Abstract
Objective
To assess the temporal relationship between premorbid lipid levels and long-term amyotrophic lateral sclerosis (ALS) risk.
Methods
From Norwegian cardiovascular health surveys (1974–2003), we collected information on total cholesterol (TC), triglycerides (TG), high-density lipoprotein cholesterol (HDL-C), low-density lipoprotein cholesterol (LDL-C), glucose, and other cardiovascular risk factors. ALS incidence and mortality were identified through validated Norwegian health registries. The relation between premorbid lipid levels and ALS risk was assessed by Cox regression models.
Results
Out of 640,066 study participants (51.5% females), 974 individuals (43.5% females) developed ALS. Mean follow-up time was 23.7 (SD 7.1) years among ALS cases. One mmol/l increase in LDL-C was associated with 6% increase in risk for ALS (hazard ratio 1.06 [95% CI: 1.01–1.09]). Higher levels of TC and TG were also associated with increased ALS risk, but only within the last 6–7 years prior to ALS diagnosis or death. No association between HDL-C and ALS risk was found. Adjusting for body mass index, birth cohort, smoking, and physical activity did not alter the results.
Conclusions
Higher levels of LDL-C are associated with increased ALS risk over 40 years later, compatible with a causal relationship. The temporal relationship between TG, TC, and ALS risk suggests that increased levels of these lipid biomarkers represent consequences of ALS.
Introduction
Hypermetabolism is a commonly observed phenomenon in amyotrophic lateral sclerosis (ALS), linked to increased functional decline and reduced survival (Citation1,Citation2). Related to aberrant energy homeostasis, it is becoming increasingly accepted that dyslipidemia plays a role in the pathological process.
In ALS, elevated levels of either total cholesterol (TC), triglycerides (TG), or low-density lipoprotein cholesterol (LDL-C) have been associated with improved survival in some (Citation3–8), but not all (Citation9–12) observational studies. Some studies also found that increased levels of high-density lipoprotein cholesterol (HDL-C) were associated with poor survival (Citation8,Citation12).
Similarly, a possible relation between blood lipid levels and ALS risk has been suggested but has proven difficult to establish firmly. Higher levels of LDL-C have been linked to an increased ALS risk (Citation13), and higher levels of HDL-C have been linked to both reduced and increased ALS risk (Citation14,Citation15). Mendelian randomization studies have implied that TC (Citation16–18) and LDL-C (Citation17–21) are causally related to ALS risk.
The pathological process possibly begins several years before symptom onset (Citation22). Prospective studies should therefore encompass a long observation period to avoid the risk of inverse causality, where associations to possible risk factors merely reflect consequences of a disease process. Furthermore, biomarkers of lipid metabolism are influenced by lifestyle factors such as physical activity (Citation23), body mass index (BMI) (Citation24), smoking (Citation23), and blood glucose levels (Citation25), elements which additionally have been linked to ALS risk (Citation26–29). Therefore, detailed information regarding lifestyle and environmental factors is needed.
We used data from large Norwegian cardiovascular health surveys and compulsory health registries to examine the temporal relationship between premorbid lipid levels and long-term ALS risk.
Method and materials
Study population
The study population comprises Norwegian citizens participating in regional health surveys (1974–2003) focused on cardiovascular risk factors. All health surveys consisted of a questionnaire and physical examination. The questionnaire requested information on lifestyle and health-related topics, such as smoking and alcohol habits, and level of physical activity. Trained personnel conducted the physical examination which included objective measurements of height, weight, heart rate, and blood pressure. A non-fasting blood sample was analyzed for circulating levels of TG, TC, and glucose. HDL-C was gradually included from 1980 onwards (Citation30). Our joint cohort constituted the following;
The Counties Study (1974–1988) invited all men and women aged 35–49 years living in three Norwegian counties (Finnmark, Oppland, and Sogn og Fjordane). Attendance rate was approximately 90%. A total of 94,022 participants participated in the survey. It was carried out in total three times with intervals of 3–5 years (Citation31). HDL-C was not included in blood samples in the first screening but was included in subsequent screenings. Altogether, 86% of the participants had measurements of HDL-C.
The age 40 program (1985–1999) invited people aged 40–45 years in all Norwegian counties except the capital of Oslo. In total, 417,098 individuals participated, with an attendance rate of 52–74% (Citation32). In 29% of the participants, the serum analyses included HDL-C (Citation33).
Cohort of Norway (CONOR) (1994–2003) is a combined cohort of standardized health data and blood samples collected through 10 regional health surveys. In total 173,236 individuals were included, and the overall participation rate was 58% (Citation34). In CONOR, near all (99.8%) had HDL-C measurements.
From these health surveys, we collected information on circulating levels of lipids and glucose, sex, year and month of birth, year and month of screening, height and weight, self-reported information on diseases (diabetes, myocardial infarction, stroke), smoking status, and levels of physical activity and education.
We excluded 1,520 individuals due to missing information on time of study inclusion. Hence, the final study cohort consisted of 640,066 individuals (). For study participants registered in multiple screenings or surveys (n = 42,709), the survey which included information on HDL-C was selected. If no HDL-C information existed, the first survey was selected.
Figure 1. Selection of participants. CONOR, Cohort of Norway; HDL-C, high-density lipoprotein cholesterol.
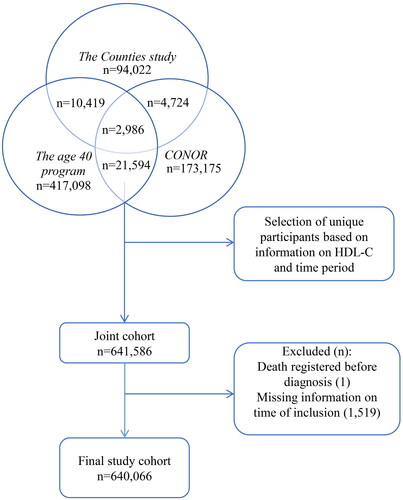
Emigration and vital status for each participant were retrieved from the National Population Registry.
Biomarkers
TG and TC were measured in blood samples in near all of the cohort (98%). HDL-C was measured in 57% and blood glucose in 52% of the cohort. All blood samples were non-fasting, and all biomarkers were registered in mmol/l. All analyses were performed at the central laboratory, Ullevål Hospital, Norway, except for two regional health surveys in CONOR, where the blood samples were analyzed at Levanger Hospital and University hospital of North-Norway, Tromsø. Calibration procedures were carried out between these laboratories (Citation34).
We calculated LDL-C using the NIH 2 equation, which has been found appropriate also for high TG values (Citation35,Citation36). To calculate LDL-C using the NIH 2 equation, the lipid biomarkers were first converted to mg/dl using recommended converting factors (TC*38.67, TG*88.57, HDL-C*38.67), and after calculation, LDL-C was subsequently converted from mg/dl to mmol/l (LDL-C*0.02586) (Citation37).
Extreme values of lipids (>50 mmol/l and <0.1 mmol/l) and glucose (<1.5 mmol/l) were set to missing for 51 participants.
Ascertainment of ALS cases
We ascertained ALS cases through the Norwegian Cause of Death Registry (“the Death Registry”) and the Norwegian Patient Registry (“the Patient Registry”). The Death Registry collects and processes all death certificates in Norway, and has digitalized data from 1951 onwards. The direct, contributing, and underlying cause of death are registered. Causes of death are coded in accordance with different revisions of the International Classification of Diseases (ICD). ALS was defined as ICD codes matching motor neuron disease registered anywhere on the death certificate. We collected all ALS deaths from the start of the first health survey (1974) to August 2021 using the following codes: ICD 8 (1969–1985): 348.0, 348.1, and 348.2; ICD 9 (1986–1995): 335.2; ICD 10 (1996 onwards): G12.2. In a previous study, the Death Registry was found reliable and has a high sensitivity to ascertain ALS cases (Citation38).
The Patient Registry includes all in- and outpatient admissions in Norwegian hospitals and private practice specialists with public reimbursement. Individual data are available from March 1, 2007. ICD 10 codes and dates are logged for each admission or consultation. ALS cases were defined as individuals who had two or more G12.2 (ICD-10) entries in the registry. We collected data from January 2007 until August 2021, with the date of incidence set to the first entry. This method has previously been validated and found reliable to identify ALS cases in Norway (Citation39). If ALS cases were identified in both the Death Registry and the Patient Registry, the Patient Registry (ALS incidence) was chosen. From a total of 974 ALS cases retrieved, 443 were found in both registries and 294 only in the Death Registry.
All Norwegian citizens have a unique personal identification number which makes linkage between registries possible.
Ethics, approval, and consent
The study was approved by the regional ethics committee (REC South East reference number 2016/1731). All participants signed informed consent at the time of screening.
Statistical analysis
Baseline characteristics for the entire cohort as well as stratified by no ALS and ALS are presented as means and standard deviations (SDs) or frequencies and percentages, as appropriate. Groups were compared by independent-samples t-test for continuous and χ2-test for categorical variables. To assess associations between exposure variables and hazard for ALS, Cox regression model were fitted. The models were adjusted for sex, age, and health survey (Model 1) or for sex, age, birth cohort, health survey, other lipids, BMI, physical activity, and smoking status (Model 2). Due to high correlation between TC and LDL-C, these biomarkers were assessed separately in Model 2. Cox model assumptions were assessed by standard statistical tests; possible nonlinear associations between continuous covariates and outcome were assessed through second-order terms, proportional hazards assumption was tested by global test and by inspecting Schoenfeld residuals graphically. Time-dependent variables were included into the model together with their interaction with natural logarithm of time, and illustrated graphically for easier interpretation. Because some variables were a part of interaction terms, results were presented as both regression coefficients (RCs) and standard errors (SEs) as well as hazard ratios (HRs) and 95% confidence intervals (CIs), where appropriate. Due to a substantial number of missing values on both exposure- and adjustment variables, normalized inverse probability weights were generated and included into regression models. Normalization of weights was performed to avoid underestimated SEs of estimates due to apparently higher sample size in pseudo-population generated by weighting. Due to a high degree of missing values, glucose as exposure variable was assessed in a separate supplementary analysis. A complete case analysis was performed as a sensitivity analysis. All tests were two-sided and results with p-values below .05 were considered statistically significant. Analyses were performed in STATA v17.
Results
Our final study cohort comprised 640,066 individuals (51.5% females). Mean age at blood sampling was 42.6 (SD 9.1) years, and mean follow-up time was 27 (SD 8) years. We identified 974 ALS cases (43.5% females), with mean age at blood sampling 44.2 (SD 8.8) years and mean follow-up time 23.7 (SD 7.1) years. For ALS cases retrieved from the Patient Registry, the mean age at diagnosis was 66.5 (SD 8.4) years, and for ALS cases retrieved from the Death Registry, the mean age at death was 67.1 (SD 9.7) years. The number of participants who reported stroke, heart disease, or diabetes at screening was generally low and similar in cases and non-cases. Baseline characteristics of the final study cohort are presented in .
Table 1. Baseline characteristics of the cohort.
LDL-C was associated with an increased and sustained ALS risk ( and ). Adjusting for age, sex, and health survey (Model 1), one mmol/l increase in LDL-C was associated with 6% higher ALS risk (RC of 0.06 [SE 0.03], corresponding to an HR of 1.06 [95% CI: 1.01–1.09]). In the model additionally adjusting for other lipid biomarkers, BMI, birth cohort, smoking, and physical activity (Model 2), estimates were similar (). Increasing levels of TC and TG were also associated with higher ALS risk, but in contrast to LDL-C these associations waned with increasing time since blood sampling ( and ). Thus, in Model 2, one mmol/l increase in TC was associated with higher risk for ALS with HR 1.18 (95% CI: 1.08–1.30) 1 year after blood sampling and HR 1.13 (95% CI: 1.04–1.23) 3 years after blood sampling, and there was no statistically significant association beyond 6.5 years after blood sampling (). Likewise, one mmol/l increase in TG was associated with higher risk for ALS with HR 1.38 (95% CI: 1.24–1.53) 1 year after blood sampling and HR 1.23 (95% CI: 1.11–1.35) 3 years after blood sampling, but not beyond 6.5 years after blood sampling (). No association at any time point was detected between HDL-C and ALS risk.
Figure 2. Risk (HR) of amyotrophic lateral sclerosis (ALS), according to increasing levels of lipid biomarkers and time since blood sampling, adjusted for sex, age, birth cohort, health survey, other lipids, BMI, physical activity, and smoking. LDL-C, low-density lipoprotein cholesterol; HDL-C, high-density lipoprotein cholesterol.
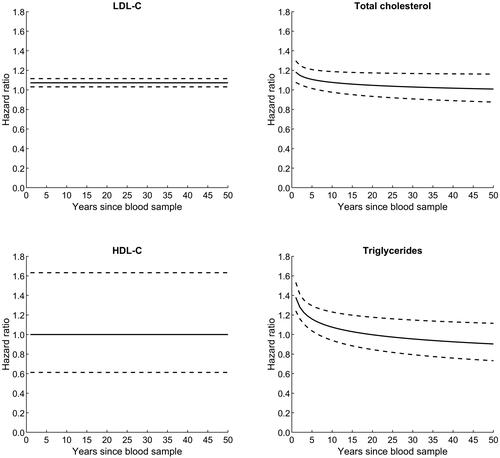
Table 2. Risk of amyotrophic lateral sclerosis (ALS) for one-unit (mmol/l) increase in premorbid levels of circulating lipids.
Adding glucose as a covariate in both models did not change the results substantially. Glucose was not in itself associated with ALS risk (Supplementary Table 1).
A complete case analysis of the final study cohort yielded comparable results except for LDL-C (Supplementary Table 2). Thus, high TC and TG was associated with increased ALS risk in a time-dependent manner also here (for TG in Model 1 only). No association was seen between LDL-C and ALS risk in this complete case analysis. Compared to our main cohort, a larger proportion of study participants in this sub sample had higher education, but still equal between cases (36.5%) and non-cases (37.2%).
Discussion
In this large population-based cohort study, we assessed the association between circulating levels of lipid biomarkers in adulthood to later ALS risk. Our main finding was that higher levels of LDL-C were associated with increased ALS risk decades after blood sampling. This association was independent of sex and age at blood sampling, and other potential confounding factors. The sustained temporal relationship between LDL-C and ALS risk militates against reverse causality. HDL-C was not associated with ALS risk. The association between TC, TG, and ALS risk was less clear. While higher levels of both TC and TG were associated with increased ALS risk in the period most adjacent to blood sampling, the association waned over time, suggesting that elevated levels of TC and TG represent early consequences of the disease processes rather than risk factors.
Our finding of a link between LDL-C and long-term ALS risk adds to a growing body of evidence suggesting that LDL-C may be causally linked to ALS. Premorbid increased levels of LDL-C have in a large Swedish cohort study, along with increased levels of apolipoprotein B, been associated with an increased ALS risk (Citation13). Further, several Mendelian randomization studies have highlighted genetically predicted increased LDL-C as a potential causal risk factor for ALS (Citation17–21). Among these, Xia et al. (Citation18) found no effect of lipid-modifying lifestyles on ALS risk, and demonstrated a potential mediating role for LDL-C in the pathway from polyunsaturated fatty acids (PUFAs) to ALS. In their nested case-control study, could Bjørnevik et al. (Citation15) however not replicate the findings of LDL-C on ALS risk. As addressed in the article, associations could vary according to time from blood sampling to ALS onset. In line with this, a recent study found significant trajectory changes in mean concentrations of LDL-C and TC up to 7 years prior to symptom onset in ALS, compared to matched controls (Citation40). Taken together, this highlights the need for long follow-up time when studying risk factors for neurodegenerative diseases with possible long prodromal phases.
In a recent case-control study from the ALS registry in Swabia (Citation8) higher concentrations of TC, but not HDL-C, LDL-C, or TG, were linked to increased ALS risk. Blood samples were however taken at or after diagnosis; hence their study was more suitable for studying the predictive value of these biomarkers on ALS survival. Higher TG levels have previously not been linked to ALS risk, but associated with an increased survival at time of diagnosis (Citation4,Citation6–8). Some (Citation16–18), but not all (Citation19,Citation21) Mendelian randomization studies have proposed high levels of TC as a causal risk factor for ALS. Of note, if a risk factor is time-dependent or cumulative, effect estimates or causal interpretation in Mendelian randomization studies can be misleading (Citation41) and possibly explain diverging results on TC and ALS risk. While we cannot exclude that both high TC and TG can act as risk factors for ALS, our analyses indicated that their association with ALS risk was restricted to the 6–7 years closest to ALS disease or death. This temporal relationship harmonizes with a prodromal phase as suggested from mild motor impairment in unaffected gene mutation carriers (Citation22), and elevated prediagnostic neurofilament light chain levels up to 5 years before ALS diagnosis (Citation42). The association between TC, TG, and ALS is therefore most compatible with secondary phenomena.
In our study, increasing levels of HDL-C were not associated with ALS risk. In two previous cohort studies, higher HDL-C levels were associated with either a lower (Citation14), or an increased ALS risk (Citation15). Again, insufficient observation periods may explain diverging results, together with differences in case ascertainment, power, and study populations.
Increasing levels of blood glucose was not independently associated with ALS risk in our supplementary analysis. This agrees with a large Swedish cohort study (Citation13). Still, this analysis was attenuated by a large number of missing values as well as the lacking possibility to discriminate between participants with type 1 and type 2 diabetes, which could be relevant in ALS etiology (Citation43). Therefore, firm conclusions on any association between blood glucose levels and ALS risk are difficult.
Dyslipidemia has been linked to ALS through several pathways. First, increasing LDL-C and dyslipidemia are associated with enhanced oxidative stress (Citation44), which is a potential crucial part of disease mechanism in ALS (Citation45). Second, PUFAs can modulate levels of LDL-C (Citation46). Depending on which type, PUFAs can display both pro- and anti-inflammatory effects and have been linked to both increased and reduced ALS risk (Citation47,Citation48). Third, a recent study on knockout mice found that TDP-43, a protein observed mislocalized and considered a pathological hallmark in ALS, can mediate SREBF2, which regulates cholesterol metabolism (Citation49).
The strengths of our study are the population-based design with prospectively collected data, a large cohort, and a mean follow-up time lasting almost three decades. The health surveys had a similar design, and except for a part of the analyses in CONOR, all analyses were performed in the same laboratory. Furthermore, we were able to adjust for most relevant potential confounding factors, and all anthropometric data were measured objectively and standardized.
Our study has limitations. As for all observational studies, we cannot exclude possible bias from unmeasured confounding factors. We had limited information on use of statins in our data and therefore could not adjust for this. Most blood samples were taken prior to the time period when large-scale statin use made its entrance in the Norwegian population (around 1995). Moreover, statins are probably not associated with ALS risk (Citation50). We did not have information on HDL-C, LDL-C, and glucose in a substantial proportion of participants. Participants in voluntary health surveys could differ from the general population in terms of lifestyle, education, and socioeconomic status, affecting study generalizability. However, in a substantial part of the cohort (CONOR), the population representativeness regarding smoking, education, and alcohol habits have been reported adequate (Citation51). Further, the level of education in our main cohort compared to the general population (Citation52) agreed. The sub sample constituting our sensitivity analysis had a higher educational level than our main study cohort. In addition to reduced power, this could possibly explain the lack of association of LDL-C on ALS risk in this sub sample, and further argues for addressing missing data through the use of probability weights in our primary analysis. Because the Patient Registry was first initiated in 2007, we used both incidence (the Patient Registry) and mortality (the Death Registry) for case ascertainment. As cases retrieved from the Death Registry (approximately one third) also will incorporate survival time after diagnosis, this combination challenges the calculation of exact time from blood sampling to ALS and consequentially the temporal effects of the different lipid biomarkers on ALS risk. Still, compared to the long follow-up in the current study, the mean survival time for ALS is short. Also, ALS mortality data have a good accuracy for incidence rates (Citation38,Citation53). Ultimately, age at diagnosis or death in the current study agreed with what has been reported for the Norwegian ALS population (Citation38,Citation54), suggesting adequate representativeness.
In conclusion, our results add further evidence linking dyslipidemia to ALS risk. Thus, higher LDL-C is associated with increased ALS risk several decades later. The association between high TC and TG on ALS risk appears to be more short-term, suggesting a possible shift in how lipids relate to ALS pathogenesis at different stages. Further studies are needed to explore whether dyslipidemia is a modifiable risk factor in ALS.
Supplemental Material
Download Zip (72 KB)Acknowledgments
We are grateful for data supplied from the Norwegian Patient Registry, the Norwegian Cause of Death Registry, and the Norwegian Institute of Public Health, and for financial support from the patient organization ALS Norway.
Data availability
The authors do not have permission to share data.
Disclosure statement
The authors report there are no competing interests to declare.
Additional information
Funding
References
- Jésus P, Fayemendy P, Nicol M, Lautrette G, Sourisseau H, Preux PM, et al. Hypermetabolism is a deleterious prognostic factor in patients with amyotrophic lateral sclerosis. Eur J Neurol. 2018;25:97–104.
- Steyn FJ, Ioannides ZA, van Eijk RPA, Heggie S, Thorpe KA, Ceslis A, et al. Hypermetabolism in ALS is associated with greater functional decline and shorter survival. J Neurol Neurosurg Psychiatry. 2018;89:1016–23.
- Ahmed RM, Highton-Williamson E, Caga J, Thornton N, Ramsey E, Zoing M, et al. Lipid metabolism and survival across the frontotemporal dementia-amyotrophic lateral sclerosis spectrum: relationships to eating behavior and cognition. J Alzheimers Dis. 2018;61:773–83.
- Huang R, Guo X, Chen X, Zheng Z, Wei Q, Cao B, et al. The serum lipid profiles of amyotrophic lateral sclerosis patients: a study from south-west China and a meta-analysis. Amyotroph Lateral Scler Frontotemporal Degener. 2015;16:359–65.
- Ingre C, Chen L, Zhan Y, Termorshuizen J, Yin L, Fang F. Lipids, apolipoproteins, and prognosis of amyotrophic lateral sclerosis. Neurology 2020;94:e1835–e44.
- Mandrioli J, Rosi E, Fini N, Fasano A, Raggi S, Fantuzzi AL, et al. Changes in routine laboratory tests and survival in amyotrophic lateral sclerosis. Neurol Sci. 2017;38:2177–82.
- Dorst J, Kühnlein P, Hendrich C, Kassubek J, Sperfeld AD, Ludolph AC. Patients with elevated triglyceride and cholesterol serum levels have a prolonged survival in amyotrophic lateral sclerosis. J Neurol. 2011;258:613–7.
- Michels S, Kurz D, Rosenbohm A, Peter RS, Just S, Bäzner H, et al. Association of blood lipids with onset and prognosis of amyotrophic lateral sclerosis: results from the ALS Swabia registry. J Neurol. 2023;270:3082–90.
- Barone M, Viggiani MT, Introna A, D'Errico E, Scarafino A, Iannone A, et al. Nutritional prognostic factors for survival in amyotrophic lateral sclerosis patients undergone percutaneous endoscopic gastrostomy placement. Amyotroph Lateral Scler Frontotemporal Degener. 2019;20:490–6.
- Paganoni S, Deng J, Jaffa M, Cudkowicz ME, Wills AM. Body mass index, not dyslipidemia, is an independent predictor of survival in amyotrophic lateral sclerosis. Muscle Nerve. 2011;45: 612–24.
- Rafiq MK, Lee E, Bradburn M, McDermott CJ, Shaw PJ. Effect of lipid profile on prognosis in the patients with amyotrophic lateral sclerosis: insights from the olesoxime clinical trial. Amyotroph Lateral Scler Frontotemporal Degener. 2015;16:478–84.
- Janse van Mantgem MR, van Rheenen W, Hackeng AV, van Es MA, Veldink JH, van den Berg LH, et al. Association between serum lipids and survival in patients with amyotrophic lateral sclerosis: a meta-analysis and population-based study. Neurology 2023;100:e1062–e71.
- Mariosa D, Hammar N, Malmström H, Ingre C, Jungner I, Ye W, et al. Blood biomarkers of carbohydrate, lipid, and apolipoprotein metabolisms and risk of amyotrophic lateral sclerosis: a more than 20-year follow-up of the Swedish AMORIS cohort. Ann Neurol. 2017;81:718–28.
- Thompson AG, Talbot K, Turner MR. Higher blood high density lipoprotein and apolipoprotein A1 levels are associated with reduced risk of developing amyotrophic lateral sclerosis. J Neurol Neurosurg Psychiatry. 2022;93:75–81.
- Bjornevik K, O'Reilly ÉJ, Cortese M, Furtado JD, Kolonel LN, Le Marchand L, et al. Pre-diagnostic plasma lipid levels and the risk of amyotrophic lateral sclerosis. Amyotroph Lateral Scler Frontotemporal Degener. 2021;22:133–43.
- van Rheenen W, van der Spek RAA, Bakker MK, van Vugt J, Hop PJ, Zwamborn RAJ, et al. Common and rare variant association analyses in amyotrophic lateral sclerosis identify 15 risk loci with distinct genetic architectures and neuron-specific biology. Nat Genet. 2021;53:1636–48.
- Chen X, Yazdani S, Piehl F, Magnusson PKE, Fang F. Polygenic link between blood lipids and amyotrophic lateral sclerosis. Neurobiol Aging. 2018;67:202.e1–e6.
- Xia K, Klose V, Högel J, Huang T, Zhang L, Dorst J, et al. Lipids and amyotrophic lateral sclerosis: a two-sample Mendelian randomization study. Eur J Neurol. 2023;30:1899–906.
- Zeng P, Zhou X. Causal effects of blood lipids on amyotrophic lateral sclerosis: a Mendelian randomization study. Hum Mol Genet. 2019;28:688–97.
- Bandres-Ciga S, Noyce AJ, Hemani G, Nicolas A, Calvo A, Mora G, et al. Shared polygenic risk and causal inferences in amyotrophic lateral sclerosis. Ann Neurol. 2019;85:470–81.
- Esteban-García N, Fernández-Beltrán LC, Godoy-Corchuelo JM, Ayala JL, Matias-Guiu JA, Corrochano S. Body complexion and circulating lipids in the risk of TDP-43 related disorders. Front Aging Neurosci. 2022;14:838141.
- Benatar M, Granit V, Andersen PM, Grignon AL, McHutchison C, Cosentino S, et al. Mild motor impairment as prodromal state in amyotrophic lateral sclerosis: a new diagnostic entity. Brain. 2022;145:3500–8.
- Muga MA, Owili PO, Hsu CY, Chao JC. Association of lifestyle factors with blood lipids and inflammation in adults aged 40 years and above: a population-based cross-sectional study in Taiwan. BMC Public Health. 2019;19:1346.
- Brown CD, Higgins M, Donato KA, Rohde FC, Garrison R, Obarzanek E, et al. Body mass index and the prevalence of hypertension and dyslipidemia. Obes Res. 2000;8:605–19.
- Parhofer KG. Interaction between glucose and lipid metabolism: more than diabetic dyslipidemia. Diabetes Metab J. 2015;39:353–62.
- Zhan Y, Fang F. Smoking and amyotrophic lateral sclerosis: a mendelian randomization study. Ann Neurol. 2019;85:482–4.
- Nakken O, Meyer HE, Stigum H, Holmøy T. High BMI is associated with low ALS risk: a population-based study. Neurology 2019;93:e424–e32.
- Fang F, Hållmarker U, James S, Ingre C, Michaëlsson K, Ahlbom A, et al. Amyotrophic lateral sclerosis among cross-country skiers in Sweden. Eur J Epidemiol. 2016;31:247–53.
- Zhang L, Tang L, Huang T, Fan D. Association between type 2 diabetes and amyotrophic lateral sclerosis. Sci Rep. 2022;12:2544.
- Foss O, Urdal P. Cholesterol for more than 25 years: could the results be compared throughout all this time? Nor Epidemiol 2003;13:85–8.
- Selmer R, Tverdal A. Serum total cholesterol and mortality from ischaemic heart disease, all cardiovascular causes and all causes: 25-year follow-up of the first cardiovascular screening in Finnmark, Oppland and Sogn og Fjordane. Nor J Epidemiol 2003;13:115–25.
- Norwegian Institute of Public Health. 40 årsundersøkelsene (1985–1999) [Online] [updated 2019 Jun 16; cited 2023 May 19]. 2013. Available at: https://www.fhi.no/div/helseundersokelser/landsomfattende-helseundersokelser-lhu/helseundersokelser/40-aringsundersokelsene/.
- Tverdal A, Hjellvik V, Selmer R. Heart rate and mortality from cardiovascular causes: a 12 year follow-up study of 379,843 men and women aged 40–45 years. Eur Heart J. 2008;29:2772–81.
- Naess O, Søgaard AJ, Arnesen E, Beckstrøm AC, Bjertness E, Engeland A, et al. Cohort profile: cohort of Norway (CONOR). Int J Epidemiol. 2008;37:481–5.
- Higgins V, Leiter LA, Delaney SR, Beriault DR. Validating the NIH LDL-C equation in a specialized lipid cohort: Does it add up? Clin Biochem. 2022;99:60–8.
- Sampson M, Ling C, Sun Q, Harb R, Ashmaig M, Warnick R, et al. A new equation for calculation of low-density lipoprotein cholesterol in patients with normolipidemia and/or hypertriglyceridemia. JAMA Cardiol. 2020;5:540–8.
- SI Units Conversion Table. Ann Pharmacother. 1996;30:96–103.
- Nakken O, Lindstrøm JC, Tysnes O-B, Holmøy T. Mortality trends of amyotrophic lateral sclerosis in Norway 1951–2014: an age-period-cohort study. J Neurol. 2016;263:2378–85.
- Nakken O, Lindstrøm JC, Tysnes O-B, Holmøy T. Assessing amyotrophic lateral sclerosis prevalence in Norway from 2009 to 2015 from compulsory nationwide health registers. Amyotroph Lateral Scler Frontotemporal Degener. 2018;19:303–10.
- Thompson AG, Marsden R, Talbot K, Turner MR. Primary care blood tests show lipid profile changes in pre-symptomatic amyotrophic lateral sclerosis. Brain Commun. 2023;5:fcad211.
- Holmes MV, Ala-Korpela M, Smith GD. Mendelian randomization in cardiometabolic disease: challenges in evaluating causality. Nat Rev Cardiol. 2017;14:577–90.
- Bjornevik K, O'Reilly EJ, Molsberry S, Kolonel LN, Le Marchand L, Paganoni S, et al. Prediagnostic neurofilament light chain levels in amyotrophic lateral sclerosis. Neurology 2021;97:e1466-74–e74.
- Kioumourtzoglou MA, Rotem RS, Seals RM, Gredal O, Hansen J, Weisskopf MG. Diabetes mellitus, obesity, and diagnosis of amyotrophic lateral sclerosis: a population-based study. JAMA Neurol. 2015;72:905–11.
- Mitra S, Deshmukh A, Sachdeva R, Lu J, Mehta JL. Oxidized low-density lipoprotein and atherosclerosis implications in antioxidant therapy. Am J Med Sci. 2011;342:135–42.
- Xiong L, McCoy M, Komuro H, West XZ, Yakubenko V, Gao D, et al. Inflammation-dependent oxidative stress metabolites as a hallmark of amyotrophic lateral sclerosis. Free Radic Biol Med. 2022;178:125–33.
- Fernandez ML, West KL. Mechanisms by which dietary fatty acids modulate plasma lipids. J Nutr. 2005;135:2075–8.
- Xia K, Wang Y, Zhang L, Tang L, Zhang G, Huang T, et al. Dietary-derived essential nutrients and amyotrophic lateral sclerosis: a two-sample Mendelian randomization study. Nutrients 2022;14:920.
- O'Reilly ÉJ, Bjornevik K, Furtado JD, Kolonel LN, Le Marchand L, McCullough ML, et al. Prediagnostic plasma polyunsaturated fatty acids and the risk of amyotrophic lateral sclerosis. Neurology 2020;94:e811–e9.
- Ho WY, Chang JC, Lim K, Cazenave-Gassiot A, Nguyen AT, Foo JC, et al. TDP-43 mediates SREBF2-regulated gene expression required for oligodendrocyte myelination. J Cell Biol 2021;220:e201910213
- Mariosa D, Kamel F, Bellocco R, Ronnevi LO, Almqvist C, Larsson H, et al. Antidiabetics, statins and the risk of amyotrophic lateral sclerosis. Eur J Neurol. 2020;27:1010–6.
- Norwegian Institute of Public Health. CONOR: Research potential, design and representativeness [Online] [cited 2023 Nov 2]. 2013. Available at: https://www.fhi.no/globalassets/dokumenterfiler/studier/conor/conor.pdf.
- Statistics Norway. Educational attainment of the population [Online] [cited 2023 Nov 6]. Available at: https://www.ssb.no/en/statbank/table/08921/.
- Marin B, Couratier P, Preux PM, Logroscino G. Can mortality data be used to estimate amyotrophic lateral sclerosis incidence? Neuroepidemiology 2011;36:29–38.
- Benjaminsen E, Alstadhaug KB, Gulsvik M, Baloch FK, Odeh F. Amyotrophic lateral sclerosis in Nordland county, Norway, 2000–2015: prevalence, incidence, and clinical features. Amyotroph Lateral Scler Frontotemporal Degener. 2018;19:522–7.