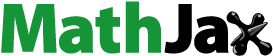
Abstract
Pancreatic ductal adenocarcinoma (PDAC) is one of the leading causes of cancer-related death. Therefore, we intend to explore novel strategies against PDAC. The exosomes-based biomimetic nanoparticle is an appealing candidate served as a drug carrier in cancer treatment, due to its inherit abilities. In the present study, we designed dasatinib-loaded hybrid exosomes by fusing human pancreatic cancer cells derived exosomes with dasatinib-loaded liposomes, followed by characterization for particle size (119.9 ± 6.10 nm) and zeta potential (−11.45 ± 2.24 mV). Major protein analysis from western blot techniques reveal the presence of exosome marker proteins CD9 and CD81. PEGylated hybrid exosomes showed pH-sensitive drug release in acidic condition, benefiting drug delivery to acidic cancer environment. Dasatinib-loaded hybrid exosomes exhibited significantly higher uptake rates and cytotoxicity to parent PDAC cells by two-sample t-test or by one-way ANOVA analysis of variance, as compared to free drug or liposomal formulations. The results from our computational analysis demonstrated that the drug-likeness, ADMET, and protein-ligand binding affinity of dasatinib are verified successfully. Cancer derived hybrid exosomes may serve as a potential therapeutic candidate for pancreatic cancer treatment.
Introduction
Pancreatic ductal adenocarcinoma (PDAC) is a refractory malignant tumour with a 5-year survival rate of about 11–12%, mainly due to failure of early diagnosis, aggressive tumour biological behaviour and insensitivity towards conventional therapies [Citation1–3]. Therapeutic resistance in PDAC is a prominent clinic challenge, partly due to its complex microenvironment consisting of unique components, including dense desmoplastic stroma (e.g. abundant pancreatic stellate cell) and compressed blood vessels to form biological and physical barriers that restrict drug delivery [Citation1,Citation4]. Therefore, there are still unmet medical needs to explore novel drug delivery strategies or targeted therapeutic agents against pancreatic cancer.
Exosomes are natural nanosized carriers (30–100 nm) secreted by almost all cell types under physiological or pathological conditions [Citation5,Citation6]. They are involved in intracellular communication by shuttling biomolecules (e.g. transcription factors, surface protein and nucleic acid) to target cells in an autocrine, paracrine and (or) endocrine manner [Citation7,Citation8]. Exosomes-based nancarriers display excellent biocompatibility, low immunogenicity, desired biological barriers permeability and targeted homing ability. However, the low yield and drug-loading efficiency of exosomes limit their potential application as a drug delivery platform. Recently, hybrid exosome-based nanocarriers, such as, exosome–nanoparticle (e.g. gold, magnetic, Metal-Organic Framework, porous silicon, Pt, mPEG-PLGA) [Citation9–14] hybrid structure, exosome–liposome hybrid structure [Citation15], are emerging as promising drug delivery systems. Exosome–liposome hybrid exosomes is to take advantages of both exosomal and liposomal drug delivery system, overcoming their respective shortcomings. A few studies have demonstrated the improvement of stability, targeting ability and efficiency for hybrid exosomes delivery system. For example, Sato et al. constructed engineering hybrid exosomes by membrane fusion with exosomes from RAW264.7 macrophages and several types of phospholipid-based liposomes, and evaluated their targeting ability to cervical cancer cells [Citation16]. Lin et al. developed a kind of hybrid exosomes with liposomes and HEK293FT cell-derived exosomes via simple incubation for delivering CRISPR–Cas9 system in the mesenchymal stem cells [Citation17]. Rayamajhi et al. hybridized exosomes derived from mouse macrophage J774A.1 with synthetic liposome to exploit the tumour targeting characteristic of macrophage [Citation18]. Sun et al. designed a clodronate-loaded liposome and murine fibroblast L-929 cells-derived exosome hybrid drug delivery system with fibroblast homing properties for the treatment of pulmonary fibrosis [Citation19]. Piffoux et al. produced hybrid exosomes with liposome and exosome derived from murine mesenchymal stem cells (MSC) or human umbilical vein endothelial cells (HUVEC) to improve cellular uptake [Citation20]. However, these researches on hybrid exosomes are mainly focused on exosomes secreted from normal cell lines, such as macrophages, fibroblast, MSC, HUVEC or human HEK293FT cells. Few papers have addressed the use of hybrid exosomes derived from cancer cells. And none of the studies have been done to construct drug-loaded hybrid exosomes derived from human tumour cells for pancreatic cancer therapy. The aim of using cancer cells as exosomes source is to exploit the tumour targeting characteristic of hybrid exosomes derived from cancer cells. Therefore, there is a need for developing cancer cells-derived hybrid exosomes for antineoplastic agents (such as dasatinib) delivery.
The role of activated Cdc42-associated kinase (ACK1) activation in pancreatic cancer progression and survival suggests the promising clinical effects of utilizing an ACK1 inhibitor against pancreatic cancer [Citation21,Citation22]. Dasatinib, as a type of ACK1 inhibitor, is a multitargeted tyrosine kinase inhibitor which more recently has been used as a potent anti-pancreatic cancer agent. The efficiency of dasatinib has been examined as monotherapy or combination therapies in pancreatic cancer metastases inhibition, enhancing anti-proliferative effects, inducing apoptotic cell death, and reversing drug resistance [Citation23–25]. However, the conventional route of dasatinib delivery is hampered by low aqueous solubility, pH-dependent absorption, short half-life (3–4 h), and low specificity related adverse effects, which may lead to having poor therapeutic outcome [Citation26,Citation27]. To circumvent the above-mentioned issues, employing nanotechnology in dasatinib delivery may be a potential solution for therapeutic improvements.
In this study, exosomes derived from human pancreatic cancer cells were hybridised with dasatinib-loaded liposomes via repeated freeze-thaw cycles to formulate hybrid exosomes, and subsequently used them as novel drug carriers for dasatinib delivery. We then evaluated the membrane fusion of exosomes with liposomes, characterized their physicochemical properties, examined their tumour targeting ability and in vitro anti-pancreatic cancer activity. Our study will provide an experimental basis for hybrid exosomes development and application for PDAC treatment.
Materials and methods
Materials
Dulbecco’s Modified Eagle’s Medium (DMEM), RPMI 1640, phosphate buffered saline (PBS), 0.25% trypsin, Penicillin-Streptomycin were purchased from Macgene (China), foetal bovine serum (FBS) was purchased from Gibco (Grand Island, NY, USA), Exosome depleted FBS was purchased from VivaCell (Shanghai, China). Egg phosphatidylcholines (EPC), cholesterol, coumarin 6, DMSO were purchased from Sigma-Aldrich. NBD-DMPE, rho-DMPE, DiD were purchased from Thermo Fisher Scientific. Dasatinib was purchased from MedChemExpress. SephadexG-50 were obtained from GE Ltd. Methanol and chloroform were purchased from Tongguang fine chemical company (Beijing). Antibody CD9, CD81 and GAPDH were purchased from Proteintech (Rosemont, IL, USA).
Preparation of dasatinib-loaded liposomes
Dasatinib liposomes were prepared by thin lipid film hydration followed by sonication and extrusion [Citation28]. Briefly, EPC, cholesterol, PEG2000-DSPE and dasatinib were dissolved in the mixed solvent of chloroform and methanol (3:1 v/v) at a molar ratio of 66:18:2.5:3.5. The solvent was evaporated at 40 °C and the resultant dried lipid film was resuspended in 5% glucose. The mixture was sonicated for 6 min in an ice water bath, followed by sequential extrusion through 400 nm and 200 nm polycarbonate membranes three times. The unentrapped dasatinib was removed from the liposome dispersion by a SephadexG-50 column.
Exosome isolation
Exosomes were isolated from the supernatant of human pancreatic cancer cell lines—Panc0403 and HuP-T3, which were kindly provided by Professor Wang (Mayo Clinic, USA), by combining ultracentrifugation with ExoQuick precipitation methods [Citation29]. In brief, Panc0403 cells were cultured in DMEM containing 10% FBS and 1% penicillin-streptomycin, while HuP-T3 cells were maintained in RPMI-1640 supplemented with 10% FBS and 1% penicillin-streptomycin. All cell lines were grown to 60% confluency in 150 mm2 culture dishes, before the medium was replaced with exosome-free serum media for a further 48 h. The supernatant was collected and centrifuged at 2000 × g for 10 min and 12,000 × g for 30 min, followed by ultrafiltration through a 30 kDa membrane. The exosome-enriched solution was mixed well with the appropriate volume of ExoQuick (1:5 v/v) by inverting the tube gently and refrigerated it overnight. The mixture was then collected and centrifuged twice at 1500 × g for 30 and 5 min, respectively. The precipitate was re-suspended in 1 × PBS and stored at 4 °C. Subsequently, the protein concentration of exosomes was determined via BCA assay.
Preparation of dasatinib-loaded hybrid exosomes
The hybrid exosome was prepared by membrane fusion through a repeated freeze-thaw method as described before [Citation16]. 300 μg/mL exosome was mixed with an equal volume of dasatinib liposomes (100 μmol/L). The mixture was frozen in liquid nitrogen and thawed at room temperature for 15 min. The freeze–thaw cycle was repeated for 10 times. The hybrid exosomes were stored at 4 °C.
Preparation of coumarin 6-loaded hybrid exosomes
Coumarin 6 was used as the fluorescent probes to label liposomes or hybrid exosomes [Citation19]. Coumarin 6 liposomes (lipids: coumarin = 200:1, w/w) or hybrid exosomes were prepared as the same procedures with those of dasatinib liposomes or hybrid exosomes.
Characterisation of hybrid exosomes
The measurements of particles size, polydispersity index (PDI) and zeta potential were performed with Nano-ZS 90 (Malvern, UK). Dasatinib was measured by an ultraviolet spectrophotometer (UV-Vis-2550, Shimadzu, Japan). To estimate the content of dasatinib encapsulated in the hybrid exosomes, the hybrid exosomes were destroyed by methanol. After centrifugation, the absorbance of the supernatant was determined via UV spectrum at 322 nm. The encapsulation efficiency (EE) and drug-loading capacity (DL) were calculated via the following equations [Citation18]:
(1)
(1)
(2)
(2)
where W1 means the mass of encapsulated dasatinib in hybrid exosomes, Wt means the mass of feeding dasatinib and W*means the total mass of dasatinib hybrid exosomes.
Western blot analysis
Cancer cells, purified exosomes or hybrid exosomes were lysed with RIPA buffer and quantified with the BCA assay. Specific antibodies were used to examine protein expression. Samples protein (20 μg) was electrophoresed by SDS-PAGE and transferred onto PVDF membranes as described previously [Citation17]. Membranes were blocked with 5% non-fat milk in PBS-containing 1% Tween-20 (PBST) for 2 h, and incubated with anti-rabbit CD9, anti-mouse CD81 and anti-mouse GAPDH antibodies at 4 °C overnight. Then, the membranes were washed with PBST, and incubated with HRP-conjugated secondary antibodies for 1 h at room temperature. Protein bands were developed and detected with chemiluminescence.
Fluorescence resonance energy transfer assay
The fusion efficiency between liposomes and exosomes was quantified as the lipid mixing ratio by fluorescence resonance energy transfer (FRET) assay as described previously [Citation16]. Liposomes labelled with 1 mol%NBD-DMPE and rho-DMPE (1:1 v/v) were fused with exosomes through the freeze-thaw method, and the fluorescence of the mixture was measured by the SpectraMax ID5 multimode microplate reader (Molecular Devices, USA). Excitation of NBD-DMPE at 460 nm induces fluorescence emission of NBD-DMPE (530 nm) and rho-DMPE (588 nm). The fluorescence intensity at 530 nm increase while that at 588 nm decrease as a result of membrane fusion-related rho-DMPE dilution. The fusion efficiency was evaluated using calibration samples with known fluorescence intensities. The efficiency for FRET dilution (EFD) of liposome-exosome was defined as follow:
(3)
(3)
where F530 and F588 represent the fluorescence intensity at 530 nm and 588 nm, respectively.
Total internal reflection fluorescent microscope technique
Since dasatinib does not have autofluorescence, hence coumarin 6 was used as the fluorescent probes to label liposomes or hybrid exosomes to observe the membrane fusion with the same preparation process as dasatinib’s. The total internal reflection fluorescent microscope (TIRFM) technique was used to imaged and analysed membrane fusion between exosomes and liposomes to further confirm the results shown in the FRET assay. 300 μg/mL exosomes was mixed with 10 μmol/L DiD (100:1 v/v) at 37°C for 30 min to obtain the DiD-labelled exosomes, which then fused with coumarin 6 loaded liposomes (100 μmol/L) at 1:1 volume ratio via the freeze-thaw method. The fluorescence-labelled liposomes and exosomes were observed under TIRFM (LEICA Microsystems, Wetzlar, Germany). Images processing were conducted by Image J software.
Drug release in vitro
The profile of dasatinib release from hybrid exosomes and liposomes in phosphate-buffered saline (PBS) buffer at pH 7.4 and 5.5 were determined, respectively [Citation18]. Briefly, dasatinib (1.5 ml of 30 µg/mL) encapsulated in hybrid exosomes or liposomes was placed in a dialysis bag (12,000–14,000 molecular weight cutoff). The dialysis bags were sealed and then immersed into 10 ml of release medium with constant shaking at 100 rpm at 37 °C. A volume of 0.2 ml release medium was taken at predetermined time intervals and replaced with an equal volume of respective buffers. The concentration of released dasatinib was determined at 322 nm by Cytation 1 microplate reader (BioTek, UK). The absorbance was translated to dasatinib concentration using a standard curve prepared through serial dilutions of dasatinib. The cumulative dasatinib release rate was quantified by using the Equationformula (4)(4)
(4) . Each experiment was repeated for triplicate.
(4)
(4)
where m1 represents the amount of dasatinib in release media, while mtotal means the amount of dasatinib in 100% release.
Cellular uptake
To evaluate the intracellular uptake of the hybrid exosomes, flow cytometry and cell imaging analysis were performed. Due to the lack of autofluorescence in dasatinib, coumarin 6 was used as the fluorescent probes to label liposomes or hybrid exosomes. Coumarin 6, coumarin 6 liposomes, coumarin 6 hybrid exosomes are used to replace dasatinib, dasatinib liposomes and dasatinib hybrid exosomes, respectively. The differences in fluorescence intensities observed on the flow cytometer reflect the uptake of fluorescent particles in cells, and also indirectly represent the relative cellular uptake of dasatinib loaded particles. For flow cytometry analysis [Citation30], HuPT-3 and Panc0403 cells in the logarithmic growth phase were counted and seeded into 6-well plates at a density of 4 × 105cells/well. Cells were grown to confluency at 37 °C, in a 5% CO2 incubator for 24 h. PBS, coumarin 6, coumarin 6 liposomes, coumarin 6 hybrid exosomes diluted with medium (final concentration of coumarin 6 was 0.2 μg/mL or 0.02 μg/mL) were incubated with cells for 4 h. The cells were then washed, digested and collected for coumarin 6 analysis (ACEA Bio, China).
Cell imaging analysis
To detect intracellular accumulation and distribution, 1 × 105 of cells were seeded into a 24 well plate and incubated with coumarin 6, coumarin 6 liposomes, coumarin 6 hybrid exosomes at a concentration of 0.2 μg/mL coumarin 6 for 4 h. Thereafter, the cells were washed with PBS three times and fixed with 4% paraformaldehyde (v/v) at room temperature for 30 min. Cell nuclei were then stained with DAPI (5 μg/mL) for 10 min. Finally, the cells were washed and the images were obtained by using the Cytation 1 automatic microplate reader (BioTek, USA) under the GFP channel [Citation19].
Cell proliferation MTS assay
HuPT-3 and Panc0403 cells were seeded into 96-well plates at a density of 6 × 103 cells/well and allowed to attach overnight. Cells were then subjected to the treatment of dasatinib, dasatinib liposomes and dasatinib hybrid exosomes at indicated concentrations. After 72 h, 20 μL of MTS tetrazolium reagent was added to each well and incubated for another 2 h by using the MTS Assay Kit (CellTiter 96 AQueous One Solution Cell proliferation assay, Promega Corporation, USA) according to the manufacturer’s manuals. The absorbance was determined at 490 nm by ELx800 reader (BioTek, UK) [Citation31]. The inhibition effect was calculated by Equation (5). All experiments were performed in triplicate.
(5)
(5)
Cell apoptosis assessment
Flow cytometry was used to determine the apoptosis of HuPT-3 and Panc0403 cells [Citation32]. Briefly, Cells (4 × 105) were seeded in 6-well plates and treated with dasatinib, dasatinib liposomes and dasatinib hybrid exosomes at a concentration of 1 µM dasatinib for 24 h. Subsequently, detached and attached cells were collected and washed twice with ice-cold PBS. Cells were stained by Annexin V-FITC/PI apoptosis detection kit according to the manufacturer’s suggestion. Then the samples were detected by flow cytometry. Both Annexin V (+) cells were regarded as apoptotic cells.
Molecules property calculation related to lipinski’s rules and ADMET
A total of 91 molecular properties were calculated and predicted by ADMETlab 2.0 for drug-likeness and ADMET (Absorption, Distribution, Metabolism, Excretion, and Toxicity) estimation [Citation33]. Lipinski’s rules were adopted as the main criteria for drug-likeness estimation [Citation34], including: 1). Molecular weight is lower than 500 Da; 2). Number of hydrogen acceptor is lower than 10; 3). Number of hydrogen donor is lower than 5; 4). logP is lower than 5.
Molecular docking
Autodock Vina [Citation35] was adopted as the molecular docking procedure to discover the optimum protein-ligand binding structure and poses for the ACK1- dasatinib complex. The 3D structure of ACK1 was adopted from the PDB database (PDB ID: 4HZR) [Citation36]. The initial 3D structure of dasatinib was calculated by the RDKit package [Citation37]. The docking grid box, the exhaustiveness and the number of sampled poses were set as 60, 100 and 20, respectively. Vina score of the top 1 binding pose was reported representing the binding free energy. Then, PyMol [Citation38] was used to visualize the protein-ligand complex with 3D structures. LigPlot+ [Citation39] was adopted to analyse contacts and interactions between ligand structures and protein residues.
Statistical analysis
All data were presented as mean ± SD of three independent experiments. SPSS software was used to perform statistical analysis. A paired-samples t-test was applied for statistical analyses between two groups, one-way ANOVA with Bonferroni Correction for post hoc analysis was used for multiple comparisons. p < .05 was considered statistically significant.
Results
Preparation and characterisation of hybrid exosomes
To improve drug delivery to pancreatic cancer, we prepared dasatinib-loaded hybrid exosomes by fusing human pancreatic cancer cells derived exosomes with dasatinib-loaded liposomes (). Liposomes, exosomes, and hybrid exosomes were characterised for size, surface property, and protein expression. The average size of liposomes, exosomes and hybrid exosomes were approximately 118.9 nm, 106.47 nm and 119.90 nm, respectively (). The particle size of hybrid exosomes was similar to that of liposomes, indicating that hybridisation of liposomes and exosomes did not alter the size of hybrid exosomes. As shown in , the zeta potential of liposomes, exosomes and hybrid exosomes were approximately −7.43 mV, −0.66 mV and −11.45 mV, respectively, indicating that hybrid exosomes exhibited enhanced stability. The encapsulation efficiency (EE) of dasatinib hybrid exosomes is 88.70 ± 1.53%, and drug-loading capacity (DL) is 2.16 ± 0.04%, which illustrated that dasatinib was well incorporated into hybrid exosomes. Coumarin 6 was used as the fluorescent probes to label hybrid exosomes. The encapsulation efficiency of coumarin 6 hybrid exosomes is 99.16 ± 0.29%, and the drug-loading capacity (DL) is 0.31 ± 0.05%. Because of the low ratio of coumarin 6 and lipid (lipids:coumarin = 200:1, w/w), the encapsulation rate of coumarin 6 in hybrid exosomes is higher than that of dasatinib. The protein expression of cells, exosomes and hybrid exosomes was analyzed in the Western blot assay. As shown in , the presence of CD9 and CD81 in those two types of exosomes suggests that we successfully extracted exosomes from cell culture supernatant. Hybrid exosomes display similar protein bands to that of exosomes, indicating the retention of exosomal biomarkers after hybridisation, confirming the presence of exosomes in hybrid exosomes. The obtained hybrid exosomes are relative stable at 4 °C for more than 15 d without obvious deposition.
Figure 1. Schematic representation of dasatinib-loaded hybrid exosome construction. Exosomes were collected from cell culture supernatant after 48 h by combination ultracentrifugation with ExoQuick precipitation method. Dasatinib liposomes were prepared by thin film lipid hydration followed by sonication and extrusion. Exosomes were fused with dasatinib-liposomes via 10 freeze-thaw cycles.
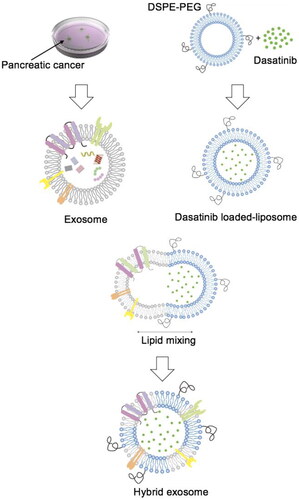
Figure 2. Characterisation of hybrid exosomes. The particle sizes (A) and zeta potentials (B) of liposomes, exosomes, and hybrid exosomes. Data were expressed as the mean ± SD (n = 3), *p < .05. (C) Protein expressions of CD9, C81 and GAPDH in cells, exosomes and hybrid exosomes derived either from Panc0403 or HuP-T3 in Western blot analysis. Images are representative of three independent experiments.
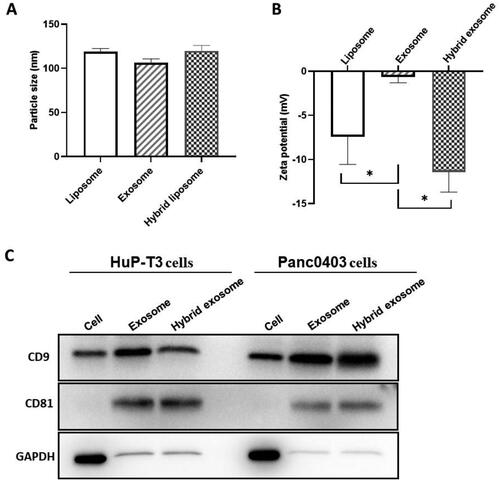
Confirmation of membrane fusion between exosomes and liposomes
First, we perform TIRFM to directly visualise the lipid merging of DiD-labelled exosomes with coumarin 6-loaded liposomes. As shown in , coumarin 6 incorporated liposomes are green, while DiD-labelled exosomes are red. The merged orange fluorescence indicates colocalization of exosomes and liposomes, confirming the membrane fusion between these two vehicles.
Figure 3. Evaluation of membrane fusion of exosomes with liposomes. (A) Colocalization of cancer-derived exosomes and liposomes in hybrid exosomes by total internal reflection fluorescence microscopy (TIRFM). The green fluorescence indicates coumarin 6-labelled liposomes, red fluorescence indicates DiD-labelled exosomes extracted from HuP-T3 and Panc0403 cells respectively, and orange merged fluorescence indicates colocalization of exosomes and liposomes. Images are representative of three independent experiments. Scale bar, 1 μm. (B) The fusion curve of hybrid exosomes derived from HuP-T3 cells and Panc0403 cells by fluorescence resonance energy transfer (FRET) assays followed with (C) Quantitative analysis of FRET efficiency for the blank liposome and hybrid exosomes. Lipid dilution ratio is used to define the membrane fusion efficiency. The lipid dilution ratio of labelled liposomes is calculated through standard curve created by plotting FRET efficiency (EFD) vs lipid dilution ratio. Liposomes labelled with 1 mol%NBD-DMPE and rho-DMPE (1:1 v/v) was fused with exosomes though freeze-thaw method, and the fluorescence intensities of the mixture were analysed at 530 and 588 nm. Data were expressed as the mean ± SD (n = 3), ***p < .001.
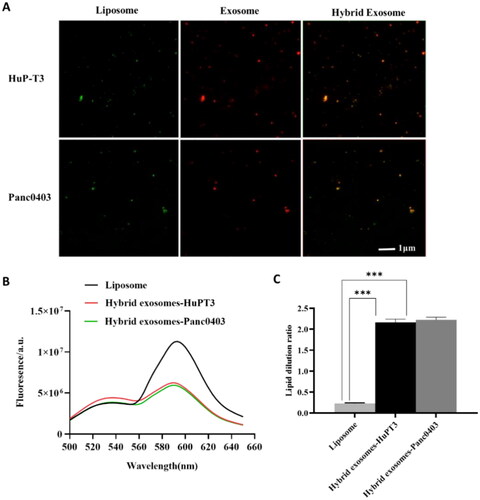
Next, we perform a FRET assay to quantitatively measure the membrane fusion efficiency. Generally, the lipid dilution ratio is used to evaluate membrane fusion. The equation for the calibration curve plotted by EFD vs lipid dilution ratio is given as: y = 0.0543x + 0.2451, R2=0.9902. Fusion 1 mol%NBD-DMPE and rho-DMPE (1:1 v/v) labelled liposomes with unlabelled exosomes result in fluorescence at 530 nm increase while that at 588 nm decrease compared with unfused liposomes due to the fluorescent lipid dilution (). The lipid dilution ratio of samples was calculated from the linear regression, and the results are shown in . Under repeated freeze-thaw cycles, those two origins of exosomes had similar membrane fusion efficiency with 2.17 and 2.23 for HuPT-3 and Panc0403, respectively. The difference of membrane fusion efficiency between different cells derived exosomes and liposomes is unknown at present, we speculated that this may be related to the complexity and composition difference of exosome membrane, such as the activity of highly specified fusogenic transmembrane proteins.
Drug release kinetics from hybrid exosomes
The in vitro dasatinib release behaviour from liposomes and hybrid exosomes was investigated in pH 7.4 and pH 5.5 PBS at 37 °C (). Results show that Panc0403 and HuP-T3 cells derived hybrid exosomes displayed similar accumulative release behaviour. Dasatinib hybrid exosomes showed burst release in both pH conditions up to the first 8 h. After the initial burst release, sustained drug release was observed up to 96h. As a result, enhanced drug release characteristic was detected in acidic condition (pH 5.5) when compared to that in physiological condition (pH 7.4). The hybrid exosomes from Panc0403 and HuP-T3 cells released 76.44% and 91.60% of the drug in the acidic environment after 96 h incubation, when compared to 61.36% and 68.97% drug release in a normal physiological condition, respectively. The release rates of dasatinib from both liposomes and hybrid exosomes were higher under acidic pH conditions (pH 5.5) than that under physiological pH conditions (pH 7.4). This pH-sensitive drug release in acidic condition benefitted drug delivery to acidic cancer environment.
Figure 4. In vitro release kinetics of dasatinib from liposome, HuPT3-derived hybrid exosomes (A) and Panc0403-derived hybrid exosomes (B) in PBS buffer with pH 5.5 and pH 7.4 at 37 °C. At each predetermined interval, 200 μl of each sample was determined for absorbance detection at 322 nm and replaced with equal volume of respective buffers. The absorbance was translated to dasatinib concentration using a standard curve prepared through serial dilutions of dasatinib, followed by quantification of cumulative dasatinib release rate. Each experiment was repeated for triplicate.
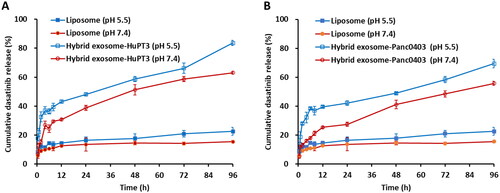
Efficient cellular uptake of hybrid exosomes by their parent cells
To determine the effect of origins of hybrid exosomes on cellular uptake, we fluorescently labelled hybrid exosomes with coumarin 6, incubated them with donor and recipient cells, and then compare the fluorescence intensity inside cells. The results suggest that HuP-T3 cells derived hybrid exosomes are taken up at greater quantities by their parent cells than recipient cells, indicating that exosomes have a specific tropism, according to their characteristics and cell origin (Left panel of ). A similar uptake tendency is observed in Panc0403 cell that more autologous hybrid exosomes were accumulated into donor cells compared with allogeneic hybrid exosomes (Right panel of ). Our findings preliminarily demonstrated cancer cell-type tropism in these two types of cancer-derived hybrid exosomes, and thus we intended to utilise autologous exosomes in further uptake and cytotoxicity assay.
Figure 5. Analysis cellular uptake of hybrid exosomes in HuP-T3 cells (left panels) and Panc0403 cells (right panels) by flow cytometry. Coumarin 6 is used as fluorescence probe to determine uptake ratio. Treated with different types of formulations for 4 h, two cell lines were collected for FITC detection. The influences of PEG modification and cellular heterogeneity on cellular uptake after incubated different types of hybrid exosomes in HuP-T3 (Left panels of ) and Panc0403 (Right panels of ) cells. The cellular uptake efficiency of various coumarin 6 formulations in HuP-T3 (Left panel of ) and Panc0403 (Right panel of ) cells. NEG means negative control, H means HuP-T3 cells, P means Panc0403 cells. Data were expressed as the mean ± SD (n = 3). *p < .05, **p < .01, ***p < .001.
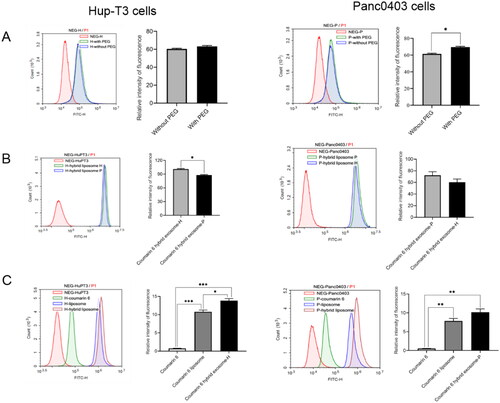
Next, we investigated the influence of PEGylation on hybrid exosomes uptake in those two cell lines with a final concentration of coumarin 6 at 0.2 μg/mL. In HuP-T3 cells, the addition of PEG did not influence the uptake level of hybrid exosomes (p > .05) (Left panel of ), while in Panc0403 cells, a significantly high level of internalization could be observed when hybrid exosomes were functionalized with PEG (p < .05) (Right panel of ). As PEGylation did not compromise the in vitro uptake in our study, hybrid exosomes were modified with PEG to improve their stability and increase circulation time in the subsequent experiments.
To gain more insight into specific cell uptake, a comparative analysis was conducted to investigate the uptake efficiency of different formulation vehicles, which had a finial coumarin 6 concentration of 0.02 μg/mL. The results showed the order of vesicle uptake by HuP-T3 cells is: coumarin 6 hybrid exosome > coumarin 6 liposome > free coumarin 6 > blank control (Left panel of ). The overall uptake benefits of Panc0403 cells-based hybrid exosomes (p = .1) were similar to those found in hybrid exosomes fabricated from HuP-T3 cells (Right panel of ). These results emphasize that hybrid exosomes exhibit higher uptake in cancer cells than liposomes.
Hybrid exosomes show apparently intracellular accumulation in cytoplasm
The intracellular accumulation and distribution of hybrid exosomes was observed by using a Cytation 1 fluorescent microscope. The fluorescence intensity of the coumarin 6 in the HuP-T3 and Panc0403 cells was used as an indicator for the cellular uptake of different coumarin 6 formulations. show the fluorescent microscopic images of HuP-T3 and Panc0403 cells at 4 h after applying free coumarin 6, coumarin 6 liposomes and coumarin 6 hybrid exosomes, respectively. The strongest green fluorescence of coumarin 6 hybrid exosomes were observed in cytoplasm as compared to those of coumarin 6 liposomes, free coumarin 6. The result from apparently indicates that hybrid exosomes mainly locate into the cytoplasm of HuP-T3 and Panc0403 cells with their better intracellular accumulation, which is consistent to the quantitative results from the above cellular uptake (as shown in ).
Figure 6. Representative images of HuP-T3 cells (A) and Panc0403 cells (B) treated with free coumarin 6, coumarin 6 liposomes and coumarin 6 hybrid exosomes, respectively. After incubation with the various coumarin 6 formulations for 4 h, the cells were stained with DAPI and imaged by Cytation 1 automatic microplate reader. Blue colour indicates the nucleus of cells stained with DAPI. Green colour shows the drug in HuP-T3 cells or Panc0403 cells after applying various coumarin 6 formulations. The fluorescence intensity of the coumarin 6 in the HuP-T3 and Panc0403 cells was quantified using ImageJ software. Scale bar: 100 μm. Data were expressed as the mean ± SD (n = 3). *p < .05.
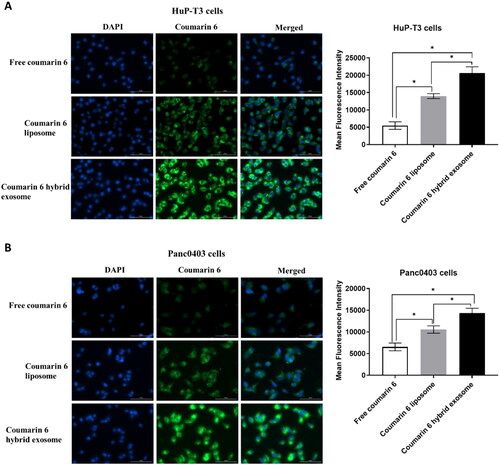
Dasatinib hybrid exosomes inhibit tumour cell proliferation and induce apoptosis
To test if higher dasatinib delivery efficiency could translate in a potentially higher cell toxicity, we evaluated the inhibitory effect of different dasatinib formulations on the growth of HuP-T3 and Panc0403 cells by MTS assays. As shown in , all three dasatinib formulations inhibited cell proliferation of HuP-T3 and Panc0403 cells in a dose-dependent manner. The viability of HuP-T3 cells treated with all those three formulations was substantially lower than that of Panc0403 cells, suggesting that HuP-T3 cells are more sensitive to dasatinib than Panc0403 cells. Dasatinib hybrid exosomes showed significant higher inhibitory effects on both HuP-T3 and Panc0403 cell growth as compared to dasatinib alone or its liposomal formulation when the concentration of dasatinib is higher than 0.123 μmol/L (p < .05). The cytotoxicity of those dasatinib formulations in both cell lines follows the order of dasatinib hybrid exosomes > dasatinib liposomes > dasatinib. The enhanced cytotoxicity was a result of higher cell internalization of hybrid exosomes compared to liposomes, which adds another advantage of using hybrid exosomes as a carrier for therapeutic cargoes delivery.
Figure 7. Effects on PDAC cell proliferation and apoptosis treated with different dasatinib formulations in vitro by MTS assay and flow cytometry analysis, respectively. Inhibitory proliferation detection of HuP-T3 cells (A) and Panc0403 cells (B) treated with different dasatinib formulations by MTS assay. These two types of cell lines were treated with free dasatinib, dasatinib liposomes and dasatinib hybrid exosomes at the concentration of 0.00137, 0.0137, 0.0412, 0.123, 0.37, 1.11, 3.3, 10 and 20 μmol/L dasatinib for 72 h, followed by incubating MTS agent for 2 h, respectively. The absorbance was determined at 490 nm. Data expressed as means ± SD (n = 3). *p < .05, indicates the comparison of dasatinib hybrid exosomes to dasatinib liposomes; # p < .05, indicates the comparison of dasatinib hybrid exosomes to dasatinib. Apoptosis assessment of HuP-T3 cells (C) and Panc0403 cells (D) treated with varying dasatinib samples at 1 μmol/L of dasatinib concentration for 24h by flow cytometry analysis. Data are shown as the mean ± SD. a, p < .05, compared with control; b, p < .05, compared with dasatinib group; c, p < .05, compared with dasatinib liposome group.
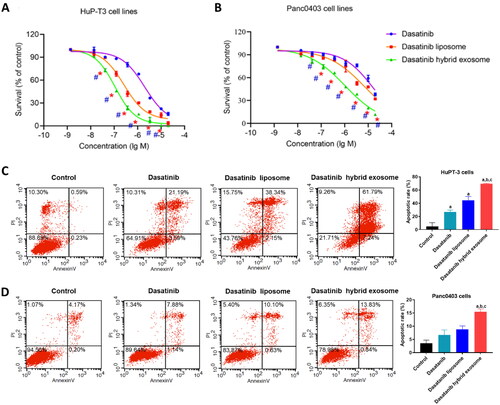
The therapeutic efficiency of dasatinib hybrid exosomes was further confirmed by cell apoptosis assay ()). The cell apoptosis rates of HuP-T3 cells and Panc0403 cells treated with dasatinib hybrid exosomes were 69.53 ± 0.70% and 15.43 ± 1.07%, respectively, which were significantly higher than that of treatment with dasatinib and dasatinib liposomes. The above results indicated that the fusion of the exosomes into liposomes enhanced the targeting capability to HuP-T3 cells and Panc0403 cells, promoting cellular uptake of dasatinib-loaded hybrid exosomes, thereby resulting in greater cytotoxicity.
Drug-likeness, ADMET, and protein-ligand binding affinity of dasatinib are verified by computational methods
To provide a landscape of the molecular properties and the drugability of dasatinib, the physicochemical and ADMET properties were calculated by ADMETlab2.0 as listed in and . Regarding the drug-likeness of dasatinib, Lipinski’s rules were as the main criteria and the related molecular properties were listed in , suggesting that all required properties of dasatinib obey the rules. Meanwhile, most of the other drug-likeness criteria calculated by ADMETlab 2.0 in also acknowledge the availability of dasatinib such as the Pfizer Rule, Golden Triangle, PAINS, BMS Rule, and Chelator Rule.
Table 1. Determination of drug likeness of dasatinib by lipinski’s rules.
Table 2. The physicochemical and ADMET properties of dasatinib.
The ADMET properties of dasatinib have been predicted with considering as below: (1) Absorption: the predicted values showed that dasatinib could be well absorbed with good bioavailability and permeability. (2) Distribution: the therapeutic index of dasatinib may be low as the plasma protein binding possibility is high, and it is less possible for dasatinib to pass the blood-brain barrier. (3) Metabolism: dasatinib is less likely to bind with CYP450 enzymes which could slow down the drug metabolism. (4) Excretion: results showed that dasatinib could have a short half-life and low clearance. (5) Toxcity: there are potential toxicities on specific targets for dasatinib, including hERG Blockers, human hepatotoxicity, drug-induced liver injury, carcinogenicity, respiratory Toxicity, and several nuclear hormone receptor toxicities.
Hence, to discover the chemical structural evidence that support the finding of interaction and relation between dasatinib and ACK1, molecular docking via Autodock Vina was applied to explore the optimum binding pose for dasatinib – ACK1 complex. The chemical structure of dasatinib and ACK1 were shown in , together with the structure of molecular docking simulated dasatinib - ACK1 complex (). The Vina score of the top 1 binding pose is −8.251 (kcal/mol), indicating that there is strong binding bioactivity of the dasatinib – ACK1 complex. Then, PyMol and LigPlot + were adopted to visualise the binding modes in 2D () and 3D spaces (). The overall results showed that there is a hydrogen contact between dasatinib and Asp134 (A) and extensive hydrophobic interactions, which inferred the interaction of dasatinib and ACK1 and implied the potential bioactive site of ACK1. Therefore, the results from our computational analysis demonstrated that the drug-likeness, ADMET, and protein-ligand binding affinity of dasatinib are verified successfully.
Figure 8. Interaction mode between dasatinib and ACK1 proposed by molecular docking analysis. (A) Chemical structure of dasatinib. (B) Chemical structure of ACK1 (PDB ID: 4HZR). (C) Binding pose of dasatinib – ACK1 complex. (D) Interaction profile of dasatinib – ACK1 complex. (E) Representative structure of dasatinib in the binding site of ACK1 kinase.

Discussion
Due to the frequency of ACK1 dysregulation in PDAC, treatments aimed at disrupting this kinase cascade represents a promising therapeutic target for PDAC. Dasatinib is a multitargeted tyrosine kinase inhibitor as one of the ACK1 inhibitors. However, the use of dasatinib as a monotherapy has failed to demonstrate clinical benefit in patients with metastatic PDAC following a phase II study [Citation40], due to drug resistance in response to the dasatinib treatment. To overcome dasatinib resistance, low aqueous solubility and short half-life, a dasatinib delivery system employing nanotechnology might be a potential solution for therapeutic improvements. In our study, dasatinib showed a stronger inhibitory effect in HuP-T3 cells than in Panc0403 cells, suggesting that HuP-T3 cells are more sensitive to dasatinib than Panc0403 cells. Dasatinib hybrid exosomes showed significant higher inhibitory effects on the growth of HuP-T3 and Panc0403 cells, as compared to dasatinib alone or its liposomal formulation.
In this study, we developed dasatinib hybrid exosomes by fusing the membranes of exosomes derived from cancer cells with dasatinib liposomes. Fusing exosomes with liposomes by 10 freeze-thaw cycles is a relatively simple and cost-effective method to yield fused vehicles with high membrane fusion efficiency, desired physicochemical properties and appropriate protein/lipid ratios, as well as increased cellular uptake [Citation16]. Dehydration and ice growth are regarded as potential driving forces leading to membrane structural and functional alternation, including fusion that eventually generates large vehicles [Citation41–43]. During freezing, ice crystal formation could disrupt membrane integrity and the volume of unfreeze matrix reduction may concentrate the liposome and exosome to a closer proximity that eventually forces them aggregation and fusion [Citation43,Citation44]. Vehicles also could be aggregated by PEG-induced energy barrier reduction [Citation45,Citation46]. Despite of this, another research conclude that contents exchange could occur in the absence of fusion and fission when vehicles were subjected to a freeze-thaw cycle. During the process, vehicles still largely maintain their membrane identity, while content exchange is relied on membrane destabilisation, content leakage and uptake of content from the surrounding phase during the freeze-thaw process [Citation47]. However, it remains to be investigated whether content exchanging is involved in our study.
Results from cellular uptake tests confirmed that hybrid exosomes may retain the association of exosomes with their parent cells, in agreement with other experiment [Citation18]. In general, direct fusion and endocytosis are the main interaction ways for exosome uptake [Citation48,Citation49]. We thus hypothesized that cell-type tropism may be influenced by membranous substances of exosomes derived from their parent cells, for example unique lipid components (e.g. phosphatidylserine, phosphatidylcholine) [Citation50–52], and adhesive molecules (e.g. integrins, immunoglobulins, glycoproteins, tetraspanin webs) [Citation53–56] in in vitro specific uptake. Nevertheless, the selectivity of exosomal uptake is far more complex than mentioned before. Several researches suggested the influence of recipient cells [Citation57], tumour microenvironment pH [Citation58] and cytokines [Citation59] on uptake selection. We speculated that the cell type tropism of hybrid exosomes in our study might be attributed to unique exosomal lipid component and surface proteins derived from parent cancer cells. However, protein functionalities on the hybrid exosomes need to be further clarified due to the negative effect of repeated freeze-thaw cycles. More cell types, such as normal pancreatic cells or other cancer cells, need to be taken into consideration in order to fully understand the selectivity of human pancreatic cancer cells derived hybrid exosomes.
Generally, the extent of cell uptake is reduced upon PEGylation of nanoparticles, since PEG-induced steric hindrance may impair direct interaction of liposomes and cells [Citation60,Citation61]. While in our study, PEG modified hybrid exosomes could improve cellular uptake, at least in HuP-T3 cells, which was consistent with a previous report that unexpectedly high uptake efficiency of PEGylated anionic hybrid exosomes [Citation27]. Potential explanations include electrostatic cell-surface repulsions reduction, modification of PEG-lipids with apparent stability and membrane fusion induction [Citation27,Citation45,Citation62]. The evidence given from cellular uptake may clarify the enhanced cytotoxicity effect of hybrid exosomes-based dasatinib formulation.
The result with high release in dasatinib hybrid exosomes is probably related to high exosomal membrane fluidity at low pH value that make drug leakage from hybrid exosomes [Citation63]. In acidic condition, the aggregation and disruption in the structure of vesicles with increased fluidity render their faster drug release. All in all, higher drug release of hybrid exosomes in the acidic microenvironment is a desirable characteristic for drug delivery agents. As cancer cells are often characterized by an acidic microenvironment, this property may benefit in tumour growth suppression.
Since previous studies have confirmed the round shape of hybrid exosomes after freeze-thaw cylces and identified no significant difference in exosomes morphology before and after membrane fusion [Citation16,Citation64], we didn’t conduct transmission electron microscopy to test the shape and size of hybrid exosomes in the present study. Instead, we confirmed the size and shape of hybrid exosomes by Nano ZS 90 and TIRFM assays. Additionally, underlying mechanisms for exosome-liposome nanocarriers in drug delivery have not yet been fully understood, such formulations need to be further optimized and purified from the mixture through methods like a Nycodenz density gradient, in subsequent studies. Moreover, in order to transfer hybrid exosomes from bench to bedside, biodistribution and hybrid exosomes-biology interaction need to be clarified in healthy and diseased organisms. Therefore, more studies are required to investigate the in vivo efficiency of dasatinib loaded hybrid exosome in animal models and identify the potential immune response. Importantly, cancer-derived exosomes are generally implicated in tumorigenesis and metastasis, it remains necessary to demonstrate the safety of exosome-based formulation thoroughly, although evidence given from Tianyuan et al. suggested that cancer cells would lose tumorgenicity when being rapid immersed in liquid nitrogen [Citation65]. To fully characterise these two cell lines (Panc0403 and HuP-T3 cells) in our study since the exosomes released from PDAC cells, we firstly obtained both mRNA and miRNA expression profiles of the human pancreatic cancer Panc0403 and HuP-T3 cell lines from the database of TCPA-cancer cell lines which is a comprehensive resource generated from The Cancer Proteome Atlas (TCPA), respectively [Citation66]. Secondly, we created a table including their mRNA expression data of those receptors shown in Supplementary Table 1(A), which might be involved in the epithelial mesenchymal transition (EMT), tumour metastases and evade immune surveillance in these two cell lines. Thirdly, we obtained the corresponsive microRNAs data in these two cells (Supplementary Table 1(B)) followed by detecting the search page of the Dependency Map (DepMap) portal via a TargetScanTools (TargetScan Release 8.0) to visualize microRNA expression profiles and retrieve their predicted regulatory targets of human microRNAs [Citation67]. Certainly, more and deeper investigation studies in the future might be helpful to evaluate the effect of tumour cell derivation on drug release and efficacy when using this type of exosome-based delivery system for further development and clinical application.
Importantly, cancer-derived exosomes are generally implicated in tumorigenesis and metastasis, it remains necessary to demonstrate the safety of exosome-based formulation thoroughly. Due to inherent tumour heterogeneity and highly desmoplastic and immunosuppressive tumour microenvironment in PDAC, it will definitely need determine if cancer-derived exosomes home to the tumour of origin and overcome the properties of the adverse microenvironment by testing them in an animal model of PDAC. Future studies will focus on the evaluation of in vivo efficiency and safety of dasatinib loaded hybrid exosome in animal models and identify the potential immune response. To verify our findings more accurately on a wider range of applications, additional chemotherapeutic agents will further be selected to examine the effect of our hybrid exosome delivery system in future studies. Other research focuses to identify and characterise different types of cellular exosomes derived from the tumour tissues, such as fibroblasts, endothelial cells, perivascular cells, or inflammatory cells will also be useful and necessary for their therapeutic applications. Also, scalability and reproducibility should be taken into consideration, which may highlight the importance of synthesis optimization of hybrid exosomes. In addition, techniques limitations may reduce exosome-based nanocarrier ability to specifically target their parent cell line, and therefore, we intend to supplement or enhance the specificity of hybrid exosomes by incorporating targeting moieties in subsequent studies.
Conclusion
In summary, our study demonstrates the feasibility and efficacy of hybrid exosomes used as a drug carrier for cancer treatment with several advantages over other formulations, such as uniform size, enhanced cellular uptake and most importantly, a relative strong anti-cancer effect. Hybrid exosomes may retain the homing ability of exosomes, and thereby delivering dasatinib into cancer cells from which they were derived, leading to better drug accumulation and inhibitory activities in cellular proliferation. Our study demonstrates that hybrid exosomes have the potential as drug carriers to improve anticancer efficacy.
Authors contributions
Zhou XF and Zhuang YT contributed equally to this study; Zhou XF, Zhuang YT, Liu XH, Shi YC, Zhang L, Li R, Zhao YL and Yao HJ performed the experiments;Gu YW, Li J, Wang JT and Chen HB analysed the data; Zhuang YT wrote the manuscript; All authors approved the final version of the manuscript.
Disclosure statement
No potential conflict of interest was reported by the author(s)
Data availability statement
The data that support the findings of this study are available from the corresponding author upon reasonable request.
Additional information
Funding
References
- Siegel RL, Miller KD, Fuchs HE, et al. Cancer statistics, 2022. CA Cancer J Clin. 2022;72(1):7–33. doi: 10.3322/caac.21708.
- Seo YD, Katz MH. Preoperative therapy for pancreatic adenocarcinoma-precision beyond anatomy. Cancer. 2022;128(16):3041–3056. doi: 10.1002/cncr.34273.
- Gaianigo N, Melisi D, Carbone C. EMT and treatment resistance in pancreatic cancer. Cancers. 2017;9(9):122. doi: 10.3390/cancers9090122.
- Yu S, Zhang C, Xie KP. Therapeutic resistance of pancreatic cancer: roadmap to its reversal. Biochim Biophys Acta Rev Cancer. 2021;1875(1):188461. doi: 10.1016/j.bbcan.2020.188461.
- Chen X, Liang H, Zhang J, et al. Secreted microRNAs: a new form of intercellular communication. Trends Cell Biol. 2012;22(3):125–132. doi: 10.1016/j.tcb.2011.12.001.
- Kumar D, Gupta D, Shankar S, et al. Biomolecular characterization of exosomes released from cancer stem cells: possible implications for biomarker and treatment of cancer. Oncotarget. 2015;6(5):3280–3291. doi: 10.18632/oncotarget.2462.
- Lee JE, Moon PG, Lee IK, et al. Proteomic analysis of extracellular vesicles released by adipocytes of Otsuka Long-Evans Tokushima fatty (OLETF) rats. Protein J. 2015;34(3):220–235. doi: 10.1007/s10930-015-9616-z.
- Zhang HG, Grizzle WE. Exosomes: a novel pathway of local and distant intercellular communication that facilitates the growth and metastasis of neoplastic lesions. Am J Pathol. 2014;184(1):28–41. doi: 10.1016/j.ajpath.2013.09.027.
- Khongkow M, Yata T, Boonrungsiman S, et al. Surface modification of gold nanoparticles with neuron-targeted exosome for enhanced blood-brain barrier penetration. Sci Rep. 2019;9(1):8278. doi: 10.1038/s41598-019-44569-6.
- Wang J, Chen P, Dong Y, et al. Designer exosomes enabling tumor targeted efficient chemo/gene/photothermal therapy. Biomaterials. 2021;276:121056. doi: 10.1016/j.biomaterials.2021.121056.
- Cheng G, Li W, Ha L, et al. Self-assembly of extracellular vesicle-like metal-organic framework nanoparticles for protection and intracellular delivery of biofunctional proteins. J Am Chem Soc. 2018;140(23):7282–7291. doi: 10.1021/jacs.8b03584.
- Yong T, Zhang X, Bie N, et al. Tumor exosome-based nanoparticles are efficient drug carriers for chemotherapy. Nat Commun. 2019;10(1):3838. doi: 10.1038/s41467-019-11718-4.
- Sancho-Albero M, Martín-Pardillos A, Lujan L, et al. Exosomes loaded with ultrasmall Pt nanoparticles: a novel low-toxicity alternative to cisplatin. J Nanobiotechnology. 2022;20(1):473. doi: 10.1186/s12951-022-01675-4.
- Mo J, Da X, Li Q, et al. The study of exosomes-encapsulated mPEG-PLGA polymer drug-loaded particles for targeted therapy of liver cancer. J Oncol. 2022;2022:4234116–4234110. doi: 10.1155/2022/4234116.
- Cheng L, Zhang X, Tang J, et al. Gene-engineered exosomes-thermosensitive liposomes hybrid nanovesicles by the blockade of CD47 signal for combined photothermal therapy and cancer immunotherapy. Biomaterials. 2021;275:120964. doi: 10.1016/j.biomaterials.2021.120964.
- Sato YT, Umezaki K, Sawada S, et al. Engineering hybrid exosomes by membrane fusion with liposomes. Sci Rep. 2016;6(1):21933. doi: 10.1038/srep21933.
- Lin Y, Wu J, Gu W, et al. Exosome-liposome hybrid nanoparticles deliver CRISPR/Cas9 system in MSCs. Adv Sci. 2018;5:1700611. doi: 10.1002/advs.201700611.
- Rayamajhi S, Nguyen TDT, Marasini R, et al. Macrophage-derived exosome-mimetic hybrid vesicles for tumor targeted drug delivery. Acta Biomater. 2019;94:482–494. doi: 10.1016/j.actbio.2019.05.054.
- Sun L, Fan M, Huang D, et al. Clodronate-loaded liposomal and fibroblast-derived exosomal hybrid system for enhanced drug delivery to pulmonary fibrosis. Biomaterials. 2021;271:120761. doi: 10.1016/j.biomaterials.2021.120761.
- Piffoux M, Silva AKA, Wilhelm C, et al. Modification of extracellular vesicles by fusion with liposomes for the design of personalized biogenic drug delivery systems. ACS Nano. 2018;12(7):6830–6842. doi: 10.1021/acsnano.8b02053.
- Lutz MP, Esser IB, Flossmann-Kast BB, et al. Overexpression and activation of the tyrosine kinase src in human pancreatic carcinoma. Biochem Biophys Res Commun. 1998;243(2):503–508. doi: 10.1006/bbrc.1997.8043.
- Mahajan K, Coppola D, Chen YA, et al. Ack1 tyrosine kinase activation correlates with pancreatic cancer progression. Am J Pathol. 2012;180(4):1386–1393. doi: 10.1016/j.ajpath.2011.12.028.
- Duong HQ, Yi YW, Kang HJ, et al. Combination of dasatinib and gemcitabine reduces the ALDH1A1 expression and the proliferation of gemcitabine-resistant pancreatic cancer MIA PaCa-2 cells. Int J Oncol. 2014;44(6):2132–2138. doi: 10.3892/ijo.2014.2357.
- Morton JP, Karim SA, Graham K, et al. Dasatinib inhibits the development of metastases in a mouse model of pancreatic ductal adenocarcinoma. Gastroenterology. 2010;139(1):292–303. doi: 10.1053/j.gastro.2010.03.034.
- Ma L, Wei J, Su GH, et al. Dasatinib can enhance paclitaxel and gemcitabine inhibitory activity in human pancreatic cancer cells. Cancer Biol Ther. 2019;20(6):855–865. doi: 10.1080/15384047.2019.1579956.
- Zhang Y, Zeng X, Wang H, et al. Dasatinib self-assembled nanoparticles decorated with hyaluronic acid for targeted treatment of tumors to overcome multidrug resistance. Drug Deliv. 2021;28(1):670–679. doi: 10.1080/10717544.2021.1905751.
- Dong C, Li B, Li Z, et al. Dasatinib-loaded albumin nanoparticles possess diminished endothelial cell barrier disruption and retain potent antileukemia cell activity. Oncotarget. 2016;7(31):49699–49709. doi: 10.18632/oncotarget.10435.
- Zeng F, Ju RJ, Liu L, et al. Efficacy in treating lung metastasis of invasive breast cancer with functional vincristine plus dasatinib liposomes. Pharmacology. 2018;101(1-2):43–53. doi: 10.1159/000480737.
- Qian X, Xie F, Cui D. Exploring purification methods of exosomes from different biological samples. Biomed Res Int. 2023;2023:2336536. doi: 10.1155/2023/2336536.
- Xu L, Faruqu FN, Lim YM, et al. Exosome-mediated RNAi of PAK4 prolongs survival of pancreatic cancer mouse model after loco-regional treatment. Biomaterials. 2021;264:120369. doi: 10.1016/j.biomaterials.2020.120369.
- Behroozi F, Abdkhodaie MJ, Abandansari HS, et al. Engineering folate-targeting diselenide-containing triblock copolymer as a redox-responsive shell-sheddable micelle for antitumor therapy in vivo. Acta Biomater. 2018;76:239–256. doi: 10.1016/j.actbio.2018.05.031.
- Zhang L, Lin Z, Chen Y, et al. Co-delivery of docetaxel and resveratrol by liposomes synergistically boosts antitumor efficiency against prostate cancer. Eur J Pharm Sci. 2022;174:106199. doi: 10.1016/j.ejps.2022.106199.
- Xiong G, Wu Z, Yi J, et al. ADMETlab 2.0: an integrated online platform for accurate and comprehensive predictions of ADMET properties. Nucleic Acids Res. 2021;49(W1):W5–W14. doi: 10.1093/nar/gkab255.
- Lipinski CA, Lombardo F, Dominy BW, et al. Experimental and computational approaches to estimate solubility and permeability in drug discovery and development settings. Adv Drug Deliv Rev. 2001;46(1-3):3–26. doi: 10.1016/s0169-409x(00)00129-0.
- Trott O, Olson AJ. AutoDock vina: improving the speed and accuracy of docking with a new scoring function, efficient optimization, and multithreading. J Comput Chem. 2010;31(2):455–461. doi: 10.1002/jcc.21334.
- Burley SK, Berman HM, Kleywegt GJ, et al. Protein data bank (PDB): the single global macromolecular structure archive. Methods Mol Biol. 2017;1607:627–641. doi: 10.1007/978-1-4939-7000-1_26.
- Landrum G. RDKit: a software suite for cheminformatics, computational chemistry, and predictive modeling. Greg Landrum. 2013;8:31.
- DeLano WL. Pymol: an open-source molecular graphics tool. CCP4 Newslett Pro Crystallogr. 2002;40(1):82–92.
- Laskowski RA, Swindells MB. LigPlot+: multiple ligand-protein interaction diagrams for drug discovery. J Chem Inf Model. 2011;51(10):2778–2786. doi: 10.1021/ci200227u.
- Chee CE, Krishnamurthi S, Nock CJ, et al. Phase II study of dasatinib (BMS-354825) in patients with metastatic adenocarcinoma of the pancreas. Oncologist. 2013;18(10):1091–1092. doi: 10.1634/theoncologist.2013-0255.
- Cabrera E, Welch LC, Robinson MR, et al. Cryopreservation and the freeze–thaw stress response in yeast. Genes (Basel). 2020;11(8):835. doi: 10.3390/genes11080835.
- Rudolph AS, Crowe JH. Membrane stabilization during freezing: the role of two natural cryoprotectants trehalose and proline. Cryobiology. 1985;22(4):367–377. doi: 10.1016/0011-2240(85)90184-1.
- Costa AP, Xu X, Burgess DJ. Freeze-anneal-thaw cycling of unilamellar liposomes: effect on encapsulation efficiency. Pharm Res. 2014;31(1):97–103. doi: 10.1007/s11095-013-1135-z.
- Siow LF, Rades T, Lim MH. Characterizing the freezing behavior of liposomes as a tool to understand the cryopreservation procedures. Cryobiology. 2007;55(3):210–221. doi: 10.1016/j.cryobiol.2007.08.002.
- Meyuhas D, Nir S, Lichtenberg D. Aggregation of phospholipid vesicles by water-soluble polymers. Biophys J. 1996;71(5):2602–2612. doi: 10.1016/S0006-3495(96)79452-3.
- Lentz BR, Lee J. Poly(ethylene glycol) (PEG) mediated fusion between pure lipid bilayers a mechanism in common with viral fusion and secretory vesicle release. Mol Membr Biol. 1999;16(4):279–296. doi: 10.1080/096876899294508.
- Litschel T, Ganzinger KA, Movinkel T, et al. Freeze-thaw cycles induce content exchange between cell-sized lipid vesicles. New J Phys. 2018;20(5):055008. doi: 10.1088/1367-2630/aabb96.
- Gurung S, Perocheau D, Touramanidou L, et al. The exosome journey: from biogenesis to uptake and intracellular signalling. Cell Commun Signal. 2021;19(1):47. doi: 10.1186/s12964-021-00730-1.
- van Niel G, D'Angelo G, Raposo G. Shedding light on the cell biology of extracellular vesicles. Nat Rev Mol Cell Biol. 2018;19(4):213–228. doi: 10.1038/nrm.2017.125.
- Donoso‐Quezada J, Ayala‐Mar S, González‐Valdez J. The role of lipids in exosome biology and intercellular communication: function, analytics and applications. Traffic. 2021;22(7):204–220. doi: 10.1111/tra.12803.
- Toda Y, Takata K, Nakagawa Y, et al. Effective internalization of U251-MG-secreted exosomes into cancer cells and characterization of their lipid components. Biochem Biophys Res Commun. 2015;456(3):768–773. doi: 10.1016/j.bbrc.2014.12.015.
- Zhan Q, Yi K, Li X, et al. Phosphatidylcholine-engineered exosomes for enhanced tumor cell uptake and intracellular antitumor drug delivery. Macromol Biosci. 2021;21:e2100042. doi: 10.1002/mabi.202100042.
- Smyth TJ, Redzic JS, Graner MW, et al. Examination of the specificity of tumor cell derived exosomes with tumor cells in vitro. Biochim Biophys Acta. 2014;1838(11):2954–2965. doi: 10.1016/j.bbamem.2014.07.026.
- Mulcahy LA, Pink RC, Carter DR. Routes and mechanisms of extracellular vesicle uptake. J Extracell Vesicles. 2014;3(1):24641. doi: 10.3402/jev.v3.24641.
- Escrevente C, Keller S, Altevogt P, et al. Interaction and uptake of exosomes by ovarian cancer cells. BMC Cancer. 2011;11(1):108. doi: 10.1186/1471-2407-11-108.
- Rana S, Yue S, Stadel D, et al. Toward tailored exosomes: the exosomal tetraspanin web contributes to target cell selection. Int J Biochem Cell Biol. 2012;44(9):1574–1584. doi: 10.1016/j.biocel.2012.06.018.
- Horibe S, Tanahashi T, Kawauchi S, et al. Mechanism of recipient cell-dependent differences in exosome uptake. BMC Cancer. 2018;18(1):47. doi: 10.1186/s12885-017-3958-1.
- Parolini I, Federici C, Raggi C, et al. Microenvironmental pH is a key factor for exosome traffic in tumor cells. J Biol Chem. 2009;284(49):34211–34222. doi: 10.1074/jbc.M109.041152.
- Lima LG, Ham S, Shin H, et al. Tumor microenvironmental cytokines bound to cancer exosomes determine uptake by cytokine receptor-expressing cells and biodistribution. Nat Commun. 2021;12(1):3543. doi: 10.1038/s41467-021-23946-8.
- Verhoef JJ, Anchordoquy TJ. Questioning the use of PEGylation for drug delivery. Drug Deliv Transl Res. 2013;3(6):499–503. doi: 10.1007/s13346-013-0176-5.
- Hong RL, Huang CJ, Tseng YL, et al. Direct comparison of liposomal doxorubicin with or without polyethylene glycol coating in C-26 tumor-bearing mice: is surface coating with polyethylene glycol beneficial? Clin Cancer Res. 1999;5(11):3645–3652.
- Sadzuka Y, Kishi K, Hirota S, et al. Effect of polyethyleneglycol (PEG) chain on cell uptake of PEG-modified liposomes. J Liposome Res. 2003;13(2):157–172. doi: 10.1081/lpr-120020318.
- Laulagnier K, Motta C, Hamdi S, et al. Mast cell- and dendritic cell-derived exosomes display a specific lipid composition and an unusual membrane organization. Biochem J. 2004;380(Pt 1):161–171. doi: 10.1042/BJ20031594.
- Lv Q, Cheng L, Lu Y, et al. Thermosensitive exosome–liposome hybrid nanoparticle‐mediated chemoimmunotherapy for improved treatment of metastatic peritoneal cancer. Adv Sci. 2020;7:2000515. doi: 10.1002/advs.202000515.
- Ci T, Li H, Chen G, et al. Cryo-shocked cancer cells for targeted drug delivery and vaccination. Sci Adv. 2020;6(50):eabc3013. doi: 10.1126/sciadv.abc3013.
- Chen MM, Li J, Mills GB, et al. Predicting cancer cell line dependencies from the protein expression data of reverse-phase protein arrays. J Clin Oncol Clin Cancer Inform. 2020;4:357–366. doi: 10.1200/CCI.19.00144.
- McGeary SE, Lin KS, Shi CY, et al. The biochemical basis of microRNA targeting efficacy. Science. 2019;366(6472):eaav1741. doi: 10.1126/science.aav1741.