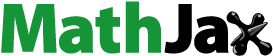
ABSTRACT
Piezoelectric sensors for underwater sonar require high hydrostatic piezoelectric properties (dh, gh and HFOM), and research is being conducted on various types of piezoelectric composites to improve them. In this study, research was conducted to improve the hydrostatic piezoelectric properties of 1–3 type piezoelectric composites using PZT piezoelectric ceramics and polymers. First, the appropriate material and volume fraction of the piezoelectric ceramic were selected through numerical analysis, and then experimental specimens were produced by applying various porosity of the polymer matrix. As a result of the experiment, it was confirmed that dh increased by 75% as the porosity of the polymer matrix increased. This phenomenon was analyzed to be caused by a change in elastic modulus. In addition, gh and HFOM also increased by 70% and 210% as the porosity of the polymer matrix increased. These results experimentally demonstrated that controlling the porosity of the polymer matrix is useful for improving the hydrostatic piezoelectric properties of type 1–3 piezoelectric composites.
1. Introduction
PZT is a representative piezoelectric material commonly employed in various sensor applications. Specifically, for piezoelectric sensors used in underwater application, there is a requirement for high hydrostatic piezoelectric characteristics, including dh, gh, and HFOM (hydrostatic figure of merit). However, single-phase PZT piezoelectric ceramics suffer from the drawback of having low hydrostatic piezoelectric properties. Consequently, numerous efforts have been undertaken to enhance hydrostatic piezoelectric properties. A representative example is a PZT-polymer composite material in which PZT piezoelectric ceramics and polymers are arranged in a composite form [Citation1].
Piezoelectric composites come in various shapes, including 0–3 type, 1–3 type, 2–2 type, 2–3 type and 3–3 type, depending on the connection type of piezoelectric ceramic and polymer matrix, and several related studies have been reported [Citation2–5]. Among them, the 1–3 type piezoelectric composite is a structure in which a piezoelectric ceramic pillar is inserted into a polymer matrix, as shown in , and the dielectric and piezoelectric properties vary depending on the volume fraction of the piezoelectric ceramic pillar and the polymer matrix. Additionally, it exhibits high hydrostatic piezoelectric properties compared to piezoelectric composites of other shapes [Citation6–8].
Various methods are being used to predict the hydrostatic piezoelectric properties of these 1–3 type piezoelectric composites, but research based on numerical analysis is being actively reported. Among them, Smith et al. [Citation9] theoretically calculated the physical properties of 1–3 type piezoelectric composites using a numerical analysis model developed with the equivalent circuit method. According to these studies, the physical properties and volume fraction of piezoelectric ceramics were found to affect the hydrostatic piezoelectric properties of 1–3 type piezoelectric composites. In addition, Christian N. Della et al. [Citation10,Citation11] calculated the effect of increasing the porosity of the polymer matrix on the hydrostatic piezoelectric properties of 1–3 type piezoelectric composites using a numerical model.
In this study, to improve the hydrostatic piezoelectric properties of type 1–3 piezoelectric composites, specimens using a porous polymer matrix were fabricated and their characteristics were analyzed. To design the piezoelectric composite, the hydrostatic piezoelectric properties were calculated according to the type and volume fraction of the piezoelectric ceramic using numerical analysis, and the material and volume fraction with the best performance were selected. Based on the selected piezoelectric ceramic and volume fraction, a 1–3 type piezoelectric composite using a porous polymer matrix was fabricated, and a microsphere was used as a medium for forming pores in the polymer matrix. Finally, the effect of the porosity of the polymer matrix of the fabricated 1–3 piezoelectric composite with a porous polymer matrix on the hydrostatic piezoelectric properties was analyzed.
2. Method
2.1. Numerical analysis method for design 1–3 type piezoelectric composites
Numerical analysis was conducted to design a piezoelectric composite with improved hydrostatic piezoelectric properties by calculating the dielectric and piezoelectric properties using the following piezoelectric equation [Citation9,Citation12,Citation13].
Here, s represents the elastic compliance matrix, V represents the volume fraction of the piezoelectric ceramic, ε represents the dielectric constant, and d represents the piezoelectric charge constant. Additionally, the superscripts p and m indicate piezoelectric ceramic and polymer matrices, respectively.
Additionally, the superscripts E and T represent constant electric field and constant stress, respectively.
The piezoelectric charge constants (d31 and d33) obtained in EquationEquations 3(3)
(3) and Equation4
(4)
(4) are calculated as hydrostatic piezoelectric charge constant (dh) by Equationequation (5)
(5)
(5) . Continuously calculate the hydrostatic piezoelectric voltage constant (gh) and HFOM (Hydrostatic figure of merit) using Equationequations (6)
(6)
(6) and (Equation7
(7)
(7) ).
The software used for the numerical analysis technique is Mathematica SW ver.12.0 [Citation14]. The variables for numerical analysis were calculated with the volume fraction (V) of two types of piezoelectric ceramics (PZT-5A, PZT-5 H) in the range of 0 to 100%, and the material properties of each piezoelectric ceramic are shown in [Citation15]. The polymer matrix used was a commercial Araldite-based CY1300/HY1301, and its material properties are presented in . Based on the hydrostatic piezoelectric properties calculated through numerical analysis, a piezoelectric material with excellent hydrostatic piezoelectric properties and its volume ratio were determined.
Table 1. Material properties of piezoelectric materials for numerical analysis.
Table 2. Material properties of polymer matrix for numerical analysis.
2.2. Fabrication method of porous polymer-applied 1–3 type piezoelectric composite
shows the process for fabricating a 1–3 type piezoelectric composite using a porous polymer. The process occurs in the following order : Piezo-preform array → polymer curing → grinding → electroding → poling. First, powder injection molding (PIM) was used to produce the piezo-preform, and the process diagram is shown in . The PIM process consists of mixing, injection, degreasing and sintering processes. The material (feedstock) for PIM was used by mixing PZT piezoelectric ceramic and organic binder. The average particle size of the PZT piezoelectric ceramic used was about 3 μm, and LDPE, EVA, and wax were used as organic binders. The feedstock was molded into a green body for piezo-preform through a powder injection molding machine (NEX-500, Nissei). The green body of the piezo-preform has a pillar diameter of approximately 1.2 mm, as shown in (a). Afterward, the green body was placed in n-heptane and kept at 40°C for 24 hours to remove more than 95% of the wax. LDPE and EVA were heated to 500°C in an electric furnace at a rate of 0.5°C per minute and maintained for 2 hours to vaporize. After all organic binders were removed through solvent and heat debinding process, sintering was performed at 1250°C for 2 hours, and the sintered piezo-preform is shown in (b). The diameter of the piezo-preform column showed a value of 1 mm, which was about 16% shrinkage compared to the green body.
To prepare the porous polymer matrix, polymer (CY1301/HY1301, Robnor) and microsphere (Expancel 461 DET 40 d25, AkzoNebel) were used. This polymer is the same material as the polymer used in the numerical analysis, and the mixing volume ratio of resin (CY1300) and hardner (HY1301) is 2.68:1. The microsphere has a density of 30 kg/m3 and a particle size of 40um, as shown in . In order for the polymer matrix to have a final porosity of 0% to 30%, the volume ratios of polymer to microspheres were set at 10:0, 9:1, 8:2, and 7:3. The materials prepared according to each mixing ratio were placed in a paste mixer (HPM-1.5S, HANtech) and mixed uniformly at 1,000 RPM for 5 minutes. The mixed polymer matrix was added to the piezo-preform and completely cured for 48 hours at room temperature. The cured specimen was processed to the standard specimen size (20 × 20 × 5(t)㎜) for measuring dh, and Cu electrodes were attached to the upper and lower parts using silver epoxy. Afterwards, the direct-current (DC) poling was performed in silicone oil bath at 2.5kV/mm for 15 minutes. shows the 1–3 type piezoelectric composite specimens fabricated according to the porosity of the polymer matrix.
Figure 5. Fabricated piezoelectric composite according to the porosity of the polymer matrix : (a) 0%, (b)10%, (c) 20% and (d) 30%.

Table 3. Characteristics of microspheres used to create pores in the polymer matrix.
A dh-meter (PM-300 H, Piezotest) was used as the measuring equipment to analyze the hydrostatic pressure characteristics of the manufactured specimen, and a schematic diagram of the measuring equipment is shown in . The measurement principle involves applying low-frequency air pressure of 110 Hz to a specimen placed in a sealed container, measuring the amount of internal charge generated, and evaluating dh and dielectric constant. Additionally, the dielectric constant is calculated using Equationequation (8)(8)
(8) based on the measured capacitance (Cp) and the specimen’s size, and gh is derived according to Equationequation (6)
(6)
(6) in section 2.
Here, A represents the area of the specimen, t represents the thickness of the specimen, and represents the permittivity of the vacuum.
3. Result and discussion
3.1. Numerically calculated hydrostatic piezoelectric properties of 1–3 type composite according to volume fraction of PZT
shows the results of the dh value according to PZT calculated using numerical analysis. As a result of the analysis, the dh of PZT-5 H rapidly increased up to 20% of the volume fraction and then began to decrease, while the dh of PZT-5A increased up to 20% and then stabilized at a constant value.
Figure 7. The dh value according to the PZT volume fraction of 1–3 type piezoelectric composite calculated by numerical analysis method.

The piezoelectric charge constants (d33 and d31) of the piezoelectric composite increase as the volume fraction of piezoelectric ceramic increases, as described by Equationequations (3)(3)
(3) to (Equation4
(4)
(4) ). Additionally, dh is represented in the form of d33 +2d31, as shown in Equationequation (5)
(5)
(5) of Section 2, with d31 having a negative value. Consequently, it can be inferred that in regions where the volume fraction of piezoelectric ceramic is low (0% to 20%), the value of d33 increases significantly in comparison to -d31, thus contributing to the increase in dh. These results exhibited a similar trend to those analyzed by AVELLANEDA et al. [Citation16]. Furthermore, at a 20% volume fraction of piezoelectric ceramic, PZT-5 H demonstrated a dh value approximately three times higher than that of PZT-5A. This difference can be attributed to the high d33 characteristic of PZT-5 H, which is a relatively soft material (refer to ). It appears to have induced a more significant change in value compared to PZT-5A.
displays the gh results, indicating that both materials exhibit a rapid decrease up to 30% and then stabilize at a constant value. This trend can be attributed to the linear increase in the dielectric constant as the volume fraction of piezoelectric ceramic increases.
Figure 8. The gh value according to the PZT volume fraction of 1–3 type piezoelectric composite calculated by numerical analysis method.

As shown in , the HFOM of both PZT-5A and PZT-5 H exhibited a rapid increase when the volume fraction of piezoelectric ceramic changed from 0% to 10%, after which it began to decrease. Notably, at a 10% volume fraction of piezoelectric ceramic, the HFOM value for PZT-5 H reached approximately 2500 pC/N, more than five times higher than that of PZT-5A. Consequently, for the fabrication of a 1–3 type piezoelectric composite with improved hydrostatic piezoelectric properties, PZT-5 H was chosen as the piezo-ceramic material, and the volume fraction of piezoelectric ceramic was determined to be 10%.
3.2. Hydrostatic piezoelectric properties of 1–3 type piezoelectric composite according to the porosity of the polymer matrix
This section analyzes the hydrostatic piezoelectric properties of specimens fabricated using the method described in section 2.2. The volume fraction of PZT-5 H for fabricate the specimen was 10%, as shown in section 3.1, and the porosity of the polymer matrix was 0%, 10%, 20%, and 30%.
shows the microstructure of type 1–3 piezoelectric composite with a polymer matrix porosity of 30%. The shape of the pores was almost spherical, and the sizes varied from very small pores up to 30um. This difference in pore size appears to be due to the fact that the positions where the pores are cut are different depending on the thickness of the specimen.
shows the dh measurement results as a function of the porosity of the polymer matrix, demonstrating a tendency to increase by approximately 75%, ranging from 100 pC/N to 175 pC/N, as the porosity increased from 0% to 30%. This phenomenon is presumed to occur due to the reduction in the elastic modulus as the porosity of the polymer matrix increases, leading to an increase in the d33 value. Furthermore, the d33 value of 1–3 type piezoelectric composites is commonly influenced by three factors: the piezoelectric charge constant of the ceramic material, the volume fraction of the ceramic, and the elastic modulus of the polymer matrix [Citation17]. The pores within the polymer matrix of this specimen reduce the connectivity between polymers, resulting in a decrease in the elastic modulus of the polymer matrix [Citation18]. It can be inferred that this reduced elastic modulus of the polymer matrix will affect the increase in the displacement of the piezoelectric composite in response to external stress, thereby leading to an increase in d33. Similar results were also observed in a study by Christian N. Della et al., where they assessed the impact of a porous polymer matrix through numerical analysis [Citation10].
displays the gh value, which exhibited a tendency to increase by approximately 70%, ranging from 16 mV·m/N to 27 mV·m/N, as the porosity increased from 0% to 30%. The gh value is inversely proportional to the dielectric constant, and the dielectric constant of a 1–3 type piezoelectric composite depends on both the type and the volume fraction of the piezoelectric ceramic [Citation19]. This is attributed to the significant disparity in dielectric constants between the piezoelectric ceramic and the polymer matrix. As shown in , the dielectric constant of the PZT-5 H used in this study being approximately 850 times higher than that of the polymer. In other words, for specimens where the type and volume fraction of piezoelectric ceramics are fixed, the impact of the polymer matrix’s porosity on the dielectric constant can be considered negligible. Therefore, it was analyzed that the variation in the gh value in response to changes in the polymer matrix’s porosity exhibits a similar trend to the change in the dh value. Finally, shows the HFOM value, which is the product of dh and gh, and increased from 1620 fPa−1 to 5035 fPa−1 as the porosity increased from 0 to 30%.
Through this study, it was experimentally confirmed that increasing the porosity of the polymer matrix has an effect on improving the hydrostatic piezoelectric properties of 1–3 type piezoelectric composites.
4. Conclusion
In this study, we manufactured type 1–3 piezoelectric composite specimens using a porous polymer matrix and analyzed their hydrostatic piezoelectric properties based on the matrix’s porosity.
Initially, the hydrostatic piezoelectric properties of 1–3 type piezoelectric composites according to material and volume fraction were analyzed through numerical analysis. The results indicated that the highest hydrostatic piezoelectric properties were achieved when using PZT-5 H as the piezoelectric ceramic material with a 10% volume fraction. Subsequently, we produced specimens based on these findings.
To introduce pores into the polymer matrix, we employed microspheres and varied the polymer matrix’s porosity from 0% to 30% in 10% intervals. Consequently, the dh and gh values of the type 1–3 piezoelectric composite produced in this study increased by 75% and 70%, respectively, as the porosity ranged from 0% to 30%. Moreover, the HFOM value increased from 1620 fPa−1 to 5035 fPa−1 with increasing porosity from 0% to 30%. Therefore, it was analyzed that the pores of the polymer matrix have a significant impact on the improvement of the hydrostatic piezoelectric properties of type 1–3 piezoelectric composites.
Acknowledgments
The authors acknowledge the financial support from the Virtual Engineering Platform Project of the Ministry of Trade, Industry and Energy(MOTIE) of Korea(grant number : P0022336) and KICET own policy project.
Disclosure statement
No potential conflict of interest was reported by the author(s).
Additional information
Funding
References
- Akdogan EK, Allahverdi M, Safari A. Piezoelectric composites for sensor and actuator applications. IEEE Trans Ultrason Ferroelectr Freq Control. 2005;52(5):746–775. doi: 10.1109/TUFFC.2005.1503962
- Newnham R, Skinner D, Cross L. Connectivity and piezoelectric-pyroelectric composites. Mater Res Bull. 1978;13(5):525–536. doi: 10.1016/0025-5408(78)90161-7
- Stevenson JW, Reidmeyer MR, Huebner W. Fabrication and characterization of PZT/Thermoplastic polymer composites for high‐frequency phased linear arrays. J Am Ceram Soc. 1994;77(9):2481–2484. doi: 10.1111/j.1151-2916.1994.tb04628.x
- Smith WA, editor the role of piezocomposites in ultrasonic transducers. Proceedings, IEEE Ultrasonics Symposium; 1989: IEEE. DOI: 10.1109/ULTSYM.1989.67088
- Fiore D, Gentilman R, Pham H, et al., editors. Recent developments in 1-3 piezocomposite transducer fabrication. ISAF’96 Proceedings of the Tenth IEEE International Symposium on Applications of Ferroelectrics; 1996: IEEE. DOI: 10.1109/ISAF.1996.602806
- Lee HJ, Zhang S. Design of low-loss 1-3 piezoelectric composites for high-power transducer applications. IEEE Trans Ultrason Ferroelectr Freq Control. 2012;59(9):1969–1975. doi: 10.1109/TUFFC.2012.2415
- Nan C-W, Weng G. Influence of polarization orientation on the effective properties of piezoelectric composites. J Appl Phys. 2000;88(1):416–423. doi: 10.1063/1.373675
- Hayward G, Bennett J. Assessing the influence of pillar aspect ratio on the behavior of 1-3 connectivity composite transducers. IEEE Trans Ultrason Ferroelectr Freq Control. 1996;43(1):98–108. doi: 10.1109/58.484469
- Smith WA, Shaulov A, Auld B, editor Tailoring the properties of composite piezoelectric materials for medical ultrasonic transducers. IEEE 1985 Ultrasonics Symposium; San Francisco, CA, USA. IEEE; 1985.
- Della CN, Shu D. Performance of 1–3 piezoelectric composites with porous piezoelectric matrix. Appl Phys Lett. 2013;103(13):132905. doi: 10.1063/1.4822109
- Della CN, Shu D. The performance of 1–3 piezoelectric composites with a porous non-piezoelectric matrix. Acta Materialia. 2008;56(4):754–761. doi: 10.1016/j.actamat.2007.10.022
- Chan HLW, Unsworth J. Simple model for piezoelectric ceramic/polymer 1-3 composites used in ultrasonic transducer applications. IEEE Trans Ultrason Ferroelectr Freq Control. 1989;36(4):434–441. doi: 10.1109/58.31780
- Taunaumang H, Guy I, Chan H. Electromechanical properties of 1‐3 piezoelectric ceramic/piezoelectric polymer composites. J Appl Phys. 1994;76(1):484–489. doi: 10.1063/1.357099
- Mathematica WRI. Version 12.0. Wolfram research, inc. Champaign, IL: Wolfram Research, Inc; 2019.
- Berlincourt D, Krueger H, Near C. Properties of Morgan electro ceramic ceramics. Technical Publication TP-226: Morgan Electro Ceramics; 2000. http://www.ultrasonic-resonators.org/misc/references/articles/Berlincourt__'Properties_of_Morgan_Electro_Ceramic_Ceramics'_(Morgan_Technical_Publication_TP-226).pdf
- Avellaneda M, Swart PJ. Calculating the performance of 1–3 piezoelectric composites for hydrophone applications: an effective medium approach. J Acoust Soc Am. 1998;103(3):1449–1467. doi: 10.1121/1.421306
- Kei-Yu Z, Xing-Yun J, Bao-Song W, editors Study on the Stress Stability of PZT Composites. Sixth IEEE International Symposium on Applications of Ferroelectrics; 1986: IEEE. DOI: 10.1109/ISAF.1986.201135
- Gentilman RL, Fiore D, Pham-Nguyen H, et al., editors. Manufacturing of 1-3 piezocomposite SonoPanel transducers. Smart structures and materials 1995: industrial and commercial applications of smart structures technologies; 1995: SPIE. 10.1117/12.209340
- Jayendiran R, Arockiarajan A. Modeling of dielectric and piezoelectric response of 1-3 type piezocomposites. J Appl Phys. 2012;112(4). doi: 10.1063/1.4748057