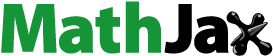
Abstract
Respiratory tract infections are a common cause of morbidity and mortality globally. The current paper aims to treat this respiratory disorder. Therefore, we elucidated the phytochemical profile of Euphorbia milii flowers and isolated chlorogenic acid (CGA) for the first time. The electrospraying technique was utilized to prepare CGA nanoparticles in polyvinyl alcohol (PVA)/PLGA polymeric matrix. Complete in vitro characterizations were performed to determine particle size, polydispersity index (PDI), zeta potential, loading efficiency (LE), scanning electron microscopy and in vitro release study. The optimum formula (F2) with a particle size (454.36 ± 36.74 nm), a surface charge (–4.56 ± 0.84 mV), % of LE (80.23 ± 5.74), an initial burst (29.46 ± 4.79) and % cumulative release (97.42 ± 4.72) were chosen for further activities. In the murine lung infection model, PVA/PLGA NPs loaded with CGA (F2) demonstrated in vivo antibacterial activity against Pseudomonas aeruginosa. Using a plaque assay, the in vitro antiviral activity was investigated. The F2 exhibited antiviral activity against coronavirus (HCoV-229E) and (Middle East respiratory syndrome coronavirus (MERS-CoV), NRCEHKU270). The IC50 of F2 against HCoV-229E and MERS-CoV was 170 ± 1.1 and 223 ± 0.88 µg/mL, respectively. The values of IC50 of F2 were significantly lower (p < .05) than that of free CGA. Therefore, the encapsulation of CGA into electrospray PVA/PLGA NPs would be a promising tool as an antimicrobial agent.
Introduction
Recently, respiratory tract infections have caused an increased rate of morbidity and mortality worldwide [Citation1]. Numerous studies have focussed on this type of infection during the coronavirus disease 2019 (COVID-19) epidemic due to its detrimental effects on global public health and the economy [Citation2]. Respiratory tract infections can be caused by multiple viral or bacterial pathogens [Citation3].
Pseudomonas aeruginosa is one of the key pathogenic bacteria which can cause respiratory tract infections [Citation4]. These bacteria can cause infections in patients experiencing cystic fibrosis and non-cystic fibrosis bronchiectasis. Additionally, they cause infections in individuals with chronic obstructive airway diseases [Citation5]. Many viruses that cause respiratory tract infections have the potential to cause epidemic and pandemic infectious diseases [Citation6]. Severe acute respiratory syndrome coronavirus 2 (SARS-CoV-2), influenza virus and Middle East respiratory syndrome coronavirus (MERS-CoV) are forms of respiratory tract infectious viruses [Citation6].
Respiratory tract infections present a global challenge due to the increasing prevalence of multidrug-resistant bacteria and the very limited number of antiviral agents available, which necessitates the search for novel treatment strategies. Natural substances have recently served as a crucial starting point for developing medicinal ingredients for treating different disorders [Citation7]. For instance, numerous plants have demonstrated potent in vitro and in vivo antimicrobial activity, which has prompted a more focussed investigation into the antimicrobial properties of numerous plants derived materials [Citation8]. A number of active compounds found in plants can effectively inhibit the growth of certain microbes [Citation9]. One of these promising compounds, chlorogenic acid (CGA) exhibited high biological activity, particularly anti-inflammatory, antiviral and antimicrobial properties [Citation10].
However, the low bioavailability and instability of CGA have restricted its application [Citation11]. CGA can be absorbed in its intact form or after hydrolysis to caffeic and quinic acids in the gut [Citation12]. After oral administration, only 30% of CGA passes through the GIT membrane into the blood circulation [Citation13]. Additionally, CGA is susceptible to enzymatic degradation via polyphenol oxidase, resulting in the formation of lactones and shikimates [Citation14], in addition to its poor aqueous solubility [Citation15]. Regarding these aforementioned characteristics of CGA, an effective strategy for encapsulation within a polymeric matrix is urgently required.
Electrospraying has recently employed as a potential nano/microparticle preparation approach. Electrospraying is based on the application of an electric field to a charged liquid droplet, leaving a capillary in order to deform the interface and create a Taylor cone. The electric force resulting from the high voltage helps to overcome the droplet’s surface tension, leading to the ejection of particles [Citation16]. Higher loading efficiency (LE), narrower particle size distribution and simplicity of particle formulation via one single technical step are the key advantages of electrospraying over traditional nanoencapsulation methods [Citation17].
Polyvinyl alcohol (PVA) is a non-toxic polymer approved for use in several devices, including contact lenses and artificial organs [Citation16]. PVA is valuable for drug delivery formulations intended for alternate administration routes because of its advantages, biocompatibility and bioadhesiveness [Citation18]. Furthermore, PVA's high water solubility offers additional advantages over conventional or complex drug preparation techniques, allowing safe preparation methods that do not harm pharmaceuticals during formulation [Citation16]. Due to its biodegradability and biocompatibility, poly lactic-co-glycolic acid (PLG) has been widely utilized in multiple nanotechnology pharmaceutical applications. It has been approved by FDA [Citation19,Citation20]. Notably, few studies have investigated PVA/PLGA nano-formulations. For example, Panda et al. discussed the in vitro pharmaceutical characterization of PVA/PLGA nanofibres [Citation21].
In this study, we aimed to elucidate the phytochemical constituents of Euphorbia milii Des Moul. flowers ethanol extract, isolating one of the major phenolic acid compounds for the first time, CGA. Electrosprayed PLGA/PVA NPs, a novel nanodelivery system, were used to encapsulate CGA to overcome its physicochemical obstacles and enhance its oral bioavailability. Moreover, we investigated the antibacterial and antiviral properties of the optimized electrosprayed PLGA/PVA NPs loaded with CGA.
Results
LC–ESI-MS/MS analysis
A total of 32 compounds were tentatively identified in E. milii flower extract using LC–ESI-MS/MS in a negative mode. The main substances were several types of flavonoids and their glycosides, phenolic and carboxylic acids. The comprehensive profile is listed in . While shows the total ion chromatogram of detected metabolites in negative ion mode.
Figure 1. The total ion chromatogram of E. milii flowers extract presented the major identified metabolites (quinic acid, chlorogenic acid, quercetin-3-glucuronide, quercitrin, kaempferol-3-O-α-l-rhamnoside and naringenin according to the retention time) via LC–ESI-MS/MS in negative ion mode.
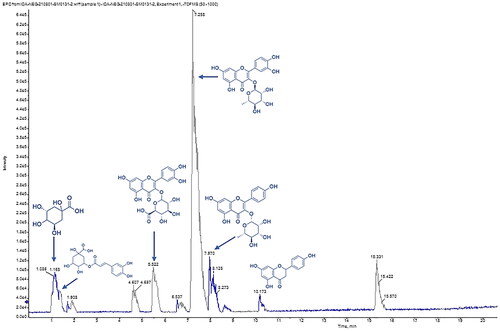
Table 1. Phytochemical profiling of E. milii by LC–MS/MS analysis (negative mode ESI).
Phytochemical elucidation of CGA
The CGA was isolated from E. milii flower extract for the first time. ESI-MS, 1H and 13C NMR data were compared with those described in the literature [Citation22,Citation23]. CGA ESI-MS m/z was 353.3 [M–H]– with a molecular formula C16H18O9. Figure S1 demonstrates the chemical structure of CGA, while 1H NMR (DMSO-d6, 400 MHz) and DEPT 13C NMR (DMSO-d6, 100 MHz) results are as follows: 1H NMR δ: 1.82 (H, t, H-2a), 2.06 (H, d, J = 13.2, H-2b), 5.08 (H, t, H-3), 3.58 (H, d, J = 9, H-4), 3.93 (H, s, H-5), 1.92 (H, d, J = 12, H-6a), 1.97 (H, d, J = 12, H-6b), 7.05 (H, s, H-2′), 6.79 (H, d, J = 8.4, H-5′), 7.01 (H, d, J = 8.4, H-6′), 7.45 (H, d, J = 15.6, H-7′), and 6.18 (H, d, J = 15.6, H-8′).
DEPT 13C NMR δ: 73.9 (C, C-1), 37.7 (CH2, C-2), 68.4 (CH, C-3), 71.4 (CH, C-4), 70.7 (CH, C-5), 36.6 (CH2, C-6), 175.4 (C, C-7), 126.0 (C, C-1′), 115.2 (CH, C-2′), 146.0 (C, C-3′), 148.8 (C, C-4′), 116.2 (CH, C-5′), 121.8 (CH, C-6′), 145.4 (CH, C-7′), 114.7 (CH, C-8′), and 166.2 (C, C-9′).
Electrosprayed PVA/PLGA NPs loaded with CGA
In vitro evaluation
summarizes the results of particle size, PDI, zeta potential and LE for CGA loaded in PVA/PLGA NPs, with representative data displayed in . Particle size increased significantly (p < .05) with increasing PLGA concentration, from 275.89 ± 27.23 to 632.54 ± 40.62 nm and % LE from 64.21 ± 4.85 to 83.45 ± 7.53. At a constant concentration of PVA (10% w/v), a higher concentration of PLGA resulted in negative surface charge values for NPs ranging from −2.89 ± 0.52 to −7.25 ± 1.69 mV as the PLGA concentration increased.
Figure 2. Representative graphs of Malvern’s Zetasizer instrument showed measurements of (A) particle size and PDI and (B) ζ-potential values of PVA/PLGA NPs displaying good quality results.
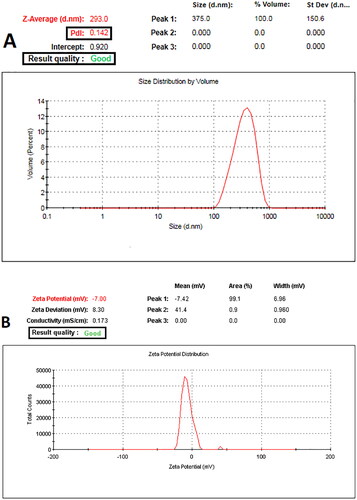
Table 2. Composition and physicochemical characterizations of electrosprayed PVA/PLGA NPs loaded with CGA.
The SEM morphological examination () revealed spherical PVA/PLGA NPs (F2) with dimensions comparable to their diameters as measured by DLS.
In vitro release study
Regarding the in vitro release study of PVA/PLGA NPs loaded with CGA (), all NP formulations exhibited a significantly higher % of cumulative release compared with free CGA (p < .05). As shown in , at four hours, the % cumulative release of F1, F2 and F3 was equal to 93.48 ± 8.21, 97.42 ± 4.72 and 87.46 ± 8.79, respectively. At the same time, the % cumulative release of free CGA was 37.53 ± 5.69 in PBS release media at pH 7.4. All NP formulations emphasize a biphasic release pattern with an initial burst after 15 min followed by a gradual four-hour release (). The initial bursts of F1, F2 and F3 were equal to 25.45 ± 2.13, 29.46 ± 4.79 and 20.46 ± 2.12, respectively, with a significant increase (p < .05) compared to the free CGA (5.78 ± 0.54). Remarkably, F1 and F2 had significantly higher (p < .05) initial burst and % cumulative release values
Fourier transform infrared spectroscopy (FTIR)
The characteristic peaks of free CGA, PLGA 50:50, PVA and PVA/PLGA NPs (F2) have been displayed in . The hydrogen bond of the dimeric form of CGA can be attributed to the broad and intense band that is located at about 3444 cm−1. At 1631 cm−1, a second strong absorption band caused by C=O stretching is seen. This band is typical of organic acids with a weak bipolar carbonyl group. Regarding the PVA spectrum, the stretching bands of OH, C=O and C–O are in 3452, 1638 and 1139 cm−1, respectively. Also, strong CH2 bending vibrational peaks at 1415 cm−1 could be observed. FTIR peaks of PLGA demonstrated in are highly correlated with a previously investigated analysis performed by our group [Citation24]. Stretching C–H bands at 2923 and 2853 cm−1 is a common denominator found in all FTIR spectra for free CGA, PLGA 50:50, PVA and PVA/PLGA NPs (F2).
Differential scanning analysis (DSA)
The effect of the electrospraying process on the crystallinity of GCA after being encapsulated into PVA/PLGA NPs was analysed using differential scanning colorimetry analysis (DSC). The endothermic peaks of PLGA 50:50 and PVA have been shown at 47.45 and 191.86 °C, respectively () as previously stated in several studies [Citation20]. CGA displayed two distinctive endothermic peaks at 199.95 and 208.99 °C [Citation25]. PVA/PLGA NPs (F2) thermogram showed the peaks of PLGA 50:50 and PVA at an approximately similar position with no peaks appearing in the range of 190–210 °C.
In vitro antibacterial activity
Before in vivo analysis, the agar well diffusion method determined the susceptibility of bacteria to free CGA, PVA/PLGA NPs loaded with CGA (F2), and blank NPs. Interestingly, free CGA and PVA/PLGA NPs loaded with CGA (F2) revealed antimicrobial potential against P. aeruginosa isolates, whereas the blank NPs did not exhibit antibacterial activity.
In vivo antibacterial assay
Bacterial burden in lung tissues
The quantity of CFU/g lung tissues is shown in . Group IV (treated with PVA/PLGA NPs loaded with CGA, F2) resulted in a significant decline (p < .05) in the quantity of CFU/g lung tissues.
Survival curve of mice
The Kaplan–Meier survival curve was constructed (). In group I, three mice died after three days, and two mice died after five days. Two mice in group II died after three days, one on the fourth day and the remaining on the fifth. Regarding group III, two mice died after four days, and one mouse died after eight days. Interestingly, group IV mice were all alive at the conclusion of the two weeks.
Histopathological studies
demonstrates the effect of free CGA and CGA-loaded PVA/PLGA NPs (F2) on the histological characteristics of the infected mice’s lung tissues. Group I displayed dilated bronchioles with evident inflammation and areas of congestion. Group II showed destructed and dilated bronchioles surrounded by chronic inflammation, alveolar fibrosis and congested vessel. Group III showed a relatively decreased inflammation with bronchioles of average size surrounded by alveoli of average size and few congested vessels. Group IV exhibited normal-sized alveoli separated by fibrous septa and normal-sized bronchioles.
Figure 9. H&E stained lung sections of (A) group I showing dilated bronchioles (red arrows) with obvious inflammation (green arrows) and areas of congestion (black arrow) (×200). (B) Group II showed destructed and dilated bronchioles (blue arrows) surrounded by chronic inflammation (black arrow), alveolar fibrosis (red arrows) and congested vessel (green arrow) (×200). (C) Group III showed partial lessening of inflammation with average-sized bronchioles (black arrow) surrounded by average-sized alveoli (blue arrows) and few congested vessels (red arrow) (×200). (D) Group IV showing alveoli with normal size separated by fibrous septa (blue arrows) and bronchioles with normal size (black arrow) (×200). The TNF-α immunostaining of (E) group I displaying strong positive TNF-α immunostaining with score 3 (×100). (F) Group II displaying strong positive TNF-α immunostaining with score 3 (×100). (G) Group III displaying moderate positive TNF-α immunostaining with score 2 (×100). (H) Group IV displaying negative TNF-α immunostaining with score 0 (×100).
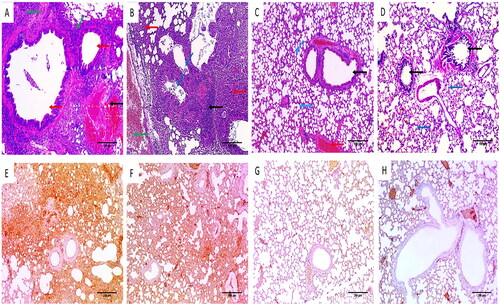
Immunohistochemical studies
The tumour necrosis factor-alpha (TNF-α) immunostaining of the lung tissues is elucidated in . Both groups I and II displayed a strong positive TNF-α immunostaining with a score of 3. Group III displayed a moderate positive TNF-α immunostaining with a score of 2. In contrast, group IV demonstrated a negative TNF-α immunostaining with a score of 0.
Antiviral activity
Cytotoxicity of the free CGA, PVA/PLGA NPs loaded with CGA, and placebo on vero-E6 cells
The values of CC50 of the free CGA, PVA/PLGA NPs loaded with CGA (F2), and blank NPs were determined using the MTT assay on vero-E6 cells. The cytotoxicity curves of the free CGA and F2 NPs are shown in . The placebo did not exhibit any toxicity on vero-E6 cells.
Antiviral activity of the free CGA, PVA/PLGA NPs loaded with CGA and placebo
The antiviral activity of the free CGA against the tested low pathogenic human coronavirus (HCoV-229E) was significantly lower (p < .05) than PVA/PLGA NPs loaded with CGA (F2), as shown in . In contrast, the blank NPs did not exhibit any antiviral activity.
Figure 11. Antiviral activity of (A) the free CGA (with IC50 of 1.8 ± 0.1 mg/mL) and (B) PVA/PLGA NPs loaded with CGA (with IC50 of 170 ± 1.1 µg/mL) against the low pathogenic human coronavirus (HCoV-229E). Antiviral activity of (C) the free CGA (with IC50 of 2.1 ± 0.09 mg/mL) and (D) PVA/PLGA NPs loaded with CGA (with IC50 of 223 ± 0.88 µg/mL) against MERS-CoV (NRCEHKU270).
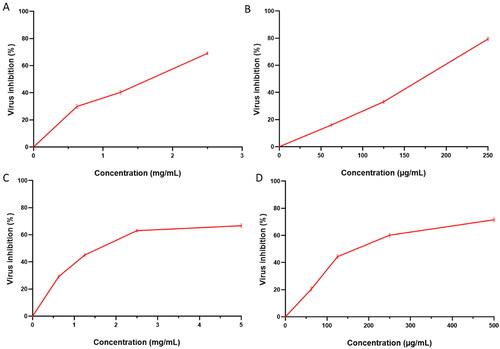
Regarding the antiviral activity against MERS-CoV (NRCEHKU270), PVA/PLGA NPs loaded with CGA (F2) exhibited an enhanced antiviral activity compared with the free CGA, as shown in .
Selectivity index (SI) of the free CGA and PVA/PLGA NPs loaded with CGA (F2) against the tested low pathogenic human coronavirus (HCoV-229E) was 2.8. Also, the SI for the free CGA and PVA/PLGA NPs loaded with CGA (F2) against MERS-CoV (NRCEHKU270) was 2.
Discussion
During the COVID-19 pandemic, there were widespread respiratory tract infections worldwide [Citation26]. Consequently, it is crucial to discover novel therapeutic approaches for treating these infections. Plants are a rich source of bioactive phytochemicals that could be identified and used to treat respiratory tract infections [Citation27]. Euphorbia (Euphorbiaceae) is the third most common genus of flowering plants [Citation28]. The Euphorbiaceae family is a well-known plant family involving different species utilized as folklore plants. Earlier studies confirmed the Euphorbia plants’ antiviral, antibacterial, antimalarial, antifungal, anti-inflammatory, anthelmintic and anticancer properties [Citation29]. Numerous studies have been conducted to highlight the phytochemical profile of various Euphorbia spp.
Prior research allowed for the identification of several groups of secondary metabolites with significant therapeutic benefits, such as polyphenolic compounds [Citation30]. Therefore, we investigated the phytochemical profile of E. milii Des Moul. flowers and isolated CGA for the first time. Then, CGA nanoparticles were prepared in a PVA/PLGA polymeric matrix using the electrospraying technique.
Regarding the optimization process during preparation of electrosprayed PVA/PLGA NPs loaded with CGA, increasing PLGA concentration from 0.05 to 0.10% w/v significantly produces NPs with higher % LE because CGA molecules prefer to remain within the PLGA matrix rather than escape into an aqueous PVA solution [Citation24]. The non-significant increase (p > .05) in % LE in F3 (PLGA concentration of 0.15% w/v) compared to F2 is attributable to poor uniformity of electrosprayed dispersion, which also negatively affects PDI value (0.295, 0.082) with larger particle size (632.54, 40 nm) [Citation20]. PVA/PLGA nanoparticles with a higher PLGA concentration have more negative charges, which increase the negative zeta potential values of CGA loaded into PVA/PLGA NPs ().
Fabrication of CGA into PVA/PLGA NPs produced formulations with a smaller particle size and a higher surface area leading to significant enhancement of CGA release from its nano formula rather than free unprocessed CGA. Generally, the superior wettability of PVA could enhance the solubility and release of CGA. The addition of PLGA would provide a sustained release profile of CGA NPs [Citation21]. The F3 has the largest particle size (632.54 ± 40.62 nm), which negatively affected the release behaviour resulting in a significant (p < .05) decrease in the initial burst (20.46 ± 2.12) and % cumulative release (87.46 ± 8.79) values. The initial burst of CGA-loaded PVA/PLGA NPs was attributed to the presence of free drug molecules deposited on the external surface of NPs, followed by the gradual erosion of the polymeric network that caused the prolonged release of CGA over four hours [Citation16]. Furthermore, F2 was chosen as the optimum formula for further studies of the antibacterial and antiviral activities due to the significantly higher (p < .05) values of % of LE (80.23 ± 5.74), initial burst (29.46 ± 4.79) and % cumulative release (97.42 ± 4.72). Additionally, F2 has an adequate particle size (454.36 ± 36.74 nm) with an adequate surface charge (–4.56 ± 0.84 mV) that provides stable NPs with minimal aggregation risk.
Generally, there is no considerable shift in the FTIR bands for all the components of NPs after the electrospraying process. The distinguishing peaks of PVA, PLGA and CGA have been clearly displayed in the same position with a probably higher intensity indicating no risk of a chemical interaction occurring between the individual component of PVA/PLGA NPs loaded with CGA [Citation20]. DSC thermogram confirms the presence of CGA in its amorphous state as it has been prepared in a molecular form during the preparation of electrosprayed solution.
P. aeruginosa pathogenic bacteria are among the most common bacteria which can cause respiratory tract infections [Citation31]. Using the agar well diffusion method, we investigated the in vitro antibacterial activity of free CGA, PVA/PLGA NPs loaded with CGA and blank NPs. The free CGA and PVA/PLGA NPs loaded with CGA demonstrated antimicrobial activity against P. aeruginosa isolates, whereas the blank NPs exhibited no antibacterial activity as they did not contain any active constituents. Agar well diffusion technique is commonly utilized to assess the antimicrobial potential of microbial or plant extracts by exploring the appearance of inhibition zones around the performed wells [Citation32]. Then, the in vivo antibacterial activity of the free CGA and PVA/PLGA NPs loaded with CGA was investigated using a lung infection model in mice. PVA/PLGA NPs loaded with CGA exhibited a substantial decrease in the CFU/g lung tissues (p < .05) and improved the survival of the tested mice. The superior antibacterial activity of electrosprayed PVA/PLGA NPs loaded with CGA might be attributed to the efficient penetration power of the nanosystem to extravagate the cellular membrane of P. aeruginosa bacteria.
Common methods for studying bacterial lung infections include histopathology and immunostaining of infected lung tissues [Citation33]. In this study, PVA/PLGA NPs loaded with CGA (F2) restored the normal-sized alveoli and bronchioles while reducing inflammation and congestion induced by the bacterial infection. These effects can be attributed to the enhanced activity of CGA by the electrospraying technique. Regarding the TNF-α immunostaining, PVA/PLGA NPs loaded with CGA resulted in a negative TNF- α immunostaining of lung tissues with a score of zero. TNF-α is considered a primary regulator of inflammatory reactions and is involved in the pathogenesis of numerous infectious and inflammatory diseases [Citation34].
The in vitro antiviral activity of the free CGA, PVA/PLGA NPs loaded with CGA, and blank NPs was investigated against the low pathogenic human coronavirus (HCoV-229E) and MERS-CoV (NRCEHKU270) using plaque assay. Plaque assay is a commonly used quantitative method for determining the number of infectious viruses [Citation35] by determination of the number of the formed plaques in the cell culture following infection with the studied virus [Citation36]. In the current study, PVA/PLGA NPs loaded with CGA had significantly lower (p < .05) values of the half maximal inhibitory concentration (IC50) against the low pathogenic human coronavirus (HCoV-229E) and MERS-CoV (NRCEHKU270). The value of IC50 represents the drug concentration needed to achieve an in vitro 50% inhibition. Therefore, lower values of IC50 indicate more potent antiviral activity [Citation36]. A future study is needed to investigate the effectiveness of the formula against the tested viruses in vivo.
Materials and methods
General chemicals and materials
Silica gel F254 utilized the purification method of column chromatography (CC). (Merck, Kenilworth, NJ, 70–230 mesh). The structure of the active compound was determined by spectroscopic methods (Supplementary data).
Plant material and isolation of CGA
Euphorbia milii Des Moul. flowers were collected from a local garden in Gharbia Governorate in March 2021. The plant identification and preparation are illustrated in Supplementary data.
LC–ESI-MS/MS metabolomics analysis
Extract of E. milii flowers was analysed in the Proteomics and Metabolomics Unit, Children’s Cancer Hospital (57357), adopting procedures previously reported by Attallah et al. [Citation37].
Preparation and optimization of electrosprayed PVA/PLGA NPs
Aqueous PVA solution (10% w/v) was prepared after stirring for two hours at 85 °C to fasten PVA's solubility, followed by cooling for one hour. Various concentrations of PLGA (0.05, 0.10 and 0.15% w/v) were dissolved in DMF (1 mL) after the addition of CGA (0.1% w/v) [Citation38] and then mixed with an aqueous PVA solution (9 mL) on a magnetic stirrer for half an hour to ensure homogeneity [Citation21] (formula coding and composition shown in ). The electrospraying procedure was performed using NANON-01B, Mecco, Ltd. (Fukuoka, Japan). The controlling process parameters were carefully optimized during electrospraying. The applied voltage, feed rate and the distance between the collector and needle tip were adjusted to be 28 kV, 0.1 mL/h and 9 cm, respectively [Citation39].
In vitro investigations of the electrosprayed PVA/PLGA NPs (Supplementary data)
Measurement of particle diameter, polydispersity index (PDI) and surface charge (Supplementary data).
Determination of electrosprayed PVA/PLGA NPs LE (Supplementary data).
Morphological imaging using the scanning electron microscope (SEM) (Supplementary data).
In vitro release study (Supplementary data).
Analysis of drug–polymer interaction
FTIR
The spectral bands of free CGA, PLGA 50:50, PVA and PVA/PLGA NPs (F2) have been detected using FTIR spectrophotometer (Bruker Tensor 27, Mannheim, Germany) in the range of 4000–400 cm−1 after mixing with KBr and compressed into small disks [Citation40].
Differential scanning colorimetry analysis
The endothermic peaks of free CGA, PLGA 50:50, PVA and PVA/PLGA NPs (F2) were investigated using a differential scanning calorimeter (PerkinElmer, Inc., STA 6000, Waltham, MA) at a purge rate of N2 and heating rate equal to 40 mL/min and 10 °C/min, respectively [Citation24].
In vitro antibacterial activity
See Supplementary data.
In vivo antibacterial potential
Animals
Forty male Swiss albino mice weighing 19–25 g were utilized for in vivo antibacterial assay. The study procedures followed the ethical guidelines of the Faculty of Pharmacy Research Ethical Committee, Tanta University, and the study was accredited with an approval number of TP/RE/08/22P-0030.
Experiment
Using a lung infection model, the in vivo antibacterial potential of free CGA and PVA/PLGA NPs loaded with CGA (F2) was investigated [Citation41].
The lung infection was induced using the bacterial suspension (×107 CFU/mL) of isolate number 15 by putting 25 µL of the suspension in the nares of mice. The animals were then allocated into four groups (each containing 10 mice):
Group I (the control group): did not receive any treatment.
Group II received blank PVA/PLGA NPs.
Group III administered free CGA.
Group IV administered PVA/PLGA NPs loaded with CGA (F2).
Treatments were administered orally after 4, 24 and 48 h. The concentration of CGA was 50 mg/kg.
After 48 h, five mice were anaesthetized from each group and euthanized. Lungs were dissected for histological and immunohistochemical studies. Furthermore, the bacterial burden was determined by homogenization of the lungs in phosphate-buffered saline, and then the resulting suspension was serially diluted and plated into Mueller–Hinton agar. The plates were incubated overnight, and the obtained colonies were counted. The remaining mice were left for two weeks to calculate the survival rate [Citation42].
Histology and immunohistochemical studies
Lungs were stained with haematoxylin and eosin (H&E) stain [Citation20], as shown in the supplementary data. Immunostaining was performed using a polyclonal antibody of TNF-α (Invitrogen, Carlsbad, CA, PA1-0281), as presented in the supplementary data [Citation43,Citation44].
Antiviral activity
Viruses and cells
See Supplementary data.
MTT cytotoxicity test
This test was conducted to determine the half-maximal cytotoxic concentration (CC50) of free CGA, PVA/PLGA NPs loaded with CGA (F2), and blank PVA/PLGA NPs using MTT, as previously described in [Citation45,Citation46] (Supplementary data).
Plaque assay
The plaque assay was performed to determine the antiviral activity of free CGA, PVA/PLGA NPs loaded with CGA (F2) and blank PVA/PLGA NPs (Supplementary data) as previously described [Citation45,Citation47]. The SI was calculated according to the following formula:
Statistics
See Supplementary data.
Conclusions
In the current work, the phytoconstituents of Euphorbia milii Des Moul. flowers ethanol extract were elucidated using LC–MS/MS. Overall, 32 compounds were identified. In addition, CGA was specifically isolated and characterized from this species for the first time. Fabrication of CGA into an optimum PVA/PLGA nanodelivery background via electrospraying technique could be considered a promising strategy showing a substantial improvement of CGA antibacterial action on P. aeruginosa bacteria and antiviral activity against MERS-CoV and the low pathogenic human coronavirus (HCoV-229E). These findings might contribute to future research to investigate the effectiveness of CGA PVA/PLGA NPs against various other species of pathogenic bacteria and viruses.
Author contributions
Conceptualization: Dalia Abdelkader, Walaa Negm and Engy Elekhnawy. Data curation and formal analysis: Asmaa Saleh, Thanaa A El-Masry, Duaa Eliwa and Badriyah Alotaibi. Funding acquisition: Badriyah Alotaibi. Investigation, methodology, writing – original draft: review and editing: Dalia Abdelkader, Duaa Eliwa, Walaa Negm and Engy Elekhnawy.
Supplemental Material
Download MS Word (90.2 KB)Acknowledgements
This research was funded by the Deanship of Scientific Research at Princess Nourah bint Abdulrahman University, Riyadh, Saudi Arabia, through the Research Groups Program Grant No. RGP-1443-0044. Therefore, the authors gratefully acknowledge the DSR's technical and financial support.
Disclosure statement
The authors declare no potential conflict of interest.
Data availability statement
The data supporting the findings of this study are available within the article (and/or) its supplementary materials.
Additional information
Funding
References
- Troeger C, Blacker B, Khalil IA, et al. Estimates of the global, regional, and national morbidity, mortality, and aetiologies of lower respiratory infections in 195 countries, 1990–2016: a systematic analysis for the global burden of disease study 2016. Lancet Infect Dis. 2018;18(11):1191–1210.
- Olaimat AN, Aolymat I, Shahbaz HM, et al. Knowledge and information sources about COVID-19 among university students in Jordan: a cross-sectional study. Front Public Health. 2020;8:254.
- Pattemore PK, Jennings LC. Epidemiology of respiratory infections. Pediatr Respir Med. 2008;435.
- Endres A, Hügel C, Boland H, et al. Pseudomonas aeruginosa affects airway epithelial response and barrier function during rhinovirus infection. Front Cell Infect Microbiol. 2022;12:143.
- Bayes H, Ritchie N, Irvine S, et al. A murine model of early Pseudomonas aeruginosa lung disease with transition to chronic infection. Sci Rep. 2016;6(1):10.
- Sharma A, Tiwari S, Deb MK, et al. Severe acute respiratory syndrome coronavirus-2 (SARS-CoV-2): a global pandemic and treatment strategies. Int J Antimicrob Agents. 2020;56(2):106054.
- Jamshidi-Kia F, Lorigooini Z, Amini-Khoei H. Medicinal plants: past history and future perspective. J Herbmed Pharmacol. 2018;7(1):1–7.
- Chassagne F, Samarakoon T, Porras G, et al. A systematic review of plants with antibacterial activities: a taxonomic and phylogenetic perspective. Front Pharmacol. 2021;11:2069.
- Alibi S, Crespo D, Navas J. Plant-derivatives small molecules with antibacterial activity. Antibiotics. 2021;10(3):231.
- Liu D, Meng S, Xiang Z, et al. Antimicrobial mechanism of reaction products of Morus notabilis (mulberry) polyphenol oxidases and chlorogenic acid. Phytochemistry. 2019;163:1–10.
- Shi G, Rao L, Yu H, et al. Yeast-cell-based microencapsulation of chlorogenic acid as a water-soluble antioxidant. J Food Eng. 2007;80(4):1060–1067.
- Nallamuthu I, Devi A, Khanum F. Chlorogenic acid loaded chitosan nanoparticles with sustained release property, retained antioxidant activity and enhanced bioavailability. Asian J Pharm Sci. 2015;10(3):203–211.
- Olthof MR, Hollman PC, Katan MB. Chlorogenic acid and caffeic acid are absorbed in humans. J Nutr. 2001;131(1):66–71.
- Jaiswal R, Matei MF, Subedi P, et al. Does roasted coffee contain chlorogenic acid lactones or/and cinnamoylshikimate esters? Food Res Int. 2014;61:214–227.
- Rui L, Xie M, Hu B, et al. Enhanced solubility and antioxidant activity of chlorogenic acid–chitosan conjugates due to the conjugation of chitosan with chlorogenic acid. Carbohydr Polym. 2017;170:206–216.
- Felice B, Prabhakaran MP, Zamani M, et al. Electrosprayed poly(vinyl alcohol) particles: preparation and evaluation of their drug release profile. Polym Int. 2015;64(12):1722–1732.
- Jaworek A, Sobczyk AT. Electrospraying route to nanotechnology: an overview. J Electrostat. 2008;66(3–4):197–219.
- Abdelkader D, Osman M, El-Gizawy S, et al. Characterisation and in vitro stability of low-dose, lidocaine-loaded poly(vinyl alcohol)-tetrahydroxyborate hydrogels. Int J Pharm. 2016;500(1–2):326–335.
- Alotaibi B, El-Masry TA, Elekhnawy E, et al. Aqueous core epigallocatechin gallate PLGA nanocapsules: characterization, antibacterial activity against uropathogens, and in vivo reno-protective effect in cisplatin induced nephrotoxicity. Drug Deliv. 2022;29(1):1848–1862.
- Almukainzi M, El-Masry TA, Negm WA, et al. Gentiopicroside PLGA nanospheres: fabrication, in vitro characterization, antimicrobial action, and in vivo effect for enhancing wound healing in diabetic rats. Int J Nanomedicine. 2022;17:1203–1225.
- Panda BP, Wei MX, Shivashekaregowda NKH, et al. Design, fabrication and characterization of PVA/PLGA electrospun nanofibers carriers for improvement of drug delivery of gliclazide in type-2 diabetes. Multidisc Digit Publ Inst Proc. 2020;78:14.
- Suarez-Quiroz ML, Campos AA, Alfaro GV, et al. Isolation of green coffee chlorogenic acids using activated carbon. J Food Compos Anal. 2014;33(1):55–58.
- Hernandez CE, Chen H-H, Chang C-I, et al. Direct lipase-catalyzed lipophilization of chlorogenic acid from coffee pulp in supercritical carbon dioxide. Ind Crops Prod. 2009;30(3):359–365.
- Abdelkader DH, Abosalha AK, Khattab MA, et al. A novel sustained anti-inflammatory effect of atorvastatin—calcium PLGA nanoparticles: in vitro optimization and in vivo evaluation. Pharmaceutics. 2021;13(10):1658.
- Owusu-Ware SK, Chowdhry BZ, Leharne SA, et al. Thermal decomposition of chlorogenic acid in different atmospheres. J Pharm Pharmacol. 2010;62:1357–1358.
- Subbarao K, Mahanty S. Respiratory virus infections: understanding COVID-19. Immunity. 2020;52(6):905–909.
- Nallusamy S, Mannu J, Ravikumar C, et al. Exploring phytochemicals of traditional medicinal plants exhibiting inhibitory activity against main protease, spike glycoprotein, RNA-dependent RNA polymerase and non-structural proteins of SARS-CoV-2 through virtual screening. Front Pharmacol. 2021;12:1704.
- Smeriglio A, Denaro M, Trombetta D, et al. New insights on Euphorbia dendroides L. (Euphorbiaceae): polyphenol profile and biological properties of hydroalcoholic extracts from aerial parts. Plants. 2021;10(8):1621.
- Perera SD, Jayawardena UA, Jayasinghe CD. Potential use of Euphorbia hirta for dengue: a systematic review of scientific evidence. J Trop Med. 2018;2018:1–7.
- Magozwi DK, Dinala M, Mokwana N, et al. Flavonoids from the genus Euphorbia: isolation, structure, pharmacological activities and structure–activity relationships. Pharmaceuticals. 2021;14(5):428.
- Jurado-Martín I, Sainz-Mejías M, McClean S. Pseudomonas aeruginosa: an audacious pathogen with an adaptable arsenal of virulence factors. Int J Mol Sci. 2021;22(6):3128.
- Balouiri M, Sadiki M, Ibnsouda SK. Methods for in vitro evaluating antimicrobial activity: a review. J Pharm Anal. 2016;6(2):71–79.
- Meyerholz DK, Beck AP. Histopathologic evaluation and scoring of viral lung infection. Methods Mol Biol. 2020;2099:205–220.
- Jang D-I, Lee A-H, Shin H-Y, et al. The role of tumor necrosis factor alpha (TNF-α) in autoimmune disease and current TNF-α inhibitors in therapeutics. Int J Mol Sci. 2021;22(5):2719.
- Ács N, Gambino M, Brøndsted L. Bacteriophage enumeration and detection methods. Front Microbiol. 2020;11:2662.
- Mendoza EJ, Manguiat K, Wood H, et al. Two detailed plaque assay protocols for the quantification of infectious SARS-CoV-2. Curr Protoc Microbiol. 2020;57(1):cpmc105.
- Attallah NG, El-Sherbeni SA, El-Kadem AH, et al. Elucidation of the metabolite profile of Yucca gigantea and assessment of its cytotoxic, antimicrobial, and anti-inflammatory activities. Molecules. 2022;27(4):1329.
- Sharaf NS, Shetta A, Elhalawani JE, et al. Applying Box–Behnken design for formulation and optimization of PLGA-coffee nanoparticles and detecting enhanced antioxidant and anticancer activities. Polymers. 2021;14(1):144.
- Almukainzi M, El-Masry TA, Negm WA, et al. Co-delivery of gentiopicroside and thymoquinone using electrospun m-PEG/PVP nanofibers: in-vitro and in vivo studies for antibacterial wound dressing in diabetic rats. Int J Pharm. 2022;625:122106.
- Abdelkader DH, Negm WA, Elekhnawy E, et al. Zinc oxide nanoparticles as potential delivery carrier: green synthesis by Aspergillus niger endophytic fungus, characterization, and in vitro/in vivo antibacterial activity. Pharmaceuticals. 2022;15(9):1057.
- van Hoek ML, Kaushal A, Bishop BM, et al. Intraperitoneal treatment with antimicrobial peptide rescues mice from a pulmonary Francisella infection. bioRxiv; 2019. p. 603233.
- Binsuwaidan R, Sultan AA, Negm WA, et al. Bilosomes as nanoplatform for oral delivery and modulated in vivo antimicrobial activity of lycopene. Pharmaceuticals. 2022;15(9):1043.
- Abdelkader DH, Elekhnawy E, Negm WA, et al. Insight into fucoidan-based PEGylated PLGA nanoparticles encapsulating methyl anthranilic acid: in vitro evaluation and in vivo anti-inflammatory study. Mar Drugs. 2022;20(11):694.
- Miotto A, Lins TA, Montero E, et al. Immunohistochemical analysis of the COX-2 marker in acute pulmonary injury in rats. Ital J Anat Embryol. 2009;114(4):193–199.
- Attallah NG, El-Kadem AH, Negm WA, et al. Promising antiviral activity of Agrimonia pilosa phytochemicals against severe acute respiratory syndrome coronavirus 2 supported with in vivo mice study. Pharmaceuticals. 2021;14(12):1313.
- Alotaibi B, Negm WA, Elekhnawy E, et al. Antibacterial, immunomodulatory, and lung protective effects of Boswellia dalzielii oleoresin ethanol extract in pulmonary diseases: in vitro and in vivo studies. Antibiotics. 2021;10(12):1444.
- Hegazy A, Mostafa I, Elshaier YA, et al. Robust antiviral activity of santonica flower extract (Artemisia cina) against avian and human influenza A viruses: in vitro and chemoinformatic studies. ACS Omega. 2022;7(45):41212–41223.