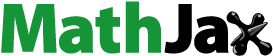
Abstract
The biogenic manufacture of nanoparticles utilising endophytic fungus is an eco-friendly, cost-effective, and secure alternative to constructing chemical methods. The prime focus of the study was to fabricate ZnONPs using the biomass filtrate of endophytic Xylaria arbuscula isolated from Blumea axillaris Linn. and to evaluate their biological properties. The characterisation of the biosynthesized ZnO-NPs was done utilising both spectroscopic and microscopic methods. The bioinspired NPs showed a surface plasmon peak at 370 nm; SEM and TEM micrographs illustrated the hexagonal organisation; XRD spectra proved the crystalline phase as hexagonal wurtzite; EDX analysis confirmed the presence of zinc and oxygen atoms; and the zeta potential analysis proved the stability of ZnONPs. In addition, they also demonstrated significant concentration-dependent inhibition of antimicrobial, antioxidant, anti-inflammatory, and antidiabetic potential in comparison with the reference drugs. In vitro cytotoxicity and wound healing potential of ZnONPs were examined in L929 cell lines, illustrating that they accelerated the wound healing process by roughly 95.37 ± 1.12% after a 24-h exposure to ZnONPs. The photocatalytic activity of the ZnONPs was examined by degrading the methylene blue dye under solar irradiation. In conclusion, our outcomes showed that mycosynthesized ZnONPs possessed potent bioactivity and could be an excellent choice for biomedical applications.
Introduction
One of the most active and rapidly expanding research fields in contemporary material science is the field of nanoscience and nanotechnology, which focuses on designing novel compounds at the nanoscale (1–100 nm) that can be deployed in a variety of applications [Citation1,Citation2]. Nanoparticles (NPs) are atom clusters with unique properties such as surface morphology, quantum size, crystal structure, charge, shape, optical effect, distribution, zeta potential, and thermal conductivity, which facilitate their integration into biotechnological and biomedical domains [Citation1–4]. A crucial aspect of nanotechnology is the fabrication of nanoparticles, which is generally accomplished through an array of physical, chemical, and biological approaches [Citation5]. For physical or chemical methodologies, extreme circumstances, including large quantities of noxious chemicals, high temperature, high pressure, and energy, are required and have an impact on the environment, resulting in the production of NPs with minimal stability. Therefore, it is vital to investigate NPs fabrication procedures that are secure, inexpensive, clean, and readily scaleable. As a result, interest has grown in biological methods or green nanotechnology that employ harmless chemicals and gentle procedures [Citation3]. “Green nanotechnology” is a term that refers to a method for fabricating nanomaterials that is ecologically beneficial by eliminating or minimising the usage of hazardous materials [Citation6]. The biological synthesis of NPs involving green chemistry, an ecofriendly-based approach, makes use of the active compounds of several biological entities such as plants, fungi, actinomycetes, yeast, bacteria, algae, and cyanobacteria [Citation7]. Among these microbe-mediated synthesis of NPs serves to be an innovative method with a wide range of applications in the pharmaceutical and agricultural sectors. This method has also added the benefit of extending the life of NPs because of the physio-chemical characteristics of microbe-based NPs, which overcome the drawbacks of traditional chemical and physical approaches [Citation5].
Investigations into the production of various metal NPs including copper, silver, zinc, gold, magnesium, titanium, and alginate, have been carried out using a variety of microorganisms, particularly bacteria and fungi. Among them, fungi might be employed as the most effective biotechnological agent for the eco-friendly synthesis of nanoparticles [Citation8]. Fungi are currently considered a good addition to the list of microorganisms utilised for the amalgamation of NPs due to their simplicity of scaling up, flexibility, biomass processing, easy maintenance, downstream handling, high efficiencies of fungal metabolites, extracellular redox proteins, and enzyme production, tolerance to higher metal concentrations, the presence of mycelia presenting an enlarged surface area, and economic viability [Citation7,Citation9–11]. In specific, endophytic fungi are more appropriate for the synthesis of NPs since they are capable of producing huge quantities of biomass that can withstand flow pressure and agitation, are fastidious in their growth, secrete large amounts of enzymes, and are simple to handle in downstream processing, making it simple to fabricate NPs that can be used immediately in a variety of applications [Citation12]. Endophytes are a group of unexplored microorganisms residing within healthy plant tissues and having an array of interactions with their host plants. These endophytic organisms possess several host-distinctive traits and have several potentials for synthesising antibacterial, antiviral, antioxidant, insulin-mimicking, and immunosuppressive activities [Citation12,Citation13]. Bioactive constituents synthesised by living machinery have displayed significant potential in the therapy of several diseases, including heart disease, diabetes, cancer, and infectious diseases [Citation14].
Zinc is one of the most vital nutrients for both humans and animals and plays a crucial role in DNA replication, DNA repair, oxidative stress, and cell cycle progression. When compared to the traditional sources of zinc, ZnONPs showed much lower toxicity, a higher rate of absorption, greater bioavailability, immune system enhancement, antioxidant defence, superior biocompatibility, and acting as potent antimicrobial agents [Citation15,Citation16]. ZnONPs have gained widespread attention among the metal oxide NPs due to their unique characteristics including their electro-optical and chemical properties that can be changed by turned morphology of these NPs beside UV-protection, electrical conductivity, and photocatalytic activity, which hence increase their applicability aspects [Citation1,Citation17]. NPs are often employed in biomedicine due to their favourable interactions with biological membranes, nucleic acids, protein acids, and receptors owing to their small dimensions [Citation18]. ZnONPs are an essential candidate with a broad range of biomedical applications, including drug delivery, medical diagnosis, antibacterial, anti-inflammation. anticancer, wound healing properties, and new activities such as bioimaging, diabetic therapy, anticoagulant, and thrombolytic potential [Citation3, Citation19]. Many researchers have synthesised ZnONPs from endophytic fungi such as Aspergillus niger [Citation3], Trichoderma viride [Citation20], Xylaria acuta [Citation21], Alternaria tenuissima [Citation22], Cochliobolus geniculatus [Citation23], and Aspergillus terreus [Citation24]. Several reports suggest that biogenic synthesis of ZnONPs using endophytic fungi displays superior antimicrobial [Citation20,Citation25], antioxidant [Citation20,Citation22], anticancer [Citation22] activities, and photocatalytic dye degradation efficiency [Citation22,Citation25]. ZnONPs are a desirable option since they are stable, biocompatible, and non-toxic at a minimum concentration and are generally recognised as safe by the US Food and Drug Administration [Citation19].
Presently, green synthesis of NPs is in high demand due to its extensive application in biomedicine, eco-friendliness, and cost-effectiveness. Therefore, the study was aimed at investigating the endophytic fungi-mediated fabrication of ZnONPs utilising Xylaria arbuscula (MN904863) isolated from Blumea axillaris Linn. as a capping agent and zinc acetate (Zn (CH3COO)2 2H2O) as a precursor salt. To the best of our knowledge, this is the first report that attempts to figure out the potent biological activities of ZnONPs fabricated employing the endophytic fungal extracts of Xylaria arbuscula. Characterising the mycogenically synthesised ZnONPs using UV–Vis spectroscopy, FT-IR, SEM, XRD, TEM, DLS, EDX, and Zeta Potential is another crucial objective. Finally, biofabricated nanoparticles were evaluated for their potential biological activities, including antimicrobial activity against pathogenic bacteria and fungi, as well as their in vitro antidiabetic, anti-inflammatory, cytotoxic, and wound healing efficacy for their myriad applications in biomedicine.
Materials and methods
Zinc acetate (Zn (CH3COO)2 2H2O), Malt extract broth, Muller Hinton broth, potato dextrose broth, 2,2-diphenylpicrylhydrazyl (DPPH), Bovine serum albumin, Methylene blue, MTT [3-(4,5-Dimethylthiazol-2-yl)-2-5-diphenyltetrazolium bromide], Ethidium bromide, Agar agar, were purchased from Hi-media, Mumbai, India. The clinical pathogens, including bacteria and fungi, were maintained in the Biopharmacy Lab, Department of Microbial Biotechnology, Bharathiar University, Tamil Nadu, India. The L929 cell line was obtained from NCCS (National Centre for Cell Sciences), Pune, India.
Endophytic fungi
The endophytic fungus Xylaria arbuscula was isolated from Blumea axillaris collected from the Valparai Hills region of Coimbatore, Tamil Nadu, India (10.37°N, 76.97°E) [Citation26].
Fungal extract preparation for ZnO-NPs synthesis
Xylaria arbuscula was inoculated in a 500 ml Erlenmeyer flask containing 300 ml of malt extract broth medium and incubated at 26 ± 2 °C in a rotary shaker (150 rpm) for 5 days. After fermentation, the fungal biomass was harvested, filtered using Whatman filter paper No.1, and washed with sterile double-distilled water to remove any residual media components in order to prevent contamination. Then 15 g of harvested wet fungal biomass were resuspended in 100 ml of sterile distilled water and incubated in a rotary shaker (150 rpm) at 26 ± 2 °C for 24 h. The suspension was then filtered using Whatman filter paper No.1 to obtain the cell-free supernatant and centrifuged for 15 min at 10,000 rpm to remove any cellular debris. The obtained cell-free filtrates were then used for the biosynthesis of ZnONPs. To fabricate ZnONPs, 100 ml of mycelium-free filtrate was mixed with zinc acetate (Zn (CH3COO)2 2H2O) as a precursor salt solution at a concentration of 1 mM and then incubated in a rotary shaker (150 rpm) at 28 °C and pH 9 for 72 h. The formation of ZnONPs through fungal extract-mediated synthesis was confirmed by observing the development of a creamy white precipitate in the reaction mixture. The precipitates were then collected and dried at 150 °C to obtain the white powder of ZnONPs [Citation27].
Characterisation of ZnO-NPs
The ZnONPs fabricated using endophytic fungal extract were characterised using various spectroscopic and microscopic techniques. To determine their optical properties, the UV-Vis spectrum of ZnONPs was measured using a Jasco V-750 double-beam UV-Vis spectrophotometer at a wavelength range of 300-600 nm. For determining the capping agents present on the surface of ZnONPs, the synthesised nanoparticle powder was mixed with potassium bromide to make KBr pellets, which were examined using JASCO FT/IR-4600 with a wavenumber range of 400–4000cm−1 at a resolution of 1 cm−1. The crystalline phase of the bioinspired ZnO NPs was detected using an X-Ray diffractometer (Rigaku-ULTIMA-IV), supplied with a Cu Kα. The size and external surface geometry of the biosynthesized nanoparticles were examined using Transmission electron microscopy (Zeiss-EM10C) and Scanning electron microscopy (Cam Scan MV2300) respectively. Energy-dispersive X-ray spectroscopy was used to detect the elemental compositions of the biofabricated nanoparticles. The average size and zeta potential of the nanoparticles were determined by measuring the dynamic fluctuations of light scattering intensity (Zetasizer Nano ZS, Malvern instruments Ltd, UK).
Biological applications of biogenically synthesised ZnO-NPs
Antibacterial activity
The agar-disc diffusion assay was used to examine the antibacterial potential of bioinspired ZnONPs. The antibacterial action was studied using eight different types of bacteria: Staphylococcus aureus, Escherichia coli, Enterococcus faecalis, Pseudomonas aeruginosa, Proteus mirabilis, Staphylococcus epidermidis, Klebsiella pneumoniae, and Bacillus subtilis. 0.5 OD McFarland bacterial suspensions were prepared, and by using a sterile cotton swab, a homogeneous bacterial lawn was made on the freshly prepared Muller-Hinton agar plates. Whatman filter paper was cut into 6-mm discs and sterilised. Then, 50 µL of varied concentrations (25, 50, 75, 100 µg/mL) of ZnONPs were spotted on the sterile disc under aseptic conditions and placed on the surface of the agar medium. Gentamicin was used as a positive control, while DMSO served as a negative control. After 24 h of incubation at 37 °C, the potential of ZnONPs was determined by measuring the inhibitory zones (in mm). The experiments were repeated three times, and the mean inhibition zones were recorded for each trial [Citation28].
Antifungal activity
The antifungal potency of the bioinspired ZnONPs against Candida albicans and Aspergillus niger, was determined by disc diffusion assay. A 50 µL aliquot of the spore suspension of every fungal strain was disseminated on the SDA (Sabouraud dextrose agar) medium, and a homogenous lawn was created using the spread plate method. Different concentrations (25, 50,75,100 µg/mL) of ZnONPs were spotted on the sterile filter discs and placed on the surface of the solidified agar medium under aseptic conditions. The plates were incubated at 28 °C for 48 h and following incubation, each disc loaded with the sample was observed for the appearance of the zone of inhibition (mm). Amphotericin B and DMSO were employed as positive and negative controls, respectively. The tests were performed in triplicate, and the mean inhibition zones were measured for each repetition [Citation29].
Antioxidant activity (DPPH assay)
To investigate whether ZnONPs might be effective to scavenge free radicals, the standard DPPH assay was performed in multiple concentrations ranging from 10 µg/mL to 500 µg/mL. Briefly, 10 µL of varied concentrations of the test sample was mixed with 190 µL of DPPH reagent in a 96-well plate and incubated in the dark at 37 °C for 60 min. Ascorbic acid served as a positive control, while methanol was employed as a negative control. After the incubation period, the absorbance of the samples was recorded at 517 nm using a microplate reader. The % scavenging activity of the DPPH free radical was calculated by
(1)
(1)
where Abs control isthe absorbance of the control (DPPH solution without sample), and Abs sample is the absorbance of the ZnONPs (DPPH solution plus test sample). Additionally, the concentration at which free radicals are significantly reduced by 50% was measured (IC50) [Citation30].
In vitro antidiabetic activity
The anti-diabetic potential of biogenic ZnONPs was assessed using α-amylase inhibition bioassay as described by Hussain et al. [Citation31] with few modifications. Briefly, an aliquot of 10 µL of multiple concentrations of fabricated ZnONPs in 96-well microtiter plate was mixed with 25 µL of α-amylase (1 U/mL) solution and 15 µL of PBS, followed by the addition of 40 µL of starch solution, and then the reaction mixture was incubated for 30 min at 50 °C. Following incubation, 20 µL of 1 M hydrochloric acid and 90 µL of iodine solution were added to the wells, and the absorbance was recorded at 450 nm. Acarbose was employed as the standard drug, and the reaction was performed in triplicates. The percentage inhibition was determined using the following algorithm:
(2)
(2)
where: A control - the absorbance of the control solution (no sample); A sample - the absorbance of the sample solution.
In vitro anti-inflammatory activity
The anti-inflammatory efficacy of the mycosynthesized ZnONPs was evaluated using an in vitro albumin denaturation assay according to Sharifi-Rad et al. [Citation32] with minor modifications. Briefly, 200 µL of bovine serum albumin was mixed with 2 ml of the multiple concentrations of ZnONPs (10, 50, 100, 250, 500 μg/ml) and 2.8 ml of Phosphate buffered saline (pH 6.4). The resulting mixture was incubated in a water bath for 15 min at 37 °C and then heated for 5 min at 70 °C. The absorbance of the reaction mixture was recorded at 660 nm after being cooled to room temperature. Diclofenac sodium has been employed as a reference drug, and the experiment was performed in a similar manner. The following algorithm was used to figure out the per cent inhibition for protein denaturation:
(3)
(3)
In vitro cytotoxic activity against L929 cell line
The cytotoxic effect of the biogenically synthesised ZnONPs was examined in L929 cell lines using an MTT assay according to the modified protocol of Faisal et al. 2021 [Citation33]. L929 cells were cultured in Dulbecco’s Modified Eagle Medium (DMEM) supplemented with 10% foetal bovine serum and incubated at 37 °C in a 5% humidified CO2 atmosphere. For this assay, a concentration of 1 × 105 cells per well was cultivated in sterile 96-well microtitre plates at 37 °C for 24 h in 5% CO2. Following incubation, the cells were treated with varying concentrations of synthesised ZnONPs, and the plates were incubated for 24 h to examine the cytotoxic efficacy of the ZnONPs. After that, 100 µL of MTT dye at a concentration of 5 mg/mL PBS solution was poured into the wells containing medium, and NPs were incubated for 3 h. DMSO was employed to dissolve the purple-coloured formazan crystals. The absorbance was recorded at 570 nm using an ELISA microplate reader (Epoch BioTek). The L929 cells that were not treated with the ZnONPs served as a control. The percentage of cell viability was determined according to the following equation:
(4)
(4)
Ethidium bromide (EtBr) or AO staining
The dual staining of AO/EtBr with microscopic analysis was employed to detect cellular apoptosis. L929 cells were seeded at a concentration of 1x105 cells per well in 24-well microtitre plate and treated with the synthesised ZnONPs at 37 °C for 24h. The treated cells were gently rinsed with PBS (Phosphate buffered saline; pH 7.2) at room temperature. An aliquot of 9 µL of cell suspension was incubated with 1 µL of AO/EtBr dye mixture on a clean microscopic slide and covered with glass slips. Following 2-3 min of incubation, the cells were viewed under a fluorescence microscope (MEIJITECHNO pE-300 lite, Japan) [Citation34].
In vitro wound healing activity
An in vitro wound scratch assay was performed to assess the wound healing potential of ZnONPs according to the previously reported method by Veeraraghavan et al. [Citation35]. To achieve this, L929 fibroblast cells were cultured in the 6-well plates at a concentration of 1x104 cells per well and maintained for growth until they reached 90% confluency. Following incubation, the cell confluency was observed under a microscope, and the wound was created by scratching the cells with the help of a sterile micropipette tip, then washed with fresh medium to remove the cell debris. Generated wounds with uniform breath were treated with different concentrations (25, 50, 75, and 100 µg/ml) of biosynthesized ZnONPs and incubated at 37 °C for 24 h in a humidified environment (5% CO2). At the predetermined intervals, the closure of the wound scratch was microscopically monitored, and the images were captured using an inverted microscope. The percentage of wound healing was calculated using the following algorithm:
(5)
(5)
where At0 = scratch area at time 0, At1 = scratch area at specified time interval.
Photocatalytic activity
The photocatalytic effect of the bioinspired ZnONPs was evaluated by the degradation of methylene blue under solar irradiation. To perform the assay, a stock solution was prepared by dispersing 20 ppm of methylene blue (MB) in 50 ml of deionised water. Briefly, 20 mg of the synthesised ZnONPs was mixed with 45 ml of the methylene blue dye solution and incubated in the dark for 30 min in order to attain the adsorption-desorption equilibrium. Following incubation, the reaction mixture was exposed to sunlight, and the samples were collected every 30 min and centrifuged for 20 min at 15000 rpm to remove the catalyst used in the reaction. UV spectrum was recorded at regular time intervals using a UV-Visible spectrophotometer (Jasco V-750 double-beam) until complete degradation of the MB dye was achieved. The percentages of MB dye degradation were calculated according to the following equation:
(6)
(6)
where Mo is the original concentration of MB and M is the MB concentration after catalytic degradation at regular time intervals [Citation36]. All the results of the biological activities presented in the study were expressed as mean ± SD.
Results and discussion
Green synthesis of zinc oxide nanoparticles (ZnONPs)
Metal nanoparticles are synthesised using green chemistry or biological processes. Using cellular enzymes and secondary metabolites, endophytic microorganisms like bacteria, fungi, and actinomycetes are skilled in converting metal ions into metallic NPs like Ag, Au, Zn, and Cu [Citation37,Citation38]. When exposed to high levels of metallic ion stress, endophytic fungi establish a defence mechanism to minimise the toxicity of metal ions by precipitating metal ions at the nanoscale scale to generate NPs. Due to their resistance to metallic ion stress, endophytic fungi have become a promising option for NP production. We synthesised the zinc oxide nanoparticles in our study using an extract from the previously discovered endophytic fungus Xylaria arbuscula.
ZnONPs were synthesised using an endophytic fungal extract of Xylaria arbuscula as a capping and stabilising agent. When the reaction was carried out between the fungal extract and Zinc acetate solution, the appearance of a white precipitate confirmed the biosynthesis of ZnO nanoparticles. The bioactive compounds contained in the fungal extract could be attributed to the reduction of Zn+ ions, resulting in the production of highly stable, eco-friendly ZnO nanoparticles. After proper reduction, the colour-transformed solution was subjected to centrifugation for 15 min at 12,000 rpm to obtain pure nanoparticles. The pellets were dried in an oven at 80 °C after being washed thrice with distilled water. The dried NPs were milled to a fine powder using a pestle and mortar and subsequently calcined at 500 °C for 2 h to remove biomolecules and any impurities. The ground powder was stored at 4 °C for physiochemical characterisations and biological applications.
Characterisation of mycofabricated ZnONPs
The formation of the biogenic ZnONPs was initially confirmed by the colour change (from colourless to white) in the reaction mixture due to the reduction of Zn ions by the bioactive constituents present in the fungal extract. After proper reduction, the optical properties of the biogenic ZnONPs were assessed by recording the absorbance using the UV-Vis spectrometer. The results of the UV-spectroscopy were obtained as absorbance versus wavelength plots with a characteristic peak position within the wavelength range of 300–600 nm. The UV-Vis spectrum of the synthesised ZnONPs exhibited a surface plasmon resonance peak at 370 nm, which is a sign of a distinctive peak of the ZnONPs (). The result of our study is relatively in corroboration with earlier studies in which the maximum absorption band of mycofabricated ZnONPs via Xylaria acuta was around 370 nm [Citation21]. Similarly, the maximum SPR peak for bioinspired ZnONPs synthesised by employing Fraxinus rhynchophylla [Citation39] and Aspergillus terreus [Citation40] was also perceived at 370 nm. However, due to the broad absorbance peak at 370 nm, it was thought that the ZnONPs might be stable and monodispersed in nature.
Figure 1. Green synthesis of ZnONPs using Xylaria arbuscula fungal extract: (A) UV absorption spectrum of biologically synthesized ZnO nano particles, (B) XRD pattern of fabricated ZnONPs, and (C) FTIR spectrum of zinc oxide nanoparticles synthesized using fungal extract.
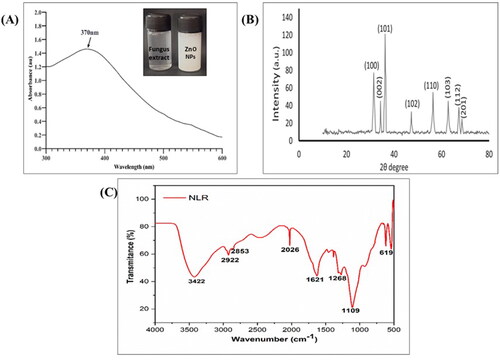
The crystalline size and structure of the biosynthesized ZnONPs were investigated using X-ray diffraction analysis (XRD). Numerous 2θ values or diffraction angles of 31.5°, 34.2°, 36.3°, 56.4°, 62.7°, and 67.3° were observed at respective reflection planes of (100), (002), (101), (102), (110), (103), (112), and (201) as shown in . Thus, the XRD diffractogram of ZnONPs showcased its hexagonal crystalline nature, which is in agreement with the findings documented for the biofabricated ZnONPs [Citation41]. The crystalline nature of ZnONPs was further confirmed after comparison with previously released data from the Joint Committee on Powder Diffraction Standards. The average size of mycofabricated ZnONPs at the most prominent reflection plane (101) was found to be 21 nm according to the Debye-Scherrer equation. The indexation reveals that all the characteristic peaks were of ZnONPs, indicating that they are free from any other impurities and significantly possess the standard hexagonal wurtzite crystalline phase, which is consistent with the results of earlier studies [Citation36].
The FTIR spectrum affirms the existence of diverse functional groups responsible for the capping of bioinspired NPs and the reduction of Zinc ions. portrayed the FTIR spectrogram of ZnONPs and exhibited characteristic peaks at 3422, 2922, 2853, 2026, 1621, 1268, 1109, and 619 cm−1. The broad vibrational peak at 3422 cm−1 in the spectra indicates the O-H stretching, showing the involvement of alcohols or polyphenols as a functional group, whereas the absorption bands observed at around 2922 cm−1 and 2853 cm−1 were associated with the aliphatic hydrocarbons having C-H and CH2 stretch. Also, the peaks obtained due to the amide groups in the range 1621 cm−1 might be due to the C = O stretching. In addition, the vibrational peaks at 1268 cm−1 and 1109 cm−1 correspond to CO stretch and C-N stretch (aromatic amines), respectively. The absorption peak at 619 cm−1 clearly indicates the Zn-O bond stretch, suggesting that ZnONPs were effectively synthesised. The different functional groups detected from the FTIR spectrum revealed that the phenols, alcohols, aliphatic hydrocarbons, amides, and aromatic groups might act as capping and reducing agents of zinc ions to ZnONPs which is in concurrence with the previous literature [Citation40].
The surface morphology of ZnONPs was examined using a scanning electron microscope (SEM) at a magnification of 45 and a 15 kV acceleration voltage. reveals a highly characteristic hexagonal shape. EDX analysis can be used to confirm the composition and dispersion of the nanoparticles through elemental mapping and spectrum analysis. The output curve of the EDX analysis clearly illustrates an intense peak for zinc (), which confirmed the existence and synthesis of ZnONPs. The other distinct peaks in the EDX spectra correspond to carbon, oxygen, and sodium. The additional peaks might be due to the bioactive constituents of the fungal extract, indicating their involvement as a capping and reducing agent for the synthesis of NPs.
A deeper insight into the morphology and exact nanostructure of ZnONPs was observed using TEM micrographs, as depicted in . The examination revealed the synthesised ZnONPs in non-agglomerated spherical to hexagonal shapes. On the other hand, the synthesised nanoparticles' size was evaluated by DLS. DLS is a practical, easy, and non-destructive technique for determining the distribution of particle dimensions. The results of our experiment revealed that synthesised NPs average size range of 116 nm, as shown in .
Figure 3. TEM Image of ZnONPs: (A) Green synthesized ZnONPs, (B) Enlarged view of a ZnONPs, (C) Dispersion of ZnONPs, (D) SAED Pattern, (E) Size distribution analysis of ZnONPs by dynamic light scattering (DLS), and (F) Surface zeta potential of synthesized ZnONPs.
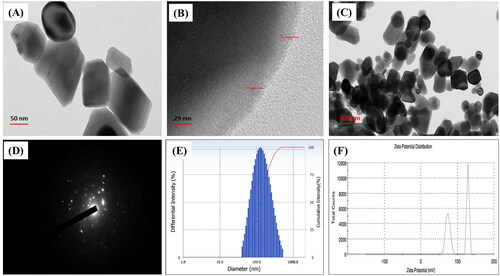
The zeta potential and size distribution of mycofabricated ZnONPs have been analysed using the dynamic light scattering technique. The zeta potential (ZP) is one of the crucial factors in determining the surface charge and stability of the nanoparticles in suspension, as it provides the net electrostatic potential of NPs [Citation41]. Significantly, particles with high zeta potential are thought to be extremely stable nanoparticles Neutral NPs are those possessing a zeta potential between 10 mV and +10mv, whereas strongly cationic and anionic NPs are those with a Zeta potential greater than +30mv or less than 30 mV, respectively [Citation20]. In our study, the zeta potential of ZnONPs showed potential stability with a limited chance of nanoparticle aggregation in the range of 50 mV to +100 mV (). The high positive potential value of the synthesised ZnONPs indicates that they are highly stable and strongly cationic in nature. Due to the electrostatic force of repulsion, nanoparticles with strong positive or negative ZP never agglomerate, but those with minimum ZP frequently flocculate [Citation41].
Biological applications of biogenically synthesised ZnO-NPs
Antioxidant activity
Free radicals are unstable molecules that the cell produces in response to environmental and other stimuli. Antioxidants are substances that assist in preventing or reducing cell damage caused by these unstable molecules [Citation42]. The in vitro antioxidant potential of the ZnONPs was screened using the most widely adopted method, the DPPH (2,2-diphenyl-1-picrylhydrazyl) free radical scavenging assay. DPPH is a stable compound, reduced by accepting an electron [Citation43], and the quenching of these free radicals by the antioxidants is confirmed by the change in colour from purple to yellow [Citation31], where the rate of discolouration depends on the concentration and potency of antioxidants [Citation44]. DPPH free radicals were exposed to varying doses of ZnONPs and it was found that NPs successfully scavenged the free radicals in a concentration-dependent manner, which was evident from . The maximum scavenging activity of ZnONPs was calculated to be 83.99 ± 0.93% recorded at 500 µg/mL while the minimum scavenging activity 66.21 ± 2.01% was recorded at 10 µg/mL. Similarly, Faisal et al. [Citation36] carried out a biogenic, eco-friendly synthesis of ZnONPs using the fruit extracts of Myristica fragrans and suggested that the antioxidant potential increases proportionally with the concentration of the nanoparticles. The obtained results were in agreement with the recent study Gao et al. 2019 [Citation45], in which the mycosynthesized NPs using A. niger displayed maximum inhibition of 57.74% at a 100 µg/mL concentration of ZnONPs, suggesting that the free radical quenching ability of the NPs may be due to the bioactive compounds of the fungal biomass. Thus, the results indicate that the ZnONPs synthesised using endophytic fungi served as a promising candidate for quenching the deleterious free radicals, which might be due to the presence of biologically active constituents in the fungal biomass.
Table 1. DPPH Scavenging potential of biogenically synthesised ZnONPs.
Antibacterial activity
The mycosynthesized ZnONPs were assessed for their antibacterial potential against Gram positive (Staphylococcus aureus, Staphylococcus epidermidis, Bacillus subtilis, Enterococcus faecalis) and Gram-negative (Escherichia coli, Pseudomonas aeruginosa, Klebsiella pneumoniae, Proteus mirabilis) bacteria by disc diffusion method. Multiple dosages were employed to determine the susceptibility of the bacteria, ranging from 25 µg/mL to 100 µg/mL. The use of antibiotics and antimicrobial resistance (AMR) has now become inevitable, necessitating the development of novel therapeutic strategies [Citation27]. One of the most promising strategies for battling bacterial antibiotic resistance is the application of metal NPs and their oxides [Citation46]. The measured zone of inhibition values of varying doses of ZnONPs against the bacterial cultures is reported in . However, as shown by the findings, at the highest concentration, 100 µg/mL ZnONPs had a strong bactericidal effect and recorded a maximum zone of inhibition (in mm) against E. coli (1.5 ± 0.08 mm), P. aeruginosa (1.4 ± 0.05 mm), E. Faecalis (1.4 ± 0.07 mm) followed by S. aureus (1.2 ± 0.04), S. epidermidis (1.0 ± 0.04 mm), B. subtilis (1.0 ± 0.04 mm) while a comparatively minimum zone was recorded for P. mirabilis (0.9 ± 0.02 mm) and K. pneumoniae (0.7 ± 0.02 mm). The outcomes of the assay displayed a significant dose-dependent effect of ZnONPs on bacterial cultures, as shown in . ZnONPs have been postulated as a mode of action against bacteria based on the generation of reactive oxygen species (ROS) as a consequence of light, the dissolution of metal oxide nanoparticles, along with the electrostatic correspondence of nanoparticles with the cell walls of the microorganisms. The antibacterial potential of the ZnONPs could be attributed to their predominant aggregation on the outer surface of the bacterial membrane. The ROS produced by the NPs triggers membrane permeability upon interaction with the bacterial membrane, resulting in cell death [Citation47]. The results were approximately correlated with the results of Ganesan et al. [Citation48], where the highest concentration of mycosynthesized ZnONPs exhibited significant inhibition of bacterial growth against S. aureus and E. coli. The dose-dependent bactericidal action of ZnONPs synthesised using endophytic fungi (Aspergillus sp.) against pathogenic strains (K. pneumoniae and B. subtilis) was noticed in the investigation of Kumar et al. [Citation25], and this correlates with the current investigation. The research of Khan et al. [Citation49], determined that the effective antibacterial activity elucidated by the ZnONPs was attributed to the adhesion of NPs to the bacterial cell wall, leading to a progressive metabolic response resulting in the generation of ROS. Several studies have been conducted using mycofabricated ZnONPs and explored a broad spectrum of antibacterial efficacy against various pathogenic strains, including S. aureus, Escherichia coli, Pseudomonas aeruginosa, and Klebsiella pneumoniae [Citation22,Citation36]. This manifests that the mycofabricated ZnONPs have potent antibacterial properties that could inhibit a wide range of bacterial pathogens and be explored as antibacterial agents.
Figure 4. Antimicrobial activity of green synthesized ZnONPs: (A) Antibacterial activity of synthesized ZnONPs through disc diffusion method, (B) Antifungal activity of ZnONPs against Candida albicans and Aspergillus niger.
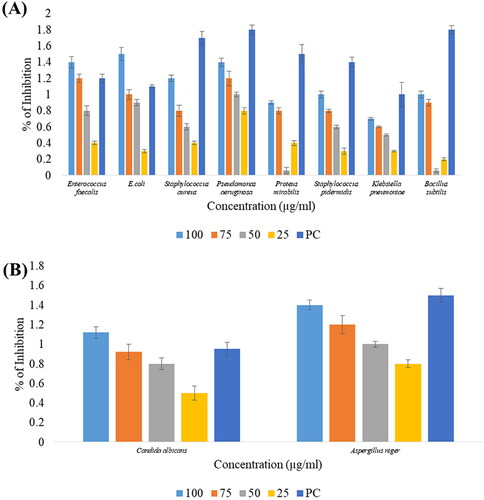
Table 2. Antibacterial activity of biologically synthesised ZnONPs.
Antifungal activity
In this experiment, the antifungal efficacy of ZnONPs was evaluated against Candida albicans and Aspergillus niger at various doses using the disc diffusion method. The mycofabricated ZnONPs were found to possess a dose-dependent inhibitory effect and are more effective against A. niger than C. albicans as shown in and . The highest zone of inhibition against A. niger was determined to be 1.4 ± 0.05 mm at 100 µg/mL while the lowest inhibitory zone was calculated to be 0.8 ± 0.04 mm at 25 µg/mL whereas for C. albicans it was determined to be 1.12 ± 0.06 mm at 100 µg/mL and 0.5 ± 0.07 mm at 25 µg/mL respectively. The synthesised ZnONPs contribute to the fungistatic mechanism by inhibiting mycelial growth through their physical and chemical interactions with the sterols in fungal membranes [Citation21]. The antifungal potency of ZnONPs might be due to their disruptive activity and permeability of the cell membrane, which impair the integrity of the cell membrane, resulting in cell damage and death [Citation50]. Moreover, similar results were observed earlier, where ZnONPs synthesised using Acacia caesia (L.) displayed significant fungicidal activity against A. niger and C. albicans [Citation51]. Our findings are consistent with the previous research in which C. albicans was found to be more sensitive towards the ZnONPs synthesised using endophytic Alternaria tenuissima possessing 24.43 ± 1.25 mm inhibitory zone at a concentration of 400 µg/mL [Citation22].
Table 3. Antifungal activity of synthesised ZnONPs.
In vitro antidiabetic activity
Diabetes mellitus is a metabolic disorder marked by chronic hyperglycaemia due to the insensitivity of body cells to insulin or diminished insulin synthesis [Citation49,Citation52]. Reducing postprandial hyperglycaemia is one of the most effective tactics for mending diabetes, which may be accomplished by inhibiting α-amylase, an enzyme that converts carbohydrates into glucose and consequently plays a crucial part in the regulation of blood glucose levels [Citation31,Citation52]. The α-amylase enzyme effectiveness was assessed for the mycosynthesized ZnONPs by the spectrophotometric method and determined that the percentage inhibition increases with the concentration of the synthesised NPs, as shown in . The prepared ZnONPs were found to exhibit notable α-amylase inhibition 97.78 ± 0.48% at a higher concentration of 500 µg/mL compared to standard acarbose. Similarly, the inhibitory pattern of ZnONPs synthesised using the aqueous fruit extract of Myristica fragrans towards α-amylase is dose-dependent with a maximum inhibition of about 73.23 ± 0.42% at 400 µg/mL [Citation36]. Altogether, the results of our study were consistent with those of previous reports [Citation49,Citation53,Citation54] showing that the green synthesised ZnONPs possess a dose-dependent inhibitory impact on α-amylase activity. As a result, our findings suggest that the bioinspired ZnONPs may act as possible inhibitors of enzymes that break down carbohydrates, which could increase their potential for use in the efficient treatment of diabetes mellitus.
Table 4. In vitro α-amylase inhibitory activity of Bio fabricated ZnONPS.
In vitro anti-inflammatory activity
Protein denaturation is the primary cause of inflammation, and the potential of the nanoparticle’s protein denaturation was investigated as a part of an inquiry into the mechanism of anti-inflammatory action [Citation55]. Protein denaturation is a detrimental process in which a functional protein loses its biological function as a result of structural modifications spurred on by external stimuli such as chemicals, heat, etc. [Citation56]. Anti-inflammatory activity is one of the intriguing studies that attempt to examine the protective ability of NPs rather than their destructive aspect [Citation55]. Therefore, it is necessary to investigate the anti-inflammatory potential of the biosynthesized nanoparticles at the onset of their application as a therapeutic agent. The maximum inhibition of protein denaturation obtained employing the ZnONPs was found to be 96.77 ± 0.23% at 500 µg/mL concentration which was extremely close to the obtained value from the standard drug diclofenac sodium 98.45 ± 0.66% at maximum concentration, as shown in . Similarly, biogenic ZnONPs synthesised using L. edodes were found to inhibit protein denaturation in a dose-dependent manner with a maximum inhibition % of about 86.45 ± 0.60 in a similar trend of inhibition exhibited by diclofenac [Citation57].
Table 5. Albumin denaturation inhibition activity of biosynthesized ZnONPs.
Cytotoxic potential of ZnONPs
Bioactive compounds and nanoparticles with effective anticancer efficacy have become a focus of research in recent years due to their non-toxic and chemopreventive nature [Citation58]. The anticancer effect of mycofabricated ZnONPs was evaluated in L929 cell lines at varying concentrations using MTT assay and the results are depicted in and . The results suggest that the synthesised NPs have a defendable cytotoxic activity with a maximum inhibition of 79.00 ± 0.36% at 100 µg/mL which in turn reduces the cell viability. The dissolution of ZnONPs into Zn 2+, generation of ROS, and DNA damage are the three primary reactions behind the cytotoxic effect of ZnONPs. Furthermore, physical characteristics, including surface chemistry, size, and dosage, determine the total uptake, elimination, and anticancer activities of the NPs [Citation59]. Our results thus augment and support the findings of Gao et al. [Citation45], who reported the dose-dependent cytotoxic potential of ZnONPs synthesised using fungal biomass against HepG2 cell lines.
Figure 5. In vitro cytotoxicity of synthesized ZnONPs against L929 cell lines at various concentrations.
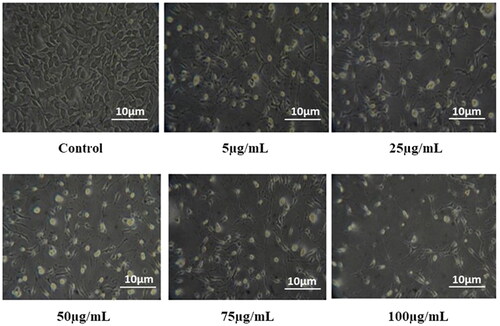
Table 6. Percentage of inhibition against the in vitro cytotoxicity of ZnONPs on L929 cell lines.
Further, the mycosynthesized ZnONPs-treated cells were stained by AO/EtBr to identify cellular death, and the results are shown in . Ethidium bromide was only absorbed by dead tissue and produced red fluorescence by intercalating with damaged DNA, but acridine orange was taken up by healthy cells and produced green fluorescence. Using the AO/EB assay, ZnONPs can be evaluated and contrasted with controls based on their capacity to destabilise cell membranes [Citation60]. The results showed an increase in early apoptotic cells and a decrease in viable cells. Dual staining with AO and EB was used to look for different signs of apoptosis in nucleate alternations. Cells that were still alive appear green, while cells that were dying appear orange or red. As shown in , when cells were exposed to ZnONPs, the membranes broke down, and more lysosome vacuoles were made than in untreated cells. Nanoparticles can get through the cellular membrane and affect the mRNA expression of suppression genes, which causes the production of reactive oxygen species (ROS) to rise in the cell, indicating that nanoparticles have a high ability to kill cells. When there is more ROS and oxidative stress, ZnONPs negatively affect the cell’s lipids, proteins, and nucleic acids. Increased ROS can damage membranes through lipid peroxidation and protein denaturation, which leads to cell death through necrosis and damage to DNA strands, which leads to cell death through a process called apoptosis [Citation60, Citation61]. As supporting the current findings, a similar result pattern was observed for the green synthesised ZnONPs using Clitorea Ternatea [Citation62].
In vitro wound scratch assay
The ultimate objective of wound healing management is to protect the wound site from infection-causing microbes and promote tissue wound healing [Citation35]. The intricate and active process of wound healing entails managing angiogenesis, connective tissue repair, and re-epithelialization. It is a response to cellular injury targeted at reconstructing the damaged tissue. According to testimony, the increased wound contraction and the quicker epithelialization time are primarily responsible for any evaluated drug’s wound-healing activity [Citation63]. An in vitro wound scratch assay was performed to address the impact of biosynthesized ZnONPs on wound healing in L929 cell lines, and the results are illustrated in . The wound healing efficacy was determined by visualising the closure of the wound at four different time intervals (0h, 4h, 18h, 24h) after their treatment with ZnONPs, and the results were compared with the control (non-treated with ZnONPs). The results displayed that the ZnONPs promote wound healing in a dose and time-dependent pattern and were found to exhibit maximum wound healing of 95.37 ± 1.12% at a concentration of 100 µg/mL after 24 h of incubation as compared to 87.58 ± 1.06% by the control. The nanoparticle treatment results in rapid closure of the wound as compared to the untreated cells, which is evident from . The nanosize, shape, surface chemistry, porosity, and capacity of the metal to withstand disintegration in an aqueous solution may contribute to the wound-healing efficacy of NPs [Citation64]. Similarly, collagen-blended ZnONPs embedded in niosome nanocomposite exhibited faster wound healing in the 3T3 fibroblast cell line with 99.24% cell migration after 72h while the untreated cells possessed a minimum cellular migration of 27.35% [Citation65]. The findings are consistent with those documented by El-sayed et al. [Citation64] in which mycofabricated ZnONPs using Monascus purpureus showcased complete wound closure after 48 h indicating its fastest wound healing efficacy. From the results, it was confirmed that the cell migration was obviously faster in the cells treated with ZnONPs, but it also prevented bacterial infection in wounds as it possessed significant antimicrobial activity.
Table 7. Percentage of wound healing activity at different time intervals using varied concentrations of biosynthesized ZnONPs.
3.4. Photocatalytic activity
The photocatalytic degradation efficacy of the fabricated ZnONPs was investigated through the degradation of methylene blue dye under sunlight irradiation. During the degradation process, the dye solution was kept in darkness for 30 min to attain absorption-desorption equilibrium and exposed to solar irradiation. The time-dependent absorption spectra of the MB dye can be witnessed . Moreover, the dye degradation process was confirmed by the gradual change in the colour of the dye from deep blue to colourless with increasing exposure time to sunlight. The degradation efficiency of 98.06% was recorded after 240 min of exposure to sunlight, which is evident from . In order to excite valence electrons and allow them to move from the valence band to the conduction band, a positive hole (h+) must be lifted inside the valence band after the dye has been adsorbed onto the surface of the catalyst (zinc). Adsorbed water molecules will react with the positive holes and free electrons on the surface of the photocatalyst, resulting in the production of superoxide anion O2•- and OH- radicals from the positive hole reaction with the water. The dye molecules are degraded by these light-generated radicals into simple molecules like CO2 and H2O. The results of our study were significantly higher than the degradation efficiency reported for the degradation of methylene blue by ZnONPs synthesised using an aqueous extract of Myristica fragrans which inhibits only 88% [Citation36].
Conclusion
This research has provided insights into the rising contribution of endophytic fungi to the fabrication of nanoparticles. Here we provide an eco-friendly, non-toxic, and inexpensive method to fabricate ZnONPs using the endophytic fungus Xylaria arbuscula isolated from Blumea axillaris Linn. The mycogenically fabricated ZnONPs were exclusively characterised using UV–Vis spectroscopy, FT-IR, SEM, XRD, TEM, DLS, EDX, and Zeta Potential. The analysis revealed that ZnONPs are nanoscale, cylindrical rods, and hexagonal-shaped in nature and were found to be highly stable. Further, the synthesised ZnONPs displayed appreciable biological properties, including antimicrobial activity against clinical pathogens, antioxidant, antidiabetic, anti-inflammatory, cytotoxic, and wound-healing activities. Eventually, it also possessed dye degradation potential, which was confirmed by photocatalytic activity. The synergistic interaction between ZnONPs and bioactive compounds produced by endophytic fungi, which are present on the surface of NPs, may contribute to the potent biological properties of ZnONPs. Hence, it was concluded that the biogenically synthesised ZnONPs could be utilised for a variety of medical applications as well as environmental applications. However, further research is needed for the isolation and purification of bioactive compounds.
Author contributions
Preethi Kathirvel designed the experiments and administered the project. Lavanya Nehru and Gayathri Devi Kandasamy performed the lab experiments. Mohammed Ali Alshehri and Abdulrahman Alasmari contributed to the analysis tools. Lavanya Nehru and Gayathri Devi Kandasamy analysed the data, prepared the figures, and wrote the original draft. Chellasamy Panneerselvam and Vanaraj Sekar contributed to the formal analysis and data curation. Preethi Kathirvel proofread the final version of the manuscript. All authors have read and agreed to the published version of the manuscript.
Acknowledgments
The authors gratefully thank DST-PURSE (PHASE-II), DST-FIST supported Department of Microbial Biotechnology, Bharathiar University, Tamil Nadu, India for providing suitable research facilities. Dr. Abdulrahman Alasmari is grateful to the Deanship of Scientific Research (DSR), University of Tabuk, Tabuk, Saudi Arabia for providing funding of this research through project no: S-1440-341.
Disclosure statement
No potential conflict of interest was reported by the author(s).
Data availability statement
All data were included in the manuscript file. The data that support the findings of this study are available from the corresponding author (P.K), upon reasonable request.
Additional information
Funding
References
- Lal S, Verma R, Chauhan A, et al. Antioxidant, antimicrobial, and photocatalytic activity of green synthesized ZnO-NPs from Myrica esculenta fruits extract. Inorg Chem Commun. 2022;141:109518. doi: 10.1016/j.inoche.2022.109518.
- El-Belely EF, Farag MM, Said HA, et al. Green synthesis of zinc oxide nanoparticles (ZnO-NPs) using Arthrospira platensis (class: Cyanophyceae) and evaluation of their biomedical activities. Nanomaterials. 2021;11(1):95. doi: 10.3390/nano11010095.
- Abdelkader DH, Negm WA, Elekhnawy E, et al. Zinc oxide nanoparticles as potential delivery carrier: green synthesis by Aspergillus niger endophytic fungus, characterization, and in vitro/in vivo antibacterial activity. Pharmaceuticals. 2022;15(9):1057. doi: 10.3390/ph15091057.
- Shah R, Shah SA, Shah S, et al. Green synthesis and antibacterial activity of gold nanoparticles of digera muricata. pharmaceutical-sciences. 2020;82(2):374–378. doi: 10.36468/pharmaceutical-sciences.659.
- El-Moslamy SH, Elnouby MS, Rezk AH, et al. Scaling-up strategies for controllable biosynthetic ZnO NPs using cell free-extract of endophytic Streptomyces albus: characterization, statistical optimization, and biomedical activities evaluation. Sci Rep. 2023;13(1):3200. doi: 10.1038/s41598-023-29757-9.
- Khan SH. Green nanotechnology for the environment and sustainable development. In Green materials for wastewater treatment. Springer; 2020. p. 13–46. doi: 10.1007/978-3-030-17724-9_2.
- Salem SS, Fouda A. Green synthesis of metallic nanoparticles and their prospective biotechnological applications: an overview. Biol Trace Elem Res. 2021;199(1):344–370. doi: 10.1007/s12011-020-02138-3.
- El-Sayed ESR, Abdelhakim HK, Ahmed AS. Solid-state fermentation for enhanced production of selenium nanoparticles by gamma-irradiated Monascus purpureus and their biological evaluation and photocatalytic activities. Bioprocess Biosyst Eng. 2020;43(5):797–809. doi: 10.1007/s00449-019-02275-7.
- Hussein HG, El-Sayed ESR, Younis NA, et al. Harnessing endophytic fungi for biosynthesis of selenium nanoparticles and exploring their bioactivities. AMB Expr. 2022;12(1):68. doi: 10.1186/s13568-022-01408-8.
- El-Sayed ESR, Abdelhakim HK, Zakaria Z. Extracellular biosynthesis of cobalt ferrite nanoparticles by Monascus purpureus and their antioxidant, anticancer and antimicrobial activities: yield enhancement by gamma irradiation. Mater Sci Eng C Mater Biol Appl. 2020;107:110318. doi: 10.1016/j.msec.2019.110318.
- Faisal, S., Khan, M. A., Jan, H., Shah, S. A., Shah, S., Rizwan, M., & Akbar, M. T. (2021). Edible mushroom (Flammulina velutipes) as biosource for silver nanoparticles: from synthesis to diverse biomedical and environmental applications. Nanotechnology, 32(6), 065101. doi: 10.1088/1361-6528/abc2eb.
- Singh T, Jyoti K, Patnaik A, et al. Biosynthesis, characterization and antibacterial activity of silver nanoparticles using an endophytic fungal supernatant of Raphanus sativus. J Genet Eng Biotechnol. 2017;15(1):31–39. doi: 10.1016/j.jgeb.2017.04.005.
- Clarance P, Luvankar B, Sales J, et al. Green synthesis and characterization of gold nanoparticles using endophytic fungi Fusarium solani and its in-vitro anticancer and biomedical applications. Saudi J Biol Sci. 2020;27(2):706–712. doi: 10.1016/j.sjbs.2019.12.026.
- Imran M, Jan H, Faisal S, et al. In vitro examination of anti-parasitic, anti-Alzheimer, insecticidal and cytotoxic potential of Ajuga bracteosa wallich leaves extracts. Saudi J Biol Sci. 2021;28(5):3031–3036. doi: 10.1016/j.sjbs.2021.02.044.
- Anwar MM, Aly SS, Nasr EH, et al. Improving carboxymethyl cellulose edible coating using ZnO nanoparticles from irradiated Alternaria tenuissima. AMB Expr. 2022;12(1):1–11. doi: 10.1186/s13568-022-01459-x.
- Hatab MH, Rashad E, Saleh HM, et al. Effects of dietary supplementation of myco-fabricated zinc oxide nanoparticles on performance, histological changes, and tissues Zn concentration in broiler chicks. Sci Rep. 2022;12(1):18791. doi: 10.1038/s41598-022-22836-3.
- Mohamed AA, Abu-Elghait M, Ahmed NE, et al. Eco-friendly mycogenic synthesis of ZnO and CuO nanoparticles for in vitro antibacterial, antibiofilm, and antifungal applications. Biol Trace Elem Res. 2021;199(7):2788–2799. doi: 10.1007/s12011-020-02369-4.
- Alhujaily M, Albukhaty S, Yusuf M, et al. Recent advances in plant-mediated zinc oxide nanoparticles with their significant biomedical properties. Bioengineering. 2022;9(10):541. doi: 10.3390/bioengineering9100541.
- Mishra P, Ahmad A, Al-Keridis LA, et al. Doxorubicin-conjugated zinc oxide nanoparticles, biogenically synthesised using a fungus Aspergillus niger, exhibit high therapeutic efficacy against lung cancer cells. Molecules. 2022;27(8):2590. doi: 10.3390/molecules27082590.
- Kaur T, Bala M, Kumar G, et al. Biosynthesis of zinc oxide nanoparticles via endophyte Trichoderma viride and evaluation of their antimicrobial and antioxidant properties. Arch Microbiol. 2022;204(10):620. doi: 10.1007/s00203-022-03218-9.
- Sumanth B, Lakshmeesha TR, Ansari MA, et al. Mycogenic synthesis of extracellular zinc oxide nanoparticles from Xylaria acuta and its nanoantibiotic potential. IJN. 2020;ume 15:8519–8536.) doi: 10.2147/IJN.S271743.
- Abdelhakim HK, El‐Sayed ER, Rashidi FB. Biosynthesis of zinc oxide nanoparticles with antimicrobial, anticancer, antioxidant and photocatalytic activities by the endophytic alternaria tenuissima. J Appl Microbiol. 2020;128(6):1634–1646. doi: 10.1111/jam.14581.
- Kadam VV, Ettiyappan JP, Balakrishnan RM. Mechanistic insight into the endophytic fungus mediated synthesis of protein capped ZnO nanoparticles. Mat Sci Eng b. 2019;243:214–221. doi: 10.1016/j.mseb.2019.04.017.
- El-Sayed ESR, Mousa SA, Abdou DA, et al. Exploiting the exceptional biosynthetic potency of the endophytic Aspergillus terreus in enhancing production of Co3O4, CuO, Fe3O4, NiO, and ZnO nanoparticles using bioprocess optimization and gamma irradiation. Saudi J Biol Sci. 2022;29(4):2463–2474. doi: 10.1016/j.sjbs.2021.12.019.
- Kumar RV, Vinoth S, Baskar V, et al. Synthesis of zinc oxide nanoparticles mediated by Dictyota dichotoma endophytic fungi and its photocatalytic degradation of fast green dye and antibacterial applications. S Afr J Bot. 2022;151:337–344. doi: 10.1016/j.sajb.2022.03.016.
- Lavanya N, Manon V, Saranya N, et al. Endophytic fungal isolation from Blumea axillaris: identification and biological activity of secondary metabolites. Not Sci Biol. 2021;13(2):10953–10953. doi: 10.15835/nsb13210953.
- Mohamed AA, Fouda A, Abdel-Rahman MA, et al. Fungal strain impacts the shape, bioactivity and multifunctional properties of green synthesised zinc oxide nanoparticles. Biocatal Agric Biotechnol. 2019;19:101103. doi: 10.1016/j.bcab.2019.101103.
- Zafar, S., Faisal, S., Jan, H., Ullah, R., Rizwan, M., Abdullah , & Khattak, A. (2022). Development of iron nanoparticles (FeNPs) using biomass of enterobacter: its characterization, antimicrobial, anti-Alzheimer’s, and enzyme inhibition potential. Micromachines, 13(8), 1259. doi: 10.3390/mi13081259.
- Abomuti MA, Danish EY, Firoz A, et al. Green synthesis of zinc oxide nanoparticles using salvia officinalis leaf extract and their photocatalytic and antifungal activities. Biology. 2021;10(11):1075. doi: 10.3390/biology10111075.
- Faisal, S., Khan, S., Abdullah , Zafar, S., Rizwan, M., Ali, M., … & Akbar, F. (2022). Fagonia cretica-mediated synthesis of manganese oxide (MnO2) nanomaterials their characterization and evaluation of their bio-catalytic and enzyme inhibition potential for maintaining flavor and texture in apples. Catalysts, 12(5), 558. doi: 10.3390/catal12050558.
- Hussain, T., Faisal, S., Rizwan, M., Zaman, N., Iqbal, M., Iqbal, A., & Ali, Z. (2022). Green synthesis and characterization of copper and nickel hybrid nanomaterials: investigation of their biological and photocatalytic potential for the removal of organic crystal violet dye. Journal of Saudi Chemical Society, 26(4), 101486. doi: 10.1016/j.jscs.2022.101486.
- Sharifi-Rad M, Pohl P, Epifano F, et al. Green synthesis of silver nanoparticles using Astragalus tribuloides delile. root extract: characterization, antioxidant, antibacterial, and anti-inflammatory activities. Nanomaterials. 2020;10(12):2383. doi: 10.3390/nano10122383.
- Faisal, S., Abdullah Jan, H., Shah, S. A., Shah, S., Rizwan, M., & Masood, R. (2021). Bio-catalytic activity of novel Mentha arvensis intervened biocompatible magnesium oxide nanomaterials. Catalysts, 11(7), 780. doi: 10.3390/catal11070780.
- Prasannaraj G, Venkatachalam P. Green engineering of biomolecule-coated metallic silver nanoparticles and their potential cytotoxic activity against cancer cell lines. Adv Nat Sci. 2017;8(2):025001. doi: 10.1088/2043-6254/aa6d2c.
- Veeraraghavan VP, Periadurai ND, Karunakaran T, et al. Green synthesis of silver nanoparticles from aqueous extract of Scutellaria barbata and coating on the cotton fabric for antimicrobial applications and wound healing activity in fibroblast cells (L929). Saudi J Biol Sci. 2021;28(7):3633–3640. doi: 10.1016/j.sjbs.2021.05.007.
- Faisal, S., Jan, H., Shah, S. A., Shah, S., Khan, A., Akbar, M. T., … & Syed, S. (2021). Green synthesis of zinc oxide (ZnO) nanoparticles using aqueous fruit extracts of myristica fragrans: their characterizations and biological and environmental applications. ACS Omega, 6(14), 9709–9722. doi: 10.1021/acsomega.1c00310.
- Joshi CG, Danagoudar A, Poyya J, et al. Biogenic synthesis of gold nanoparticles by marine endophytic fungus-Cladosporium cladosporioides isolated from seaweed and evaluation of their antioxidant and antimicrobial properties. Process Biochem. 2017;63:137–144. doi: 10.1016/j.procbio.2017.09.008.
- Soliman H, Elsayed A, Dyaa A. Antimicrobial activity of silver nanoparticles biosynthesised by rhodotorula sp. strain ATL72. Egyptian Journal of Basic and Applied Sciences. 2018;5(3):228–233. doi: 10.1016/j.ejbas.2018.05.005.
- Wang Z, Que B, Gan J, et al. Zinc oxide nanoparticles synthesized from fraxinus rhynchophylla extract by green route method attenuates the chemical and heat induced neurogenic and inflammatory pain models in mice. J Photochem Photobiol B. 2020;202:111668. doi: 10.1016/j.jphotobiol.2019.111668.
- Mousa SA, El-Sayed ESR, Mohamed SS, et al. Novel mycosynthesis of Co 3 O 4, CuO, Fe 3 O 4, NiO, and ZnO nanoparticles by the endophytic Aspergillus terreus and evaluation of their antioxidant and antimicrobial activities. Appl Microbiol Biotechnol. 2021;105(2):741–753. doi: 10.1007/s00253-020-11046-4.
- Al-Kordy HM, Sabry SA, Mabrouk ME. Statistical optimization of experimental parameters for extracellular synthesis of zinc oxide nanoparticles by a novel haloalaliphilic Alkalibacillus sp. W7. Sci Rep. 2021;11(1):1–14. doi: 10.1038/s41598-021-90408-y.
- Al-Radadi, Najlaa S, Hussain, Tahir, Faisal, Shah, Ali Raza Shah, Syed,. (2022). Novel biosynthesis, characterization and bio-catalytic potential of green algae (Spirogyra hyalina) mediated silver nanomaterials. Saudi J Biol Sci, 29(1), 411–419. doi: 10.1016/j.sjbs.2021.09.013.
- Shah S, Shah SA, Faisal S, et al. Engineering novel gold nanoparticles using Sageretia thea leaf extract and evaluation of their biological activities. J Nanostruct Chem. 2022;12(1):129–140. doi: 10.1007/s40097-021-00407-8.
- Ullah R, Shah S, Muhammad Z, et al. In vitro and in vivo applications of Euphorbia wallichii shoot extract-mediated gold nanospheres. Green Processing and Synthesis. 2021;10(1):101–111. doi: 10.1515/gps-2021-0013.
- Gao Y, Arokia Vijaya Anand M, Ramachandran V, et al. Biofabrication of zinc oxide nanoparticles from Aspergillus niger, their antioxidant, antimicrobial and anticancer activity. J Clust Sci. 2019;30(4):937–946. doi: 10.1007/s10876-019-01551-6.
- Faisal, S., Jan, H., Abdullah , Alam, I., Rizwan, M., Hussain, Z., … & Uddin, M. N. (2022). In vivo analgesic, anti-inflammatory, and anti-diabetic screening of Bacopa monnieri-synthesized copper oxide nanoparticles. ACS Omega, 7(5), 4071–4082. doi: 10.1021/acsomega.1c05410.
- Faisal, S., Rizwan, M., Ullah, R., Alotaibi, A., Khattak, A., Bibi, N., & Idrees, M. (2022). Paraclostridium benzoelyticum bacterium-mediated zinc oxide nanoparticles and their in vivo multiple biological applications. Oxid Med Cell Longev, 59940332022. doi: 10.1155/2022/5994033.
- Ganesan V, Hariram M, Vivekanandhan S, et al. Periconium sp.(endophytic fungi) extract mediated sol-gel synthesis of ZnO nanoparticles for antimicrobial and antioxidant applications. Mater Sci Semicond Process. 2020;105:104739. doi: 10.1016/j.mssp.2019.104739.
- Khan, M. I., Shah, S., Faisal, S., Gul, S., Khan, S., Abdullah , … & Shah, W. A. (2022). Monotheca buxifolia driven synthesis of zinc oxide nano material its characterization and biomedical applications. Micromachines, 13(5), 668. doi: 10.3390/mi13050668.
- Sivasankarapillai VS, Krishnamoorthy N, Eldesoky GE, et al. One-pot green synthesis of ZnO nanoparticles using Scoparia dulcis plant extract for antimicrobial and antioxidant activities. Appl Nanosci. 2022;:1–11. doi: 10.1007/s13204-022-02610-7.
- Ashwini J, Aswathy TR, Rahul AB, et al. Synthesis and characterization of zinc oxide nanoparticles using Acacia caesia bark extract and its photocatalytic and antimicrobial activities. Catalysts. 2021;11(12):1507. doi: 10.3390/catal11121507.
- Jan H, Khan MA, Usman H, et al. The Aquilegia pubiflora (himalayan columbine) mediated synthesis of nanoceria for diverse biomedical applications. RSC Adv. 2020;10(33):19219–19231. doi: 10.1039/D0RA01971B.
- Rehman H, Ali W, Khan NZ, et al. Delphinium uncinatum mediated biosynthesis of zinc oxide nanoparticles and in-vitro evaluation of their antioxidant, cytotoxic, antimicrobial, anti-diabetic, anti-inflammatory, and anti-aging activities. Saudi J Biol Sci. 2023;30(1):103485. doi: 10.1016/j.sjbs.2022.103485.
- Al-Radadi, N. S., Faisal, S., Alotaibi, A., Ullah, R., Hussain, T., Rizwan, M., … & Ali, Z. (2022). Zingiber officinale driven bioproduction of ZnO nanoparticles and their anti-inflammatory, anti-diabetic, anti-Alzheimer, anti-oxidant, and anti-microbial applications. Inorganic Chemistry Communications, 140, 109274. doi: 10.1016/j.inoche.2022.109274.
- Vijayakumar N, Bhuvaneshwari VK, Ayyadurai GK, et al. Green synthesis of zinc oxide nanoparticles using Anoectochilus elatus, and their biomedical applications. Saudi J Biol Sci. 2022;29(4):2270–2279. doi: 10.1016/j.sjbs.2021.11.065.
- Kyene MO, Droepenu EK, Ayertey F, et al. Synthesis and characterization of ZnO nanomaterial from Cassia sieberiana and determination of its anti-inflammatory, antioxidant and antimicrobial activities. Sci Afr. 2023;19:e01452. doi: 10.1016/j.sciaf.2022.e01452.
- Amin ZS, Afzal M, Ahmad J, et al. Synthesis, characterization and biological activities of zinc oxide nanoparticles derived from secondary metabolites of Lentinula edodes. Molecules. 2023;28(8):3532. doi: 10.3390/molecules28083532.
- Faisal, S., Al-Radadi, N. S., Jan, H., Abdullah , Shah, S. A., Shah, S., … & Bibi, N. (2021). Curcuma longa mediated synthesis of copper oxide, nickel oxide and Cu-Ni bimetallic hybrid nanoparticles: characterization and evaluation for antimicrobial, anti-parasitic and cytotoxic potentials. Coatings, 11(7), 849. doi: 10.3390/coatings11070849.
- Jan H, Shah M, Andleeb A, et al. Plant-based synthesis of zinc oxide nanoparticles (ZnO-NPs) using aqueous leaf extract of Aquilegia pubiflora: their antiproliferative activity against HepG2 cells inducing reactive oxygen species and other in vitro properties. Oxid Med Cell Longev. 2021;2021:4786227. doi: 10.1155/2021/4786227.
- Oves M, Aslam M, Rauf MA, et al. Antimicrobial and anticancer activities of silver nanoparticles synthesized from the root hair extract of Phoenix dactylifera. Mater Sci Eng C Mater Biol Appl. 2018;89:429–443. doi: 10.1016/j.msec.2018.03.035.
- Nita M, Grzybowski A. The role of the reactive oxygen species and oxidative stress in the pathomechanism of the age-related ocular diseases and other pathologies of the anterior and posterior eye segments in adults. Oxid Med Cell Longev. 2016;2016:3164734. doi: 10.1155/2016/3164734.
- Alahmdi MI, Syed K, Vanaraj S, et al. 2022). In vitro anticancer and antibacterial activity of green synthesized zno nps using Clitorea ternatea flower extract: inhibits MCF-7 cell proliferation via intrinsic apoptotic pathway.
- Das G, Seo S, Yang IJ, et al. Synthesis of biogenic gold nanoparticles by using sericin protein from Bombyx mori silk cocoon and investigation of its wound healing, antioxidant, and antibacterial potentials. IJN. 2023;ume 18:17–34.) doi: 10.2147/IJN.S378806.
- El-Sayed ESR, Mansour DS, Morsi RM, et al. Gamma irradiation mediated production improvement of some myco-fabricated nanoparticles and exploring their wound healing, anti-inflammatory and acetylcholinesterase inhibitory potentials. Sci Rep. 2023;13(1):1629. doi: 10.1038/s41598-023-28670-5.
- Malathi S, Balashanmugam P, Devasena T, et al. Enhanced antibacterial activity and wound healing by a novel collagen blended ZnO nanoparticles embedded niosome nanocomposites. J Drug Delivery Sci Technol. 2021;63:102498. doi: 10.1016/j.jddst.2021.102498.