Abstract
Locally advanced head and neck squamous cell carcinoma (LA-HNSCC) is characterized by high rate of recurrence, resulting in a poor survival. Standard treatments are associated with significant toxicities that impact the patient’s quality of life, highlighting the urgent need for novel therapies to improve patient outcomes. On this regard, noble metal nanoparticles (NPs) are emerging as promising agents as both drug carriers and radiosensitizers. On the other hand, co-treatments based on NPs are still at the preclinical stage because of the associated metal-persistence.
In this bioconvergence study, we introduce a novel strategy to exploit tumour chorioallantoic membrane models (CAMs) in radio-investigations within clinical equipment and evaluate the performance of non-persistent nanoarchitectures (NAs) in combination with radiotherapy with respect to the standard concurrent chemoradiotherapy for the treatment of HPV-negative HNSCCs. A comparable effect has been observed between the tested approaches, suggesting NAs as a potential platinum-free agent in concurrent chemoradiotherapy for HNSCCs. On a broader basis, our bioconvergence approach provides an advance for the translation of Pt-free radiosensitizer to the clinical practice, positively shifting the therapeutic vs. side effects equilibrium for the management of HNSCCs.
Introduction
Head and neck squamous cell carcinoma (HNSCC) is the sixth most common cancer by incidence worldwide and accounts for more than 90% of head and neck cancers [Citation1,Citation2]. HNSCCs are generally associated either with tobacco and alcohol consumption or human papillomavirus (HPV) infection, especially HPV-16 and 18, which are responsible for an increasing incidence of oropharyngeal cancers [Citation3]. The prognosis and survival of HNSCC patients depends on many variables, including: the stage of cancer development (early vs. locally advanced tumours), the site of involvement, and the HPV status [Citation4]. While the curative response for patients with early-stage cancer is high, the majority of locally advanced HNSCC (LA-HNSCC) patients, especially those with HPV negative tumors, may not respond or even relapse after receiving multimodality/combined therapy, exhibiting a poor five-year survival rate of about 25%-40% [Citation4]. Indeed, these malignancies are challenging to treat and often require combined treatments (surgery, radiotherapy, and systemic therapy). According to the international guidelines, RT is indicated as an exclusive therapy in early-stage tumours and in combination with surgery or concurrent chemotherapy in advanced stages. Definitive cisplatin (CDDP)-based chemoradiotherapy (CTRT) has become the standard treatment for LA-HNSCC in patients younger than 70 years old, despite its severe associated toxicities and common resistance [Citation5]. Based on multiple randomized trials and meta-analysis, CTRT relies on cisplatin at a dose 100 mg/m2 given every 3 weeks concurrently with RT [Citation6–8]. However, up to 40% of patients require modifications of the treatment regimen (i.e. dose reduction, delays, and omissions) because of the associated adverse events (AEs), as renal insufficiency, electrolyte imbalances, myelo- and oto-toxicity [Citation9]. In addition, radiation-induced toxicity mainly depends on the dose of RT to the surrounding organs at risk (OAR), such as the spinal cord, brainstem, brachial plexus, salivary glands, swallowing organs, and larynx [Citation10]. The administered dose to OAR cannot be completely avoided despite the recent advancement in RT technologies which allowed an increase in the dose to the target while sparing healthy tissue (e.g. Intensity Modulated RadioTherapy -IMRT- and Volumetric Modulated Arc Therapy -VMAT-) [Citation10]. Overall, more effective and less toxic treatment strategies to improve the outcome of HNSCCs patients are strongly demanded. In this regard, the emerging role of metal nanoparticles (NPs) as radiosensitizers represents a promising approach in preclinical research [Citation11]. The mechanism of action by which they induce cellular toxicity is notably different compared to platinum-based drugs. Indeed, the cytotoxic effect of cisplatin is linked to its ability to bind the purine bases of the DNA and interfere with the DNA repair mechanism, causing damage and subsequently apoptosis-induced cell death [Citation12]. On the other hand, NPs may enhance the physical, chemical, and biological effects of RT on biological systems via multiple mechanisms, leading to the elevated generation of toxic substances such as radicals or electrons, consumption of radical scavengers, and fixation of IR induced damage, which enhance the biological response to IR [Citation13]. These effects are accentuated in the presence of elements with high atomic numbers (Z) [Citation14]. Consequently, the accumulation of high-Z atoms (such as gold, Z = 79) within the target tissue amplifies the deposition of energy and intensifies the radiobiological effects, promoting direct and indirect DNA damage and cell death [Citation14]. A comprehensive discussion regarding the mechanisms behind the radiosensiting effect of gold NPs (comprising the dependance from size and shape) can be find elsewhere [Citation15]. In general, several concerns about the size, shape and biocompatibility have limited the translation of NPs to the clinical practice [Citation15,Citation16]. In order to avoid the metal persistence, we developed a family of biodegradable plasmon nano-architectures (NAs) with excretable features demonstrated after intravenous or intranasal administration in healthy mice [Citation17–19]. NAs are composed by ∼100nm silica nanocapsules including plasmonic ultrasmall noble metals (USNPs, ∼3nm) in the inner core that are reversibly aggregated with charged polymers. Besides biodistribution assays, NAs have been evaluated for targeted drug delivery, chemo/photothermal treatments, and photoacoustic/ultrasound imaging applications [Citation20–22].
Within our bioconvergence approach, HPV-negative HNSCCs chorioallantoic membrane (CAM) tumour models have been exploited to evaluate the effects of gold NAs based concurrent chemoradiotherapy in comparison to the gold standard (i.e. cisplatin-based CTRT). To perform this investigation, we optimized a custom-designed holder that supports evaluations on CAMs with clinical equipment and the implementation of rational physical parameters. Indeed, its design replicates in CAMs the irradiation conditions employed in the clinical practice to mimic the target coverage and the OAR sparing. To the best of our knowledge, the employment of CAMs for evaluating the effects of ionizing radiation and combined treatments on tumour response is very limited [Citation23]. Among these, Che et al. evaluated the DNA damage induced by γ-radiation, using a 60Co-source Gammacell Research irradiator, for the treatment of pancreatic ductal adenocarcinoma [Citation24]. Kleibeuker et al. investigated the influence of γ-ray on the vasculature of the CAM and tumours generated with colon carcinoma and oesophageal adenocarcinoma cells [Citation25]. In our experimental set-up, RT was delivered with a Varian DHX LINearACcelerator (LINAC) that delivers a total X-ray dose of 1 Gy at 6 MV photons. Compared to the abovementioned studies (both using Gammacell irradiator), our irradiation technique allows to deliver the radiation dose on the target while sparing the chick embryo. In Gammacell, instead, the entire egg is uniformly irradiated, determining potential superimposition of the radiation effects to embryos and tumours, reducing the translational potential of the data.
Overall, besides confirming the enhanced effect of multimodal treatments compared to the monomodal approaches, NAs-based CTRT demonstrated comparable effects to the platinum-based strategy avoiding both clearance issues and severe toxicities. Furthermore, we demonstrate that the association of advanced preclinical models with clinical equipment is crucial to promote the development of promising therapeutic strategies in translational research.
Materials & methods
Synthesis of gold nano-architectures (AuNAs)
Synthesis of gold seeds. Gold ultrasmall nanoparticles (Au USNPs) of 3 nm in size were prepared as follows. 20 mL of Milli-Q® water was added with 10 µL of poly(sodium 4-styrenesulfonate) (PSS, 70 kDa, 30% in water; Sigma-Aldrich, #527483) and 200 µL of HAuCl4 aqueous solution (29.4 mM; Alfa Aesar, #36400). 200 µL of NaBH4 aqueous solution (211 mM; Sigma-Aldrich, #452882) was quickly added during vigorous stirring, turning the suspension from colourless to red in colour. The mixture was further stirred for 10 minutes at room temperature.
Synthesis of gold arrays. The Au USNPs-bearing polymeric arrays were formed by slowing dropping 75 µL of poly(L-lysine) (PL, 15–30 kDa, 40 mg/mL in Milli-Q® water; Sigma-Aldrich, #7890) to the suspension. The arrays were stirred for 20 minutes at room temperature, then collected by 3-minute centrifugation at 17092 rcf and resuspended in 2 mL of Milli-Q® water.
Synthesis of gold nanoarchitectures (AuNAs). A modified Stöber protocol was applied to form the silica shell. The array suspension was shortly sonicated and dropped to 70 mL of absolute ethanol (Sigma-Aldrich, #24105), previously mixed with 40 µL of tetraethyl orthosilicate (TEOS, 98%; Sigma-Aldrich, #131903) and 2.4 mL of ammonium hydroxide (Sigma-Aldrich, #221228). The final mixture was mildly shaken at room temperature for 3 hours. The synthesized NAs were collected by 30-minute centrifugation at 3220 rcf. NAs were resuspended in 2 mL of ethanol and washed once with ethanol and once with Milli-Q® water to discard unreacted reagents. A short spin (14 seconds at 14462 rcf) allowed to remove bigger NAs by precipitation: the supernatant was collected and washed in ethanol. AuNAs were stored in 1 mL of ethanol at -20 °C.
NAs characterization
Transmission electron microscopy (TEM)
ZEISS Libra 120, operated at 120 kV accelerating voltage was used to collect TEM. NAs suspension was applied on 300-mesh carbon-coated copper grids and dried before acquisition. TEM images were analyzed using ImageJ software. Particle diameter and silica shell size were measured on at least 100 nanoparticles.
Inductively coupled Plasma-Mass spectrometry (ICP-MS) analysis
The gold content in AuNAs was assessed employing ICP-MS Agilent 7700 (Agilent Technologies, Santa Clara, CA, USA). NAs were placed in pressure vessels and pre-digested using freshly made aqua regia made of 3:1 hydrochloric acid (34–37% in HCl, TraceMetal Grade, Thermo Fisher Scientific; #A508-P500) and nitric acid (65% Suprapur®, Sigma-Aldrich; #1.00441). Then, samples were stirred at 200 °C following an irradiation protocol using CEM Discover SP-D digestion microwave (CEM, Matthews, NC, USA). Samples were diluted to 3 ml using 3% nitric acid solution and analyzed. Au quantification was performed by analysis against standard calibration curves (gold standard 1000 ppm, Absolute Standards, inc.; #56079), using 10 ppm Hg (10,000 ppm, Sigma-Aldrich; #75111) in 3% nitric acid solution as internal standard.
2D Cell culture
SCC-25 cancer cell line (Human Squamous Cell Carcinoma, purchased from the American Type Culture Collection - ATCC) was cultured in Dulbecco’s Modified Eagle Medium/Nutrient Mixture F12 (DMEM/F12; Gibco 11039-021) supplemented with 10% foetal bovine serum (FBS), 4 mM L-glutamine, 1 mM sodium pyruvate, 100 U/mL penicillin, 100 mg/mL streptomycin and 400 ng/mL of hydrocortisone. The cells were kept in a humidified incubator set at 37 °C and 5% CO2.
Chorioallantoic membrane (CAM) assay
CAM assay was performed following the step-by-step procedure reported in Sarogni et al. [Citation26] Briefly, fertilized red Leghorn chicken eggs were incubated at 37 °C in a humidified incubator (FIEM). The start of incubation was assigned as Embryonic Day of Development (EDD) 0. On EDD3, the blunt end of each egg was drilled to form a small hole and all eggs were placed back in the incubator in vertical position up to EDD6. On EDD6, the hole was further cut into a small window of ∼1 cm2 and the SCC-25 cells were transplanted on the CAM. 2x106 cells were diluted in a 1:1 mixture of Matrigel (Corning, Ref 354234) and serum-free cell culture medium to a final volume of 25 μL per egg. At EDD10, tumour-bearing eggs were randomized and divided into different treatment conditions: i) serum-free cell culture medium/RT; ii) CisPt/RT; iii) NAs/RT.
Eggs treatments
CisPt treatment. The dose of drug in CisPt-treated eggs was equivalent to the amount used in our previous investigation, corresponding to ∼4 μg of Pt per egg (688 μM) [Citation27].
NAs treatment. The NAs group was treated with ∼24 μg of gold. All treatments were topically administered after resuspension in 30 μL of serum-free medium.
Radiation treatment. The Radiotherapy (RT) treatment was administered at EDD11 with a Varian DHX linac (Varian Medical System, Inc., Palo Alto City, California, USA) giving a total radiation dose of 1 Gy with 6 MV photons. For the irradiation a Polymethylmethacrylate (PMMA) holder of external size 21x16x21cm3 and internal size 19x14x20cm3 was specifically designed to accommodate six eggs (), allowing an efficient delivery of the radiation dose (). Before the irradiation, the holder was filled with saline solution (NaCl 0.9%) at 37 °C. The irradiation technique was studied to deliver a uniform dose to the tumours grafted on the upper part of the CAMs, minimizing the contribution to the alive embryos. The holder was irradiated with two lateral 5x18cm2 opposite half-field (gantry angle of 90 and 270 degrees, upper half-field open) (Figure S1) with the isocenter in the mid lateral and longitudinal plane of the holder at an optimal height. The optimal height was derived from the study of 10 treatment plans on different X-rays Computed Tomography (XCT) simulations to account for different chickens and CAMs positions (Figure S2A-B). The dosimetric studies were done with the Treatment Planning System (TPS) Varian Eclipse 16.1 and the Acuros XB algorithm to better account for tissue inhomogeneities and egg air chambers (Figure S2C) [Citation28].
Following RT, the eggs were daily monitored and photographed until EDD14, the day of tumours harvesting. Tumour volume was calculated using the following formula ½ × (length × width2).
Figure 1. A) Custom-designed holder to locate six eggs for efficient radiation dose delivery. B) Dose distribution on the six eggs holder. C) Scheme of the experimental set up. At EDD10 the eggs were randomized and distributed in three groups for topical administration of medium (control), cisplatin and NAs. Radiotherapy was delivered at EDD11. Tumours were monitored until EDD14.
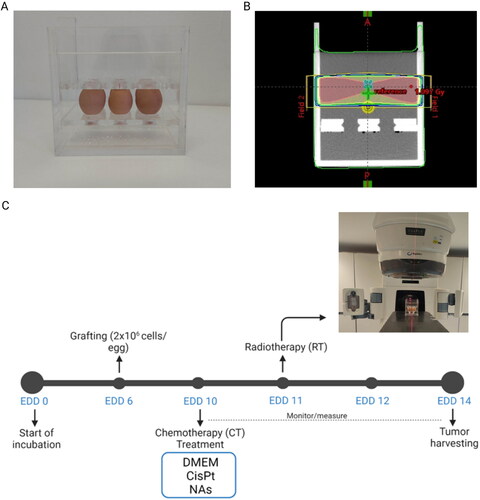
Results and discussion
The highly vascularized CAM supports the fast composition of standardized alternative in vivo tumour models, as previously demonstrated with both HNSCCs and pancreatic ductal adenocarcinoma cells [Citation27,Citation29]. It should be noted that CAMs respect the 3Rs concept, in accordance with the European Parliament Directive 2010/63/EU [Citation26]. Due to their intrinsic features, these biomodels perfectly suits for preclinical investigations in oncological research, high-throughput drug-screening, and metastasis evaluations [Citation30]. Regardless an elaborated and ethical tumour representation, CAMs offer a variety of technical and practical advantages, including the rapid tumour mass formation, versatility, non-aseptic laboratory requirement, and cost-effectiveness [Citation30]. The observation of the tumours allows a rapid monitoring of both volumetric and morphological changes by following the application of specific treatments. These features enable an initial assessment of the tumour treatment response. Indeed, the establishment of appropriate treatment schedules in preclinical research plays a crucial role to improve the therapeutic planning in clinical practice. To this end, a custom-designed egg-holder that replicates in CAMs the target coverage and the OAR sparing was developed ().
Specifically, the holder was designed to be irradiated with 6 MV photons, the highest employed energy in current head and neck cancer radiotherapy treatments [Citation31]. The irradiation technique involved two laterally opposite half-fields (gantry angles of 90 and 270 degrees, upper half-field open) in an isocentric mode to obtain a uniform coverage of the grafted tumours while reducing the radiation field divergence (lower half-field blocked) towards the embryos (). Tumours are located on the CAMs at different heights compared to the egg bottom due to the different air chamber volume of each model. To ensure the optimal neoplasm coverage, the CAM was irradiated with sufficient lower margin by taking into accounts the radiation field penumbra. In this regard, 10 different eggs were analyzed by XCTs (Figure S2 A-B) to estimate the values of the air chamber height. The lower margin of the radiation field was chosen as the maximal air chamber height plus the field penumbra, which is 5 mm at the isocenter depth of 8 cm.
It should be noted that the lateral irradiation technique induces a sufficient build-up of the radiation dose deposited by the megavoltage X-rays ensuring secondary electrons reach the target even in presence of the air-tissue interface. XCTs of CAMs also allowed the estimation of the distance between the embryo and the radiation field lower limit, resulting in a median overlapping between the embryos and the radiation field of 5 mm (comparable to the penumbra region). Embryos are then spared from radiation in agreement with the dose toxicity curve (RT: 2, 4, 6, and 8 Gy) determined in not-grafted eggs (Figure S3). The various treatments were well-tolerated by the embryos, confirming the safety of this irradiation technique. However, only one death event out of eight specimens was recorded with RT 8 Gy, probably due to excessive overlapping of the chick embryo with the radiation field due to its movements.
At EDD 10, tumour-bearing embryos were randomized and divided into three groups of study: i) serum-free cell culture medium ± RT (control); ii) CisPt ± RT; iii) NAs ± RT (). The chemo treatment (CT) was administered on EDD10 as already standardized elsewhere [Citation26]. The applied dosage of cisplatin (6 μg) results from the clinically administered amount of the drug in human (100 mg/m2) and the weight of chicken embryo at EDD10. Its antitumor effects on HNSCCs CAMs have been previously reported [Citation27,Citation32]. The amount of gold administered through NAs (∼24 μg) is determined by considering the cisplatin-gold ratio in the nanoarchitectures loaded with both the drug and the gold USNPs (1:6 Pt/Au, determined by ICP-MS), which also showed an interesting antiangiogenic activity in previous investigations [Citation27]. NAs have been produced and characterized by SOPs (Figure S4) [Citation17]. RT treatment was delivered at EDD11 to allow a homogeneous distribution of the chemo-treatment in the neoplasm and the biodegradation of NAs, as described in both 2D and 3D SCC-25 models by Santi and co-workers [Citation33]. After the application of the treatments, the tumours were daily monitored until EDD14 to evaluate the volume fold change, which is the variation of tumours size compared to the pre-treatment ().
Figure 2. A) Example of tumour-grafted CAMs during the experimental window. Images were taken with a portable digital microscope (DinoLite). Scale bar = 2 mm. B) Comparison between the non-irradiated and irradiated groups. The data are reported as mean ± SD of 2 independent experiments. N = at least 3 eggs per condition. Statistical analysis was performed by two-way ANOVA (Mann–Whitney test). *p < 0.05, **p < 0.005. C) Comparison of each CT + RT treatment vs. RT group. Tumour volume fold change was calculated over the EDD14.
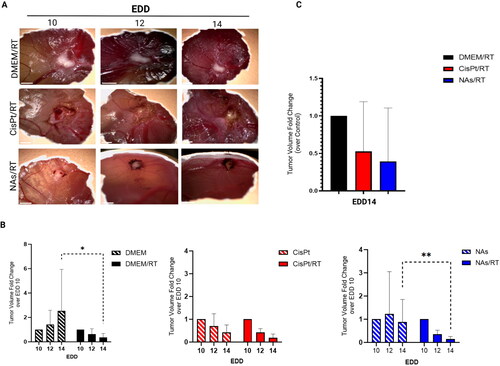
Although the tumour volume fold change is not a direct predictor of the clinical overall survival (OS) rate, it may suggest a trend in the disease-free survival and locoregional control in HNSCC patients [Citation34]. In general (), the condition RT alone resulted in a lower average tumour volume fold change (∼0.36) compared to each CT alone group (CisPt: ∼0.42, NAs: ∼0.88; dashed bars), confirming that radiotherapy is an effective treatment. These findings are consistent with those reported in literature, as it is widely recognized that concurrent radiotherapy yields the most favourable outcomes in LA-HNSCC cancer. This has been elucidated in the initial meta-analysis of chemotherapy in head and neck cancer (MACH-NC) and subsequent updates [Citation6–8]. Regardless of the radiotherapy fractionation method employed, the treatment confers a significant advantage in terms of loco-regional control and overall survival, with a notable increase of +6.5% at 5 years [Citation6–8]. As expected, NAs condition alone did not show a significant tumour-arresting effect compared to monomodal cisplatin because the low toxicity profile of the nano-architectures and their building blocks. On the other hand, the dual NAs/RT treatment resulted in a significant tumour reduction, especially at EDD14 (, blue panel) due to the radio-sensitizing effect associated to gold. Eventually, it is interesting to notice that, although not statistically significant, both concomitant treatments (CisPt/RT and NAs/RT) caused an improved effect on tumour volume compared to the RT group alone (). Indeed, the tumour volume fold change of NAs/RT (∼0.39 mm3) was comparable and even smaller of CisPt/RT (∼0.52 mm3) after normalization over the RT administration alone (set as equal to 1). These results corroborate the evidence of the critical role that rationally designed gold nanomaterials may have in replacement/reducing cisplatin employment in concomitant treatments in the clinical practice. The available evidence for the use of Au NPs to enhance the efficacy of radiotherapy primarily comes from preclinical studies, and only a few have demonstrated in vivo their effectiveness as radiosensitizers for HNSCCs. For example, Piccolo et al. investigated the radiosensitizing effect of Au NPs both in vitro and in vivo models of head and neck cancer, demonstrating a decrease in cellular viability and enhanced tumour cell killing, respectively, compared to standard RT [Citation35]. Popovtzer et al. instead, employed in vivo models of HNSCC to evaluate the biological mechanism and toxicity profile of targeted Au NPs followed by conventional 6 MV RT. They have shown that the use of Au NPs was associated with a significant improvement in tumour radiosensitivity with an enhanced and accelerated apoptosis compared to conventional RT [Citation36]. Remarkably, our study further confirms the potential of non-persistent gold NAs to improve radio-oncology treatments. At the same time, this works stresses NAs potentiality to overcome the clinical limitations of Pt-based treatments associated to their toxicity.
Conclusion
HPV-negative HNSCCs CAM model was employed to assess the potential radiosensitizing effects of NAs in comparison to radiotherapy and cisplatin-based chemoradiotherapy. The comparable tumour-shrinking effect among NAs/RT and CisPt/RT together with the non-persistence of the NAs building blocks suggest that the nano-architectures may be a potential candidate for the development of a Pt-free chemoradiotherapy approach for HNSCCs management. Moreover, this study further confirms the pivotal role of the in vivo CAM models for a rapid assessment of tumours treatment response in compliance with the 3R’s concept on the employment of animal models for scientific purposes. On this regard, we have introduced a novel approach for the employment of CAMs with clinical equipment for radiation experiments that allows the implementation of rational physical parameters. On the other hand, further in vivo investigations are needed to shed light on the molecular mechanisms underlying the radiosensitizing effect of NAs as well as on the cancer-associated pathways involved in the tumour-arresting effect. Overall, this study confirms bioconvergence as a promising approach in preclinical research and paves the way for the establishment of future platinum-free treatment strategies for the management of head and neck cancer.
Limitation of the study
Although the CAM model finds increasing application for the screening of anti-cancer drugs according to the 3 R principles (reduction, refinement, and replacement), some limitations may reduce their employment when investigating specific biological conditions. Indeed, the versatility, low experimental cost and easy handling of this alternative in vivo model are associated with short follow-up period for the evaluation of the treatment response as well as the absence of a fully developed immune system for the study of inflammatory responses to specific treatments. The model itself is susceptible to pH, osmolarity, humidity and oxygen content, as well as fungi contamination. It is quite hard to discriminate between neovascularization and the rearrangement of pre-existing blood vessels. Moreover, as demonstrated in this manuscript, home-made device has to be developed to investigate some approaches (such as radiotherapy). On top of that, the immune system develops from embryonic day of development (EDD) 14, therefore the model is not suitable for studying the interactions between cancer cells and immune cells nor for replicating the tumour-host immune system interactions. Despite these limitations, CAMs offer a unique platform to assess initial effects of novel therapeutic approaches, including nanomaterials-based ones, concurrent radiotherapy, and combined strategies. Thus, CAMs are models of special interest in preclinical studies as a translational step prior to commit with more cumbersome and expensive in vivo studies. Future investigations should focus on patient-derived CAMs to compare similarities/discrepancies between the original and modelized neoplasms.
Authors’ contributions
In vivo experiments: A.G, P.S, A.Z., N.G., and V.F. Synthesis: V.F., M.L.E., and A.Z. Irradiation experiments: N.G., A.G., F.D.M., F.P., P.P., and S.L. Data analysis: A.G., and P.S. Project design and coordination: V.V., and F.P. All Authors have discussed the data and contributed to write the manuscript.
ianb_a_2309233_sm5609.jpg
Download JPEG Image (636.2 KB)ianb_a_2309233_sm5605.jpg
Download JPEG Image (441.1 KB)ianb_a_2309233_sm5583.jpg
Download JPEG Image (524 KB)ianb_a_2309233_sm5496.jpg
Download JPEG Image (602.4 KB)ianb_a_2309233_sm5283.jpg
Download JPEG Image (691.1 KB)ianb_a_2309233_sm5580.jpg
Download JPEG Image (567.1 KB)ianb_a_2309233_sm5453.jpg
Download JPEG Image (481.8 KB)ianb_a_2309233_sm5162.docx
Download MS Word (2.9 MB)ianb_a_2309233_sm5578.jpg
Download JPEG Image (610.2 KB)ianb_a_2309233_sm5274.jpg
Download JPEG Image (369 KB)Disclosure statement
Authors declare no conflicts of interest.
Data availability statement
All data underlying the study are available upon request to the corresponding Authors.
Additional information
Funding
References
- Johnson DE, Burtness B, Leemans CR, et al. Head and neck squamous cell carcinoma. Nat Rev Dis Primers. 2020;6(1):92. doi:10.1038/s41572-020-00224-3.
- Mehanna H, Paleri V, West CML, et al. Head and neck cancer - Part 1: epidemiology, presentation, and prevention. BMJ. 2010;341:c4684. doi:10.1136/bmj.c4684.
- McDermott JD, Bowles DW. Epidemiology of head and neck squamous cell carcinomas: impact on staging and prevention strategies. Curr. Treat. Options Oncol. 2019;20(5):43. doi:10.1007/s11864-019-0650-5.
- Mody MD, Rocco JW, Yom SS, et al. Head and neck cancer. Lancet. 2021;398(10318):2289–2299. doi:10.1016/S0140-6736(21)01550-6.
- Haddad RI, Hicks WL, Hitchcock YJ, et al. NCCN guidelines version 1.2023 head and neck cancers continue NCCN guidelines panel disclosures. 2022.
- Pignon J-P, Le Maître A, Maillard E, MACH-NC Collaborative Group., et al. Meta-analysis of chemotherapy in head and neck cancer (MACH-NC): an update on 93 randomised trials and 17,346 patients. Radiother Oncol. 2009;92(1):4–14. doi:10.1016/j.radonc.2009.04.014.
- Blanchard P, Baujat B, Holostenco V, et al. Meta-analysis of chemotherapy in head and neck cancer (MACH-NC): a comprehensive analysis by tumour site. Radiother Oncol. 2011;100(1):33–40. doi:10.1016/j.radonc.2011.05.036.
- Lacas B, Carmel A, Landais C, et al. Meta-analysis of chemotherapy in head and neck cancer (mach-NC): an update on 107 randomized trials and 19,805 patients, on behalf of MACH-NC group. Radiother Oncol. 2021;156:281–293. doi:10.1016/j.radonc.2021.01.013.
- de Roest RH, van der Heijden M, Wesseling FWR, et al. Disease outcome and associated factors after definitive platinum based chemoradiotherapy for advanced stage HPV-negative head and neck cancer. Radiother Oncol. 2022;175:112–121. doi:10.1016/j.radonc.2022.08.013.
- Van den Bosch L, van der Schaaf A, van der Laan HP, et al. Comprehensive toxicity risk profiling in radiation therapy for head and neck cancer: a new concept for individually optimised treatment. Radiother Oncol. 2021;157:147–154. doi:10.1016/j.radonc.2021.01.024.
- Cui L, Her S, Borst GR, et al. Radiosensitization by gold nanoparticles: will they ever make it to the clinic? Radiother Oncol. 2017;124(3):344–356. doi:10.1016/j.radonc.2017.07.007.
- Dasari S, Tchounwou PB. Cisplatin in cancer therapy: molecular mechanisms of action. Eur J Pharmacol. 2014;740:364–378. doi:10.1016/j.ejphar.2014.07.025.
- Rosa S, Connolly C, Schettino G, et al. Biological mechanisms of gold nanoparticle radiosensitization. Cancer Nanotechnol. 2017;8(1):2. doi:10.1186/s12645-017-0026-0.
- Bilynsky C, Millot N, Papa A-L. Radiation nanosensitizers in cancer therapy-from preclinical discoveries to the outcomes of early clinical trials. BioengTranslMed. 2022;7:e10256. doi:10.1002/btm2.10256.
- Wu Y, Zhu K, Zhang X, et al. Emerging plasmonic nanoparticles and their assemblies for cancer radiotherapy. Adv Drug Deliv Rev. 2023; 194:114710. doi:10.1016/j.addr.2023.114710.
- Domingues C, Santos A, Alvarez-Lorenzo C, et al. Where is nano today and where is it headed? A review of nanomedicine and the dilemma of nanotoxicology. ACS Nano. 2022;16(7):9994–10041. doi:10.1021/acsnano.2c00128.
- Cassano D, Mapanao A-K, Summa M, et al. Biosafety and biokinetics of noble metals: the impact of their chemical nature. ACS Appl Bio Mater. 2019;2(10):4464–4470. doi:10.1021/acsabm.9b00630.
- Mapanao AK, Giannone G, Summa M, et al. Biokinetics and clearance of inhaled gold ultrasmall-in-nano architectures. Nanoscale Adv. 2020;2(9):3815–3820. doi:10.1039/d0na00521e.
- Zamborlin A, Ermini ML, Summa M, et al. The fate of intranasally instilled silver nanoarchitectures. Nano Lett. 2022;22(13):5269–5276. doi:10.1021/acs.nanolett.2c01180.
- Mapanao AK, Santi M, Voliani V. Combined chemo-photothermal treatment of three-dimensional head and neck squamous cell carcinomas by gold nano-architectures. J Colloid Interface Sci. 2021;582(Pt B):1003–1011. doi:10.1016/j.jcis.2020.08.059.
- Mapanao AK, Santi M, Faraci P, et al. Endogenously triggerable ultrasmall-in-nano architectures: targeting assessment on 3D pancreatic carcinoma spheroids. ACS Omega. 2018;3(9):11796–11801. doi:10.1021/acsomega.8b01719.
- Armanetti P, Pocoví-Martínez S, Flori A, et al. Dual photoacoustic/ultrasound multi-parametric imaging from passion fruit-like nano-architectures. Nanomedicine. 2018;14(6):1787–1795. doi:10.1016/j.nano.2018.05.007.
- Mapanao AK, Che PP, Sarogni P, et al. Tumor grafted - chick chorioallantoic membrane as an alternative model for biological cancer research and conventional/nanomaterial-based theranostics evaluation. Expert Opin Drug Metab Toxicol. 2021;17(8):947–968. doi:10.1080/17425255.2021.1879047.
- Che PP, Mapanao AK, Gregori A, et al. Biodegradable ultrasmall-in-nano architectures loaded with cisplatin prodrug in combination with ionizing radiation induces DNA damage and apoptosis in pancreatic ductal adenocarcinoma. Cancers. 2022;14(12):3034. doi:10.3390/cancers14123034.
- Kleibeuker EA, Ten Hooven MA, Castricum KC, et al. Optimal treatment scheduling of ionizing radiation and sunitinib improves the antitumor activity and allows dose reduction. Cancer Med. Med2015;4(7):1003–1015. doi:10.1002/cam4.441.
- Sarogni P, Mapanao AK, Marchetti S, et al. A standard protocol for the production and bioevaluation of ethical in vivo models of HPV-negative head and neck squamous cell carcinoma. ACS Pharmacol Transl Sci. 2021;4(3):1227–1234. doi:10.1021/acsptsci.1c00083.
- Sarogni P, Mapanao AK, Gonnelli A, et al. Chorioallantoic membrane tumor models highlight the effects of cisplatin compounds in oral carcinoma treatment. IScience. 2022;25(3):103980. doi:10.1016/j.isci.2022.103980.
- Fogliata A, Nicolini G, Clivio A, et al. Dosimetric evaluation of acuros XB advanced dose calculation algorithm in heterogeneous media. Radiat Oncol. 2011;6(1):82. doi:10.1186/1748-717X-6-82.
- Sarogni P, Zamborlin A, Mapanao AK, et al. Hyperthermia reduces irradiation-induced tumor repopulation in an in vivo pancreatic carcinoma model. AdvBiol. 2023; 7(10):2200229. doi:10.1002/adbi.202200229.
- Fischer D, Fluegen G, Garcia P, et al. The CAM model-Q&a with experts. Cancers. 2022;15(1):191. doi:10.3390/cancers15010191.
- DiBartolo D, Carpenter TJ, Santoro JP, et al. Novel VMAT planning technique improves dosimetry for head and neck cancer patients undergoing definitive chemoradiotherapy. Int J Radiat Oncol Biol Phys. 2022;112(5):e33. doi:10.1016/j.ijrobp.2021.12.077.
- Mapanao AK, Sarogni P, Santi M, et al. Pro-apoptotic and size-reducing effects of protein corona-modulating nano-architectures enclosing platinum prodrug in in vivo oral carcinoma. Biomater Sci. 2022;10(21):6135–6145. doi:10.1039/d2bm00994c.
- Santi M, Mapanao AK, Cassano D, et al. Endogenously-activated ultrasmall-in-nano therapeutics: assessment on 3D head and neck squamous cell carcinomas. Cancers . 2020;12(5):1063. doi:10.3390/cancers12051063.
- Russo E, Accorona R, Iocca O, et al. Does tumor volume have a prognostic role in oropharyngeal squamous cell carcinoma? A systematic review and meta-analysis. Cancers. 2022;14(10):2465. doi:10.3390/cancers14102465.
- Piccolo O, Lincoln JD, Melong N, et al. Radiation dose enhancement using gold nanoparticles with a diamond linear accelerator target: a multiple cell type analysis. Sci Rep. 2022;12(1):1559. doi:10.1038/s41598-022-05339-z.
- Popovtzer A, Mizrachi A, Motiei M, et al. Actively targeted gold nanoparticles as novel radiosensitizer agents: an in vivo head and neck cancer model. Nanoscale. 2016;8(5):2678–2685.