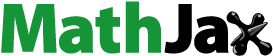
Abstract
The development of haemoglobin-based oxygen carrier (HBOC) is an excellent supplement to pre-hospital emergency blood transfusions. In this study, a new type of HBOC was prepared by using human cord haemoglobin (HCHb) and glutaraldehyde (GDA) and Bis(3,5-dibromosalicyl) fumarate (DBBF) to modify (DBBF-GDA-HCHb), the changes of physicochemical indexes during its preparation were evaluated, while a traditional type of GDA-HCHb was prepared, and the oxygen-carrying capacity of two type of HBOC was evaluated by a rat model of 135.0% exchange transfusion (ET). Eighteen SD male rats were selected, and were randomly divided into control group (5.0% albumin), DBBF-GDA-HCHb group and GDA-HCHb group. The 12 h survival rate of the C group was 16.67%, and the two HBOC groups were both 83.33%. Compared with GDA-HCHb, DBBF-GDA-HCHb can reduce lactic acid content by supplying oxygen to hypoxic tissues in a more timely manner, and can also can improve the reduction of MAP due to ischaemia.
Background
A transfusion-related event occurs every 2 s in the United States (US), approximately 300 million units of red blood cells (RBCs) are used clinically each year, and this trend is also increasing significantly [Citation1]. Study has pointed out that only about 3% of age-eligible people donate blood, resulting in a blood donation rate and blood amount that fall short of current needs [Citation2]. Nearly 35% of prehospital deaths in trauma is haemorrhage, and haemorrhagic shock (HS) accounts for about 20% of military casualties are on arrival to field hospitals and an additional 5% require urgent transfusion [Citation3,Citation4]. A review [Citation5] reported that nearly 90% of potentially survivable battlefield deaths was haemorrhage, and if better haemorrhage control include field-ready blood, blood components, or blood substitutes the lives should could be saved. However, there are certain risks and limitations associated with transfusion of whole blood (WB) or RBCs. So the high and fast-growing demand for the substitute of WB or RBCs at the sites of need is necessary.
Haemoglobin (Hb)-based oxygen (O2) carriers (HBOC) can replace RBCs for clinical transfusion in terms of oxygen-carrying capacity. Natanson et al. [Citation6] and Silverman and Weiskopf [Citation7] reported 3711 and 3489 cases in clinical research reports in the US. The five HBOC products were used to rescue patients with traumatic haemorrhage, and the survival rates were 91.6% and 91.7%, respectively. And a large number of experimental studies [Citation8–11] have shown that HBOC had good potential for the treatment of acute myocardial infarction and ‘stroke’, and it also had a pioneering therapeutic effect in ‘tumor’ chemotherapy/radiotherapy. Therefore, HBOC is of great clinical significance, and the potential amount of HBOC is also enormous.
As a cross-linking agent, GDA has two longer carbon chain aldehyde groups with no specific reaction site of Hb. So, the size of poly-Hb molecules will be uneven with GDA, resulting in two different consequences. One is that the average molecular weight will be too low with high content dimer, the other one is that it is too large. If the average molecular weight was too low, the utilization of Hb would be reduced, and that too high content dimer would reduce the stability of Hb. Haem release, because of unstable Hb, also enhances the toxicity of cell-free Hb, causing lipid peroxidation and mitochondrial damage, and increases production of reactive oxygen species (ROS) [Citation12]. Haem selectively triggers pro-inflammatory receptors and activates the proteasome. Elevated plasma dimer levels lead to dimer filtration in glomerular and subsequent tubular damage. ROS can induce cytotoxicity by protein oxidation and nucleic acid and reduce the oxygen-carrying capacity of Hb through the formation of Methemoglobin (MetHb). While the average molecular weight is too large, it is not good for the recovery of ischaemic capillaries, resulting in reducing the colloid osmotic pressure and increasing its viscosity, which will seriously affect the haemodynamics of polymerized Hb and the oxygen supply capacity of the tissue. Therefore, the US FDA requires that the average molecular weight should be reduced in the approval for the Phase III clinical trial of Biopure’s HBOC-201 [Citation13]. Therefore, it is crucial to seek to one way to solve the uniform molecular weight distribution (MWD) to reduce the dimer content as much as possible on the basis of lower average molecular weight.
In erythrocytes, tetramers and dimers transform each other, maintaining a relative balance, and tetramers are more than dimers. But after leaving erythrocytes, tetramers are not protected by 2,3-DPG, and are easier to dissociate into dimers. In order to maintain the structural and functional integrity of tetramers, it is a wise choice to find a way to maintain it after Hb comes out of erythrocytes. Bis(3,5-dibromosalicyl) fumarate (DBBF) is an intramolecular crosslinker of Hb.
DBBF has a structure with bifunctional group, and two different reactions can occur under different oxygenation states of Hb. Under oxygenation conditions, β182 Lys and β282 Lys can be intramolecularly cross-linked with the amino group of Hb to form a stable amide structure specifically. While under deoxygenation conditions, DBBF can specifically intramolecularly cross-link α199 Lys and α299 Lys with the amino group of Hb, resulting in a stable amide structure that stabilizes the structure of the Hb tetramer. However, clinical trials of intramolecular crosslinked product (DCLHb) have shown that the products have significant vascular activity and a short half-life [Citation5]. Therefore, purely intramolecular crosslinked products(tetramers) were required to be improved by polymerization methods. Theoretically, the application of the combined polymerization methods of GDA and DBBF (double polymerization, Dopoly) can reduce the content of dimer without increasing the content of super macromolecules.
The Hb content of human cord blood (HCB) is higher than that of peripheral blood, so, compared with peripheral blood, the same dose of HCB can get more Hb, whose immunogenicity may also be lower than that of animal-derived blood or adult peripheral blood because the cells are not fully differentiated. The material used in this study is HCB after stem cell extraction, the material source is extensive due to the presence of stem cell centres (Sichuan New Life Stem Cell Technology Co., Ltd., Tianjin Cord Blood Haematopoietic Stem Cell Bank, et al.). In this study, free human cord haemoglobin (HCHb) was used as material to prepare HBOC, which was modified to meet the oxygen physiological needs of the body. Since low average molecular weight HBOC with low dimer belongs to the development trend, it is the first time to prepare HBOC with low average molecular weight using free HCHb with Dopoly. The main purpose of this study is to investigate the effect of Dopoly in increasing the polymerization ratio of HCHb without increasing the average molecular weight of Dopoly-HCHb and to evaluate the efficacy of 135% exchange transfusion (ET) in rats of a new type of product.
Material and methods
Material
Human cord blood (Sichuan New Life Stem Cell Technology Co., Ltd., Sichuan, China), GDA (Sigma, St. Louis, MO, AR, G6257-1L, batch number), DBBF (500 g, Shenzhen Gewuzhixin Chemical Technology Co., Ltd., Shenzhen, China), albumin (10 g, 20%, 50 mL Baxter, Registration Certificate No: 20160018), sodium pentobarbital (Sigma, St. Louis, MO, batch number 20171225); heparin sodium injection (Nanjing Xinbai Pharmaceutical Co., Ltd., Nanjing, China, batch number 20171004); 0.9% sodium chloride injection (Sichuan Kelun Pharmaceutical Co., Ltd., Sichuan, China, batch number W2017032607); anhydrous glucose (Chengdu Kelong Chemical Reagent Factory, Chengdu, China, batch number: 2017060101), sodium hydroxide (Chengdu Kelong Chemical Reagent Factory, batch number: 2017071001), inositol hexaphosphoric acid (IHP) (Chengdu Kelong Chemical Reagent Factory, batch number 2018091801), disodium hydrogen phosphate (Chengdu Kelong Chemical Co., Ltd, 500 g, batch number 2018050401), sodium dihydrogen phosphate (Chengdu Kelong Chemical Co., Ltd., 500 g, batch number 2017070102j), high-performance liquid chromatography (HPLC) (Waters 2695, Milford, MA, 2489 UV/visible Detector), size exclusion chromatography HPLC column series (Sepax Technologies Zenix-C SEC-300 300 Å 3 µm; Newark, DE), blood gas analyser (TCS Scientific Corp, New Hope, PA).
Purification of HCHb
The HCB was washed with pyrogen-free 0.9% saline, and centrifuged with 3000 prm/min for 15 min at 4 °C, then the supernatant was removed, and repeated three times to obtain RBCs. Add 2 times the volume of rupture fluid was added into the RBCs to obtain the free HCHb, impurities were removed, added reducing agent and protective agent were added into the HCHb solution and deoxygenates, followed by filtration and ultrafiltration to obtain purified HCHb. The method sees the previous study [Citation14].
Intramolecular cross-linking method
Of 100.0 mL purified deoxygenated HCHb solution was put into a 250 mL suction filter flask at (25.0 ± 2.0)°C at a constant speed (300 r/min), nitrogen, and the pH (7.4, 8.0 and 8.5) was adjusted with ascorbic acid (3.0%) and sodium hydroxide solution (4.0%). Different volumes of IHP solution were added into deoxygenated HCHb solution and adjusted its concentration (5.0, 10.0 and 15.0 mM/L). Different volumes of DBBF solution were added to adjust the mole ratio of cross-linking agent to HCHb (5.0, 6.0 and 7.0), the lysine solution (mole ratio of lysine to HCHb was 40.0:1.0) was added to terminate the reaction after 180 min. The MWD, oxygen affinity and the content of MetHb of intramolecularly cross-linked HCHb with DBBF (DBBF-HCHb) were detected.
Intermolecular polymerization method of HCHb
Of 100 mL intramolecular cross-linked deoxygenated 6.0% HCHb solution was put into a 250.0 mL suction filter flask at (4.0 ± 2.0)°C, nitrogen, the solution was stirred at a constant speed (400.0 r/min) at pH7.4, the mole ratio of 0.5% GDA and HCHb was 8.0 and the sodium borohydride solution to was added to terminate the reaction after for 90 min. The product was filtered and ultra-filtered for later use, named GDA-HCHb.
Intermolecular polymerization method of DBBF-HCHb
Of 100 mL intramolecular cross-linked deoxygenated DBBF-HCHb solution was put into a 250.0 mL suction filter flask at (4.0 ± 2.0)°C, nitrogen, the solution was stirred at a constant speed (400.0 r/min), the pH (7.30 ± 0.05) was adjusted with ascorbic acid (3.0%) and sodium hydroxide solution (4.0%), the different doses of GDA was added into the DBBF-HCHb solution to adjust the mole ratio of the polymerization agent to DBBF-HCHb (5.0, 6.0, and 7.0) and the 0.1% sodium borohydride solution (mole ratio of sodium borohydride to DBBF-HCHb was 20.0:1.0) to was added to terminate the reaction after for 90.0 min. The MWD, oxygen affinity and the content of MetHb of intermolecular polymerization DBBF-HCHb with GDA (DBBF-GDA-CHb) were detected.
Detection method of MWD
The detection method of MWD was mainly analysed via HPLC (waters e2695/2489). The mobile phase was 150 mmol/L phosphate buffer (PB, pH7.4) solution, and the flow rate was 0.5 mL/min, and the detection wavelength was 214.0 nm at 25.0 °C. A standard curve based on the molecular weight marker and the time was made firstly, then loaded the sample, and calculated the molecular weight of a sample based on the time. Among them, the detection indicators include MWD, dimer, the number of dimer and average molecular weight, average molecular weight = Σmolecular weight * the proportion in spectrum area of this molecular weight of sample [Citation13].
Detection method of oxygen affinity of samples
The oxygen dissociation curve of samples was detected by Hemox Analyser (TCS Scientific Corp). The buffer was a mixed solution of 137 mM NaCl, 2.7 mM KCl, 10 mM KH2PO4, 1.8 mM NaH2PO4, pH 7.4 and the detection temperature of the instrument was set at 37 °C. The detection indicators mainly include the oxygen affinity and Hill coefficient.
Detection method of MetHb content
The MetHb content was detected by the three-wavelength method, and the optical density of the samples solution at 560, 576 and 630 nm, and calculated the MetHb content according to the formula.
135.0% Exchange transfusion and experimental group
Samples were evaluated in this study using the rat 135.0% exchange transfusion model (ETM). First, the exchange blood volume was calculated according to the bodyweight of the rat (exchange blood volume (mL) = rat bodyweight (g) × (6.5 mL/100.0 g) × 135.0%). Brief operation of rat ET experiment: (2.0 ± 0.1)% sodium pentobarbital was used for anaesthesia at a dose of 0.20 ml/100g(rat body weight). Of 0.3 mL sodium heparin solution (500 U) was injected from the femoral artery end for anticoagulation. Arterial (left) and venous (right) catheter implantation in the groyne and were systemically heparinized by injection of sodium heparin at a dose of approximately 300 U/kg (rat body weight). Of 0.5 mL rat blood was collected from the arterial catheter for blood gas analysis and mean arterial pressure (MAP) and heart rate (HR) (baseline). The bloodletting and injection speed should be controlled at 1.0 mL/min, and the bloodletting speed should be uniform during the bloodletting process. Draw 1.0 mL of blood and then injected 1.0 mL of sample (using a syringe pump) at a time. 0.5 mL blood was collected from the arteries catheter for animal MAP, HR and blood gas after ET and after 2.0 h ET. 18 SD male rats (210.0 ± 10.0) g were selected and divided into the control group (5.0% albumin group) (C), the DBBF-GDA-CHb group (3.0% DBBF-GDA-CHb + 3.0% albumin + 0.3%Vc) (D) and the GDA-HCHb group (3.0% GDA-HCHb + 3.0% albumin + 0.3% Vc) (G) according to the reperfusion samples, 6 rats in each group (). Samples from DBBF-GDA-CHb group and GDA-HCHb group were prepared within 30 min reperfusion (First, 10.0% albumin was diluted to 6.0% using sterile pyrogen-free saline, and then 6.0% albumin was mixed with 6.0% DBBF-GDA-CHb and GDA-HCHb solution and reperfused within 30 min). The main physical and chemical quality of the two products meets the internal control standards of the laboratory and had a good storage stability (see ). shows the main physical and chemical indicators of the DBBF-GDA-CHb and GDA-HCHb and shows the main physical and chemical indicators of the DBBF-GDA-CHb and GDA-HCHb at 0 point and after 2 months.
Figure 1. Schematic diagram of experimental grouping. C: control group; D: GDA-DBBF-HCHb group; G: GDA-HCHb group.
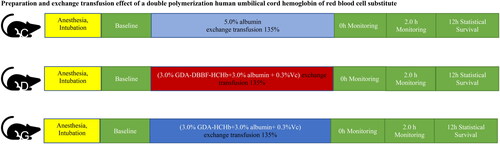
Table 1. Comparison of the main physical and chemical indicators of the two groups of products.
Table 2. Comparison of the main physical and chemical indicators of the two groups of products at 0 point and after 2 months.
Statistical methods
The data in this study were expressed in the form of mean ± standard (). The data of MWD were analysed by t-test analysis, survival rate was analysed by Kaplan–Meier analysis and the blood gas-related indexes were analysed by two-way ANOVA, p < 0.05 indicated that the data was statistically significant, *p < 0.05, **p < 0.01, ***p < 0.001 and ****p < 0.0001.
Results
The MWD of DBBF-HCHb at different pH
The concentration of IHP was 10.0 mmol/L, the mole ratio of DBBF and HCHb (Mole ratioDBBF: HCHb) was 6.0:1.0, the concentration of HCHb was 100.0 g/L at 25.0 °C, the lysine solution was added into the HCHb solution to terminate the reaction after 180 min, and the MWD was shown in of the pH with 7.4, 8.0 and 8.5. The results showed that the dimer (3.2 × 104 g/mol) content in pH8.0 lower than that in pH7.4 (P < 0.0001 as****), while the tetramer (6.4 × 104g/mol) content in the pH8.0 and 8.5 was significantly higher than that in the PH7.4 (P < 0.0001 as****), and there was no statistical difference of the dimer and tetramer content between pH8.0 and pH8.5.
The MWD of DBBF-HCHb under different IHP concentrations
The pH was 8.0, the Mole ratioDBBF: HCHb was 6.0:1.0, the concentration of HCHb was 100.0 g/L at 25.0 °C, the lysine solution was added into the HCHb solution to terminate the reaction after 180 min, and the MWD is shown in of IHP concentration with 5.0 mm/L, 10.0 mm/L and 15.0 mm/L. The results showed that the dimer content in CIHP = 10.0 mmol/L was lower than that of CIHP = 5.0 mmol/L (P < 0.0001 as****), and there was no statistical difference of the dimer and tetramert content between CIHP = 10.0 mmol/L and CIHP = 15.0 mmol/L.
The MWD of DBBF-HCHb under different Mole ratioDBBF: HCHb
The pH 8.0, the concentration of HCHb was 100.0 g/L at 25.0 °C, the IHP concentration was 10.0 mm/L, the lysine solution was added into the HCHb solution to terminate the reaction after 180 min, and the MWD is shown in of the Mole ratioDBBF: HCHb with 5.0, 6.0 and 7.0. The results showed that the dimer content in Mole ratioDBBF: HCHb with 6.0 was lower than Mole ratioDBBF: HCHb with 5.0 (P < 0.0001 as****), and there was no statistical difference of the dimer and tetramert content between Mole ratioDBBF: HCHb with 6.0 and 7.0.
The P50 and Hill co and MetHb of DBBF-HCHb under different Mole ratioDBBF: HCHb
The pH 8.0, the concentration of HCHb was 100.0 g/L at 25.0 °C, the IHP concentration was 10.0 mm/L, the lysine solution was added into the HCHb solution to terminate the reaction after 180 min. The P50 and Hill co changing are shown in and MetHb is shown in of DBBF-HCHb of the Mole ratioDBBF: HCHb with 5.0, 6.0, 7.0 and 8.0. The results showed that P50 and MetHb increased as the Mole ratioDBBF:HCHb increasing [P < 0.0001 as****(red), P<0.001 as***, P<0.01 as**, and P<0.05 as* (red as P50 and black as MetHb)] and Hill Co decreased as the Mole ratioDBBF: HCHb increased [P< 0.0001 as****(blue), P < 0.001 as***(blue), and P<0.05 as*(blue as Hill Co)].
The MWD of DBBF-GDA-CHb under the different mole ratios of GDA and DBBF-HCHb
The concentration of HCHb was 100.0 g/L at 25.0 °C, pH 8.0, the IHP concentration was 10.0 mm/L, the Mole ratioDBBF: HCHb was 6.0, after 180 min, the pH was adjusted to 7.4 using ascorbic acid, the temperature was controlled at 4 °C, and the concentration of DBBF-HCHb solution was adjusted to 60 g/L, the 0.5% GDA solution was added into the DBBF-HCHb solution by microporous titanium rod(0.5 μm), the mole ratios of GDA and DBBF-HCHb (Mole ratio GDA:DBBF-HCHb) was 6.0, the sodium borohydride solution was added to terminate the reaction after 120 min, the MWD is shown in of the Mole ratioGDA:DBBF-HCHb with 5.0, 6.0 and 7.0. The results showed that the tetramer content decreased as the Mole ratioGDA:DBBF-HCHb increased (P<0.05 as*) and the molecular weight of 1.28*105 increased as the Mole ratioGDA:DBBF-HCHb increased (P<0.01 as**).
The P50 and Hill co and MetHb of DBBF-GDA-CHb under different Mole ratioGDA:DBBF-HCHb
The concentration of HCHb was 100.0 g/L at 25.0 °C, pH 8.0, the IHP concentration was 10.0 mm/L, the Mole ratioDBBF: HCHb was 6.0, after 180 min, the pH was adjusted to 7.4 using ascorbic acid, the temperature was controlled at 4 °C, and the concentration of DBBF-HCHb solution was adjusted to 60 g/L, the 0.5% GDA solution was added into the DBBF-HCHb solution by microporous titanium rod (0.5 μm), the mole ratios of GDA and DBBF-HCHb (Mole ratioGDA:DBBF-HCHb) was 6.0, the sodium borohydride solution was added to terminate the reaction after 120 min, and the P50 and Hill co changing were shown in and MetHb changing was shown in of the Mole ratioGDA:DBBF-HCHb with 5.0, 6.0 and 7.0. The results showed that MetHb increased as the Mole ratioGDA:DBBF-HCHb increased (P<0.05 as*) and the P50 and Hill co decreased as the Mole ratioGDA:DBBF-HCHb increased [P<0.05 as*, P<0.01 as**(red as P50 and blue as Hill Co)].
Comparison of survival rate of three groups
Eighteen SD male rats were selected and divided into the control group, the DBBF-GDA-CHb group and the GDA-HCHb group according to the reperfusion samples, six rats in each group. Compared the effects of the three groups on rat 135% ETM. The results showed that the 12 h survival rate of the control group was 1/6(16.67%), and the 12 h survival rate of the DBBF-GDA-CHb group and the GDA-HCHb group were both 5/6(83.33%), and the difference was statistically significant (P = 0.029, χ2 = 7.065,) (P < 0.05 as*), as shown in .
Changing of carbon dioxide-related indicators in three groups of samples at different time points
showed that TCO2, PCO2, HCO3−, HCO3STD at the end of ET and after 2 h of the ET was significantly lower than the baseline in the control and GDA-HCHb group (P< 0.05 as*, P<0.01 as**, and P<0.0001 as****), and there was no statistical difference of TCO2, PCO2, HCO3−, HCO3STD between the baseline, end of the ET and after 2 h of the ET in DBBF-GDA-HCHb group (P > 0.05).
Changing of HR and MAP in three groups of samples at different time points
showed that HR was not statistically significant at baseline, end of the ET, and after 2h of ET in three groups. Figure 10(B) showed that compared with baseline, MAP was significantly lower at end and after 2 h of ET in the control and GDA-HCHb group (P<0.0001 as****); and the MAP of DBBF-GDA-HCHb group at end of the ET was higher than control and GDA-HCHb group (P<0.0001 as****), the MAP of GDA-HCHb group and DBBF-GDA-HCHb group were higher than control group after 2 h ET (P < 0.01 as** and P<0.05 as*).
Changing of PO2 in three groups of samples at different time points
showed that compared with the baseline, PO2 was significantly increasing at the end of ET in three groups (pP< 0.01 as**, P<0.001 as***, and P<0.0001 as****), PO2 in the control group was significantly higher than the DBBF-GDA-HCHb group after 2 h ET (P< 0.05 as*).
Changing of Beecf, BE and Lac in three groups of samples at different time points
showed that compared with baseline, base excess (BE) in the extracellular fluid compartment (BEecf) were significantly increasing at the end of the ET and after 2 h ET in the same group (P<0.05 as*, P<0.01 as**, and P<0.0001 as****), the Beecf in control group was higher than the GDA-HCHb group and the DBBF-GDA-HCHb group (P<0.001 as***). showed that the BE at end of ET and after 2 h ET was higher than baseline (P<0.05 as*, P<0.01 as**, P<0.001 as***, and P<0.0001 as****). showed that the Lac in the control group and the GDA-HCHb group at the end of ET were higher than baseline (P<0.0001 as****), and the Lac in three groups after 2 h of ET were higher than baseline (P<0.0001 as****), and the DBBF-GDA-HCHb group were significantly lower than control group and GDA-HCHb group at end of ET (P<0.0001 as****).
Discussion
Blood transfusion is an indispensable medical method for surgical operations and on-site rescue. Due to the complexity of human blood types, the insurmountable blood transfusion reaction, the difficulty of natural blood transportation and storage, the risk of infectious diseases and the increasing shortage of blood, RBC substitutes have become the focus of international attention [Citation15,Citation16]. HBOC belongs to a kind of RBC substitute.
Current research [Citation13] showed that the optimal molecular weight range of HBOC cannot be given without determining the specific indications. However, excessive dimers (αβ,αγ) content can induce renal toxicity, and it has been agreed in experimental research to reduce the proportion of dimers in polymerized Hb(αβ,αγ,α2β2,α2γ2,) products [Citation17].
The results showed that the dimer (3.2 × 104g/mol) content at pH = 8.0 was lower than that at pH = 7.5, and the dimer content in CIHP = 10.0 mmol/L was lower than that in CIHP = 5.0 mmol/L, and the dimer content in Mole ratioDBBF: HCHb was 6.0 lower than Mole ratioDBBF: HCHb was 5.0. When deoxyhemoglobin is used, the α chains are cross-linked between Lys 99 α1 and Lys 99 α2 (α-DBBF-Hb). The reaction occurs as one of the carbonyls of fumarate react with the side amine group of lysine forming an amide bond, and a dibromoaspirin group of DBBF is removed [Citation18]. The amide bond is a negatively charged functional group, and the alkaline environment can promote the reaction, so increasing the pH can increase the reaction rate, but its influence will gradually decrease with the gradual increase of pH, so the pH of this reaction was determined at 8.0. IHP is an analogue of 2,3-DPG and is an allosteric effector that correctly organizes the structure of the tetramer [Citation19] and promotes the reaction of DBBF with HCHb. Therefore, increasing the concentration of IHP can improve the efficiency of the reaction, but when it reaches a certain concentration, the effect on the reaction will be reduced, so the CIHP was selected 10 mmol/L. DBBF is the substrate, and the reaction is gradually carried out with the increase of substrate, the less dimer content, the more tetramer content. Therefore, the tetrameric content at Mole ratioDBBF: HCHb of 6.0 is higher than that with Mole ratioDBBF: HCHb of 5.0. The authors think that there may be partial dimers that cannot form a normal tetrameric structure. Because we tried to separate the unpolymerized dimer ultrafiltration and use DBBF cross-linking again, and the cross-linking effect was minimal. Therefore, with the addition of DBBF, the effective cross-linking rate has not been significantly improved.
HBOC was prepared by dopoly, the results suggested that compared with GDA-HCHb, DBBF-GDA-HCHb can reduce the dimer content from 25.0% to 8.0% on the basis of super macromolecules no more than 1.0%. The two dimers cross-linked into a stable tetramer by intramolecular cross-linking by DBBF, and GDA not only polymerizes the unpolymerized dimer again, but also polymerizes the dimers into tetramers.
Dopoly not only reduced the dimer content and maintained the structural and functional integrity of tetramers, but also decreased the oxygen affinity. There is currently no conclusive description of which oxygen affinity products are most suitable as RBC substitutes for clinical applications. But 4 of 5 different products used in sixteen trials from 1980 to 2008 had an oxygen affinity similar to or lower than normal human blood [HemAssist (P50 = 32 mmHg), Hemopure (P50 = 32–38 mmHg), PolyHeme (P50 = 26–30 mmHg), Hemolink (P50 = 34 mmHg)], which can provide oxygen to hypoxic tissues and organs more quickly [Citation6]. However, the established relationship between low oxygen affinity and increased autoxidation and possibly oxidative modification reactions suggests that these oxidative processes may actually be enhanced in some of the chemically modified HBOCs [Citation20]. So, a suitable oxygen affinity of the HBOC was prepared by dopoly (P50 = 18.23 mmHg), whose P50 is significantly higher than the GDA-HCHb. The P50 and MetHb increased as the Mole ratioDBBF:HCHb increased and the Hill Co decreased as the Mole ratioDBBF: HCHb increased. P50 increased from (12.11 ± 1.32) mmHg at purified Hb to (32.87 ± 3.04) mmHg at Mole ratioDBBF: HCHb = 8.0:1.0. This process may alter the internal binding sites of HCHb to oxygen and limit the synergistic effects of HCHb itself. DBBF, as an functional analogue of 2,3-DPG, cannot be freely dissociated from tetramers, has a positive effect on the fixation of the HCHb tetramer, but has a detrimental effect on the flexibility of the HCHb tetramer, weakening the ability of one haem to bind oxygen to the ability of another haem to bind oxygen [Citation18,Citation21]. The process of HCHb as the material for the preparation of HBOC with DBBF was similar to bovine Hb and pig Hb in the changing of P50, Hill Co and MetHb [Citation18,Citation22].
The results showed that the not only oxygen affinity of HCHb, but also the oxygen-binding properties were influenced by dopoly process. Under the same partial pressure of oxygen conditions, the content of MetHb gradually increased as the mole ratio of DBBF to HCHb increased, MetHb increased from (0.31 ± 0.21)% at purified Hb to (10.81 ± 3.02) % at Mole ratioDBBF: HCHb= 8:1. The possible reason is that HCHb is cross-linked with DBBF gradually, the oxygen affinity of DBBF-HCHb gradually decreases, resulting in that free oxygen in the solution increasing, and free oxygen interacts with HCHb. This reaction process produces excessive MetHb, which can explain why MetHb gradually increased. The reaction of GDA and DBBF-HCHb also showed the same trend. With the increasing of the mole ratio of GDA and DBBF-HCHb, the P50 and Hill Co of the products also decreased, and the content of MetHb also increased. Hill Co decreased from (2.51 ± 0.20) at DBBF-HCHb to (1.86 ± 0.12) at Mole ratioDBBF: HCHb = 8:1, while MetHb increased from(2.43 ± 0.24)% at DBBF-HCHb to (6.17 ± 2.17) % at Mole ratioGDA:DBBF-HCHb = 7:1. This is consistent with the trend in data from related studies [Citation21,Citation23] that chemical modification increases the MetHb and decreases the Hill Co of Hb. Therefore, to obtain products with better allosteric effects, the addition of cross-linking agents should be minimized when preparing HBOC. So, in the crosslinking and polymerization processes, in the absence of statistical differences in MWD, a lower proportion of crosslinkers is selected. Based on the above preparation principles, we prepared one new type of HBOC, DBBF-GDA-HCHb with low dimer and high polymerization rate by dopoly.
Supplying oxygen in time is the biggest advantage of HBOC [Citation24]. In order to minimize the effect of other damage caused by ischaemic volume, ETM was selected as the evaluation model for this new type of HBOC, because the ETM can better control the blood volume in the rat body compared with the HS model, it only evaluates its oxygen supply to hypoxic tissues and organs. The reason for choosing the 135% ETM was that if the ET volume was further expanded, there were not enough rat samples in the control group for collection because some rats could not survive for 2 h after ET. HBOC has different oxygen-carrying/releasing capacity and processes compared with RBCs. Because HBOC has no cell membrane and has a smaller molecular diameter, and it can better supply oxygen to the hypoxic tissues. Previous studies [Citation24,Citation25] have pointed out that excessive HBOC concentration can cause oxidative stress and oxidative damage, and the 2.0% HBOC formula can meet the oxygen supply to hypoxic tissues and reduce oxidative stress. However, in order to maintain the colloidal osmotic pressure of the solution during ET, a concentration of 3.0% DBBF-GDA-HCHb was chosen for this study. The 12 h survival rate of the control group was 16.67% (1/6), and the GDA-HCHb and DBBF-GDA-HCHb groups were both 83.33% (5/6), and the difference was statistically significant in rat 135.0% ETM. The results pointed out the new type of HBOC, DBBF-GDA-HCHb with low dimer and high polymerization rate by dopoly was no worse than GDA-HCHb in the overall evaluation of the ETM, which were both better than that of the albumin group.
The results showed that TCO2, PCO2, HCO3−, HCO3STD at the end of ET and after 2 h of ET significantly lower than the baseline in the control and the GDA-HCHb group (p < 0.05). TCO2 decreased from about 25 mmol/L at baseline to about 19 mmol/L after 2h of ET. PCO2 decreased from about 40 mmol/L at baseline to about 30 mmol/L after 2 h of ET. The possible reason is that the carbon dioxide and its derivatives metabolized by the body cannot be discharged into the blood in time due to the dilution of the blood, resulting in a significant decrease in the indexes after ET in the control group and the GDA-HCHb group. While there was no significant difference in the TCO2, PCO2, HCO3−, HCO3STD at the baseline, end of ET and after 2 h of ET of the DBBF-GDA-CHb group. TCO2, PCO2 HCO3−, HCO3STD approximately maintained in 20 mmol/L, 35 mmol/L, 20 mmol/L, 20 mmol/L, respectively. The results showed that the DBBF-GDA-HCHb group was better than the control group and the GDA-HCHb group in improving the carbon dioxide-related indicators after ET in rats. The possible reason is that DBBF-GDA-HCHb has a lower oxygen affinity, which can timely supply oxygen to hypoxic tissues in a state of extreme ischaemia and hypoxia, reduce acidosis and carbon dioxide-related indicators are not significantly reduced.
The results showed that the MAP was significantly lower at end of ET and after 2 h ET than baseline in the control and the GDA-HCHb group; and the MAP of the DBBF-GDA-HCHb group at end of ET was higher than control and GDA-HCHb group (103.50 ± 14.40 vs. 56.17 ± 12.43, 53.50 ± 12.33), the MAP of the GDA-HCHb group and the DBBF-GDA-HCHb group were higher than control group after 2 h ET(96.83 ± 8.30, 94.00 ± 11.31 vs. 63.83 ± 8.37). After ET, MAP was significantly reduced due to the decrease in blood volume, colloid osmotic pressure cannot meet the requirements of plasma osmotic pressure in the three groups. However, the MAP of the DBBF-GDA-HCHb group was higher than that of the other two groups after ET, because the higher content of tetramers in DBBF-GDA-HCHb group products than GDA-HCHb group, tetramers can bind to NO in endothelial cells, and decrease circulating NO, and result in vascular tension and increasing in blood pressure in right [Citation26–28]. Endogenous NO is an important signalling molecule for the autoregulation of systemic and pulmonary vascular tone, and if removed by Hb, this will result in vasoconstriction, systemic and pulmonary hypertension and decreased cardiac output [Citation28,Citation29]. After 2 h ET, both groups of GDA-HCHb and DBBF-GDA-HCHb can provide oxygen, which can relieve tissue hypoxia, improve the hypoxia condition of the body, enhance the compensatory effect of the body, and finally increase MAP.
The results showed that PO2 was significantly higher at the end of ET than at the baseline in the three groups. PO2 in the control group was significantly higher than the DBBF-GDA-HCHb group after 2 h ET (121.83 ± 7.36 vs. 104.50 ± 1.71). The PO2 is an important indicator for evaluating HBOC. PO2 represents the pressure formed by the oxygen dissolved in the solution, and the main factors affecting the change of the amount of oxygen dissolved in the blood are the amount of oxygen inhaled, and the oxygen used by the body. After 2 h ET, the PO2 in the blood of the rats in the control group was significantly higher than that in the DBBF-GDA-HCHb group, because the DBBF-GDA-HCHb group could better transport oxygen to the hypoxic site, which is beneficial to the utilization of oxygen, while the utilization rate of dissolved oxygen by the body in the control group was very low, and the free oxygen content is high, so PO2 in the blood of the rats in the control group should be significantly increased.
The results showed that the Beecf in the control group was higher than the GDA-HCHb group and the DBBF-GDA-HCHb group at end of ET (−13.78 ± 3.34 vs. −6.57 ± 2.50, −6.75 ± 2.42) (p < 0.05), and the Lac in the DBBF-GDA-HCHb group were significantly lower than the control group and the GDA-HCHb group at the end of ET (3.12 ± 0.63, 3.23 ± 0.48 vs. 1.70 ± 0.16) (p < 0.05). BE, Beecf and Lac are all important indicators to evaluate the body’s acidosis. The higher the Lac content in the body, the more obvious the state of acidosis. The important reason for acidosis is the lack of oxygen in the body. Hypoxic state, causing the body’s anaerobic respiration, results in excess lactic acid [Citation30–32]. If the delivery of oxygenated blood is disrupted, hypoxia occurs and rat’s metabolism shifts to the anaerobic pathway with the production of lactic acid. With high lactic acid production, the buffer system becomes insufficient leading to rat’s acidosis, low pH. And the lower pH was positively correlated with BE increase and a trend towards higher pCO2 levels [Citation30]. Therefore, compared with the control group, the HBOC group can better supply oxygen to the hypoxic tissues and organs. Due to the lower oxygen affinity of DBBF-GDA-HCHb, it can provide sufficient oxygen for the hypoxic body in a better and more timely manner, resulting in a significant decreasing in the lactic acid content of DBBF-GDA-HCHb group.
The RBC substitutes with dopoly can reduce the dimer content, increasing effective polymerization rate, which is more conducive to industrial production. Compared with GDA-HCHb, DBBF-GDA-HCHb can provide oxygen to hypoxic tissues and organs in a more timely manner, and can improve the reduction of MAP due to ischaemia. It is the broader application prospects of RBC substitutes with dopoly in clinical.
Author contributions
Wentao Zhou: Conceptualization, Implementation research and conduction the analysis data, Writing-original draft. Shen Li: Implementation research, Conduction research and Sample preparation. Shasha Hao: Conceptualization, Conduction research, Pharmacological studying in rats and Conduction the analysis. Xintong Xie: Conduction research, Sample detection, Data curation. Honghui Zhang: Conduction research and Sample preparation. Jiaxin Liu: Conceptualization and Supervision. Hong Wang: Conceptualization and Supervision, Interpretation of the data. Chengmin Yang: Conceptualization and Supervision.
Supplemental Material
Download MS Excel (20.9 KB)Disclosure statement
No potential conflict of interest was reported by the author(s).
Data availability statement
The authors confirm that the data supporting the findings of this study are available within the article and its supplementary materials.
Additional information
Funding
References
- Mercier Ythier J. Cultured blood versus donated blood: long-run perspectives of the economy of blood. Bio-Med Mater Eng. 2015;25(s1):199–209.
- Blood Needs & Blood Supply. 2019. https://www.redcrossblood.org/donate-blood/how-to-donate/ how-blood-donations-help/blood needs-blood-supply.html
- Eastridge BJ, Hardin M, Cantrell J, et al. Died of wounds on the battlefield: causation and implications for improving combat casualty care. J Trauma. 2011;71(1):S4–S8.
- Holcomb JB, McMullin NR, Pearse L, et al. Causes of death in US special operations forces in the global war on terrorism: 2001–2004. Ann Surg. 2007;245(6):986–991.
- Eastridge BJ, Mabry RL, Seguin P, et al. Death on the battlefield (2001–2011): implications for the future of combat casualty care. J Trauma Acute Care Surg. 2012;73(6):S431–S437.
- Natanson C, Kern SJ, Lurie P, et al. Cell-free hemoglobin-based blood substitutes and risk of myocardial infarction and death: a meta-analysis. JAMA. 2008;299(19):2304–2312.
- Silverman TA, Weiskopf RB, Planning Committee and the Speakers. Hemoglobin-based oxygen carriers: current status and future directions. J Am Soc Anesthesiol. 2009;111(5):946–963.
- García-Ruiz JM, Galán-Arriola C, Fernández-Jiménez R, et al. Bloodless reperfusion with the oxygen carrier HBOC-201 in acute myocardial infarction: a novel platform for cardioprotective probes delivery. Basic Res Cardiol. 2017;112(2):17.
- Lo KK, Bey EA, Patra B, et al. Hemoglobin-based oxygen carrier mitigates transfusion-mediated pancreas cancer progression. Ann Surg Oncol. 2013;20(6):2073–2077.
- Lee NP, Chan KT, Choi MY, et al. Oxygen carrier YQ23 can enhance the chemotherapeutic drug responses of chemoresistant esophageal tumor xenografts. Cancer Chemother Pharmacol. 2015;76(6):1199–1207.
- Li CX, Wong BL, Ling CC, et al. A novel oxygen carrier “YQ23” suppresses the liver tumor metastasis by decreasing circulating endothelial progenitor cells and regulatory T cells. BMC Cancer. 2014;14(1):1–8.
- Munoz CJ, Pires IS, Baek JH, et al. Apohemoglobin-haptoglobin complex attenuates the pathobiology of circulating acellular hemoglobin and heme. Am J Physiol Heart Circ Physiol. 2020;318(5):H1296–H1307.
- Kewei Y, Guoying S, Yanpeng Z, et al. A preparation method of polymerized hemoglobin with low polymer content: CN111217904A[P]. 2020-06-02.
- Li F, Zhang H, Wang J, et al. Purification and viral inactivation of hemoglobin from human placenta blood. Artif Cells Blood Substit Immobil Biotechnol. 2009;37(2):57–60.
- Meledeo MA, Peltier GC, McIntosh CS, et al. Freeze-dried plasma mitigates the dilution effects of a hemoglobin-based oxygen carrier (HBOC-201) in a model of resuscitation for hemorrhage and hemodilution. J Trauma Acute Care Surg. 2019;87(1):S83–S90.
- Gupta AS. Hemoglobin-based oxygen carriers: Current state-of-the-art and novel molecules. Shock (Augusta, GA). 2019;52(1):70.
- Benitez Cardenas AS, Samuel PP, Olson JS. Current challenges in the development of acellular hemoglobin oxygen carriers by protein engineering. Shock. 2019;52(1):28–40.
- D’Agnillo F, Alayash AI. Site-specific modifications and toxicity of blood substitutes. The case of diaspirin cross-linked hemoglobin. Adv Drug Deliv Rev. 2000;40(3):199–212.
- Laberge M, Kövesi I, Yonetani T, et al. R-state hemoglobin bound to heterotropic effectors: models of the DPG, IHP and RSR13 binding sites. FEBS Lett. 2005;579(3):627–632.
- Alyash A. Redox and radical reactions of hemoglobin solutions: toxicities and protective strategies. Blood substitutes. London: Academic Press; 2006. p. 197–205.
- Eike JH, Palmer AF. Effect of Cl- and H + on the oxygen binding properties of glutaraldehyde-polymerized bovine hemoglobin-based blood substitutes. Biotechnol Prog. 2004;20(5):1543–1549.
- Cai J, Meng W, Yuan Z, et al. Effect of different reaction conditions on properties of DBBF-modified porcine hemoglobin products. J Zhejiang Univ (Eng Sci). 2009;43(12):2323–2326.
- Alayash AI, Summers AG, Wood F, et al. Effects of glutaraldehyde polymerization on oxygen transport and redox properties of bovine hemoglobin. Arch Biochem Biophys. 2001;391(2):225–234.
- Zhou W, Zhang H, Chen G, et al. Guiding principles for designing research on HBOCs[M]//NANOBIOTHERAPEUTIC BASED BLOOD SUBSTITUTES. Series Editor:Thomas Ming Swi Chang.World Scientific Publishing Co. Pte. Ltd. 5 Toh Tuck Link, Singapore. 2022. p. 39–62.
- Li Y, Yan D, Hao S, et al. Polymerized human placenta hemoglobin improves resuscitative efficacy of hydroxyethyl starch in a rat model of hemorrhagic shock. Artif Cells Nanomed Biotechnol. 2015;43(3):174–179.
- Chen JY, Scerbo M, Kramer G. A review of blood substitutes: examining the history, clinical trial results, and ethics of hemoglobin-based oxygen carriers. Clinics. 2009;64(8):803–813.
- Alayash AI. Blood substitutes: why haven’t we been more successful. Trends Biotechnol. 2014;32(4):177–185.
- Yu B, Liu Z, Chang TMS. Polyhemoglobin with different percentage of tetrameric hemoglobin and effects on vasoactivity and electrocardiogram. Artif Cells Blood Substit Immobil Biotechnol. 2006;34(2):159–173.
- Kim HW, Greenburg AG. Artificial oxygen carriers as red blood cell substitutes: a selected review and current status. Artif Organs. 2004;28(9):813–828.
- Antończyk A, Ochota M, Niżański W. Umbilical cord blood gas parameters and apgar scoring in assessment of new-born dogs delivered by cesarean section. Animals. 2021;11(3):685–697.
- Nicolescu LC, Nicolescu CM, Mihu AG, et al. The effect of red blood cell transfusion on peripheral tissue oxygen delivery and consumption in septic patients. Transfus Clin Biol. 2021;28(1):5–10.
- Gonzales Carazas MM, Gavidia CM, Davila Fernandez R, et al. Biological evaluation of a mechanical ventilator that operates by controlling an automated manual resuscitator. A descriptive study in swine. PLoS One. 2022;17(3):e0264774(1–18).