Abstract
Hepatitis A virus (HAV) is mainly transmitted via contaminated food and water. HAV infection is a major global public health problem. Thus, developing a simple, rapid detection method is crucial for containing HAV epidemics, particularly in developing regions with limited laboratory resources. This study established a feasible HAV detection solution by combining reverse transcription multienzyme isothermal rapid amplification (RT-MIRA) and lateral flow dipstick (LFD) strips. Primers targeting the conserved 5’UTR sequence of HAV were used in the RT-MIRA-LFD assay. RNA extraction was enhanced by obtaining RNA directly from the centrifuged supernatant. Our study found that MIRA amplification could be finished in 12 min at 37 °C and naked-eye observation of the LFD strips in 10 min. The detection sensitivity of this method reached 1 copy/μl. RT-MIRA-LFD was compared to conventional RT-PCR using 35 human blood samples. The accuracy of the RT-MIRA-LFD method was 100%. The convenience, sensitivity, and rapidness of this detection method could provide a considerable advantage for diagnosing and controlling HAV infection, especially in regions with limited medical resources.
Introduction
Hepatitis A virus (HAV), classified as hepatovirus, is a small, unenveloped symmetrical RNA virus. It belongs to the micro ribonucleic acid viruses and is the cause of infectious or epidemic hepatitis [Citation1]. HAV spreads through the fecal-oral route and is commonly contracted by ingesting food or water contaminated with the faeces of infected patients and vegetables fertilised with faeces and uncooked shellfish [Citation2–4]. In developing regions, the source pollution of primary and processed agricultural products is still a serious problem. Although, the infection of HAV is more common in developing countries, an increased number of HAV cases has been reported in developed countries [Citation5]. In developed cities, food may also be contaminated by unkilled HAV virus [Citation6]. A 2019 HAV-mediated outbreak in the United States was linked to imported fresh blackberries [Citation7]. HAV infections cause nausea, vomiting, abdominal pain, jaundice, fever, and diarrhoea [Citation8]. Approximately 10 to 15% of infected patients will have recurring symptoms within six months of the initial infection. In severe cases, acute liver failure may be life-threatening [Citation9]. Moreover, HAV infections during community-based, person-to-person outbreaks cannot be ignored [Citation10].
HAV infection is a global public health problem. Around the world, 1.5 million people are infected with HAV each year, and the number of undiagnosed infections may reach tens of millions [Citation11]. In China, hepatitis A outbreaks are a public health crisis, causing great panic and a huge shock to the economy. In 1988, 1.5 million people in Shanghai were infected by HAV transmitted by Scapharca subcrenata, with more than 300,000 presenting with symptoms [Citation6]. In that year, the cost of free treatment for hepatitis patients far exceeded the health expenditure of Shanghai [Citation12]. Because Hepatitis A often spreads through contaminated food and water, it can infect many people in a short time (a cluster outbreak) [Citation13]. Therefore, a rapid, accurate, and economical diagnostic test for HAV is urgently needed to control hepatitis A epidemics, especially in resource-limited settings [Citation14].
The current gold standard for diagnosing HAV infections is an enzyme-linked immunosorbent assay (ELISA) based on serum anti-HAV IgM levels, which is less effective in detecting recent infections because of a stringent window for initial tests [Citation15]. During an HAV outbreak, when a rapid and precise diagnosis is essential, molecular assays could be introduced to supplement or confirm serology results. Presently, reverse transcription polymerase chain reaction (RT-PCR) is a widely used molecular detection method in the clinic [Citation16]. However, it requires high-precision and expensive devices. Due to limited laboratory facilities and professionals, it cannot be popularised in resource-limited regions.
Several isothermal technologies have been developed for rapid, feasible nucleic detection. Multienzyme isothermal recombinase amplification (MIRA) is a novel nucleic acid amplification method based on recombinase polymerase amplification (RPA) [Citation17]. This technique utilises four core proteins: recombinase-Rec A, DNA helicase-GP41, single-stranded binding (SSB) protein, and DNA pol I [Citation18]. During amplification, helicase-GP41 and SSB form the D-Loop and start the reaction. Recombinase and DNA pol I then allow DNA extension rapidly at isothermal conditions. For RNA template amplification, specific primers can be used to directly synthesise cDNA using reverse transcriptase [Citation19,Citation20]. Simultaneously, the newly generated cDNA is used as a template for MIRA [Citation21]. The whole reaction can be performed at 25 to 42 °C within 5 to 30 min without requiring complex instruments, providing a major advantage over conventional PCR. The lateral flow dipstick (LFD) can produce visually observable results in a relatively short time by hybridising with labelled colloidal gold [Citation22]. Moreover, it provides a convenient, sensitive, and economical assay when combined with RT-MIRA.
To solve the current problems associated with HAV detection and popularise early diagnosis of HAV infection in resource-limited regions, our study integrated RT-MIRA and LFD dipstick technologies to generate a convenient, sensitive, and economical method of HAV diagnosis.
Method
Primer design
The HAV 5′-untranslated region (5′-UTR) is 734 bp, accounts for about 10% of the whole genome, and is the most conserved sequence [Citation16,Citation23]. Therefore, we designed primers targeting 5′-UTR region using Premier 5.0 software, according to the guidelines of AMP-Future Biotech Co. Ltd. (Weifang, China) (). The primer lengths were about 25 to 35 bp, and the amplicon was approximately 150 to 300 bp (). Specificity analysis was performed using BLAST (http://www.ncbi.nlm.nih.gov/blast/Blast.cgi).
Table 1. The primers used for MIRA.
HAV 5’UTR template preparation
The HAV 5′-UTR was cloned into pCDNA 3.1(+) by TSINGKE Biotech Co. Ltd. (Nanjing, China). After plasmid extraction, the DNA concentration was measured using a NanoDropTM One UV spectrophotometer (ThermoFisher Scientific, Waltham, MA), and the copy number was calculated according to Kozak et al. [Citation24].
MIRA
MIRA was performed using the DNA isothermal rapid amplification kit (#WLB8201KIT, AMP-Future Biotech Co. Ltd), according to the manufacturer’s instructions. Two primer pairs (First pair: F-a1 and R-a1; Second pair: F-a1 ad R-a2) were designed to screen the appropriate (). The reaction was performed at 37 °C for 30 min. The generated products (5 μl) were purified with phenol and separated on a 2% agarose gel at 120 V for 1 h. After electrophoresis, the products were visualised using an automatic digital gel image analysis system (Tanon, Shanghai, China). Water was used as a negative control, and the template DNA provided in the kit served as the positive control.
MIRA-LFD
Forward and reverse primers (name: F-a1 labelled and R-a1 labelled) were labelled at the 5′-end with FAM or biotin, respectively (). MIRA-LFD was performed using an amplification kit (Colloidal gold test strip type) (#WLB8203KIT, AMP-Future Biotech Co. Ltd), according to the manufacturer’s instructions. The reactions were performed at 37 °C for 12 min, and the products were diluted 1:5000 with H2O. Aliquots of the diluted products (100 μl) were applied to LFDs (#JY0201, Baoying Tonghui Biotechnology Co. Ltd., Beijing, China), and the LFDs were visually observed after 10 min.
Sensitivity and specificity analysis
To determine the sensitivity of the MIRA reaction, HAV 5′-UTR plasmid DNA was serially diluted with ddH2O in 10-fold increments from 10 pg/μl to 10 fg/μl, and the MIRA products were separated by electrophoresis using a 2% agarose gel. In parallel, the dilutions were subjected to MIRA-LFD and qPCR. qPCR was performed with TaKaRa TB Green Premix Ex Taq II (Tli RNaseH Plus, TaKaRa, Dalian, China) using the Applied Biosystems 7500 Real-Time PCR system (Thermo Fisher Scientific). The qPCR primers were previously reported [Citation25]. The reaction conditions were as follows: 95 °C for 30 s, 40 cycles at 95 °C for 5 s and 60 °C for 34 s. The templates consisted of HAV 5′-UTR plasmid DNA diluted from 10 pg/μl to 10−2 fg/μl. All the amplification reactions were performed in triplicate.
For specificity analysis, the cross-reactivity of the MIRA assay was determined using common infectious viruses, including hepatitis B virus (HBV), hepatitis C virus (HCV), hepatitis E virus (HEV), human immunodeficiency virus-1 (HIV-1), herpes simplex virus (HSV-1), Japanese encephalitis virus (JEV) and rotavirus. All the templates used in specificity analysis were 1 ng/μl plasmid DNA contains the target virus sequence. We also evaluated the specificity of the MIRA-LFD reaction using the human genome as the template, as previously reported [Citation17,Citation26].
RT-MIRA
For better quantitative analysis and experimental safety, we used HAV live-attenuated vaccine strain H2 instead of live hepatitis A virus [Citation27]. Due to a mutation in the 5′-UTR of strain H2 [Citation28] resulting in virus attenuation and cell adaptation, we adjusted the reverse primer sequences accordingly (primer name: F-a1A and R-a1A)(). RNA was extracted from strain H2 using the rapid nucleic acid release agent (RNA type) (#WLR8203, AMP-Future Biotech Co. Ltd., Weifang, China). The extracted RNA was purified using the RNAclean Kit (DP412, Tiangen, China) to remove the vaccine excipients, which can inhibit the isothermal amplification.
Table 2. The primers used for RT-MIRA of HAV vaccine strain H2.
RT-MIRA was performed using the RNA isothermal rapid amplification kit (#WLB8207KIT, AMP-Future Biotech Co. Ltd). Amplification and analysis were performed as described for MIRA, using water as a negative control and plasmid DNA as a positive control.
RT-MIRA-LFD
Different from the primers used in RT-MIRA reaction, the forward and reverse primers were labelled, respectively (primer name: F-a1A labelled and R-a1A labelled) (). RNA template was also extracted from strain H2 using the rapid nucleic acid release agent. RT-MIRA-LFD was performed using an amplification kit (Colloidal gold test strip type) (#WLB8209KIT, AMP-Future Biotech Co. Ltd). Amplification and detection using LFDs were as described for MIRA-LFD.
DNA sequencing
The MIRA products were sequenced by Sanger DNA sequencing using the ABI3730XL (Taihe Biotechnology Co. Ltd., Beijing, China). Primer F-a1 was used for sequencing ().
Clinical sample analysis
A total of 35 peripheral blood samples were collected at local hospitals, of which 19 were identified as HAV-positive by RT-PCR [Citation29]. RNA was extracted from 0.5 ml peripheral blood using the rapid nucleic acid release agent (#WLR8203, AMP-Future Biotech Co. Ltd., Weifang, China). The extracted RNA was then amplified using the RT-MIRA-LED kit and detected with LFDs (lateral flow nucleic acid test notes).
Results
Selection of primers
We validated two primer pairs designed to recognise the HAV 5′-UTR (, ). To optimal primer pair was determined by performing MIRA on 10-fold serial dilutions of HAV 5′-UTR plasmid DNA (). The results showed that although both the a1 and a2 primers could detect templates at concentrations ranging from 104 copies/μl to 107 copies/μl, the a1 primers appeared to be more efficient, producing more intense bands. Therefore, the a1 primers were used for the subsequent experiments. The sequence of the MIRA product generated using the a1 primers is shown in . No other pathogens tested could be detected by MIRA using the a1 primers ().
Figure 2. MIRA products separated on a 2% agarose gel. L: 50-bp DNA Ladder; 1: positive control (∼250 bp); 2: primer a1 (∼180 bp); 3: primer a2 (∼269 bp); 4: primer a1, negative control; 5: primer a2, negative control.
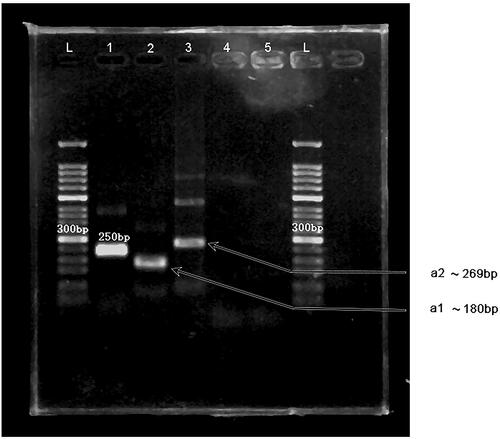
Figure 3. The MIRA products for the serial dilution of HAV plasmid DNA separated on a 2% agarose gel. L: 50-bp DNA Ladder; 1: positive control (∼250 bp); 2: 107 copies/μl template with a1 primers; 3: 106 copies/μl template with a1 primers; 4: 105 copies/μl template with a1 primers; 5: 104 copies/μl template with a1 primers; 6: 107 copies/μl template with a2 primers; 7: 106 copies/μl template with a2 primers; 8: 105 copies/μl template with a2 primers; 9: 104 copies/μl template with a2 primers.
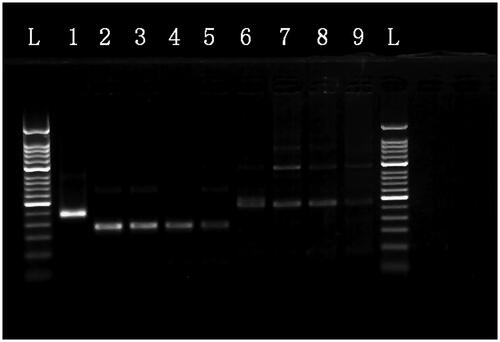
MIRA-LFD
We performed 10-fold serial dilutions of HAV5’-UTR plasmid DNA (105 copies to 0.1 copy/μL) and analysed the samples using MIRA-LFD. We found that the test strips could detect plasmid DNA at concentrations as low as 0.1 copy/μL (). In contrast, the water and human genome template samples yielded negative results. In parallel, we analysed 10-fold serial dilutions of plasmid from 107 copies to 10 copy/μL using qPCR, which had a detection limit of 102 copies (). These results indicated that MIRA-LFD was more sensitive than qPCR for detecting HAV.
Figure 6. Serial dilution of HAV plasmid DNA detected by MIRA-LFD. 1: water template; 2: human genome template; 3: 106 copies/μl template; 4: 105 copies/μl template; 5: 104 copies/μl template; 6: 103 copies/μl template; 7: 102 copies/μl template; 8: 10 copies fg/μl template; 9: 1 copy/μl template; 10: 0.1 copy/μl template.
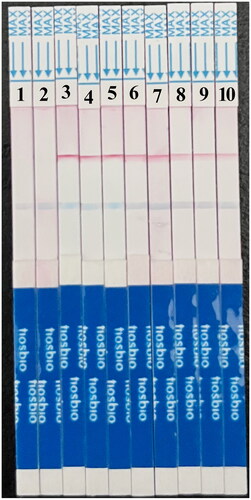
RT-MIRA
RT-MIRA was performed on 10-fold serial dilutions of strain H2 RNA (106 copies/μl to 102 copies/μl) (). All templates could be detected by this method. The sequence of the RT-MIRA product is shown in .
Figure 8. RT-MIRA products for the serial dilution of HAV vaccine strain H2 template separated on a 2% agarose gel. L: 50-bp DNA Ladder; 1: plasmid DNA template, positive control; 2: water template, negative control; 3: 106 copies/μl template; 4: 105 copies/μl template; 5: 104 copies/μl template; 6: 103 copies/μl template; 7: 102 copies/μl template
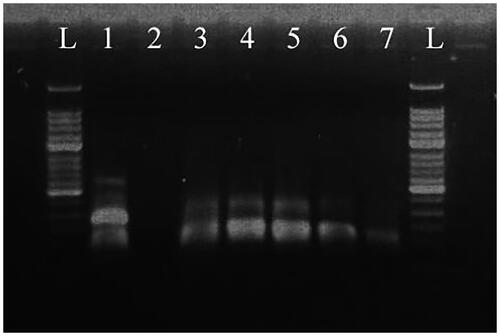
RT-MIRA-LFD
Similar to the MIRA-LFD results, the concentrations of strain H2 from 105 copies/μl down to 1 copy/μl were detected using RT-MIRA-LFD. In contrast, nothing was detected in the human genome template sample (). The limit of detection is 1.56 ± 0.28 copies/μl (95% CI = 0.7916-2.3284).
Figure 10. Serial dilution of HAV vaccine strain H2 template detected by RT-MIRA-LFD. 1: water template; 2: human genome template; 3: 105 copies/μl template; 4: 104 copies/μl template; 5: 103 copies/μl template; 6: 102 copies/μl template; 7: 10 copies/μl template; 8: 1 copy/μl template; 9: 0.1 copy/μl template.
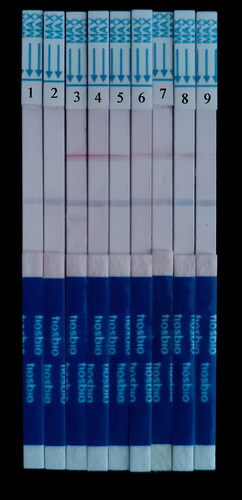
Clinical sample analysis
The RT-MIRA-LFD assay was validated using 19 positive and 16 negative clinical samples. The results obtained by RT-MIRA-LFD were consistent with the results from traditional RT-PCR (), indicating that RT-MIRA-LFD has great potential as a clinical application.
Table 3. HAV detection in clinical samples.
Discussion
We established a new field-adapted HAV detection method by combining RT-MIRA and LFD. The scheme of our method is summarised in . HAV RNA is extracted from blood samples using a rapid RNA release agent. The RNA can be obtained directly from the supernatant after moderate vortexing and centrifugation. The entire RT-MIRA reaction took only 12 min at 37 °C. The product was then diluted and applied to the test strip, with positive results visually observed after 10 min. This method could effectively avoid the disadvantages of existing detection technology and does not require complex equipment or operation. Thus, it is suitable for promotion and popularisation in resource-limited regions. Moreover, after qualification according to Kim’s method[Citation30], its detection sensitivity even reached 1 copy/μl in clinical samples, indicating this assay could play a significant role in preventing and controlling the spread of HAV infection.
With the rapid development of molecular biomedical technologies in recent years, several isothermal amplification solutions for both DNA and RNA have been advanced. Nuclear acid sequence-based amplification (NASBA) and simultaneous amplification and testing (SAT) are two current RNA isothermal amplification technologies. Both techniques involve a complex process of continuous transcription and reverse transcription between cDNA and RNA to achieve nucleic acid amplification. NASBA relies heavily on manual handling at 42 °C [Citation31], while SAT requires repeated addition of reverse transcriptase and T7 RNA polymerase [Citation32]. Thus, both techniques require specialised experts and are unsuitable for resource-limited regions.
For HAV detection, real-time reverse transcription loop-mediated isothermal amplification (RT-LAMP) can be finished in 50 min at 62.5 °C [Citation33]. Multiple cross displacement amplification (MCDA) [Citation34–36], cross-priming amplification (CPA) [Citation37], and RT-LAMP [Citation38–41] have been proved to detect various pathogens at about 60 °C within one hour. In addition, of all these methods, more than four primers need to be designed which is complicated and the verification steps of amplicons are also complicated. RPA technique, which also depends on multiple enzymes, is the foundation of MIRA [Citation18,Citation42]. The reaction can be performed at 37 ∼ 42 °C for 20 ∼ 40 min and only needs one pair of primers. However, RPA is not ideal for distinguishing mutations or identifying mutations based on nested-RPA [Citation43], and the cost of RPA assay could reach 12 dollars/reaction. Some PCR-based techniques just like real time PCR, rapid single-tube protocol using nested real-time PCR and reverse transcription-droplet digital PCR method for quantitative detection have been reported [Citation25,Citation41,Citation44–46]. Unfortunately, these methods cannot be widely popularised in remote, less-developed areas due to the high price of the machines.
Our RT-MIRA method only requires 12 min at 37 °C, which is important for the rapid detection of HAV to control the spread of the virus. RT-MIRA is a highly sensitive and selective isothermal amplification technique with minimal sample preparation and is capable of amplifying as low as 1 to 10 DNA target copies in less than 20 min [Citation47]. Additionally, it does not require the strict laboratory conditions of earlier methods [Citation18]. At room temperature, a modified reverse transcriptase synthesises cDNA strands using specifically designed primers and template RNA. Recombinase, SSB protein, and DNA polymerase in the reaction system use the newly synthesised cDNA strands as templates for rapid nucleic acid amplification [Citation17]. Furthermore, the reverse transcription and amplification steps are carried out simultaneously [Citation21], with the product cDNAs amplified directly, greatly simplifying the process, and speeding up the analysis. After amplification, an LFD strip can be used to detect the products using labelled primers.
The whole detection process can be completed within 30 min compared to three hours for ELISA, four hours for RT-PCR and one hour for RT-LAMP. The cost of MIRA reaction is about 6.5 dollars/sample, which is roughly half that of the RPA reaction. Moreover, MIRA reaction does not require an expensive PCR instruments, and only requires constant temperature reaction at 37 °C for 12 min. And if the time cost and the difficulty of primer design was considered, the total cost of each reaction of has no obvious difference compared with RT-LAMP [Citation48]. Therefore, this technique is a feasible approach for detecting potential HAV infections via human blood samples, which could improve the diagnosis and control of HAV [Citation22].
The current study only tested our new RT-MIRA assay using blood samples; however, stool and food samples also play vital roles in HAV diagnosis and disease control [Citation49]. Thus, further studies are essential for assessing the feasibility of using this RT-MIRA-LFD on other biological sample types.
In summary, the combination of RT-MIRA and LFD is a convenient, sensitive, and economical approach that does not require special instrumentation. The entire RT-MIRA-LFD process only takes 12 min at 37 °C, and the results can be visualised ten minutes after applying the reaction products to the test strip. Therefore, this method has great potential for use in resource-limited regions, especially in high HAV endemic areas.
Author contributions
All the authors involved played an important role and contributed to the article. All authors approved the submitted version. YQP and JY contributed to the research design. MLS and YZ analysed the data. MLS provided clinical samples and conducted the molecular biology experiments. JY performed the experiments and provided study supervision. YQP and MLS wrote the manuscript.
Disclosure statement
No potential conflict of interest was reported by the author(s).
Data availability statement
The data sets used and/or analysed during this study are available from the corresponding author on reasonable request.
Additional information
Funding
References
- Pintó RM, Pérez-Rodríguez F-J, Costafreda M-I, et al. Pathogenicity and virulence of hepatitis a virus. Virulence. 2021;12(1):1174–1185.
- Vaughan G, Goncalves Rossi LM, Forbi JC, et al. Hepatitis a virus: host interactions, molecular epidemiology and evolution. Infect Genet Evol. 2014;21:227–243.
- Enkirch T, et al. Hepatitis a outbreak linked to imported frozen strawberries by sequencing, Sweden and Austria, June to September 2018. Euro Surveill. 2018;23(41):1800528.
- Dirks RAM, Jansen CCC, Hägele G, et al. Quantitative levels of norovirus and hepatitis a virus in bivalve molluscs collected along the food chain in The Netherlands, 2013-2017. Int J Food Microbiol. 2021;344:109089.
- Chatziprodromidou IP, Dimitrakopoulou M-E, Apostolou T, et al. Hepatitis a and E in the mediterranean: a systematic review. Travel Med Infect Dis. 2022;47:102283.
- Tang YW, Wang JX, Xu ZY, et al. A serologically confirmed, case-control study, of a large outbreak of hepatitis a in China, associated with consumption of clams. Epidemiol Infect. 1991;107(3):651–657.
- McClure M, Nsubuga J, Montgomery MP, et al. A 2019 outbreak investigation of hepatitis a virus infections in the United States linked to imported fresh blackberries. Food Environ Virol. 2022;14(3):236–245.
- Abutaleb A, Kottilil S. Hepatitis A: epidemiology, natural history, unusual clinical manifestations, and prevention. Gastroenterol Clin North Am. 2020;49(2):191–199.
- Matheny SC, Kingery JE. Hepatitis A. Am Fam Physician. 2012;86(11):1027–1034. quiz 1010–2.
- Hagan LM, Montgomery MP, Lauro PL, et al. Recent incarceration among individuals infected with hepatitis a virus during person-to-Person community outbreaks, United States, 2016-2020. Public Health Rep. 2022;doi: 10.1177/00333549221108413.
- Santos GMCd, Alves CR, Pinto MA, et al. Detection of antibodies against hepatitis a virus (HAV) by a surface plasmon resonance (SPR) biosensor: a new diagnosis tool based on the major HAV capsid protein VP1 (SPR-HAVP1). Sensors. 2021;21(9):3167.
- Wang, G. and F.F. Li, Measures and experience of successful response to hepatitis A epidemic in Shanghai in 1988. Party History and Party building in Shanghai, 2020. 395(04): 34–39.
- Tsukada R, Ono S, Kobayashi H, et al. A cluster of hepatitis a infections presumed to be related to asari clams and investigation of the spread of viral contamination from asari clams. Jpn J Infect Dis. 2019;72(1):44–48.
- Vanhomwegen J, et al. Development and clinical validation of loop-mediated isothermal amplification (LAMP) assay to diagnose high HBV DNA levels in resource-limited settings. Clin Microbiol Infect. 2021;27(12):1858 e9–1858 e15.
- Hyun JJ, Seo YS, An H, et al. Optimal time for repeating the IgM anti-hepatitis a virus antibody test in acute hepatitis a patients with a negative initial test. Korean J Hepatol. 2012;18(1):56–62.
- Costafreda MI, Bosch A, Pinto RM. Development, evaluation, and standardization of a real-time TaqMan reverse transcription-PCR assay for quantification of hepatitis a virus in clinical and shellfish samples. Appl Environ Microbiol. 2006;72(6):3846–3855.
- Sun M-L, Lai H-Y, Chong N-Y, et al. Simple and feasible detection of hepatitis B virus via combination of multienzyme isothermal rapid amplification and lateral flow dipstick strip. Front Mol Biosci. 2021;8:763079.
- Piepenburg O, Williams CH, Stemple DL, et al. DNA detection using recombination proteins. PLoS Biol. 2006;4(7):e204.
- Kumar R, Kaundal P, Tiwari RK, et al. Establishment of a one-step reverse transcription recombinase polymerase amplification assay for the detection of potato virus S. J Virol Methods. 2022;307:114568.
- Zou X, Dong C, Ni Y, et al. Rapid detection of strawberry mottle virus using reverse transcription recombinase polymerase amplification with lateral flow strip. J Virol Methods. 2022;307:114566.
- Wang H, Zhang Y, Zhou J, et al. Rapid visual detection of hepatitis C virus using reverse transcription Recombinase-Aided Amplification-Lateral flow dipstick. Front Cell Infect Microbiol. 2022;12:816238.
- Zeng F, Wu M, Ma L, et al. Rapid and sensitive real-time recombinase polymerase amplification for detection of marek’s disease virus. Mol Cell Probes. 2019;48:101468.
- Yong C, et al. Nucleotide sequence heterogeneity of 5 'NCR strains of hepatitis a virus circulating in China. Chin J Microbiol Immunol. 2001;21(6):651–653.
- Kozak RA, Rutherford C, Richard-Greenblatt M, et al. Development and evaluation of a molecular hepatitis a virus assay for serum and stool specimens. Viruses. 2022;14(1):159.
- Persson S, Alm E, Karlsson M, et al. A new assay for quantitative detection of hepatitis a virus. J Virol Methods. 2021;288:114010.
- Chen X, Wang S, Tan Y, et al. Nanoparticle-Based lateral flow biosensors integrated with Loop-Mediated isothermal amplification for the rapid and visual diagnosis of hepatitis B virus in clinical application. Front Bioeng Biotechnol. 2021;9:731415.
- Ma QQ, et al. The infectivity and pathogenicity of hepatitis a virus live-attenuated vaccine strain H2 in type I interferon receptor-deficient mice. Virol Sin. 2022;37(5):740–745.
- Chen Y, Chai SA. cDNA cloning and sequence analysis of live attenuated hepatitis a virus vaccine (strain H). J Zhejiang Acad Med Sci. 1995;6(1):3–14.
- Nainan OV, Xia G, Vaughan G, et al. Diagnosis of hepatitis a virus infection: a molecular approach. Clin Microbiol Rev. 2006;19(1):63–79.
- Kim J-H, Park S-B, Roh H-J, et al. A simplified and accurate detection of the genetically modified wheat MON71800 with one calibrator plasmid. Food Chem. 2015;176:1–6.
- Ju Y, Kim HY, Ahn JK, et al. Ultrasensitive version of nucleic acid sequence-based amplification (NASBA) utilizing a nicking and extension chain reaction system. Nanoscale. 2021;13(24):10785–10791.
- Li J, Sun L, Wu X, et al. Early diagnosis of Mycoplasma pneumoniae in children: simultaneous amplification and testing (SAT) is the key. Front Pediatr. 2019;7:441.
- Yoneyama T, Kiyohara T, Shimasaki N, et al. Rapid and real-time detection of hepatitis a virus by reverse transcription loop-mediated isothermal amplification assay. J Virol Methods. 2007;145(2):162–168.
- Huang J, et al. A novel, ultrafast, ultrasensitive diagnosis platform for the detection of SARS-COV-2 using restriction endonuclease-mediated reverse transcription multiple cross displacement amplification. J Med Virol. 2022;95(2):e28444.
- Wang Y, Wang Y, Ma A-J, et al. Rapid and sensitive isothermal detection of nucleic-acid sequence by multiple cross displacement amplification. Sci Rep. 2015;5:11902.
- Wang Y, Wang Y, Zhang L, et al. Multiplex, rapid, and sensitive isothermal detection of Nucleic-Acid sequence by endonuclease Restriction-Mediated Real-Time multiple cross displacement amplification. Front Microbiol. 2016;7:753.
- Fang R, Li X, Hu L, et al. Cross-priming amplification for rapid detection of Mycobacterium tuberculosis in sputum specimens. J Clin Microbiol. 2009;47(3):845–847.
- Wong Y-P, Othman S, Lau Y-L, et al. Loop-mediated isothermal amplification (LAMP): a versatile technique for detection of micro-organisms. J Appl Microbiol. 2018;124(3):626–643.
- Wang Y, Wang Y, Lan R, et al. Multiple endonuclease restriction Real-Time Loop-Mediated isothermal amplification: a novel analytically rapid, sensitive, multiplex Loop-Mediated isothermal amplification detection technique. J Mol Diagn. 2015;17(4):392–401.
- Sharma V, Chaudhry D, Kaushik S. Evaluation of clinical applicability of reverse transcription-loop-mediated isothermal amplification assay for detection and subtyping of influenza a viruses. J Virol Methods. 2018;253:18–25.
- Kumar R, Nagpal S, Kaushik S, et al. COVID-19 diagnostic approaches: different roads to the same destination. Virusdisease. 2020;31(2):97–105.
- Kim Y, et al. Single-strand RPA for rapid and sensitive detection of SARS-CoV-2 RNA. medRxiv. 2020.
- Ng BYC, Wee EJH, Woods K, et al. Isothermal point mutation detection: toward a First-Pass screening strategy for Multidrug-Resistant tuberculosis. Anal Chem. 2017;89(17):9017–9022.
- Hu Y, Arsov I. A rapid single-tube protocol for HAV detection by nested real-time PCR. Food Environ Virol. 2014;6(3):189–195.
- Broor S, Chahar HS, Kaushik S. Diagnosis of influenza viruses with special reference to novel H1N1 2009 influenza virus. Indian J Microbiol. 2009;49(4):301–307.
- Dhakad S, Mali PC, Kaushik S, et al. Comparison of multiplex RT-PCR with virus isolation for detection, typing and Sub-typing of influenza virus from influenza-like illness cases. Indian J Med Microbiol. 2015;33(1):73–77.
- Lobato IM, O'Sullivan CK. Recombinase polymerase amplification: basics, applications and recent advances. Trends Analyt Chem. 2018;98:19–35.
- Londono MA, Harmon CL, Polston JE. Evaluation of recombinase polymerase amplification for detection of begomoviruses by plant diagnostic clinics. Virol J. 2016;13:48.
- Battistini R, Rossini I, Listorti V, et al. HAV detection from milk-based products containing soft fruits: comparison between four different extraction methods. Int J Food Microbiol. 2020;328:108661.