Abstract
Background
The use of lipid-lowering drugs is still highly controversial in patients with amyotrophic lateral sclerosis (ALS). We performed a drug-target Mendelian randomization (MR) analysis to investigate the effect of targeted lipid-lowering drugs on the risk of ALS.
Methods
First, we evaluated the causal relationship between HMG-CoA (3-hydroxy-3-methylglutaryl coenzyme A) reductase (HMGCR) inhibitors-taking trait and ALS using a bidirectional two-sample MR study. Second, we investigated the causal relationship between lipid-lowering drugs and ALS through a drug-target MR approach. The summary data for HMGCR inhibitors-taking traits were extracted from a genome-wide association study (GWAS) of medication use and associated disease in the UK Biobank. The summary data for low-density lipoprotein cholesterol and apolipoprotein B (apoB) were extracted from a meta-analysis of GWAS in individuals of European ancestry in the UKB. The GWAS summary data of ALS were obtained from the Project MinE.
Results
Our bidirectional two-sample MR showed that genetically determined increased HMGCR inhibitors-taking trait was an independent risk factor for ALS (odds ratio [OR] = 1.090, 95% confidence interval [CI] = 1.035–1.150, p = 0.001). The results of drug-target MR showed that the increased expression of the HMGCR gene in blood with the higher risk of ALS (OR = 1.21, 95% CI = 1.01–1.46; p = 0.042) through SMR method and the apoB level mediated by the APOB gene increased the risk of ALS (OR = 1.15; 95% CI =1.05–1.25; p = 0.001) through inverse-variance weighted MR method.
Conclusion
This present study provides genetic support for a positive causal effect of HMGCR inhibitors-taking trait and ALS. The reason for this may be due to the underlying disease condition behind the medication, rather than the medication itself. Our findings also suggested that HMGCR and apoB inhibitors may have potential protective effects on ALS.
1. Introduction
Amyotrophic lateral sclerosis (ALS) is a progressive and fatal neurodegenerative disease characterized by selective motor neuron degeneration leading to progressive muscle weakness and paralysis, with a median survival of about 3 years after symptom onset (Citation1). Nerve dysfunction not only affects the quality of life and life span of patients with ALS but also greatly increases the social burden. ALS is a heterogeneous disease with a complex pathogenesis and still lacks effective interventions. So far, only riluzole, an antiglutamate agent, and the antioxidant edaravone have been approved by the Food and Drug Administration (FDA) for treatment to slow the progression of ALS. It is of vital importance to identify the causal risk factors of ALS and formulate preventive strategies. The highest age of onset among patients with ALS is between 60 years and 79 years (Citation2), an age with a high risk of developing atherosclerotic vascular disease (ASVD). Compared with the general population, dyslipidemia occurs more frequently in patients with ALS (Citation3,Citation4), and the incidence of sudden cardiac death and heart failure has also been reported in 6%–10% (Citation5). The use of lipid-lowering drugs can reduce the risk of cardiovascular disease (Citation6).
However, the use of lipid-lowering drugs is still highly controversial in patients with ALS. Existing studies on the effects of statins on ALS have yielded conflicting results (Citation7–25). ALS-like symptoms have been reported to be associated with statins (Citation7,Citation8), and some studies suggested statins may affect the rate of disease progression (Citation7,Citation9,Citation10). Several recent meta-analyses and systematic reviews indicated that there existed no strong evidence to link the use of statins with the risk of ALS (Citation15,Citation16,Citation24). However, a large retrospective study suggested that statins, especially lipophilic statins, and fibrates could reduce the risk of ALS (Citation26). Apart from statins, there are few studies on the relationship between other lipid-lowering drugs and ALS. The inconsistencies of these studies may be due to the influence of unavoidable confounders and sample size issues. Furthermore, randomized controlled trials (RCTs) are difficult to carry out because of ethics and cost. In view of the above, whether lipid-lowering agents can be prescribed when hyperlipidemia and/or ASVD are present in patients with ALS remains a pressing clinical question, and there exists an urgent need for a clear statement to guide clinical management.
Mendelian randomization (MR) is a novel epidemiological approach in which genetic variants are used as instruments for the estimation of the causal relationship between a risk factor of interest and an outcome (Citation27,Citation28). Additionally, MR can provide vital information for drugs. The expression or function of protein drug targets can be influenced by variants within or near the genes that code for them, and the genetic effects can be used to predict the effects of a drug, also known as drug-target MR (Citation29). Because genetic variants are inherited approximately randomly and assigned at birth, associations between exposures and disease outcomes are not expected to be biased by confounders and reverse causation as seen in observational studies. We designed a bidirectional two-sample MR study to investigate the causal relationship between HMG-CoA (3-hydroxy-3-methylglutaryl coenzyme A) reductase (HMGCR) inhibitors-taking trait and ALS. Then, we performed a drug-target MR between HMGCR inhibitors and ALS. As proprotein convertase subtilisin/kexin type 9 (PCSK9) inhibitors, Niemann–Pick C1-like 1 (NPC1L1) inhibitors and apolipoprotein B (apoB) inhibitors are widely used in clinical practice, we analyzed the targets of these three lipid-lowering drugs together.
2. Materials and methods
2.1. Study design
All data used in this MR study were from publicly available summary-level data from genome-wide association studies (GWAS) and expression quantitative trait loci (eQTL) studies. The ethical approval included can be found in the original articles.
First, we performed a bidirectional two-sample MR study to investigate the causal relationship between HMGCR inhibitors-taking trait and ALS. Second, we conducted a drug-target MR study. We performed summary data-based MR (SMR) analysis to investigate the association of gene expression from eQTL studies, in which the most significant cis-eQTL single nucleotide polymorphism (SNP) was selected as the genetic instrument for the target gene expression (HMGCR, PCSK9, NPC1L1, and APOB) of each lipid-lowering drug, with ALS using summary data from an open GWAS. In addition, we used a random-effect inverse-variance weighted (IVW) MR method to investigate the causal association between lipid-lowering drugs and ALS, in which we used genetic variants related to low-density lipoprotein-cholesterol (LDL-C) level and apoB level mediated by these target genes as a proxy for exposure to lipid-lowering drugs. For SMR method analysis, we investigated the association of exposures of interest with LDL-C level or apoB level as a positive control study for the instrument from eQTLs. For IVW MR method analysis, positive control analysis was performed with coronary heart disease as an outcome. Reporting guidelines are in accordance with the STROBE-MR statement (Citation30). The general process of this study is shown in . Details for data sources are presented in Supplementary Table S1.
Figure 1 Flow chart showing the process for the Mendelian randomization analyses. eQTL: expression quantitative trait loci; ALS: amyotrophic lateral sclerosis; MAF: minor allele frequency; GWAS: genome-wide association studies; SMR: summary data-based mendelian randomization; IVW: inverse-variance weighted.
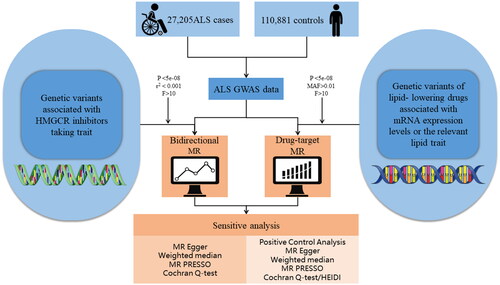
2.2. Instrumental variable selection
GWAS data for HMGCR inhibitors-taking traits were extracted from a case–control GWAS meta-analysis conducted among 318,177 European ancestry individuals from the UK Biobank (UKB) (Citation31). The definition of HMGCR inhibitor use was self-reported use of regular medications and health supplements taken weekly, monthly, or 3-monthly. Only SNPs that were independently (r2 < 0.001) associated with HMGCR inhibitors-taking trait at the genome-wide significance level (p < 5.0 × 10−8) and were rigorously selected as instrumental variables (IVs).
Available eQTLs for drug-target genes (HMGCR, PCSK9, NPC1L1, and APOB) were used as a proxy for exposure to each lipid-lowering drug. Our eQTLs summary data were obtained from eQTLGen (https://www.eqtlgen.org/) or GTEx V8 (https://gtexportal.org/). We identified common (minor allele frequency [MAF] > 1%) eQTLs SNPs significantly (p < 5.0 × 10−8) associated with blood HMGCR or PCSK9 expression and subcutaneous adipose NPC1L1 or APOB expression, as no blood or other tissue eQTLs were available at significance level for NPC1L1 and APOB. Only cis-eQTLs, defined as eQTLs within 1 Mb on either side of the coding gene, were included in this study to generate IVs.
Then, we additionally used IVs by selecting SNPs within 100kb windows from the target gene at a genome-wide significance level (p < 5.0 × 10−8) to proxy for lipid-lowering drug exposure. The summary data for LDL-C (n = 440,546) and apoB (n = 439,214) were extracted from a meta-analysis of GWAS in individuals of European ancestry in the UKB (Citation32), and only common SNPs (MAF >1%) were included. Aimed to maximize the power of the instrument for each drug, SNPs used as IVs were allowed to be in low weak linkage disequilibrium (r2 < 0.30) with each other. Details are shown in .
Figure 2 Information of genetic instruments. HMGCR: HMG-CoA reductase; PCSK9: proprotein convertase subtilisin/kexin type 9; NPC1L1: Niemann–Pick C1-like 1; APOB: apolipoprotein B; SMR: summary data-based mendelian randomization; eQTL: expression quantitative trait loci; MAF: minor allele frequency; SNP: single nucleotide polymorphism; LDL: low-density lipoprotein.
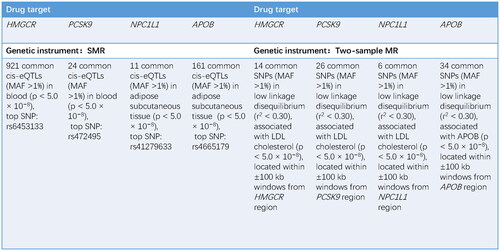
2.3. Outcome sources
The GWAS summary data of ALS were obtained from Project MinE, including 27,205 patients with ALS and 110,881 controls of European ancestry (Citation33). So far, it is the largest GWAS summary data about ALS. In the bidirectional two-sample MR study, The IVs of ALS were selected to meet the requirement of being independent (r2 < 0.001) and significant at the genome-wide level.
2.4. MR analyses
2.4.1. Primary MR analysis
The bidirectional two-sample MR study was conducted using the “Two-Sample MR” (version 0.5.6) in the statistical program R (version 4.2.2). The SMR approach was used to generate effect estimates and examine the association between the expression level of the drug-target genes and ALS, using summary data from eQTL and GWAS studies (Citation34). Allele harmonization and analysis were performed using SMR software, version 1.03 (https://cnsgenomics.com/software/smr/#Overview). We used two-sample MR analysis as another method for drug-target MR, in which the random-effect IVW method was the primary MR approach. IVW analysis was conducted using the “Two-Sample MR” (version 0.5.6) in the statistical program R (version 4.2.2). A two-sided p-value below 0.05 was considered statistically significant.
2.4.2. Sensitivity analysis
To minimize the bias caused by weak instruments, we only included SNPs with an F-statistic >10. For SMR method, the heterogeneity in dependent instruments (HEIDI) test was used to evaluate the existence of linkage in the observed association. If the p-value of HEIDI test is less than 0.05, the association is probably due to linkage (Citation35).
For the two-sample MR method, we applied the weighted median (WM) method and the MR-Egger method to validate the results of the IVW method. We tested for heterogeneity using Cochran’s Q test, with p < 0.05 indicating evidence of heterogeneity (Citation36). We used MR-Egger regression and Mendelian Randomization Pleiotropy RESidual Sum and Outlier (MR-PRESSO) to assess the potential horizontal pleiotropy of the SNPs used as instrument variants. In MR-Egger regression, the intercept term is a useful indicator of directional horizontal pleiotropy, with p < 0.05 indicating evidence of horizontal pleiotropy (Citation37). MR-PRESSO analysis can identify horizontal pleiotropy outliers and provide adjusted estimates, where p < 0.05 for the global test indicates the presence of horizontal pleiotropy outliers (Citation38). Leave-one-out analysis was used to assess the possibility that the results were driven by a single SNP.
2.4.3. Positive control analysis
We performed a positive control analysis in order to verify the reliability of IVs that were selected in our study. For IVW MR method, we conducted a positive control study by investigating the effect of IVs we selected for lipid-lowering drugs on coronary heart disease which is the main indication of lipid-lowering drugs. For SMR method, we chose LDL-C level or apoB level as the outcome of a positive control study.
3. Results
3.1. Genetic instruments selection
78 SNPs were screened as IVs of HMGCR inhibitors-taking trait and 12 SNPs were screened as IVs of ALS. We identified 921, 24, 11, and 161 cis-eQTLs from eQTLGen or GTEx Consortium for drug target genes HMGCR, PCSK9, NPC1L1, and APOB, respectively. The top cis-eQTL SNP was selected as a genetic instrument for the target gene of each lipid-lowering drug. A total of 7, 30, and 6 SNPs at or nearby genes HMGCR, PCSK9, and NPC1L1 were selected from a GWAS summary data of LDL-C levels from the UKB, respectively, and 9 SNPs within or nearby the APOB were selected from a GWAS summary data of apoB levels from the UKB (Supplementary Table S5). We calculated the F-statistics, and the F-statistics of all SNPs were over 10. For positive control analysis, based on SMR method showed significant associations between each drug-target gene and LDL-C level or apoB level when using eQTLs-proposed instruments (Supplementary Table S8), as well as between each drug and coronary heart disease when using LDL-C or apoB GWAS-proposed instruments (Supplementary Table S7, Supplementary Figures S5–S8), further ensuring the efficacy of the selected genetic instruments.
3.2. Bidirectional two-sample MR study between HMGCR inhibitors-taking trait and ALS
In our study, SNPs related to HMGCR inhibitors-taking trait and ALS were utilized to investigate the causal relationship between HMGCR inhibitors-taking trait and ALS. The detailed information is displayed in Supplementary Table S2(a,b), and the main results are visualized in and . We found that HMGCR inhibitors-taking trait was an independent risk factor for ALS (IVW odds ratio [OR] = 1.090, 95% confidence interval [CI] = 1.035–1.150, p = 0.001; WM OR= 1.110, 95%CI = 1.024–1.203, p = 0.009; ). The directional consistency of MR-Egger results (Supplementary Table S3(a), ) supported the robustness of the results. The MR-PRESSO global test showed suggestive horizontal pleiotropy for IVs (p = 0.030), which was not supported by the MR-Egger intercept (intercept = 0.001, p = 0.758). Meanwhile, MR-PRESSO showed that there was no influence of outliers. The heterogeneity of IVs was not observed by Cochran’s Q test. The leave-one-out analysis showed that the removal of any single SNP did not change the result (). In the reverse MR exploration, no evidence was found that ALS caused changed HMGCR inhibitors-taking (Supplementary Table S3(b), Supplementary Figure 9(a,b)). No heterogeneity and horizontal pleiotropy were found in reverse MR analysis (Supplementary Table S3(b)).
3.3. SMR method analysis for drug-target MR
The SMR method was conducted to evaluate the association between the gene expression of HMGCR, PCSK9, NPC1L1, or APOB and ALS. We used the most significant cis-eQTL SNP (rs6453133, rs472495, rs41279633, and rs4665179) as an IV for the target gene of each lipid-lowering drug. The results of SMR method showed evidence for the association of the increased expression of the HMGCR gene in blood with the higher risk of ALS (OR = 1.212, 95% CI = 1.01–1.46; p = 0.042; ). There was no evidence of association between the expression of the PCSK9 (OR= 1.10, 95% CI =0.97–1.24; p = 0.143; ), NPC1L1 (OR = 1.00, 95% CI = 0.93–1.06; p = 0.909; ), and APOB (OR = 1.02, 95% CI = 0.97–1.08; p = 0.411; ) genes and the risk of ALS. The results indicated that genetically proxied HMGCR inhibitors might lower the risk of ALS. HEIDI test suggested that all observed associations were not due to a linkage (p > 0.05). Details are shown in Supplementary Table S4.
3.4. IVW MR method analysis for drug-target MR
IVW MR method analysis results indicated that the apoB level mediated by the APOB gene increased the risk of ALS (OR = 1.15; 95% CI =1.05–1.25; p = 0.001; ). No heterogeneity or horizontal pleiotropy was detected by Cochran’s Q test, MR-Egger, and MR-PRESSO. IVW MR method analysis results were also supported by MR-Egger method and WM method (Supplementary Table S6). The leave-one-out analysis showed that the results were not driven by a single SNP (Supplementary Figure 4(b)). However, none of the IVW MR methods indicated any causal effects of genetically predicted LDL-C levels mediated by the HMGCR (OR = 1.09; 95% CI = 0.86–1.37; p = 0.488; ), PCSK9 (OR = 1.17; 95% CI = 0.98–1.39; p = 0.075; ), and NPC1L1 (OR = 0.88; 95% CI = 0.53–1.46; p = 0.628; ) genes on the risk of ALS. Cochran’s Q test did not find evidence of heterogeneity for all reported results (all ps > 0.05). The intercepts term in MR-Egger regression analysis suggested no overall horizontal pleiotropy (all ps > 0.05) except the relationship between LDL-C levels mediated by the PCSK9 and ALS (p = 0.035, Supplementary Table S6). All MR-PRESSO analyses suggested no overall horizontal pleiotropy (all ps > 0.05). Details are shown in Supplementary Table S6, and the main results are visualized in Supplementary Figures S1–S4.
4. Discussion
Aiming to investigate the causal relationships between HMGCR inhibitors-taking trait and ALS, we conducted a bidirectional two-sample MR study. We found that HMGCR inhibitors-taking trait was an independent risk factor for ALS. To clarify whether this causal relationship is due to genetic variation in targets of lipid-lowering drugs, we also performed a drug-target MR. In drug-target MR, four lipid-lowering drug-target genes were selected as the exposure. We conducted a causal relationship study between targets of four lipid-lowering drugs and ALS. We used two methods to evaluate the effect of these lipid-lowering drugs on ALS. We found that genetic variation in HMGCR was related to an increased risk of ALS through SMR method, while the IVW method showed consistency in direction. We also found that apoB levels mediated by APOB gene increased the risk of ALS using the IVW method, while the SMR method showed consistency in direction. The study of two primary methods in drug-target MR did not show that any of the four lipid-lowering drugs would increase the risk of ALS. HMGCR inhibitors are the cornerstone treatment for hyperlipidemia and coronary heart disease. Hyperlipidemia and coronary heart disease may be known risk factors for ALS (Citation39,Citation40). Therefore, we speculated that the increased risk of ALS caused by drug use may be due to the underlying disease behind the drug use. Similarly, an MR study of the relationship between 23 medication use traits and stroke found that the use of HMGCR inhibitors, antihypertensive drugs, antithrombotics agents, etc. increased the risk of stroke, which is obviously contrary to medical common sense (Citation41).
Our study found that genetically proxied HMGCR inhibition and apoB inhibition have a protective effect on ALS and may reduce the risk of ALS, while other types of lipid-lowering drugs in this study have no causal effect on ALS. In other words, no other lipid-lowering drugs were found to be a risk factor in the prediction of drug effect of genetic variation. The research on the relationship between lipid-lowering drugs and ALS mainly focused on statins, and there has been no RCT on the relationship between statins and ALS. Two separate population-based studies (Citation13,Citation19) found that statins were largely unrelated to ALS diagnosis (OR = 1.08; 95% CI = 0.98–1.19), but were associated with an increased risk of ALS in women (OR = 1.28; 95% CI = 1.10–1.48), particularly in the first year after initiation. However, some recent meta-analyses and systematic reviews carried out by Nabizadeh (Citation24) (RR = 0.98; 95% CI = 0.80–1.20), Hu (Citation16) (OR = 0.92; 95%CI = 0.83–1.03), Cui (Citation15) and others have indicated that there existed no strong evidence to link the use of statins to the risk of developing ALS. However, a large retrospective study conducted by Freedman et al (Citation26), including more than 10,000 patients with ALS, found that statins and fibrates were associated with lower ALS risk. This is similar to our findings. The contradictions among these studies are mainly attributed to the bias brought by confounders. For instance, the protective effect of statins on ALS may be interfered with by the effect of blood lipids, because an MR study (Citation42) and a prospective cohort study (Citation39) have provided evidence of a causal link between some lipid biomarker levels and ALS. In view of the above, MR, an epidemiological approach that assesses the causal effect of exposures on an outcome, can overcome these potential limitations.
This drug-target MR study suggested that the inhibitors of HMGCR and apoB are causally associated with reduced risk of ALS. We suggested that the association of HMGCR inhibition on ALS appeared to be independent of circulating LDL-C levels because we did not observe an overall association of LDL-C with the risk of ALS and analyses involving SNPs in the NPC1L1, PCSK9 showed no causal estimates on ALS. Statins are inhibitors of HMG-CoA (the rate-limiting enzyme in the mevalonate pathway) reductase, mainly prescribed to treat hyperlipidemia and prevent coronary heart disease and stroke. Besides, Statins also have some effects of antioxidative and anti-inflammatory (Citation43) and can improve endothelial function (Citation44). Oxidative stress (Citation45) and inflammatory mechanisms (Citation2) are important contributors to ALS. Statins may produce neuroprotection through these mechanisms. A study (Citation46) showed atorvastatin protected NSC-34 motor neurons against oxidative stress by activating PI3K, ERK, and free radical scavenging. In the case of ALS, enhancing axonal regeneration has the potential to increase muscle strength over the course of the disease. One study indicated that the mevalonate-prenylation pathway constituted an endogenous brake on the growth of axons (Citation47). A recent study found that lovastatin may reduce the risk of ALS, and the results were validated in an ALS mouse model (Citation48). Therefore, we speculate that the disruption of mevalonate synthesis by inhibiting HMGCR in the cholesterol biosynthetic pathway may promote neurite outgrowth to exert a protective effect in ALS.
The potential mechanisms underlying the relationship between apoB inhibitors and ALS remain unclear. The apoB is an essential component of all atherogenic lipoproteins and one apoB molecule is included in each circulating atherogenic lipoprotein particle. Available evidence indicated a stronger effect of apoB compared to LDL-C on cardiovascular disease (Citation49). In terms of the nervous system, through a series of cerebrospinal fluid (CSF) filtration studies and global proteomic analysis, researchers identified apoB in the CSF as the agent responsible for the induction of motor disability, motor neuron degeneration, and pathological translocation of TAR DNA-binding protein-43 (TDP-43) in sporadic ALS. Targeted removal of apoB successfully attenuated the neurotoxic capacity and improved motor disability, motor neuron death, and TDP-43 translocation (Citation50). Therefore, the inhibition of apoB may not only reduce the level of LDL-C but may also exert a protective role for ALS in some other way through its intrinsic properties. However, the mechanisms that underpin them still need to be studied.
5. Study limitations
The main strengths of this study are as follows: our sample size is sufficiently large to detect significant changes in ALS risk due to genetically predicted responses to lipid-lowering drugs. In addition, the methods we use there are less susceptible to confounding bias and reverse causation than observational studies. Moreover, we used a variety of sensitivity analyses, including positive controls, to test the efficacy of genetic instruments and the assumptions of the MR study. Our research has several limitations as well. Based on the method used, MR cannot replace authentic RCTs of medicines, and lifelong exposure to MR differs from a shorter duration of pharmacological intervention. Because of the blood-brain barrier, the cholesterol level in CNS may not be related to the blood level. Due to the lack of cholesterol GWAS data for the central nervous system, it is not possible to directly verify the conclusions. Additionally, the results of the two analytical methods we used were not cross-validated to reach a consistent conclusion. Lastly, based on the disease itself, we were also unable to test for differences in outcomes between patients receiving lipid-lowering drugs at different stages.
6. Conclusions
Taken together with the genetic data, our findings suggested that HMGCR and apoB inhibitors may have potential protective effects on ALS while proprotein convertase subtilisin/kexin type 9 and Niemann–Pick C1-like 1 inhibitors did not increase the risk of ALS. Statins and apoB inhibitors preventing and therapying ASVD may become effective supplemental treatments for patients with ALS with hyperlipidemia, although large randomized controlled clinical trials are needed.
Supplemental Material
Download Zip (1.2 MB)Acknowledgements
We appreciated the generous sharing of GWAS summary statistics from the UK Biobank and the Project MinE.
Declaration of interest
The authors report no conflicts of interest. The authors alone are responsible for the content and writing of this article.
Data availability statement
The summary statistics used in the current study are available from the corresponding author upon reasonable request. Detailed information on the summarized data sources for the instrumental variables is presented in Supplementary Table S1.
References
- Masrori P, Van Damme P. Amyotrophic lateral sclerosis: a clinical review. Eur J Neurol. 2020;27:1918–29.
- Feldman EL, Goutman SA, Petri S, Mazzini L, Savelieff MG, Shaw PJ, et al. Amyotrophic lateral sclerosis. Lancet. 2022;400:1363–80.
- Chełstowska B, Barańczyk-Kuźma A, Kuźma-Kozakiewicz M. Dyslipidemia in patients with amyotrophic lateral sclerosis – a case control retrospective study. Amyotroph Lateral Scler Frontotemporal Degener. 2021;22:195–205.
- Dupuis L, Corcia P, Fergani A, Gonzalez De Aguilar JL, Bonnefont-Rousselot D, Bittar R, et al. Dyslipidemia is a protective factor in amyotrophic lateral sclerosis. Neurology. 2008;70:1004–9.
- Pereira M, Gromicho M, Henriques A, Pronto-Laborinho AC, Grosskreutz J, Kuźma-Kozakiewicz M, et al. Cardiovascular comorbidities in amyotrophic lateral sclerosis. J Neurol Sci. 2021;421:117292.
- Delahoy PJ, Magliano DJ, Webb K, Grobler M, Liew D. The relationship between reduction in low-density lipoprotein cholesterol by statins and reduction in risk of cardiovascular outcomes: an updated meta-analysis. Clin Ther. 2009;31:236–44.
- Edwards IR, Star K, Kiuru A. Statins, neuromuscular degenerative disease and an amyotrophic lateral sclerosis-like syndrome: an analysis of individual case safety reports from vigibase. Drug Saf. 2007;30:515–25.
- Golomb BA, Kwon EK, Koperski S, Evans MA. Amyotrophic lateral sclerosis-like conditions in possible association with cholesterol-lowering drugs: an analysis of patient reports to the University of California, San Diego (UCSD) Statin Effects Study. Drug Saf. 2009;32:649–61.
- Zinman L, Sadeghi R, Gawel M, Patton D, Kiss A. Are statin medications safe in patients with ALS? Amyotroph Lateral Scler. 2008;9:223–8.
- Nefussy B, Hirsch J, Cudkowicz ME, Drory VE. Gender-based effect of statins on functional decline in amyotrophic lateral sclerosis. J Neurol Sci. 2011;300:23–7.
- Golomb BA, Verden A, Messner AK, Koslik HJ, Hoffman KB. Amyotrophic lateral sclerosis associated with statin use: a disproportionality analysis of the FDA’s adverse event reporting system. Drug Saf. 2018;41:403–13.
- Colman E, Szarfman A, Wyeth J, Mosholder A, Jillapalli D, Levine J, et al. An evaluation of a data mining signal for amyotrophic lateral sclerosis and statins detected in FDA’s spontaneous adverse event reporting system. Pharmacoepidemiol Drug Saf. 2008;17:1068–76.
- Mariosa D, Kamel F, Bellocco R, Ronnevi LO, Almqvist C, Larsson H, et al. Antidiabetics, statins and the risk of amyotrophic lateral sclerosis. Eur J Neurol. 2020;27:1010–6.
- Drory VE, Bronipolsky T, Artamonov I, Nefussy B. Influence of statins treatment on survival in patients with amyotrophic lateral sclerosis. J Neurol Sci. 2008;273:81–3.
- Cui C, Sun J, McKay KA, Ingre C, Fang F. Medication use and risk of amyotrophic lateral sclerosis – a systematic review. BMC Med. 2022;20:251.
- Hu N, Ji H. Medications on hypertension, hyperlipidemia, diabetes, and risk of amyotrophic lateral sclerosis: a systematic review and meta-analysis. Neurol Sci. 2022;43:5189–99.
- Seelen M, van Doormaal PTC, Visser AE, Huisman MHB, Roozekrans MHJ, de Jong SW, et al. Prior medical conditions and the risk of amyotrophic lateral sclerosis. J Neurol. 2014;261:1949–56.
- Chang MC, Kwak SG, Park JS, Park D. Relationship between statins and the risk of amyotrophic lateral sclerosis: a PRISMA-compliant meta-analysis. Medicine. 2021;100:e26751.
- Skajaa N, Bakos I, Horváth-Puhó E, Henderson VW, Lash TL, Sørensen HT. Statin initiation and risk of amyotrophic lateral sclerosis: a Danish population-based cohort study. Epidemiology. 2021;32:756–62.
- Weisskopf MG, Levy J, Dickerson AS, Paganoni S, Leventer-Roberts M. Statin medications and amyotrophic lateral sclerosis incidence and mortality. Am J Epidemiol. 2022;191:1248–57.
- Sørensen HT, Riis AH, Lash TL, Pedersen L. Statin use and risk of amyotrophic lateral sclerosis and other motor neuron disorders. Circ Cardiovasc Qual Outcomes. 2010;3:413–7.
- Sørensen HT, Lash TL. Statins and amyotrophic lateral sclerosis – the level of evidence for an association. J Intern Med. 2009;266:520–6.
- Zheng Z, Sheng L, Shang H. Statins and amyotrophic lateral sclerosis: a systematic review and meta-analysis. Amyotroph Lateral Scler Frontotemporal Degener. 2013;14:241–5.
- Nabizadeh F, Balabandian M, Sharafi AM, Ghaderi A, Rostami MR, Naser Moghadasi A. Statins and risk of amyotrophic lateral sclerosis: a systematic review and meta-analysis. Acta Neurol Belg. 2022;122:979–86.
- Schumacher J, Peter RS, Nagel G, Rothenbacher D, Rosenbohm A, Ludolph AC, et al. Statins, diabetes mellitus and prognosis of amyotrophic lateral sclerosis: data from 501 patients of a population-based registry in southwest Germany. Eur J Neurol. 2020;27:1405–14.
- Freedman DM, Kuncl RW, Cahoon EK, Rivera DR, Pfeiffer RM. Relationship of statins and other cholesterol-lowering medications and risk of amyotrophic lateral sclerosis in the US elderly. Amyotroph Lateral Scler Frontotemporal Degener. 2018;19:538–46.
- Emdin CA, Khera AV, Kathiresan S. Mendelian randomization. JAMA. 2017;318:1925–6.
- Davies NM, Holmes MV, Davey Smith G. Reading Mendelian randomisation studies: a guide, glossary, and checklist for clinicians. BMJ. 2018;362:k601.
- Schmidt AF, Finan C, Gordillo-Marañón M, Asselbergs FW, Freitag DF, Patel RS, et al. Genetic drug target validation using Mendelian randomisation. Nat Commun. 2020;11:3255.
- Skrivankova VW, Richmond RC, Woolf BAR, Yarmolinsky J, Davies NM, Swanson SA, et al. Strengthening the reporting of observational studies in epidemiology using Mendelian randomization: the STROBE-MR Statement. JAMA. 2021;326:1614–21.
- Wu Y, Byrne EM, Zheng Z, Kemper KE, Yengo L, Mallett AJ, et al. Genome-wide association study of medication-use and associated disease in the UK Biobank. Nat Commun. 2019;10:1891.
- Richardson TG, Sanderson E, Palmer TM, Ala-Korpela M, Ference BA, Davey Smith G, et al. Evaluating the relationship between circulating lipoprotein lipids and apolipoproteins with risk of coronary heart disease: a multivariable Mendelian randomisation analysis. PLoS Med. 2020;17:e1003062.
- van Rheenen W, van der Spek RAA, Bakker MK, van Vugt JJFA, Hop PJ, Zwamborn RAJ, et al. Common and rare variant association analyses in amyotrophic lateral sclerosis identify 15 risk loci with distinct genetic architectures and neuron-specific biology. Nat Genet. 2021;53:1636–48.
- Zhu Z, Zhang F, Hu H, Bakshi A, Robinson MR, Powell JE, et al. Integration of summary data from GWAS and eQTL studies predicts complex trait gene targets. Nat Genet. 2016;48:481–7.
- Chauquet S, Zhu Z, O'Donovan MC, Walters JTR, Wray NR, Shah S. Association of antihypertensive drug target genes with psychiatric disorders: a Mendelian randomization study. JAMA Psychiatry. 2021;78:623–31.
- Higgins JPT, Thompson SG, Deeks JJ, Altman DG. Measuring inconsistency in meta-analyses. BMJ. 2003;327:557–60.
- Burgess S, Thompson SG. Interpreting findings from Mendelian randomization using the MR-Egger method. Eur J Epidemiol. 2017;32:377–89.
- Verbanck M, Chen CY, Neale B, Do R. Detection of widespread horizontal pleiotropy in causal relationships inferred from Mendelian randomization between complex traits and diseases. Nat Genet. 2018;50:693–8.
- Thompson AG, Talbot K, Turner MR. Higher blood high density lipoprotein and apolipoprotein A1 levels are associated with reduced risk of developing amyotrophic lateral sclerosis. J Neurol Neurosurg Psychiatry. 2022;93:75–81.
- Xia K, Klose V, Högel J, Huang T, Zhang L, Dorst J, et al. Lipids and amyotrophic lateral sclerosis: a two-sample Mendelian randomization study. Eur J Neurol. 2023;30:1899–906.
- Shao W, Li T, Wang Y, Shan S, Zhang H, Xue Y. Twenty-three medication-taking traits and stroke: a comprehensive Mendelian randomization study. Front Cardiovasc Med. 2023;10:1120721.
- Zeng P, Zhou X. Causal effects of blood lipids on amyotrophic lateral sclerosis: a Mendelian randomization study. Hum Mol Genet. 2019;28:688–97.
- Blanco-Colio LM, Tuñón J, Martín-Ventura JL, Egido J. Anti-inflammatory and immunomodulatory effects of statins. Kidney Int. 2003;63:12–23.
- Ii M, Losordo DW. Statins and the endothelium. Vascul Pharmacol. 2007;46:1–9.
- Stoll LL, McCormick ML, Denning GM, Weintraub NL. Antioxidant effects of statins. Drugs Today. 1998;40:975–90.
- Lee SH, Choi NY, Yu HJ, Park J, Choi H, Lee KY, et al. Atorvastatin protects NSC-34 motor neurons against oxidative stress by activating PI3K, ERK and free radical scavenging. Mol Neurobiol. 2016;53:695–705.
- Li H, Kuwajima T, Oakley D, Nikulina E, Hou J, Yang WS, et al. Protein prenylation constitutes an endogenous brake on axonal growth. Cell Rep. 2016;16:545–58.
- Kreple CJ, Searles Nielsen S, Schoch KM, Shen T, Shabsovich M, Song Y, et al. Protective effects of lovastatin in a population-based ALS study and mouse model. Ann Neurol. 2023;93:881–92.
- Ference BA, Kastelein JJP, Ginsberg HN, Chapman MJ, Nicholls SJ, Ray KK, et al. Association of genetic variants related to CETP inhibitors and statins with lipoprotein levels and cardiovascular risk. JAMA. 2017;318:947–56.
- Wong JK, Roselle AK, Shue TM, Shimshak SJE, Beaty JM, Celestin NM, et al. Apolipoprotein B-100-mediated motor neuron degeneration in sporadic amyotrophic lateral sclerosis. Brain Commun. 2022;4:fcac207.