ABSTRACT
Transplantation of tissue-engineered trachea is an effective treatment for long-segment tracheal injury. This technology avoids problems associated with a lack of donor resources and immune rejection, generating an artificial trachea with good biocompatibility. To our knowledge, a systematic summary of basic and clinical research on tissue-engineered trachea in the last 20 years has not been conducted. Here, we analyzed the development trends of tissue-engineered trachea research by bibliometric means and outlined the future perspectives in this field. The Web of Science portal was selected as the data source. CiteSpace, VOSviewer, and the Bibliometric Online Analysis Platform were used to analyze the number of publications, journals, countries, institutions, authors, and keywords from 475 screened studies. Between 2000 and 2023, the number of published studies on tissue-engineered trachea has been increasing. Biomaterials published the largest number of papers. The United States and China have made the largest contributions to this field. University College London published the highest number of studies, and the most productive researcher was an Italian scholar, Paolo Macchiarini. However, close collaborations between various researchers and institutions from different countries were generally lacking. Despite this, keyword analysis showed that manufacturing methods for tracheal stents, hydrogel materials, and 3D bioprinting technology are current popular research topics. Our bibliometric study will help scientists in this field gain an in-depth understanding of the current research progress and development trends to guide their future work, and researchers in related fields will benefit from the introduction to transplantation methods of tissue-engineered trachea.
HIGHLIGHTS
We conducted a comprehensive bibliometric analysis of tissue-engineered trachea.
We systematically outlined the preparation methods and current development forms of tissue-engineered trachea.
We predicted future tissue-engineered trachea research trends from the perspectives of countries, institutions, researchers, and popular research topics.
1. Introduction
The trachea is an important component of the human respiratory system. It connects the larynx with the bronchi, defends against harmful substances, removes foreign bodies, and regulates the temperature and humidity of inhaled air [Citation1]. Clinically, the trachea can be damaged by tumors, trauma, or infection. When the extent of the tracheal defect is less than 1/2 or 1/3 of the total length of the adult trachea, the end-to-end anastomosis of the trachea is considered the most effective treatment program for tracheal repair [Citation2,Citation3]. However, when the tracheal defect exceeds the abovementioned limits, continued use of the end-to-end tracheal anastomosis may lead to severe postoperative complications, such as tracheal fracture and anastomotic fistula, owing to excessive anastomotic tension. In these situations, tracheal replacement is thus required for reconstruction [Citation4].
Tissue engineering refers to techniques that apply the principles of engineering and life sciences to the repair and reconstruction of functional tissues and organs using artificial biological substitutes [Citation5]. Continuous technological progress has facilitated the development of tissue engineering for tracheal repair, which has gradually become a method of choice for tracheal replacement treatments for patients with long-segment tracheal injuries. Common methods for tissue-engineered trachea preparation include perfusion [Citation6], decellularization [Citation7], electrospinning [Citation8], and 3D bioprinting [Citation9] (). The traditional tracheal repair strategy based on tissue engineering usually involves the inoculation of high-density seed cells and growth factors on preformed scaffolds, whose function is to maintain mechanical stability, whereas seed cells and growth factors ensure the biological activity of the grafts for further epithelization and vascularization in vivo [Citation7,Citation10,Citation11]. With the advancement of technology, researchers have made significant breakthroughs regarding the sources of scaffold materials under the same scaffold preparation process. The macroscopic single natural material scaffolds gradually transition to microscopic nanocomposite scaffolds [Citation12–14]. Ni et al. recently prepared a chitosan/lithium algal soil nanocomposite hydrogel, which could be effectively used for tracheoesophageal injury treatment [Citation15]. This qualitative leap has brought new opportunities for the treatment of patients. However, owing to the unsatisfactory results of clinical trials, the clinical application of the tissue-engineered trachea preparations requires time.
Figure 1. Common methods of tissue-engineered trachea preparation. (a) casting, (b) decellularization, (c) electrospinning, and (d) 3D bioprinting. Images were generated using the Medpeer software.
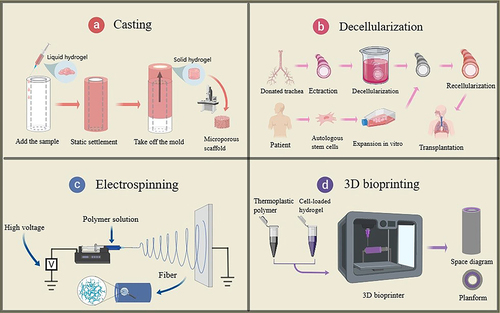
Bibliometric analysis quantitatively analyzes published literature and visualizes analytical data using professional software to help determine development trends and hot topics in specific research fields [Citation16,Citation17]. With a gradual increase in the number of studies on tissue-engineered tracheas, the number of researchers involved has also increased. However, comprehensive and detailed quantitative analyses of the current status and future trends related to tracheal tissue engineering are lacking. The purpose of this study was to analyze current research trends in tracheal tissue engineering using previously published experimental data, estimate relative impacts of experiments performed by various authors in different institutions and countries, and examine the use of keywords. The results of our study will help explore research frontiers, identify hot topics, and thus guide future research in this field.
2. Materials and methods
2.1. Data sources and search strategies
The Web of Science (WoS) database contains a large volume of high-value and high-impact research information [Citation18]. All data in this study were obtained from the core WoS database via the Yangzhou University Library, using the following search strategy: TS=(trachea*) AND (TS=(replacement) OR TS=(reconstruction) OR TS=(rebuilding) OR TS=(transplantation)) AND TS=(tissue engineering). In total, 505 studies were identified in the initial search.
2.2. Screening criteria
Literature sources related to tissue engineering and tracheal repair were then selected. The document types included ‘Article’ and ‘Review,’ whereas ‘Proceedings Papers,’ ‘Editorial Material,’ ‘Early Access,’ ‘Letter,’ ‘Retracted Publications,’ and ‘Corrections’ were removed. There were no date restrictions, and only papers in English were considered.” In total, 475 articles were identified after the screening, including 396 original articles and 79 reviews. To prevent changes in data quantity caused by database updates, literature retrieval, and downloads were completed on the same day (2023.01.05), and two researchers (XXY and SYB) conducted independent retrievals to ensure the accuracy of the final data ().
2.3. Software usage specification
In this study, we systematically analyzed the numbers of publications, journals, authors, countries, institutions, and keywords. We used CiteSpace V6.2. R2 (Drexel University, Philadelphia, PA, USA) [Citation19] and VOSviewer 1.6.18 (Leiden University, Leiden, the Netherlands) [Citation20] for the primary visualization, whereas the literature metrology analysis was conducted using the online platform https://bibliometric.com/app.
2.3.1. CiteSpace
The 475 identified manuscripts were exported from the core WoS database as ‘Plain text files.’ After the data were imported into CiteSpace, the references were checked for duplicates, but none were found. CiteSpace was used for time slicing from 1 January 2000, to 1 January 2023, with each slice representing a year. The selection criterion for the data analysis was the g-index (k = 25), and different node types were selected according to the purpose. The node association intensity was set to ‘Cosine,’ ‘Pathfinder,’ and ‘Pruning the merged network’ for the pruning area to simplify the network of node partnerships and highlight the connections between critical nodes. For the emergent word analysis in the keywords, we set the parameter r [0,1] = 0.7 through the console, with a minimum duration of 2 years, and the top 25 emergent words were selected for further analysis.
2.3.2. VOSviewer
The 475 identified studies were exported from the WoS core database as ‘Tab delimited files.’ The VOSviewer was used to generate a ‘Create-Create a map based on bibliographic date-Read data from bibliographic database files,’ and the data were imported. Different analysis categories were selected according to the purpose, the minimum occurrence times and number of nodes were set, and visual analysis was conducted.
2.3.3. Bibliometric online analysis platform
The literature metrology online analysis platform was opened, all 475 studies in the UTF-8 format were imported, and different analysis categories were selected for visualization.
3. Results
3.1. Quantitative analysis
In total, 475 articles related to tissue engineering and tracheal repair were retrieved from the WoS core collection database. The number of published articles showed an increasing trend, with a peak of 172 in the past 5 years (2018–2022), accounting for 36.2% of the total number of published articles on this topic since 2000. In 2021, the number of publications reached a record high of 42, and the number of published papers in 2022 (33) was marginally lower (). Thus, it was concluded that tissue engineering technology for tracheal repair and reconstruction has encountered some difficulties in implementation and that constraints are limiting its development. The top 10 countries/regions that published studies in this field in 2000–2023 are shown in . Among them, the United States, Netherlands, Germany, and Japan invested the earliest and have thus played pioneering roles in the development of tissue-engineered tracheas. Moreover, the United States has continued its research with outstanding results. Since 2007, researchers from China have started to study this topic. To date, China ranks second in terms of the number of research papers in tracheal tissue engineering. Moreover, since 2015, the total number of annually published papers in China and the United States has been close to or more than half of the annual number of published papers overall, which further highlights the leading role of these countries in the development of this field.
Figure 3. Annual publication volume analysis. (a, b) number of research publications per annum and growth trends for tissue-engineered trachea in general (a) and in the top 10 countries (b). The results were exported from the online analysis platform of literature metrology (http://bibliometric.com/). Blocks of different colors represent different countries.
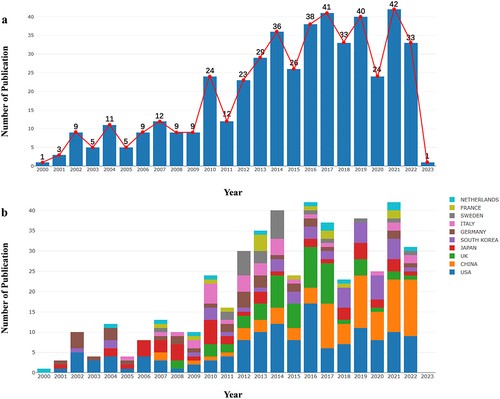
3.2. Subject and journal analysis
The 475 articles identified in this study were published in 197 different journals. The Journal Citation Reports (JCR) partitions, number of publications, impact factors, and proportion of publications in the top 10 journals with the numbers of publications and co-citations are shown in and . Most journals were in the fields of otolaryngology, thoracic surgery, and materials science. The Biomaterials journal ranked first overall for publications and co-citations, at 28 (5.895%) papers and 357 citations. Based on its impact factor (15.304) and JCR partition (Q1), it is clear that this is a high-quality journal in the field of materials science. Moreover, under the JCR evaluation system, six of the 10 journals with high publication volumes were classified as high-quality scientific publications, and the proportion of high-quality journals among the journals with high citation levels was up to 80%. Among them were the top journals in medical science, such as the Lancet and New England Journal of Medicine.
Figure 4. Subject distribution map and a dual-map overlay. (a) articles published in top 10 journals. Blocks of different colors represent different journals. (b) the dual-map overlay of journals that published articles on tissue-engineering trachea. Each small dot represents a journal, and different colors represent different clusters (cluster keyword: subject). The left area of the image represents the journals in which papers were published, the right area represents the cited journals, and the paths of different colors represent citation relationships between journals [Citation21]. There are four reference routes: two orange and two green paths.
![Figure 4. Subject distribution map and a dual-map overlay. (a) articles published in top 10 journals. Blocks of different colors represent different journals. (b) the dual-map overlay of journals that published articles on tissue-engineering trachea. Each small dot represents a journal, and different colors represent different clusters (cluster keyword: subject). The left area of the image represents the journals in which papers were published, the right area represents the cited journals, and the paths of different colors represent citation relationships between journals [Citation21]. There are four reference routes: two orange and two green paths.](/cms/asset/f53023e3-b22a-4be4-9a1b-78d64a87a790/kbie_a_2274150_f0004_oc.jpg)
Table 1. Top 10 journals in terms of the number of publications and co-citation frequency.
A dual-map overlay was applied to the discipline and journal data to link these analyses [Citation21] () . We observed that articles devoted to tissue-engineered trachea research were mainly published in journals related to physics, materials science, chemistry, molecular science, biology, and immunology, whereas references cited in these published articles were mainly published in journals related to chemistry, materials science, toxicology, molecular science, biology, nursing science, surgery, and genetics. Most studies were published in bioengineering journals (163), followed by those published in materials science (116) and surgery (88) journals. Therefore, research on tissue-engineered tracheas represents an organic combination of basic medical and engineering approaches. Multidisciplinary cooperative research is thus essential for developing effective long-segment tracheal repair technologies.
3.3. Author distribution study
A total of 223 authors were analyzed by VOSviewer, in which the minimum occurrence of a single author was set to three (). The nationalities and top 10 researchers in this field are shown in . Among them, the Italian scholar Paolo Macchiarini published 24 papers, accounting for 5.053% of the total field output, which played an important role in the development of tracheal tissue engineering. According to the clustering view analysis (), clusters in the center were associated with the Professor Macchiarini’s team from Italy (yellow), Professor Birchall’s team from the United States (red), Professor Nakamura’s team from Japan (green), and Professor Yong Xu’s team (dark blue), Xiaoxin He’s teaching team (orange), and Professor Hongcan Shi’s team (light blue) from China. Of these groups, those of Professor Macchiarini, Professor Birchall, and Professor Nakamura have relatively close cooperative relationships, whereas in China, Professor Yong Xu and Professor Xiaoxin He had many academic exchanges and performed much research in cooperation. Most of the achievements of Professors Macchiarini, Birchall, and Nakamura were between 2010 and 2016, whereas recent progress in the field of artificial tracheas is mostly described in articles from China. Nevertheless, the achievements of small isolated research teams in recent years should not be ignored ().
Figure 5. Research results and visualization of collaboration between different authors, countries, and institutions. (a) a circle represents an author, different colors represent different clusters, and lines between them represent partnerships. (b) a circle represents an author, different colors represent different years of research, darker colors are closer to the present, and lines between them represent collaborations. (c) a circle represents a country, the size of the circle is proportional to the number of publications, and the lines between circles represent partnerships. (d) a circle represents an institution, the size of the circle is proportional to the number of publications, and the lines between them represent partnerships. The thickness of the lines represents the closeness of cooperation between countries and institutions [Citation22,Citation23].
![Figure 5. Research results and visualization of collaboration between different authors, countries, and institutions. (a) a circle represents an author, different colors represent different clusters, and lines between them represent partnerships. (b) a circle represents an author, different colors represent different years of research, darker colors are closer to the present, and lines between them represent collaborations. (c) a circle represents a country, the size of the circle is proportional to the number of publications, and the lines between circles represent partnerships. (d) a circle represents an institution, the size of the circle is proportional to the number of publications, and the lines between them represent partnerships. The thickness of the lines represents the closeness of cooperation between countries and institutions [Citation22,Citation23].](/cms/asset/7c6d9f73-1230-4a43-b26a-35cb7003b228/kbie_a_2274150_f0005_oc.jpg)
Table 2. Top 10 authors in terms of the number of publications.
3.4. Analysis of countries and institutions
CiteSpace software was used to select and analyze different countries and institutions associated with the identified articles. A total of 37 nodes and 117 lines were generated in the preliminary analysis for countries/regions, with each node representing a country. The minimum number of screened publications was five () [Citation22,Citation23]. The United States ranked first with 133 papers, accounting for 28% of the total number of papers in this research field, nearly twice that of China (72 papers), which ranked second, and the United Kingdom ranked third with 57 papers. Overall, the worldwide distribution of tissue-engineered trachea research articles was mainly found in Asia, Europe, North America, and Oceania (). An in-depth analysis showed that six countries were responsible for the majority of published papers: the United States, China, the United Kingdom, Japan, South Korea, and Germany. Although other countries also published research on tissue-engineered trachea applications, their output was lower than that of the top six countries. The connections between the nodes were numerous and complicated, indicating that all countries cooperated in the research. However, only the United States, China, and the United Kingdom had a centrality > 0.1, indicating that these three countries had the closest cooperative relationships.
A total of 371 nodes and 626 lines were obtained from the preliminary analysis of the institutions. Institutions that published fewer than five articles were removed to improve the reliability of the analysis and clarity of the results. The research from the University College London was the most prominent compared to that of all global research institutions (), as these researchers from this University published 37 articles, followed by those from the Shanghai Jiao Tong University, Kyoto University, Royal National Ear, Nose and Throat Hospital, and Tongji University, with 27, 21, 20, and 17 articles, respectively.
3.5. Keyword Network and Research Hotspots
The top 20 keywords (excluding the search terms) are provided in . Among them, ‘Stem cells,’ ‘Scaffold’ and ‘In vitro’ were identified as the top three keywords in terms of frequency, and the centrality of ‘In vitro’ was found to be as high as 0.24. Stem cell research has always been the focus of tracheal tissue engineering, and most of the studies in this field included in vitro experiments. According to the analysis in , the tracheal tissue engineering keywords comprised seven clusters, of which only three were representative. Among all clusters, the largest one was ‘biomaterial,’ which covered in vitro differentiation of mesenchymal stem cells, preparation and application of hydrogels, and selection of biocompatible materials. The second largest cluster involved the preparation of scaffolds, which are most commonly used in acellular technology [Citation24] and 3D bioprinting [Citation25]. These keywords mainly concerned the preparation methods of tracheal stents, which are constantly updated with the development of science and technology. The third cluster focused on the surgical repair of the trachea. Different methods should be used to repair different tracheal pathologies (tracheal stenosis, infection, trauma, and tumors), depending on the severity of tracheal injury. These include tracheal end-to-end anastomosis [Citation26], trachea autogenous tissue reconstruction [Citation27], trachea allograft [Citation28], and tissue-engineered trachea [Citation29]. Moreover, the indications for tracheal reconstruction comprised a large part of this cluster. At the same time, analysis of the keyword sequence diagram () showed that studies on tracheal scaffolds, decellularization technology, and 3D bioprinting technology for research on tissue-engineered trachea are relatively novel and promising. Since 2019, studies on tissue-engineered trachea have become a research hotspot. In recent years, researchers have combined 3D bioprinting technology and biohydrogel materials to promote this therapy as an important means of treating patients with long-segment tracheal injuries.
Figure 7. Visualization of keywords and emergent words. (a, b) a node represents a keyword, and the size of the node is proportional to the number of times the keyword was used. The line between two nodes indicates that the two nodes appeared in the same article. Different colors represent different keyword clusters (a) or different years in which the keyword was used (b). (c) the top 25 breakout words. The dark blue line represents the year of the first occurrence, and the red line represents the duration of the eruption.
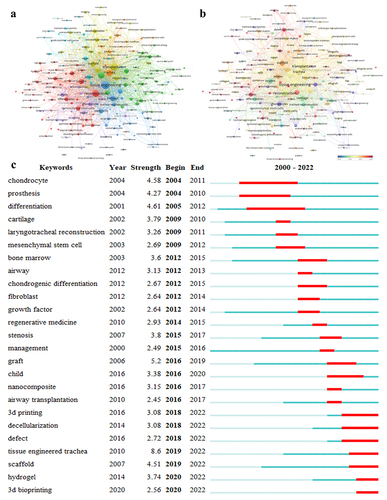
Table 3. Top 10 most frequent keywords.
The emergent keywords show the changing trends of the research hotspots for tracheal tissue engineering technologies. The earliest research in this field started with chondrocytes and gradually changed to the laryngotracheal reconstruction and tracheal prosthesis transplantation over time, followed by an in-depth exploration of various stent-supported mesenchymal stem cells. In recent years, acellular extracellular matrix, hydrogel biomaterials, and 3D bioprinting technologies have been widely studied in tissue-engineered trachea and become the focus of current research in this field ().
4. Discussion
4.1. General points
Bibliometric analyses assess published reports and utilize data such as publication year, author, source country and relevant institutions, keywords, and statistics. Further collation and analysis of the reported data are used to elucidate the overall state-of-the-art and development trends in the research field of interest, highlight current hotspots, predict future trends, and generally serve as a guiding ‘beacon’ for researchers [Citation30].
This study focuses on the application of tissue engineering technology combined with tracheal therapy. In the initial literature search stage, we selected the WOS core database to eliminate low-quality studies, and the search results showed an overall increasing trend from 2000 to 2023. The year 2010 was a turning point, and since then, the number of published studies in this field has substantially increased. This can be attributed to the outstanding contribution of Professor Macchiarini and his team in the field of tissue-engineered trachea research. In 2008, Macchiarini performed the first trachea transplant using a technique related to regenerative medicine (a decellularized trachea was used from a cadaver combined with somatic cell implantation) in a 30-year-old woman with left main bronchial atrophy. No adverse reactions were reported during a 4-month follow-up period [Citation31]. Since then, the tissue-engineered trachea technology has gradually entered the field of vision of researchers and clinical workers. With funding from a large number of foundations and participation of a large number of scholars, it is not surprising that the research output in this field has increased dramatically. The search results showed that most of the articles were from the United States and China, among which the United States ranked the first with 133 articles. A country’s academic ability is directly proportional to its economic strength. Given that the United States and some European countries (such as the United Kingdom, Germany, and France) are among the world’s most economically developed countries, it is unsurprising that they are responsible for large research outputs in this field [Citation32]. China, the most populous country in the world, has a considerable incidence of tracheal diseases in a large population base, which naturally requires greater investment in the treatment and research of such diseases [Citation33,Citation34]. Curiously, the large collaborative network of researchers in the United States ceased to exist at around 2012, and only a few scholars are still collaborating on tissue-engineered trachea research (). In contrast, China has formed a large cooperation network after 2018 led by Professors Yong Xu, Yunlang She, Xiaoxin He, and Hongcan Shi. Furthermore, Chinese research institutions, such as the Shanghai Jiao Tong University and Tongji University, are also in the top five by the research output in this area.
Journal analysis provides an understanding of the main application direction of the field, gives researchers some conventional recommendations for publication of results, and provide readers with more convenient and easy access to the latest research results [Citation35,Citation36]. Biomaterials is not only the journal with the highest number of papers published in this field but also the one with the highest number of co-citations. The selection and application of biomaterials are the key points in this field. The success of trachea transplantation must be based on the acquisition of suitable transplantation materials. Starting from constructing tracheal scaffolds with simple basic polycaprolactones [Citation37,Citation38], researchers have been trying to modify or prepare new composite materials to meet higher requirements for biocompatibility and mechanical properties [Citation39,Citation40]. Based on this factor, the rationality of the results is greatly improved, which of course is inseparable from the strict peer review system of the journal itself and the high requirements for research innovation and results [Citation41]. Comprehensive analysis shows that China, Japan, and South Korea are no worse than European and American countries, except for the United States, in terms of the number of articles published, but the source journals of high-quality research are mainly published by American and European publishers. The early establishment of high-quality professional academic journals published by Asian publishers can better promote the further development of tissue engineering field in East Asia and the entire Asian region.
4.2. Research frontier and hotspot mining
The most prominent aim of bibliometrics research is to look back through past published studies to find current research hotspots and future research trends [Citation42]. In our study, after removing the search term ‘Stem cells,’ ‘Scaffold,’ and ‘In vitro’ were the most commonly co-used keywords. Further exploration showed that keywords mainly focused on the preparation of artificial trachea and tended to be associated with in vitro experimental research. Although there were sporadic clinical studies, the results were generally unsatisfactory [Citation43,Citation44]. Hawkins and his team tried to induce targeted differentiation of human pluripotent stem cells into airway basal cells and demonstrated that these airway basal cells can promote epithelial regeneration in vivo or in vitro for trachea xenograft () [Citation45]. Moreover, bone marrow mesenchymal stem cells are also considered to have excellent performance in cell biology studies of tissue-engineered trachea. Their easy acquisition and high chondrogenic differentiation ability indicate that these cells are more suitable for clinical transformation application of tracheal transplantation than other cells, and tracheal scaffolds supported by these cells are more likely to survive in vivo () [Citation46,Citation49]. In the course of more than 20 years of research, the construction of artificial trachea has gradually changed from the original non-active scaffold using a single material to the application of a multi-material composite bioactive scaffold. This is due to the development of research and the progress of modern science and technology. Compared with acellular tracheal scaffolds and ordinary 3D printed scaffolds, the advanced extrude-based 3D bioprinted tracheal scaffolds developed by Park et al. can significantly reduce scaffold construction time, thus enhancing the activity and differentiation ability of seed cells. Polycaprolactone-derived tracheal scaffolds are also excellent with regard to their safety performance after transplantation and the general treatment needs of patients () [Citation47]. Moreover, biomaterials in the form of hydrogels are seen as a recent development and are gaining attention from researchers. Animal experiments have confirmed that tracheal stents constructed with composite materials containing hydrogels have advantages in terms of survival time and fewer complications compared with acellular scaffolds and polycaprolactone scaffolds alone [Citation50]. At the same time, it has been found that tracheal cartilage could be 3D bioprinted based on stem cells or nanoparticle – hydrogel composites, which could effectively enhance the antibacterial properties of the printed tissues and provide a good prognosis for patients () [Citation48,Citation51,Citation52]. In addition to hydrogels for 3D bioprinting that are currently a hot research topic, a 4D bioprinting hydrogel that includes the ‘time’ dimension is quietly emerging. Professor Kim reported that the 4D bioprinted silk protein hydrogel designed by his team could potentially be used for tissue engineering and biomedical applications in the future [Citation53].
Figure 8. Cell biology of tissue-engineered trachea and tracheal scaffold construction. (a) schematic diagram of targeted differentiation of airway epithelial cells from human induced pluripotent stem cells and staining for immunomarkers indicating xenografted epithelium. Adapted with permission from [Citation45], copyright 2021, Elsevier Ltd. (b) Microscopic immunohistochemical staining of neonatal chondrocytes and neovascularization from bone marrow mesenchymal stem cells. Adapted with permission from [Citation46], copyright 2018, MDPI. (c) a trachea-mimetic cellular construct of a clinically relevant size based on the advanced extruded 3D bio-printing technology. Adapted with permission from [Citation47], copyright 2021, Elsevier Ltd. (d) bacteriostatic characterization of 3D bioprinted scaffolds constructed based on gelatin methacrylamide mixed with novel superparamagnetic oxidation nanoparticles. Adapted with permission from [Citation48], copyright 2022, Elsevier Ltd.
![Figure 8. Cell biology of tissue-engineered trachea and tracheal scaffold construction. (a) schematic diagram of targeted differentiation of airway epithelial cells from human induced pluripotent stem cells and staining for immunomarkers indicating xenografted epithelium. Adapted with permission from [Citation45], copyright 2021, Elsevier Ltd. (b) Microscopic immunohistochemical staining of neonatal chondrocytes and neovascularization from bone marrow mesenchymal stem cells. Adapted with permission from [Citation46], copyright 2018, MDPI. (c) a trachea-mimetic cellular construct of a clinically relevant size based on the advanced extruded 3D bio-printing technology. Adapted with permission from [Citation47], copyright 2021, Elsevier Ltd. (d) bacteriostatic characterization of 3D bioprinted scaffolds constructed based on gelatin methacrylamide mixed with novel superparamagnetic oxidation nanoparticles. Adapted with permission from [Citation48], copyright 2022, Elsevier Ltd.](/cms/asset/274bc8f0-17de-40fe-8831-9d08a1f75373/kbie_a_2274150_f0008_oc.jpg)
Word analysis showed that terms ‘tracheal stent,’ ‘decellularization,’ ‘hydrogel,’ and ‘3D bioprinting technologies’ have been excessively used in this field since 2018, which is consistent with the overall development process of tracheal transplantation. From the initial treatment using donor tracheal tubes, acellular tracheal stent has gradually progressed to the combination of autologous seed cells of patients. Finally, with the progress of science and technology, it will step into the era of 3D bioprinted artificial trachea transplantation. The rapidly developing 3D bioprinting technology has abundant potential for future applications as a personalized treatment for long tracheal injuries. This technology combines a 3D matrix with patient stem cells cultured in vitro to ensure easy access and effectively avoid postoperative immune rejection, owing to biological matching [Citation54,Citation55]. From Professor Macchiarini’s first acellular tracheal stent used for human tracheal reconstruction, the field has advanced to the generation of a 3D bioprinted tracheal stent transplanted by Huang et al. to a 46-year-old female patient with tracheal collapse, achieving short-term success for at least 3 months. Regardless of whether tracheal injury is laryngeal or intrathoracic and whether it is a tracheal surface window rupture or a ring defect, tracheal tissue engineering using 3D bioprinting can prepare the most suitable tracheal model for patients within a relatively short time [Citation37,Citation56]. Although the early clinical treatment has been well received, it is challenging to obtain U.S. Food and Drug Administration or China Food and Drug Administration approvals for 3D bioprinting owing to the need to control many parameters, such as materials, processing technology, and structural design in the treatment process, to match each patient [Citation56]. Therefore, in recent years, few clinical studies on 3D bioprinted trachea have been published, and most of them remain at the preclinical in vivo stage.
After extensive literature analysis, we provide more accurate ideas for new studies for researchers in the field of tissue-engineered trachea. We outline these ideas in an attempt to facilitate solving hot problems in the field. At the same time, we found that in order to promote the sustainable development of the field, single-laboratory studies are not sufficient, and we must strengthen the cooperation of materials science researchers and medical professionals, who would learn from each other, carry out in-depth research in the current hot research directions (3D bioprinting/multi-material composite hydrogels/new decellularization technology) to discover new biocomposite materials, explore the combined application of multi-materials, and standardize the clinical trial process [Citation57].
4.3. Limitations
Although we attempted to avoid the influence of subjective factors on the results, this study had some limitations. Firstly, although most high-quality studies can be obtained from the WoS, the Scopus database and Google Scholar may also include important studies that could have been overlooked. Further, the fact that we considered only studies published in English may also impact the overview of research results. Secondly, only original studies and reviews were used whereas other types of papers were excluded, and this may have affected the integrity and comprehensiveness of our data.
5. Conclusions
We have comprehensively summarized the development of tissue-engineered trachea preparation over the past 20 years. The overall number of studies on this topic has been steadily increasing yearly since 2000, with the United States and China emerging as leaders in this field. Researchers from the University College London published the largest number of articles on this topic. Professor Macchiarini in Italy initiated research in this field. Professors Yong Xu, Xiaoxin He, and Hongcan Shi in China and Dr. Tendy Chiang in the United States have been the most active researchers. Currently, the main areas of tracheal tissue engineering studies are general research, development, and the application of biological materials. The current mainstream research direction involves the use of hydrogel materials for tracheal scaffold construction. Since the beginning of 2020, 3D bioprinting technology has become a focus point in the field. Furthermore, the rapid development of science and technology has gradually made 4D printing possible. To fully realize the future clinical translation of this research, new medical biomaterials should be created, combining existing materials to build artificial trachea scaffolds with superior performance.
Author contributions
XXY, SZM, and SYB conceived and designed the study. LY organized the database. SF performed statistical analysis. XXY wrote the first draft of the manuscript. XXY, SYB, and SYQ wrote sections of the manuscript. SHC participated in the structure design of the article, was responsible for the final review and proofreading of the article, and provided research funding. All authors contributed to the manuscript revision, and read, and approved the submitted final version.
Acknowledgments
We thank members of the teaching and research community in our group for their support and help with this study. We are grateful to the Yangzhou University for the high-quality experimental environment. We would like to thank Editage (www.editage.cn) for English language editing.
Disclosure statement
The authors declare that the research was conducted in the absence of any commercial or financial relationships that could be construed as a potential conflict of interest.
Data availability statement
The datasets for this study can be found in the [Web of Science] [https://www.webofscience.com/wos/alldb/basic-search].
Additional information
Funding
References
- Park JH, Hong JM, Ju YM, et al. A novel tissue-engineered trachea with a mechanical behavior similar to native trachea. Biomaterials. 2015;62:106–16. doi: 10.1016/j.biomaterials.2015.05.008
- Adamo D, Galaverni G, Genna VG, et al. The growing medical need for tracheal replacement: reconstructive strategies should overcome their limits. Front Bioeng Biotechnol. 2022;10:846632. doi: 10.3389/fbioe.2022.846632
- Etienne H, Fabre D, Gomez Caro A, et al. Tracheal replacement. Eur Respir J. 2018;51(2):1702211. doi: 10.1183/13993003.02211-2017
- Soriano L, Khalid T, Whelan D, et al. Development and clinical translation of tubular constructs for tracheal tissue engineering: a review. Eur Respir Rev. 2021;30(162):210154. doi: 10.1183/16000617.0154-2021
- Hassanzadeh P, Atyabi F, Dinarvand R. Tissue engineering: still facing a long way ahead. J Control Release. 2018;279:181–197. doi: 10.1016/j.jconrel.2018.04.024
- Boys AJ, Barron SL, Tilev D, et al. Building scaffolds for tubular tissue engineering. Front Bioeng Biotechnol. 2020;8:589960. doi: 10.3389/fbioe.2020.589960
- de Wit RJJ, van Dis DJ, Bertrand ME, et al. Scaffold-based tissue engineering: Supercritical carbon dioxide as an alternative method for decellularization and sterilization of dense materials. Acta Biomater. 2023;155:323–332. doi: 10.1016/j.actbio.2022.11.028
- O’Leary C, Soriano L, Fagan-Murphy A, et al. The fabrication and in vitro evaluation of retinoic acid-loaded electrospun composite biomaterials for tracheal tissue regeneration. Front Bioeng Biotechnol. 2020;8:190. doi: 10.3389/fbioe.2020.00190
- Galliger Z, Vogt CD, Panoskaltsis-Mortari A. 3D bioprinting for lungs and hollow organs. Transl Res. 2019;211:19–34. doi: 10.1016/j.trsl.2019.05.001
- Dhania S, Bernela M, Rani R, et al. Scaffolds the backbone of tissue engineering: advancements in use of polyhydroxyalkanoates (PHA). Int j biol macromol. 2022;208:243–259. doi: 10.1016/j.ijbiomac.2022.03.030
- Datta P, Ayan B, Ozbolat IT. Bioprinting for vascular and vascularized tissue biofabrication. Acta Biomater. 2017;51:1–20. doi: 10.1016/j.actbio.2017.01.035
- Low SS, Yew M, Lim CN, et al. Sonoproduction of nanobiomaterials - a critical review. Ultrason Sonochem. 2022;82:105887. doi: 10.1016/j.ultsonch.2021.105887
- Shin Low S, Nong Lim C, Yew M, et al. Recent ultrasound advancements for the manipulation of nanobiomaterials and nanoformulations for drug delivery. Ultrason Sonochem. 2021;80:105805. doi: 10.1016/j.ultsonch.2021.105805
- Yap JX, Leo CP, Mohd Yasin NH, et al. Recent advances of natural biopolymeric culture scaffold: synthesis and modification. Bioengineered. 2022;13(2):2226–2247. doi: 10.1080/21655979.2021.2024322
- Ni P, Ye S, Xiong S, et al. Nanocomposite hydrogel based on chitosan/laponite for sealing and repairing tracheoesophageal fistula. Int j biol macromol. 2023;126177. doi: 10.1016/j.ijbiomac.2023.126177
- Chen C, Dubin R, Kim MC. Emerging trends and new developments in regenerative medicine: a scientometric update (2000 – 2014). Expert Opin Biol Ther. 2014;14(9):1295–1317. doi: 10.1517/14712598.2014.920813
- Chen C, Hu Z, Liu S, et al. Emerging trends in regenerative medicine: a scientometric analysis in CiteSpace. Expert Opin Biol Ther. 2012;12(5):593–608. doi: 10.1517/14712598.2012.674507
- Wang J, Chi Y, Yang B, et al. The application of biomaterials in osteogenesis: a bibliometric and visualized analysis. Front Bioeng Biotechnol. 2022;10:998257. doi: 10.3389/fbioe.2022.998257
- Chen C, Song M, Glanzel W. Visualizing a field of research: a methodology of systematic scientometric reviews. PLoS One. 2019;14(10):e0223994. doi: 10.1371/journal.pone.0223994
- van Eck NJ, Waltman L. Software survey: VOSviewer, a computer program for bibliometric mapping. Scientometrics. 2010;84(2):523–538. doi: 10.1007/s11192-009-0146-3
- Ma D, Guan B, Song L, et al. A bibliometric analysis of exosomes in cardiovascular diseases from 2001 to 2021. Front Cardiovasc Med. 2021;8:734514. doi: 10.3389/fcvm.2021.734514
- Pan Y, Deng X, Zhuang Y, et al. Research trends around exercise rehabilitation among cancer patients: a bibliometrics and visualized knowledge graph analysis. Bio Med Res Int. 2022;2022:3755460. doi: 10.1155/2022/3755460
- Wang X, Li D, Huang X, et al. A bibliometric analysis and visualization of photothermal therapy on cancer. Transl Cancer Res. 2021;10:1204–1215. doi: 10.21037/tcr-20-2961
- Stocco E, Barbon S, Mammana M, et al. Preclinical and clinical orthotopic transplantation of decellularized/engineered tracheal scaffolds: a systematic literature review. J Tissue Eng. 2023;14:20417314231151826. doi: 10.1177/20417314231151826
- Matai I, Kaur G, Seyedsalehi A, et al. Progress in 3D bioprinting technology for tissue/organ regenerative engineering. Biomaterials. 2020;226:119536. doi: 10.1016/j.biomaterials.2019.119536
- He J, Xu X, Lan L, et al. End-to-side anastomosis in complex tracheal resection and reconstruction: a case series study. Transl Lung Cancer Res. 2022;11(2):165–172. doi: 10.21037/tlcr-22-32
- Ren J, Xu Y, Zhiyi G, et al. Reconstruction of the trachea and carina: surgical reconstruction, autologous tissue transplantation, allograft transplantation, and bioengineering. Thoracic Cancer. 2022;13(3):284–295. doi: 10.1111/1759-7714.14315
- Tsou KC, Hung WT, Ju YT, et al. Application of aortic allograft in trachea transplantation. J Formos Med Assoc. 2023;122(9):940–946. doi: 10.1016/j.jfma.2023.03.006
- Dharmadhikari S, Liu L, Shontz K, et al. Deconstructing tissue engineered trachea: assessing the role of synthetic scaffolds, segmental replacement and cell seeding on graft performance. Acta Biomater. 2020;102:181–191. doi: 10.1016/j.actbio.2019.11.008
- Ninkov A, Frank JR, Maggio LA. Bibliometrics: methods for studying academic publishing. Perspect Med Educ. 2022;11(3):173–176. doi: 10.1007/s40037-021-00695-4
- Macchiarini P, Jungebluth P, Go T, et al. Clinical transplantation of a tissue-engineered airway. Lancet (London, England). 2008;372:2023–2030. doi: 10.1016/s0140-6736(08)61598-6
- Schneider L, Murray P, Lévy R, et al. Time to retract Lancet paper on tissue engineered trachea transplants. BMJ. 2022;376:o498. doi: 10.1136/bmj.o498
- Xiao D, Chen Z, Wu S, et al. Prevalence and risk factors of small airway dysfunction, and association with smoking, in China: findings from a national cross-sectional study. Lancet Respir Med. 2020;8(11):1081–1093. doi: 10.1016/s2213-2600(20)30155-7
- Tu WJ, Zeng X, Liu Q. Aging tsunami coming: the main finding from China’s seventh national population census. Aging Clin Exp Res. 2022;34(5):1159–1163. doi: 10.1007/s40520-021-02017-4
- Sun HL, Bai W, Li XH, et al. Schizophrenia and inflammation research: a bibliometric analysis. Front Immunol. 2022;13:907851. doi: 10.3389/fimmu.2022.907851
- Zhao Y, Zhang X, Song Z, et al. Bibliometric analysis of ATAC-Seq and its use in cancer biology via nucleic acid detection. Front Med. 2020;7:584728. doi: 10.3389/fmed.2020.584728
- Ke D, Yi H, Est-Witte S, et al. Bioprinted trachea constructs with patient-matched design, mechanical and biological properties. Biofabrication. 2019;12(1):015022. doi: 10.1088/1758-5090/ab5354
- Park JY, Ryu H, Lee B, et al. Development of a functional airway-on-a-chip by 3D cell printing. Biofabrication. 2018;11(1):015002. doi: 10.1088/1758-5090/aae545
- Khalid T, Soriano L, Lemoine M, et al. Development of tissue-engineered tracheal scaffold with refined mechanical properties and vascularisation for tracheal regeneration. Front Bioeng Biotechnol. 2023;11:1187500. doi: 10.3389/fbioe.2023.1187500
- Sun F, Shen Z, Zhang B, et al. Biomimetic in situ tracheal microvascularization for segmental tracheal reconstruction in one-step. Bioengineering & translational medicine. Bioeng Transl Med. 2023;8(4):e10534. doi: 10.1002/btm2.10534
- Maksoud FJ, Velázquez de la Paz MF, Hann AJ, et al. Porous biomaterials for tissue engineering: a review. J Mat Chem B. 2022;10(40):8111–8165. doi: 10.1039/d1tb02628c
- Wang J, Maniruzzaman M. A global bibliometric and visualized analysis of bacteria-mediated cancer therapy. Drug Discovery Today. 2022;27(10):103297. doi: 10.1016/j.drudis.2022.05.023
- Elliott MJ, Butler CR, Varanou-Jenkins A, et al. Tracheal replacement therapy with a stem cell-seeded graft: lessons from compassionate use application of a GMP-Compliant tissue-engineered medicine. Stem Cells Transl Med. 2017;6(6):1458–1464. doi: 10.1002/sctm.16-0443
- Tan Q, Liu R, Chen X, et al. Clinic application of tissue engineered bronchus for lung cancer treatment. J Thorac Dis. 2017;9:22–29. doi: 10.21037/jtd.2017.01.50
- Hawkins FJ, Suzuki S, Beermann ML, et al. Derivation of airway basal stem cells from human pluripotent stem cells. Cell Stem Cell. 2021;28(1):79–95.e78. doi: 10.1016/j.stem.2020.09.017
- Bae SW, Lee KW, Park JH, et al. 3D bioprinted artificial trachea with epithelial cells and chondrogenic-differentiated bone marrow-derived mesenchymal stem cells. Int J Mol Sci. 2018;19(6):1624. doi: 10.3390/ijms19061624
- Park JH, Ahn M, Park SH, et al. 3D bioprinting of a trachea-mimetic cellular construct of a clinically relevant size. Biomaterials. 2021;279:121246. doi: 10.1016/j.biomaterials.2021.121246
- Theus AS, Ning L, Kabboul G, et al. 3D bioprinting of nanoparticle-laden hydrogel scaffolds with enhanced antibacterial and imaging properties. iScience. 2022;25(9):104947. doi: 10.1016/j.isci.2022.104947
- Xu Y, Dai J, Zhu X, et al. Biomimetic trachea engineering via a modular ring strategy based on bone-marrow stem cells and atelocollagen for use in extensive tracheal reconstruction. Adv Mater (Deerfield Beach, Fla). 2022;34(6):e2106755. doi: 10.1002/adma.202106755
- Pan S, Shen Z, Xia T, et al. Hydrogel modification of 3D printing hybrid tracheal scaffold to construct an orthotopic transplantation. Am J Transl Res. 2022;14:2910–2925.
- Dell AC, Wagner G, Own J, et al. 3D bioprinting using hydrogels: cell inks and tissue engineering applications. Pharmaceutics. 2022;14(12):2596. doi: 10.3390/pharmaceutics14122596
- Yang Z, Yi P, Liu Z, et al. Stem Cell-Laden Hydrogel-Based 3D Bioprinting for Bone and Cartilage Tissue Engineering. Front Bioeng Biotechnol. 2022;10:865770. doi: 10.3389/fbioe.2022.865770
- Kim SH, Seo YB, Yeon YK, et al. 4D-bioprinted silk hydrogels for tissue engineering. Biomaterials. 2020;260:120281. doi: 10.1016/j.biomaterials.2020.120281
- Lam EHY, Yu F, Zhu S, et al. 3D bioprinting for next-generation personalized medicine. Int J Mol Sci. 2023;24(7):6357. doi: 10.3390/ijms24076357
- Yildirim Ö, Arslan-Yildiz A. Development of a hydrocolloid bio-ink for 3D bioprinting. Biomater Sci. 2022;10:6707–6717. doi: 10.1039/d2bm01184k
- Huang L, Wang L, He J, et al. Tracheal suspension by using 3-dimensional printed personalized scaffold in a patient with tracheomalacia. J Thorac Dis. 2016;8:3323–3328. doi: 10.21037/jtd.2016.10.53
- Murray P. The trachea transplant scandal and “compassionate use”. BMJ. 2023;382:1808. doi: 10.1136/bmj.p1808