Ahmad Usuldin SR, et al. Efficient biomass-endopolysaccharide production from an identified wild-Serbian Ganoderma applanatum strain BGS6Ap mycelium in a controlled submerged fermentation. Biocatal Agric Biotechnol. 2021;37(2020):102166. doi: 10.1016/j.bcab.2021.102166
- Usuldin SRA, Wan-Mohtar WAAQI, Ilham Z, et al. In vivo toxicity of bioreactor-grown biomass and exopolysaccharides from Malaysian tiger milk mushroom mycelium for potential future health applications. Sci Rep. 2021;11(1):1–13. doi: 10.1038/s41598-021-02486-7
- Günter EA, Popeiko OV, Ovodov YS. Isolation of polysaccharides from the callus culture of Lemna minor L. Appl Biochem Microbiol. 2004;40(1):80–83. doi: 10.1023/B:ABIM.0000010359.68528.fe
- Usuldin SRA, Ilham Z, Jamaludin AA, et al. Lignosus rhinocerus in a high-scale stirred-tank bioreactor and its potential lipid as bioenergy. Energies. 2023;16(5):16. doi: 10.3390/en16052330
- Abdullah NR, Sharif F, Hafizah Azizan N, et al. Pellet diameter of Ganoderma lucidum in a repeated-batch fermentation for the trio total production of biomass-exopolysaccharide-endopolysaccharide and its anti-oral cancer beta-glucan response. Microbiology. 2020;6(4):379–400. doi: 10.3934/microbiol.2020023
- Usuldin SRA, Mahmud N, Ilham Z, et al. In - depth spectral characterization of antioxidative (1, 3) - β - D - glucan from the mycelium of an identified tiger milk mushroom lignosus rhinocerus strain ABI in a stirred - tank bioreactor. Biocatal Agric Biotechnol. 2020;23:23. doi: 10.1016/j.bcab.2019.101455
- Shaikh S, Shriram V, Vinay Kumar TKA, et al. Establishment of callus and cell suspension cultures of Helicteres isora L. Res Plant Biol. 2018;8:01–07. doi: 10.25081/ripb.2018.v8.3366
- Honda Y, Itano M, Sugimura Y. Biosynthesis of extracellular polysaccharides by tuberose callus. J Plant Physiol. 1997;150(1–2):46–52. doi: 10.1016/S0176-1617(97)80179-4
- Kim M-U, Cho Y-J, Lee S-Y. Production and optimization of extracellular polysaccharide by suspension cultivation of Aloe vera L. callus. 2012;16(3):233–241.
- Webster JM, Oxley D, Pettolino FA, et al. Characterisation of secreted polysaccharides and (glyco)proteins from suspension cultures of pyrus communis. Phytochemistry. 2008;69(4):873–881. doi: 10.1016/j.phytochem.2007.10.009
- Pavia DL, Lampman GM, Kriz GS, Introduction to spectroscopy. 2001, United States of America: Thomson Brooks/Cole
- Hong T, Yin, JY, Nie, SP, Xie, MY. Applications of infrared spectroscopy in polysaccharide structural analysis: progress, challenge and perspective. Food Chemistry: X. 2021;12(November):100168. doi: 10.1016/j.fochx.2021.100168
- Wang YX, Xin Y, Yin J-Y, et al. Revealing the architecture and solution properties of polysaccharide fractions from macrolepiota albuminosa (Berk.) Pegler. Food Chem. 2022;368(August 2021):130772. doi: 10.1016/j.foodchem.2021.130772
- Buddana SK, Venkata Naga Varanasi Y, Reddy Shetty P. Fibrinolytic, anti-inflammatory and anti-microbial properties of α-(1-3)-glucans produced from streptococcus mutans (MTCC 497). Carbohydr Polym. 2015;115:152–159. doi: 10.1016/j.carbpol.2014.08.083
- Huang X, Ma J, Wei L, et al. An antioxidant α-glucan from Cladina rangiferina (L.) Nyl. And its protective effect on alveolar epithelial cells from Pb2+-induced oxidative damage. Int j biol macromol. 2018;112:101–109. doi: 10.1016/j.ijbiomac.2018.01.154
- Jafari Hajati R, Payamnoor V, Ghasemi Bezdi K, et al. Optimization of callus induction and cell suspension culture of Betula pendula Roth for improved production of betulin, betulinic acid, and antioxidant activity. Vitro Cell Deve Biol - Plant. 2016;52(4):400–407. doi: 10.1007/s11627-016-9773-6
- Meftahizade H, Lotfi M, Moradkhani H. Optimization of micropropagation and establishment of cell suspension culture in Melissa officinalis L. Afr J Biotechnol. 2010;9(28):4314–4321.
- Guo MQ, Hu X, Wang C, et al. Polysaccharides: structure and solubility. In: Solubility of polysaccharides. Xu Z editor. IntechOpen; 2017. 10.5772/intechopen.71570
- Jayawardena B, Pandithavidana DR, Sameera W. Polysaccharides in solution: experimental and computational studies. In: Solubility of Polysaccharides. Xu Z, editor, 2017.
- Vayabari DAG, Ilham Z, Md Saad N, et al. Cultivation strategies of kenaf (Hibiscus cannabinus L.) as a future approach in Malaysian agriculture industry. Horticulturae. 2023;9(8. doi: 10.3390/horticulturae9080925
- Eibl R, Eibl D. Design of bioreactors suitable for plant cell and tissue cultures. Phytochem Rev. 2008;7(3):593–598. doi: 10.1007/s11101-007-9083-z
- Wan-Mohtar WAAQI, Ilham Z, Rowan NJ. Editorial: “the value of microbial bioreactors to meet challenges in the circular bioeconomy”. Front Bioeng Biotechnol. 2023;11:1181822. doi: 10.3389/fbioe.2023.1181822
- Chan HY, Abdul Halim-Lim S, Tan TB, et al. Exploring the drivers and the interventions towards sustainable food security in the food supply chain. Sustainability. 2020;12(19. doi: 10.3390/su12197890