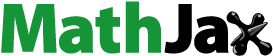
ABSTRACT
The microstructure and mechanical properties of polycrystalline spinel ceramics (MgAl2O4) with high translucency were investigated. Several kinds of polycrystalline spinel ceramics were prepared under various sintering conditions, and single-crystal spinel and sapphire were used for comparison. The bending strength of polycrystalline spinel with smaller grain size fabricated without sintering aids exceeded that of single-crystal spinel. The bending strength at 1000°C tended to be lower than that at room temperature. The fracture toughness of polycrystalline spinel prepared without sintering aids measured by SEVNB was higher than that of spinel with sintering aids. By estimation of fracture strength considering different fracture origins, the polycrystalline spinel prepared without sintering aids might be fractured mainly from defects around residual spherical voids. If the size of voids can be minimized in polycrystalline spinel fabricated without sintering aids, both high transmission and good mechanical properties could be achieved.
1. Introduction
Spinel (MgAl2O4) has great potential as an optical material in applications, such as laser oscillation, electroluminescence, and as an infrared window material. If an infrared window material is mounted on an orbiter, rocket, or airplane, it is exposed to severe conditions, such as high mechanical stress, high temperature, and large thermal shock. In such cases, the window material is required not only to have high transmittance in the infrared region but also excellent thermo-mechanical properties, such as fracture strength, fracture toughness, and thermal shock resistance. Infrared windows have used sapphire due to its high transmittance and good mechanical properties.
Because spinel is a hard-to-sinter material, the development of translucency has required the use of pressure sintering, such as hot pressing (HP) [Citation1–8], hot isostatic pressing (HIP) [Citation1,Citation9–12] and the pulsed electric current sintering (PECS) technique [Citation13–15], and sintering aids such as B2O3 [Citation9], CaO [Citation16], Cr2O3 [Citation3], Y2O3 [Citation17] and, most commonly, fluorides such as LiF [Citation4–8,Citation10,Citation13] and MgF2 [Citation5,Citation11]. The mechanical properties of polycrystalline and single-crystal spinel ceramics that have been evaluated include the fracture strength [Citation10,Citation12,Citation15,Citation17–20], elastic modulus [Citation3,Citation10,Citation13,Citation19–21], hardness [Citation1,Citation10,Citation12,Citation13,Citation15,Citation17,Citation20,Citation22], fracture toughness [Citation1,Citation2,Citation4,Citation12,Citation15,Citation17,Citation19,Citation20,Citation23–25] and their high-temperature properties [Citation4,Citation18,Citation21,Citation24,Citation25]. Although pressure sintering treatment and sintering aids were found to enhance the transmittance, low fracture strength [Citation18–20] and other mechanical properties [Citation17–20,Citation23,Citation24] tended to occur, caused by the remarkable grain growth and weakening of grain boundaries due to the sintering aids. Also, decrease in the translucency of polycrystalline spinel was found to occur by the generation of a magnesium-rich phase due to the heterogeneous distribution of LiF [Citation6,Citation20]. Fracture toughness of polycrystalline spinel was decreased with increase of grain size due to the change of fracture mode from inter-transgranular to intergranular [Citation19,Citation20,Citation24]. The reason of transition for fracture mode was suspected to the collection of submicron pores and impurities at grain boundary, and cleavage fracture by growth of (1 0 0) plane with low fracture toughness. Although a fine-grained transparent polycrystalline spinel fabricated by the PECS technique without sintering aids showed excellent fracture strength, its transmission in the visible region and fracture toughness were not very high [Citation14,Citation15]. If the fabrication conditions of polycrystalline spinel without pressure sintering or sintering aids can be adjusted, the transmission and mechanical properties may be able to be enhanced by the suppression of grain growth. However, few research studies have been reported focused on the mechanical properties and microstructure of translucent polycrystalline spinel prepared without pressure sintering or sintering aids.
In this study, we focused on the relationship between mechanical properties, such as fracture strength, hardness, and fracture toughness, and the microstructure of transparent polycrystalline spinel ceramics. Several kinds of polycrystalline spinel were fabricated under various heat conditions and with different sintering aids, and single-crystal spinel and sapphire were used for comparison. The grain size and internal residual defect size measured by microstructural observations were investigated. The fracture origin of polycrystalline spinel fabricated under different conditions was estimated based on the fracture strength and toughness.
2. Experimental procedure
2.1. Sample preparation of polycrystalline spinel
Seven types of polycrystalline spinel were prepared by varying the sintering aid and sintering conditions. The commercially starting powder were used high-purity spinel (Baikowski, S30CR, purity >99%) that was prepared by the coprecipitation method, with particle size of about 0.2–0.3 μm, MgF2, and LiF (Kojundo Chemical Laboratory, purity >99.9%) as sintering aids. The starting powder was crushed and mixed using high purity alumina ball as a medium in ethanol solvent. Each powder compact was sintered at 1250°C in a vacuum furnace. The sintered bodies were heated in argon gas by hot isostatic pressing and hot pressing with a sintering aid (SP-Hs1, SP-Hs2, SP-Hs3, and SP-Hs4), by hot isostatic pressing without a sintering aid (SP-H1 and SP-H2), and by vacuum furnace without a sintering aid (SP-V). shows the sintering aids and conditions of polycrystalline spinel fabricated in this study. For comparison with the mechanical properties of polycrystalline spinel, those of single crystal spinel and sapphire were evaluated. Single-crystal spinel and sapphire were prepared by the Verneuil and Czochralski methods, respectively. Fracture testing was performed in (1 0 0) and (1 1 0) plane for fracture surface of single crystal spinel and sapphire, respectively.
Table 1. Sintering conditions and average grain size of fabricated polycrystalline spinel.
2.2. Microstructure and optical property characterization
The fracture surface of polycrystalline spinel after the bending strength test was observed by scanning electron microscopy (SEM, JSM-5200, JEOL Co., Ltd.). The average grain size of polycrystalline spinel was estimated by particle size distribution analysis software (Mac-view Ver.4, Mountech Co., Ltd.) and SEM photographs. The grain diameters on the photograph were investigated by the software based on 300 grains of each specimen. In this study, the average grain size d of polycrystalline spinel was evaluated by the following equation [Citation26]:
where D is the apparent average grain size measured from SEM photographs of the fracture surface. The size of internal defects in polycrystalline spinel was measured by an optical microscope. After the specimen was thinned to a thickness of 1 mm, it was ground to a mirror surface using a 3 μm diamond slurry. The transmission spectrum was measured using a UV-Visible/NIR spectrophotometer (Jasco Co. Ltd., V-670) in the range of 400–1500 nm (diffraction grating switching at 850 nm) and a FTIR spectrometer (Jasco Co. Ltd., FT/IR-4000) in the range of 1500–4000 under air atmosphere.
2.3. Characterization of mechanical properties
The density of the polycrystalline spinel was measured by a method based on Archimedes’ principle using water as an immersion medium. The fracture strength was evaluated by a universal testing machine with an electric furnace (Instron 5582, Instron Japan Co., Ltd.) at room temperature and 1000°C in air. Square rods of dimensions 3 × 4 × 25 mm3 were prepared for the examination at room temperature, and 2 × 2 × 10 mm3 at 1273 K. The test was carried out by the three-point bending method at a crosshead speed of 0.5 mm/min. The three-point bending strength, σb, was calculated as:
where P is the load applied to the specimen, l the distance of lower span, W the width of the specimen, B the thickness of the specimen. l was 16 mm for the room temperature test, and 8 mm for the high-temperature test. The fracture toughness was estimated by the single-edge V-notched beam (SEVNB) method [Citation27]. The test was carried out at room temperature using the same equipment and conditions as the fracture strength test. A square rod of dimensions 2 × 2 × 10 mm3 was prepared for the examination. The specimens for the SEVNB method, which were similar in dimension to those for the bending test, were introduced at the center of the lower surface with a machined sharp V-notch of 0.5 mm length. The radius of curvature at the V-notch tip was about 20 μm. The fracture toughness, KIc, was calculated as:
where V is the width of the specimen, Y is a shape factor at (L/V) = 4.0, and γ the notch length. The Vickers hardness test was carried out by a Vickers hardness meter (Akashi Seisaku-sho Co., AVK-A) with a standard Vickers indenter at an applied load of 49 N (HV5). Vickers hardness was calculated as:
where c is the mean indent half-diagonal length, and F is the load applied to the specimen. The number of specimens for each spinel samples were measured 3 or 4 on bending strength at both temperature and fracture toughness testing, and 5 points on Vickers hardness test.
3. Results and discussion
3.1. Microstructural characterization of polycrystalline spinel
shows the experimental results of density determination by the Archimedes method for sintered polycrystalline spinel and single-crystal spinel. The apparent density of each polycrystalline spinel was almost same and the relative density was up to 99% (theoretical density: 3.58 g·cm−3). This result shows that the polycrystalline spinel could be densified sufficiently without HIP treatment and sintering aids. shows a typical SEM image of the fracture surface around the fracture origin in polycrystalline spinel. The grain size of the fabricated polycrystalline spinel was clearly increased by HIP treatment and the addition of sintering aids. It appears that transgranular fracture mainly occurred for the spinel with small grain size prepared by no use of sintering aids. In contrast, intergranular fracture was dominated to the spinel with large grain size prepared by the addition of sintering aids. Other researchers also reported that the fracture mode of fine-grained polycrystalline spinel was mixed inter-transgranular and that of coarse-grained was only intergranular [Citation19,Citation20]. and show the average grain size of sintered polycrystalline spinel, derived from SEM micrographs and Equation (1). The average grain size could be changed over a wide range between 1.4 and 62.3 μm, and the grains tended to grow with the use of HIP treatment and on addition of sintering aids. The grain size of vacuum – sintered samples (SP-V: 1.4 μm) was the smallest, and the grain size was increased by HIP treatment at the same sintering temperature (SP-H1: 6.6 μm). Although the grain size increased with the temperature of HIP treatment (SP-H2: 7.9 μm), grain growth was suppressed to less than 10 μm. In contrast, the grain growth of polycrystalline spinel fabricated with the addition of sintering aids was drastically increased; the maximum size (SP-Hs4: 62.4 μm) was obtained with the addition of LiF and HP treatment.
Figure 2. (a) SEM photographs of fracture surfaces at room temperature test of polycrystalline spinel specimens prepared without sintering aids. (b)SEM photographs of fracture surfaces at room temperature test of polycrystalline spinel specimens prepared with sintering aids. (c)SEM photographs of fracture surfaces at 1273 K test of polycrystalline spinel specimens prepared without sintering aids. (d)SEM photographs of fracture surfaces at 1273 K test of polycrystalline spinel specimens prepared with sintering aids.

3.2. Optical and mechanical properties of polycrystalline spinel
shows the apparent transmittance of visible light by bar specimens fabricated from polycrystalline spinel with thickness of 3 mm. HIPed SP-H2 without sintering aid and HPed SP-Hs4 with LiF showed high transparency, and SP-V showed low transparency to visible light. HIPed spinel with MgF2 had relatively high transparency, and the highest transparency was obtained with SP-Hs2 prepared by HIP at 1600°C. shows in-line transmittance curves of the visible and infrared transmittance in polycrystalline spinel measured under air atmosphere. SP-Hs4, which was sintered by HP treatment at 1500°C with the addition of LiF, showed the highest transmittance of about 85% from the visible to the infrared region. SP-H2, which was sintered without any sintering aid, showed high transmittance of about 80% from the visible to the infrared region. Few research study reported that polycrystalline spinel fabricated without sintering aids showed 80% transmittance in the visible region [Citation12]. SP-V showed relatively high transmittance of about 70% in the infrared region, even without HIP treatment or sintering aids. SP-Hs1, which was sintered at the lowest temperature at 1300°C, showed the lowest transmittance, about 40–60% from the visible to the infrared region. Though the SP-Hs1 specimen had a high relative density of up to 99%, it showed the lowest values of transmittance and poor mechanical properties. This result suggests that sintering temperature at 1300°C was insufficient for the fabrication of polycrystalline spinel in the present study.
shows the relationship between the average grain size and the bending strength at room temperature for polycrystalline spinel, in comparison with single-crystal spinel and sapphire. The fracture strength of polycrystalline spinel tended to decrease with increasing grain size. The fracture strength of polycrystalline spinel without sintering aids was higher than that of single-crystal spinel; in particular, the value of SP-V (348 MPa) with minimum grain size was higher than that of sapphire. shows the relationship between the average grain size and bending strength at 1000°C in polycrystalline spinel, and a comparison with single-crystal spinel and sapphire. The high-temperature strength of sapphire and that of polycrystalline spinel with over 30 μm grain size were almost same as that at room temperature. On the contrary, the high-temperature strength of single-crystal and polycrystalline spinel with grain size under 30 μm decreased at room temperature. Many studies have measured fracture toughness at high temperature and found that apparent fracture toughness decreased despite the increase in fracture energy by transition to nonlinear fracture above about 800 K [Citation2,Citation4,Citation18,Citation24]. One of the reasons for the decrease in fracture toughness is that the fracture surface changed from inter-trans granular mixed mode at room temperature to intergranular fracture at high temperature in polycrystalline spinels with and without sintering aids [Citation2,Citation4,Citation18]. shows the fracture surface of polycrystalline spinel after 1273 K strength test in this study. Fraction of intergranular fracture appeared to be increased in the fracture surface of samples without sintering aids in comparison with that at room temperature, and the grain boundary was clearly visible in SP-V. In contrast, the samples with sintering aids that occurred almost intergranular fracture at room temperature test fraction of fracture mode appeared to be the same at 1273 K test. If the high-temperature strength is related to the fracture mode transition, it is possible that the strength did not change in the large-grained spinels (SP-Hs2, Hs3, Hs4) with no fracture mode transition under both temperature conditions. However, high-temperature strength decreased in SP-Hs1 despite the fracture mode was not appeared to change. We could not explain to this reason exactly and the further detailed observation of the fracture surface should be required shows the relation of the average grain size and Vickers hardness at room temperature in polycrystalline spinel, along with that of single-crystal spinel and sapphire for comparison. The Vickers hardness of polycrystalline spinel decreased with increasing grain size until 30 μm and increased over 30 μm. The hardness of polycrystalline ceramics with relatively small grain size is controlled by the yield strength of the grains due to the Hall-Petch strengthening effect. This effect for the hardness is given by the following equation [Citation15,Citation28]:
Figure 6. Fracture strength of polycrystalline spinel at room temperature and comparison with single-crystal spinel and sapphire.

Figure 7. Fracture strength of polycrystalline spinel at 1000°C and comparison with single-crystal spinel and sapphire.

Figure 8. (a) Vickers hardness of polycrystalline spinel and comparison with single-crystal spinel and sapphire, (b) optical microscopy observations of Vickers indent for polycrystalline spinel.

where H0 is the intrinsic hardness of the grain, and κ is the Hall-Petch constant. From this relation, the hardness of polycrystalline spinel decreases with grain size. Rice et al. also reported that the Vickers hardness of polycrystalline spinel with widely varying grain size, Hv, showed the minimum value at grain sizes in the 10–50 μm range, and increased again for larger grain size [Citation22]. The dependence of hardness on grain size was similar to the results found in this study. This increase in hardness at larger grain size was attributed to the grain size of polycrystalline spinel being close to the size of indentation. shows optical microscopy observations of indentation and cracking caused by a Vickers indenter for polycrystalline spinel. The diagonal indentation and cracking size of SP-V with the smallest grain size were easily measured by an optical microscope. The diagonal length of SP-H2 could be also measured, but measurement of the cracking length was slightly difficult, because the path of crack propagation was curved and unclear. In the case of SP-Hs4 with the largest grain size, the boundary of indentation was slightly unclear, and the path of cracking propagation could hardly be distinguished. This difficulty of measurement was found with larger grain size (SP-Hs2, Hs3, Hs4). Thus, the Vickers hardness results for these samples lacked accuracy. The fracture toughness measurement by the indentation fracture (IF) method, which was calculated from the cracking propagation length, was difficult to apply with polycrystalline spinel. shows the relation between the average grain size and fracture toughness measured by the SEVNB method at room temperature in polycrystalline spinel, in comparison with the single crystal. Fracture toughness of single crystal spinel was observed to the depend on the fractured surface crystal orientation [Citation19,Citation24,Citation25]. The highest value was about 1.9 MPa·m0.5 at (1 1 1) and 1.2 MPa·m0.5 at (1 0 0). SEVNB test was evaluated 1.26 MPa·m0.5 at (1 0 0) in this study, so that the value was similar to other testing methods. The spinel specimens prepared without sintering aids gave values over 2, and SP-V achieved the maximum value (2.22 MPa·m0.5), which is higher than single crystal spinel at (1 1 1) and close to that of sapphire at (1 1 0). This value is the highest level of fracture toughness compared with other reported data for transparent polycrystalline spinel [Citation1,Citation2,Citation4,Citation12,Citation15,Citation17,Citation19,Citation20,Citation23]. The fracture toughness of polycrystalline spinel tended to decrease with grain size. The decrease of fracture toughness of polycrystalline spinel with increase of grain size was reported by the other studies [Citation19,Citation20,Citation24]. The reason of transition for fracture mode was suspected to the collection of submicron pores and impurities at grain boundary, and cleavage fracture by growth of (1 0 0) plane with low fracture toughness. shows the observation of residual defects in the polycrystalline spinel specimens. Residual defects were mainly observed in polycrystalline spinel fabricated without sintering aids. Because SP-V could not be observed by optical microscopy due to its insufficient transparency to visible light, the defects were estimated by SEM. The apparent size of internal defects was about 10 μm in every spinel regardless of grain size.
3.3. Estimation of fracture strength for polycrystalline spinel
The fracture strength of polycrystalline spinel fabricated using sintering aids was decreased by the weakening of grain boundaries at large grains. Translucent polycrystalline spinel with high strength could be fabricated by minimizing the grain size without sintering aids in this study. However, even in these spinels with higher fracture strength, large-size internal defects were found by microstructural observation. If the fracture strength was decreased by the presence of internal defects, it may be able to be improved by minimizing or eliminating internal defects. Therefore, estimation of fracture strength was carried out for fracture both from a defect at a grain boundary and at an internal void. Weak grain boundaries of coarse grains in polycrystalline spinel have been proposed to act as critical surface defects [Citation17,Citation20]. In the case of a fracture occurring due to a defect in the grain boundary of the specimen at the tensile surface, as shown in Fig. S1, the relationship between fracture toughness and fracture strength, σG was formulated by Takahashi et al. [Citation29]:
where is the fracture toughness and φ the defect size at the grain boundary. In this study, φ was assumed to be n times the average grain size (φ = nd). shows a comparison of experimental data and the fracture strength σG calculated from Eq. (6). The experimental strength was in relatively good accordance with that of medium grain size (SP-H1, H2, Hs1) spinels at n = 1, which means the defect size is the same as the grain size. On the other hand, the experimental strength of SP-V with small grain size was much lower and those of large grain size (SP-Hs2, Hs3, Hs4) spinels were higher than the estimated fracture strength. This suggested that the fracture mechanism of SP-V was different from that of the other spinels. One possible reason for the difference between the experimental and estimated strengths for the large grain-size spinel was the R-curve effect in the fracture strength test. The fracture strength test for the spinels with larger grain size was affected strongly by the R-curve effect due to the non-linear crack propagation by intergranular fracture [Citation18]. However, the fracture toughness measured by SEVNB test that was contained to R-curve effect was decreased with increase of grain size in this study as shown in . Chantikul et al. evaluated that the increasing rate of toughness curve with crack size was larger as the grain size of alumina [Citation30]. The cracking length of fracture strength test was expected longer than that of SEVNB due to difference specimen sizes between both test, R-curve effect might be emphasized at fracture strength test of large grain size spinels. Therefore, the fracture toughness KIc was corrected to KR, which considered the bridging effect, using the equation reported by Chnatikul et al. [Citation30]:
Figure 11. Comparison between experimental strength and estimated fracture strength in the case of fracture from defect at grain boundary in polycrystalline spinel.

where E is the elastic modulus [Citation19], Ki the intrinsic fracture toughness, d the average grain size, εl the bridge rapture strain, μ the coefficient of sliding friction, σR the magnitude of internal stress, and αd the ratio of bridge-spacing to grain-size. It is difficult to evaluate intrinsic fracture toughness Ki of polycrystalline spinels in this study, average value, 1.54 MPa·m0.5 of single crystal spinel at three planes [Citation19,Citation24,Citation25] was adopted for the convenience. Several numerical factors εl, μ and αd were referenced from the values of polycrystalline alumina, which was subject to intergranular fracture, as well as polycrystalline spinel used in this study. By substituting KR calculated from Eq. (7) for KIc of Eq. (6), the fracture strength σG at n = 1 with KR was estimated as shown in . The estimated fracture strength calculated from eq. (7) was in relatively good accordance with the experimental strength of spinel with large grain size. It is suggested that polycrystalline spinel specimens with large grain size fabricated with sintering aids have intrinsic low fracture strength due to their low fracture toughness and large grain size, but it might be enhanced by the appearance of the additional R-curve effect [Citation31].
In the case of fracture occurring from an internal spherical void, the relationship between the stress intensity factor and stress occurring at a peripheral crack around an internal spherical void, as shown in Fig. S2, was analyzed by Baratta [Citation32].
where KIc is the fracture toughness, υ the Poisson’s ratio, r the radius of a spherical void, L the circumferential crack length around the void, and c, k, m numerical constants. In this study, the calculation of L assumed that the grain boundary existing around the spherical void was n times the average grain size (L = nd). The apparent internal defect size of polycrystalline spinel was measured as 10 µm from the result shown in , and 2r was estimated to be 15.7 µm calculated from eq. (1) as well as calculation of the grain size. shows a comparison of experimental data and the fracture strength σd calculated from Eq. (8). The experimental strength was in good accordance with that of SP-V at n = 1, which corresponded to a defect size the same as the grain size. Medium grain-size (SP-H1, H2, Hs1) spinels also showed relatively good agreement. This suggested that the fracture of SP-V could have occurred from residual spherical voids. In the case of a fracture occurring from a residual spherical void, if the size of the void is minimized, the fracture strength may be improved further. If the fracture strength of spinel fabricated without sintering aid, for example, SP-H2 in this study, is improved further, polycrystalline spinel having both high transmission in the visible and infrared regions and high mechanical properties could be achieved.
4. Summary
Polycrystalline spinels for application in infrared light transmission windows with good mechanical properties were fabricated by variation of heat conditions and sintering aids. The relative density of every spinel was as high as 99%, and a spinel fabricated by vacuum sintering could be densified without pressure sintering or sintering aids. The average grain size could be changed gradually between 1.4 and 62.3 μm and tended to grow the most with HIP treatment and the addition of sintering aids. Spinels fabricated by HP with LiF sintering aid and HIPed at 1600°C without sintering aid showed high visible and infrared transmittances above 80%. SP-V without HIP treatment and sintering aid had relatively high transmittance of infrared light. The fracture strength of some spinel specimens with small grain size without sintering aids was higher than that of single-crystal spinel, and the strength of the spinel specimen with minimum grain size (SP-V) was higher than that of sapphire. The fracture toughness measured by SEVNB of polycrystalline spinel prepared without sintering aids was higher than that of spinel with sintering aids. High-temperature strength was decreased mainly polycrystalline spinel with small grain size due to the transition of fracture mode from room temperature. By estimation of the fracture strength considering the different fracture origins, the polycrystalline spinel specimens prepared with sintering aids were fractured from defects at grain boundaries at the tensile surface. The polycrystalline spinel specimens prepared without sintering aids might be fractured mainly from defects around residual spherical voids. The size of these defects was estimated to be almost equivalent to the grain size. If the size of voids is minimized, the fracture strength may be improved further. If the fracture strength of spinel fabricated without sintering aid can be further improved, polycrystalline spinel having both high transmission and good mechanical properties could be achieved.
Supplemental Material
Download Zip (654.8 KB)Disclosure statement
No potential conflict of interest was reported by the authors.
Supplementary material
Supplemental data for this article can be accessed online at https://doi.org/10.1080/21870764.2023.2248714
References
- Dericioglu AF, Boccaccini AR, Dlouhy I, et al. Effect of chemical composition on the optical properties and fracture toughness of transparent magnesium aluminate spinel ceramics. Mater Trans. 2005;46(5):996–1003. doi: 10.2320/matertrans.46.996
- Stewart RL, Bradt RC. Fracture of polycrystalline MgAl2O4. J Am Ceram Soc. 1980;63(11–12):619–623. doi: 10.1111/j.1151-2916.1980.tb09847.x
- Porter DF, Reed JS, Lewis D. Elastic moduli of refractory spinel. J Am Ceram Soc. 1977;60(7–8):345–349. doi: 10.1111/j.1151-2916.1977.tb15558.x
- White KW, Kelkar GP. Fracture mechanisms of a coarse-grained, transparent MgAl2O4 at elevated temperatures. J Am Ceram Soc. 1992;75(12):3440–3444. doi: 10.1111/j.1151-2916.1992.tb04446.x
- Goldstein A, Raethel J, Katz M, et al. Transparent MgAl2O4/LiF ceramics by hot-pressing: Host–additive interaction mechanisms issue revisited. J Eur Ceram Soc. 2016;36(7):1731–1742. doi: 10.1016/j.jeurceramsoc.2016.02.001
- Villalobos GR, Sanghera JS, Aggarwal ID. Degradation of magnesium aluminum spinel by lithium fluoride sintering aid. J Am Ceram Soc. 2005;88(5):1321–1322. doi: 10.1111/j.1551-2916.2005.00209.x
- Rozenburg K, Reimanis IE, Kleebe JH, et al. Sintering kinetics of a MgAl2O4 spinel doped with LiF. J Am Ceram Soc. 2008;91(2):444–450. doi: 10.1111/j.1551-2916.2007.02185.x
- Rubat du Merac M, Reimanis IE, Smith C, et al. Effect of impurities and LiF additive in hot-pressed transparent magnesium aluminate spinel. Int J Appl Ceram Technol. 2013;10:E33–E48. doi: 10.1111/j.1744-7402.2012.02828.x
- Tsukuma K. Transparent MgAl2O4 spinel ceramics produced by HIP post-sintering. J Ceram Soc Jpn. 2006;114:802–806.
- Goldstein A, Krell A, Kleebe A. Transparent ceramics at 50: Progress made and further prospects. J Am Ceram Soc. 2016;99(10):3173–3197. doi: 10.1111/jace.14553
- Ikesue A, Aung YL. Advanced spinel ceramics with highest VUV-vis transparency. J Eur Ceram Soc. 2020;40(6):2432–2438. doi: 10.1016/j.jeurceramsoc.2020.01.062
- Krell A, Klimke J, Hutzler T. Advanced spinel and sub-μm Al2O3 for transparent armour applications. J Eur Ceram Soc. 2009;29(2):275–281. doi: 10.1016/j.jeurceramsoc.2008.03.024
- Frage N, Cohen S, Meir S, et al. Spark plasma sintering (SPS) of transparent magnesium-aluminate spinel. J Mater Sci. 2007;42(9):3273–3275. doi: 10.1007/s10853-007-1672-0
- Morita K, Kim BN, Yoshida H, et al. Spark-plasma-sintering condition optimization for producing transparent MgAl2O4 spinel polycrystal. J Am Ceram Soc. 2008;92(6):1208–1216. doi: 10.1111/j.1551-2916.2009.03074.x
- Morita K, Kim BN, Hiraga K, et al. Fabrication of high-strength transparent MgAl2O4 spinel polycrystals by optimizing spark-plasma-sintering conditions. J Mater Res. 2009;24(9):2863–2872. doi: 10.1557/jmr.2009.0335
- Bratton RJ. Translucent sintered MgAl2O4. J Am Ceram Soc. 1974;57(7):283–286. doi: 10.1111/j.1151-2916.1974.tb10901.x
- Mroz T, Goldman LM, Gledhill AD, et al. Nanostructured, infrared-transparent magnesium-aluminate spinel with superior mechanical properties. Int J Appl Ceram Technol. 2012;9(1):83–90. doi: 10.1111/j.1744-7402.2011.02629.x
- Ghosh A, White KW, Jenkins MG, et al. Fracture resistance of a transparent magnesium aluminate spinel. J Am Ceram Soc. 1991;74(7):1624–1630. doi: 10.1111/j.1151-2916.1991.tb07149.x
- Salem JA, Sglavo V. Transparent armor ceramics as spacecraft windows. J Am Ceram Soc. 2013;96(1):281–289. doi: 10.1111/jace.12089
- Rubat du Merac M, Kleebe HJ, Müller MM, et al. Fifty years of research and development coming to fruition; unraveling the complex interactions during processing of transparent magnesium aluminate (MgAl2O4) spinel. J Am Ceram Soc. 2013;96(11):3341–3365. doi: 10.1111/jace.12637
- Askarpour V, Manghnani MH, Fassbender S, et al. Elasticity of single-crystal MgAl2O4 spinel up to 1273 K by Brillouin spectroscopy. Phys Chem Miner. 1993;19(8):511–519. doi: 10.1007/BF00203051
- Rice RW, Wu CC, Boichelt F. Hardness-grain-size relations in ceramics. J Am Ceram Soc. 1994;61(10):2539–2553. doi: 10.1111/j.1151-2916.1994.tb04641.x
- Rice RW, Wu CC, McKinney KR. Fracture and fracture toughness of stoichiometric MgAl2O4 crystals at room temperature. J Mater Sci. 1996;31(5):1353–1360. doi: 10.1007/BF00353117
- Sakai M, Bradt RC, Kobayashi AS. The toughness of polycrystalline MgAl2O4. J Ceram Soc Jpn. 1988;96:525–531.
- Stewart RL, Bradt RC. Fracture of single crystal MgAl2O4. J Mater Sci. 1980;15(1):67–72. doi: 10.1007/BF00552428
- Mendelson ML. Average grain size in polycrystalline ceramics. J Am Ceram Soc. 1969;52(8):443–446. doi: 10.1111/j.1151-2916.1969.tb11975.x
- Awaji H, Sakaida Y. V-notch technique for single-edge notched beam and chevron notch methods. J Am Ceram Soc. 1990;73(11):3522–3523. doi: 10.1111/j.1151-2916.1990.tb06490.x
- Ehre D, Chaim R. Abnormal Hall–Petch behavior in nanocrystalline MgO ceramic. J Mater Sci. 2008;43(18):6139–6143. doi: 10.1007/s10853-008-2936-z
- Takahashi I, Usami S, Nakakado K, et al. Effect of defect size and notch root radius on fracture. J Ceram Soc Jpn. 1985;93:186–194.
- Chantikul P, Bennison SJ, Lawn BR. Role of grain size in the strength and R-curve properties of alumina. J Am Ceram Soc. 1990;73(8):2419–2427. doi: 10.1111/j.1151-2916.1990.tb07607.x
- Baudín C, Martínez R, Pena P. High-temperature mechanical behavior of stoichiometric magnesium spinel. J Am Ceram Soc. 1995;78(7):1857–1862. doi: 10.1111/j.1151-2916.1995.tb08900.x
- Baratta FI. Stress intensity factor estimates for a peripherally cracked spherical void and a hemispherical surface pit. J Am Ceram Soc. 1978;61(11–12):490–493. doi: 10.1111/j.1151-2916.1978.tb16124.x