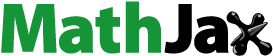
ABSTRACT
In this study, the long-term resistance of Ce-TZP/Al2O3 specimens to hydrothermal treatment is evaluated in terms of their microstructure. Treatment of the specimens is carried out in an autoclave with water at 134°C for up to 360 h. The biaxial strength and Weibull modulus of the specimens remains unchanged after 120 h of hydrothermal treatment, and their pseudo-plasticity is preserved. However, after 360 h of hydrothermal treatment the biaxial strength decreases by 12% and the Weibull modulus is also decreased. The transformation surface layer is observed through scanning electron microscopy (SEM) and transmission electron microscopy (TEM). The thickness of the transformation layer can be estimated only through TEM analysis, revealing minimal microcrack formation at the surface layer. For comparison, several Y-TZP specimens are also prepared. After 24 h of hydrothermal treatment, the monoclinic phase content in the Y-TZP specimen reached 60%, resulting in a 40% decrease in strength. This strength decrease can be attributed to microcrack formation in the surface layer.
1. Introduction
The mechanical properties of yttria-stabilized tetragonal zirconia (Y-TZP) are superior in comparison to other ceramics, making it a widely used material in various applications, such as optical connectors [Citation1] and biomedical implants [Citation2–4]. The excellent mechanical properties of Y-TZP can be attributed to the presence of a metastable tetragonal (t) phase at room temperature. However, when this metastable phase comes in contact with water, it tends to transform into a monoclinic (m) phase [Citation5,Citation6]. This phase transformation is accompanied by a 4% volume expansion [Citation7,Citation8], leading to the development of stresses and even microcracks. The formation of microcracks adversely affects the strength [Citation9]; this is referred to as low-temperature degradation (LTD).
The applications of tetragonal zirconia polycrystal (TZP) ceramics are limited because of their susceptibility to degradation. For example, a large number of hip-joint prostheses were recalled in 2003 because of the LTD of Y-TZP femoral heads [Citation6]. Such degradation has also caused communication noise in optical connectors [Citation1]. Many approaches have been explored and implemented to address this issue. Some of these approaches include:
Introducing a second phase with high elastic modulus. In this approach, the volume expansion of zirconia during phase transformation is constrained by rigid surroundings [Citation10]. The use of a second phase with high elastic modulus, e.g., alumina, has been shown to be effective. However, this method may introduce internal stresses within the composite [Citation5].
Reducing the sintering temperature. During the sintering of Y-TZP at elevated temperatures (>1450°C), yttrium ions may segregate to grain boundaries [Citation11]. Such segregations deplete the concentration of oxygen vacancies within ZrO2 grains, resulting in higher m-phase content. Through the addition of a small amount (0.1–1 mol%) of the sintering additive, Al2O3, the sintering temperature can be reduced by more than 100°C [Citation12] and the LTD resistance of Y-TZP can be improved.
Using other stabilizers. The LTD resistance of TZPs with alternative stabilizers has been evaluated. Ceria-stabilized zirconia (Ce-TZP) exhibits low degradation at low temperatures [Citation13]. However, the rapid grain growth of Ce-TZP during sintering results in lower strength [Citation14]. The grain growth at elevated temperatures can be inhibited through the addition of a second phase [Citation15]. Previous studies have demonstrated that the addition of 30 vol% Al2O3 particles was effective in inhibiting the growth of ZrO2 grains [Citation16–18].
Among these approaches, the use of ceria as a stabilizer for TZP is a promising strategy [Citation16–18]. The charge of the Ce4+ ion is the same as that of Zr4+, eliminating the need for additional oxygen vacancies to accommodate the incorporation of CeO2 into ZrO2. With a reduced number of oxygen vacancies, the transport of OH− or O2−, resulting from the decomposition of water [Citation6], is less likely. Previous studies have confirmed the LTD resistance of Ce-TZP/30%Al2O3 specimens was indeed improved [Citation17]. Although the decrease in strength of Ce-TZP/Al2O3 specimens after being soaked in water is minimal, phase transformation in a hydrothermal environment still occurs. More importantly, a detailed characterization of the microstructure of the transformation surface layer of Ce-TZP/Al2O3 specimens has yet to be performed. In the present study, the long-term resistance of Ce-TZP/Al2O3 specimens to hydrothermal degradation was evaluated and the microstructures of the transformation layers of the specimens were characterized.
The degradation of TZP depends heavily on the details of its processing technique [Citation19–23]. For example, the transition from a muffle furnace to a conveyor furnace significantly reduces the LTD resistance [Citation6]. In order to evaluate the degradation of Ce-TZP/Al2O3 specimens in water, a comparison to the results for Y-TZP would be of interest. Therefore, some Y-TZP specimens were prepared as reference specimens.
2. Experimental
Previous studies [Citation17] had suggested that the hydrothermal resistance of Ce-TZP/Al2O3 was better than that of Y-TZP; nevertheless, the microstructure characterization on the degradation layer of Ce-TZP/Al2O3 was still lacking. The following tests were conducted to evaluate the degradation resistance of Ce-TZP/Al2O3; special attention was given to the microstructure characterization of surface layer. Apart from the Ce-TZP/Al2O3 specimens, some reference Y-TZP specimens were also prepared for comparison purpose.
2.1. Ce-TZP/Al2O3 specimen
The starting powder consisted of 70 vol% ceria-stabilized zirconia (Ce-TZP) and 30 vol% alumina (Al2O3) (Daiichi Kigenso Kagaku Kogyo Co., Japan); the CeO2 content in Ce-TZP was 10 mol%. The powder was granulated by the manufacturer to facilitate the use of a die-pressing technique. Pre-firing was carried out at 1000°C for 1 h to remove the organic binder. The green compact was subsequently sintered at 1550°C for 2 h. The sintered discs were 19.1 mm in diameter and 1.8 mm in thickness. The density of sintered specimens was measured by a water displacement method. The average density value was obtained by measuring 10 specimen discs. The relative density of the specimen was calculated assuming the theoretical density of 5.56 g/cm3 [Citation24].
The cross-sections of the specimen were prepared by grinding and polishing, the thermal etching by heating at 1450°C for 0.5 h. The mean grain size was determined by lineal intercept method; at least 200 grains were counted. The grain size was then estimated by multiplying the average intercept length with a factor of 1.56 [Citation25]. The microstructure was examined by scanning electron microscopy (SEM, Nova NanoSEM 450, FEI Co., USA). Since the Ce-TZP/Al2O3 specimen was electrical insulating, a thin Pt layer was coated onto the surface before SEM observation. The Pt coating could not penetrate into the pores or microcracks under surface, the electron charging could be detected if pores and/or microcracks were present in the surface layer. The charging layer was frequently taken as the layer of phase transformation [Citation9].
The hydrothermal treatment was carried out in a Teflon vessel. The specimen discs were cleaned with acetone and alcohol, then immersed in deionized water. The autoclave was then placed in an oven at a temperature of 134°C for up to 360 h. The phase composition after hydrothermal treatment was analyzed by X-ray diffraction (XRD, TTR III, Rigaku Co., Japan) technique. The scanning was conducted in the 2θ range from 25° to 32° at a slow scanning rate of 0.24 °/min. Within this 2θ range, typical XRD peaks for t-ZrO2, m-ZrO2 and Al2O3 phase were all present; the amount of monoclinic (m) phase could therefore be estimated using the relation of Garvie and Nicholson [Citation26]. The detection depth of the XRD technique was around 5 μm.
The biaxial strength of the specimen discs was measured using a ring-on-ring jig. The diameter of the loading and supporting rings was 7 mm and 14 mm, respectively. The loading rate was 0.48 mm/min. There were 15 to 18 discs measured for each hydrothermal treatment condition. The scatter of strength values was expressed in terms of Weibull modulus [Citation17]. Apart from the SEM technique, the microstructure was also examined using transmission electron microscopy (TEM, Tecnai G2 F20, FEI Co., USA). For the TEM observation, a thin section was cut from surface region with a focused ion beam system (FIB, NanoLab 600i, FEI Co., USA).
In order to evaluate the stress-strain behavior of Ce-TZP/Al2O3 specimen, a 4-point bending at a loading rate of 10 N/s was employed. The rectangular testing bars with the dimensions of 3 × 4x30 mm were prepared for the bending test. The preparation procedures of the testing bars can be found in another report [Citation27]. Compared to the specimen disc used for biaxial bending test, the volume under tensile stress in the rectangular bar was relatively larger. The stress (σ) and strain (ε) during 4-point bending were estimated using the following equations [Citation28]:
where P was the applied load; b the width (4 mm), d the thickness (3 mm) and dde the displacement of the loading pin. The upper span (l) was 10 mm and the lower span (L) was 30 mm.
2.2. Y-TZP specimen
Yttria-stabilized zirconia (Y-TZP, Tosoh Co., Japan) reference discs were also prepared using the same die-pressing techniques. The Y2O3 content in the Y-TZP was 3 mol%. There was a small amount of Al2O3 (0.25 wt%) in the powder as reported by the manufacturer. The sintering temperature was 1450°C, the dwell time was 1 h. The techniques used for phase analysis, microstructure characterization and strength measurement were identical to those used for the Ce-TZP/Al2O3 specimen discs.
3. Results and discussion
3.1. Ce-TZP/Al2O3 specimens
The sintering temperature for the Ce-TZP/Al2O3 specimen used in this study was 1550°C. Our preliminary studies indicated a sintering temperature of 1450°C was sufficient for preparing dense Ce-TZP/Al2O3 specimens with a relative density > 95%. A higher sintering temperature of 1550°C resulted in a higher density (>99%) and larger grain size (>1 μm). A specimen with higher density contains no open pores and a larger grain size induces a higher degree of phase transformation in Ce-TZP [Citation29,Citation30]. These two microstructural characteristics facilitate the investigation of the hydrothermal degradation behavior of the Ce-TZP/Al2O3 specimen within a reasonable time frame.
A representative SEM micrograph of a sintered Ce-TZP/Al2O3 specimen is shown in . The relative density of the sintered specimen discs was higher than 99%, as shown in . The bright particles in the SEM micrograph are ZrO2 grains, with a mean size of 1.1 μm and the gray particles are Al2O3 grains, with a mean size of 0.8 μm.
Table 1. Microstructural characteristics of Ce-TZP/Al2O3 investigated in this study. Y-TZP specimens were also prepared for comparison.
The microstructure of the sintered Ce-TZP/Al2O3 specimens (before hydrothermal treatment) was also examined through TEM. A thin section collected using the FIB technique is shown in . This section was taken from the surface to a depth of 5 μm into the specimen. shows a typical micrograph in the surface region, where the bright particles represent Al2O3 grains, and the gray ones represent ZrO2 grains. Although most Al2O3 grains were located between ZrO2 grains (in inter-granular positions), one small Al2O3 grain with a size of approximately 0.1 μm was observed within a ZrO2 grain (in intra-granular position). The electron diffraction pattern of one ZrO2 grain near the surface is shown in and indicates that the grain is in the t-phase. Neither twin structures nor microcracks were observed in the surface region, .
Figure 2. (a) Thin section taken from the surface region of Ce-TZP/Al2O3 specimen before hydrothermal treatment. (b) TEM micrograph of the area indicated with a square in (a). (c) Diffraction pattern of the ZrO2 grain near surface (circled).

The XRD pattern of the sintered Ce-TZP/Al2O3 specimen is shown in . A very small amount, ~2%, of m-phase was detected in the as-sintered specimen. The XRD patterns of the Ce-TZP/Al2O3 specimen for various durations of hydrothermal treatment are also shown in the figure. The height of the ZrO2 [−111]m peak increased with the duration of the hydrothermal treatment. The amount of m-phase reached 11% and 28% after respectively 120 h and 360 h of hydrothermal treatment. A diffraction line corresponding to Al2O3 [012] was also detected within the 2θ range from 25 to 32. No shift of the ZrO2 [101]t peak was observed, suggesting that the t-phase of ZrO2 remained stable during the hydrothermal treatment. The extent of phase transformation is shown as a function of hydrothermal treatment time in .
Figure 3. XRD diffraction patterns of (a) Ce-TZP/Al2O3 and (b) Y-TZP specimens before and after hydrothermal treatments for various times.

The fracture surface of the Ce-TZP/Al2O3 specimen after hydrothermal treatment for 360 h is shown in . The fracture surface was first ground to obtain a flat surface. Though a surface layer of 6–10 μm with low density was observed, the surface charge layer was very small ~1 μm. The TEM analysis provided a much clearer image of the transformation layer [Citation29]. shows a thin section collected from the surface of the Ce-TZP/Al2O3 specimen to a depth of 12 μm after hydrothermal treatment for 360 h. Many twins were observed within the ZrO2 grains (), with one relatively large ZrO2 grain shown in . Some twins originated from the grain boundary and aligned with each other. The twins in other ZrO2 grains were small and randomly oriented (). The formation of twins was taken as evidence of phase transformation from t to m [Citation30]. The selected area electron diffraction pattern of the ZrO2 grain is shown in , indicating the presence of the m phase. The thickness of the transformation layer, as estimated from TEM micrographs (), was greater than 12 μm. Barely any microcracks were observed in this transformation layer.
Figure 5. Fracture surfaces of (a) Ce-TZP/Al2O3 specimen after hydrothermal treatment for 360 h, and (b) Y-TZP specimens after hydrothermal treatment for 24 h.

Figure 6. (a) Thin section taken from the surface region of Ce-TZP/Al2O3 specimen after hydrothermal treatment for 360 h. (b) TEM micrograph in the surface region as indicated with a square in (a). (c) TEM micrograph at higher magnification of the ZrO2 grain indicated with a square in (b). (d) Diffraction pattern of the ZrO2 grain shown in (c).

The bending strength of the Ce-TZP/Al2O3 specimen measured through biaxial loading is shown in . The data were linearly fitted to determine the Weibull modulus, with R2 values higher than 0.9. The Weibull modulus of the specimen before hydrothermal treatment was 7.2. After treatment in water for 120 h, the Weibull modulus remained almost constant at 8.8. The average biaxial strength value of the Ce-TZP/Al2O3 specimen is shown in . After hydrothermal treatment for 120 h, the strength decreased slightly by 4.3%, but the Weibull modulus was not affected. To assess the effect of the surface transformation layer on the stress – strain behavior of the Ce-TZP/Al2O3 specimen, a loading – unloading-reloading technique was applied using rectangular testing bars (). For the rectangular specimens before and after hydrothermal treatment for 120 h, a very small strain was observed when the stress reached 400 MPa during the first loading cycle. After unloading and reloading with a gradually increasing load, the non-recoverable strain increased slightly. This indicates that the Ce-TZP/Al2O3 specimen exhibits a pseudo-plastic behavior both before and after 120 h of hydrothermal treatment.
Figure 7. Weibull plots of (a) Ce-TZP/Al2O3 and (b) Y-TZP specimens before and after hydrothermal treatment.

Figure 9. Loading-unloading-reloading curves of Ce-TZP/Al2O3 specimen (a) before and (b) after hydrothermal treatment. The time for hydrothermal treatment of the specimen in (b) was 120 h.

With an increase in the duration of hydrothermal treatment to 360 h, the strength of some specimens (located in the lower left corner of was relatively low, resulting in a lower Weibull modulus (5.0).
The m-phase content increased slightly from 2% to 3% after hydrothermal treatment for 24 h, to 11% after 120 h, and to 28% after 360 h (). The thickness of the transformation layer could not be estimated using SEM because of the lack of an electron charging layer. However, the TEM observation revealed the presence of twins as a result of the t-to-m phase transformation. The transformation layer was thicker than 12 μm after 360 h of hydrothermal treatment. More importantly, no microcracks were found, even after an exhaustive search of this layer.
As illustrated in , estimating the thickness of the transformation layer in the Ce-TZP/Al2O3 specimens through SEM was challenging because of the absence of microcracks. The transformation layer was defined through TEM analysis based on the presence of twins. However, the FIB technique used in this study could only reach a depth of 12 μm, thus hindering the estimation of the thickness of the entire transformation layer, which was considerably thick.
The resulting strength decrease in the Ce-TZP/Al2O3 specimen and the change in Weibull modulus were minimal after hydrothermal treatment for 120 h (). The stress – strain curve of the Ce-TZP/Al2O3 specimen was similar to that of the as-sintered specimen (), indicating that no additional cracks were introduced into the Ce-TZP/Al2O3 specimen during hydrothermal treatment.
The Weibull modulus of the Ce-TZP/Al2O3 specimen treated for 360 h was lower (). Although the value of the Weibull modulus depends strongly on the number of specimens tested [Citation31], a decrease from 7.2 to 5.0 for a sample size of 15 to 18 specimens is significant. This implies that additional flaws are introduced into the surface layer after long-term exposure to a hydrothermal environment.
3.2. Y-TZP specimen
Yttria-stabilized zirconia (Y-TZP) discs were prepared as reference specimens using the same die-pressing, pre-sintering, and sintering techniques used above. The microstructure of the specimen is shown in . The sintered density was higher than 98% and the mean size of ZrO2 grains was relatively small (0.6 μm, ). Only the t-phase was detected in the sintered specimens (). After hydrothermal treatment for 5 h at 134°C, the content of the m-phase increased from 0% to 8%. Although the content of the m-phase was low, the biaxial strength decreased by 22% ().
The content of the m-phase increased substantially after hydrothermal treatment (). Furthermore, the intensity of ZrO2 [101]t diffraction peak @ 2θ of ~ 30 degree in degraded Y-TZP was decreased and this peak was no longer symmetrical (). The m-phase content quickly increased to a saturated value of 75% after 72 h in a hydrothermal environment (). A charging surface layer resulting from the presence of pores and/or microcracks was observed in the SEM micrograph (). From the thickness of the surface charging layer, the thickness of the transformation layer after 24 h of hydrothermal treatment was approximately 5 μm. shows the Weibull distribution for the Y-TZP specimens after 0 h, 5 h, and 24 h of hydrothermal treatment. The biaxial strength decreased with the increase in the content of the m-phase, although the Weibull modulus remained almost constant (4.1, 3.8, and 4.8, respectively). The decrease in strength indicated its dependence on the increase in m-phase content ().
The surface layer of the specimen after 24 h of hydrothermal treatment was observed using TEM. A thin section was collected from the surface region of the Y-TZP specimen through the FIB technique (). Twins were observed within the ZrO2 grains, and microcracks were observed at grain boundaries (), with most of them being parallel to the surface.
Figure 10. (a) Thin section taken from the surface region of Y-TZP specimen after hydrothermal treatment for 24 h. (b) TEM micrograph in the surface region as indicated with a square in (a).

The LTD of Y-TZP has been extensively investigated in recent decades [Citation6,Citation19–22,Citation32,Citation33]. According to the standard for dental implants, ISO 13,356, “Implants for surgery – Ceramic materials based on yttria-stabilized tetragonal zirconia (Y-TZP)”, the m-phase content should be lower than 25% after 5 h of hydrothermal treatment at 134°C. The reported 5-year survival rates for single crowns made of Y-TZP based on the standard clinical practice were higher than 91.2% [Citation34]. The Mehl – Avhrami – Johnson (MAJ) equation is used to estimate the lifetime of zirconia implants from in vitro data [Citation6,Citation32]. Although such a prediction is of great importance, Lughi et al. suggested that the lifetime prediction is accurate only if the activation energy of transformation is considered constant [Citation5]. Pezzotti et al. indicated that the m-phase contents in biomedical implants retrieved from patients were much higher than in vitro values [Citation33]. As confirmed by XRD analysis (), the change in the t-phase may contribute to discrepancies between in vitro and in vivo data. The XRD patterns, , demonstrated that the ZrO2 [101]t reflection was broader and shifted to its left-hand side with the increase in hydrothermal treatment time. Moreover, a left shoulder was observed in the t-ZrO2 reflection. Previous studies suggested that one XRD peak of orthorhombic or rhombohedral was also close to 2θ of ~ 30 degree [Citation35,Citation36]. The XRD pattern thus suggests that the tetragonal phase not only transformed to monoclinic phase, it may also transformed to other phase. Consequently, the activation energy for the t-to-m phase transformation is likely changed. When the hydrothermal treatment time was increased to 24 h, the m-phase content increased to 60% () and the biaxial strength decreased by 40% (), indicating marked degradation of the Y-TZP specimen in the hydrothermal environment.
The decrease in strength of the Y-TZP specimen during the hydrothermal environment can be attributed to the formation of microcracks [Citation8,Citation37]. Microcracks were observed in the transformation surface layer (), and they tended to parallel to the surface. Many twins were also observed within the ZrO2 grains and aligned with each other. These microstructural characteristics of Y-TZP were also reported by Munoz-Tabares et al. [Citation37,Citation38]. This demonstrates that the Y-TZP specimens prepared in this study could be regarded as reference specimens.
3.3. Ce-TZP/Al2O3 vs. Y-TZP
A summary for the characteristics of Ce-TZP/Al2O3 and Y-ZTP after degradation is shown in . A longer hydrothermal treatment time was used for Ce-TZP/Al2O3 specimen; it would produce a thicker degradation surface layer to facilitate the subsequent microstructure observation. The Ce-TZP/Al2O3 exhibits a better resistance to hydrothermal degradation in terms of time in water. The present study also suggests that the microstructure characterization on the transformation layer of TZP is challenging. When microcracks are formed in the surface layer of Y-TZP specimen, an electron charging layer can be observed. This technique was frequently applied to evaluate the aging resistance of Y-TZP [Citation9]. However, the technique was not appliable to Ce-TZP/Al2O3, the system with little microcracks in the surface region. A TEM technique is therefore needed to characterize the transformation layer. The formation of microcracks is rare in Ce-TZP/Al2O3 (), this finding is also supported by a lower strength decrease.
Table 2. Hydrothermal resistance of the Ce-TZP/Al2O3 and Y-TZP prepared in this study; corresponding data can be found in the figures indicated in brackets.
shows the biaxial strength of Ce-TZP/Al2O3 and Y-TZP specimens as a function of the m-phase content. The strength of the Ce-TZP/Al2O3 specimen was higher than that of the Y-TZP specimen for the same m-phase content. However, m-phase content is not the only factor affecting the strength [Citation18] and the formation of microcracks plays an important role in hydrothermal resistance.
Two types of transformations, homogeneous and autocatalytic, have been reported for TZPs [Citation39,Citation40]. The phase transformation in Y-TZP is typically homogeneous, such that the transformation often starts at one grain boundary and ends at another boundary within the same grain. Because volume expansion is associated with the phase transformation, stresses are induced by the transformation. These stresses are released partially through the formation of twins and partially through the generation of microcracks [Citation29]. Both twins and microcracks were observed in the degraded Y-TZP specimens ().
The phase transformation in Ce-TZP/Al2O3 is typically autocatalytic [Citation41,Citation42]. However, a direct observation of its transformation surface layer has not yet been made. TEM analysis conducted in this study provided insights into the transformation layer. The TEM micrograph shown in indicates that many small twins are formed within the ZrO2 grain. In addition, most of these twins were not aligned with each other.
Similar to elongated alumina grains with an anisotropic thermal expansion coefficient, the formation of microcracks in the twins likely requires a critical size [Citation43]. Because many small twins were randomly oriented and not aligned with each other (), the stresses associated with the randomly oriented twins did not accumulate in the same direction, preventing the formation of microcracks. The decrease in strength for the Ce-TZP/Al2O3 specimens treated in water was minimal because no microcracks were formed.
4. Conclusions
In this study, the hydrothermal resistance of Ce-TZP/Al2O3 specimens was evaluated, with Y-TZP specimens used as references. Although t-to-m phase transformation was observed in both TZP systems, the degrees of strength decrease in a hydrothermal environment varied. This discrepancy can be attributed to the presence of microcracks in the transformation surface layer. The findings of this study highlight the importance of microstructure characteristics in determining LTD resistance. If no microcrack is formed during the t-to-m phase transformation, the influence on strength is minimal. However, a TEM technique is needed to characterize the transformation surface layer with a limited number of microcracks.
Acknowledgments
This study was supported by National Science and Technology Council (NSTC 112-2923-E-002-005, MOST 111-2221-E-002-158), and Chang Gung Memorial Hospital at Linkou (CMRPG3L0751, CMRPG3L0752). The technique help on SEM from Ms. Yu-Wen Chen is appreciated.
Disclosure statement
No potential conflict of interest was reported by the author(s).
Additional information
Funding
References
- Lance MJ, Vogel EM, Reith LA, et al. Low-temperature aging of zirconia ferrules for optical connectors. J Am Ceram Soc. 2001;84(11):2731–2733. doi: 10.1111/j.1151-2916.2001.tb01085.x
- Piconi C, Maccauro G. Zirconia as a ceramic biomaterial. Biomaterial. 1999;20:1–25. doi: 10.1016/S0142-9612(98)00010-6
- Drouin J, Cales B. Yttria-stabilized zirconia ceramic for improved hip joint head. In: Andersson OH, Happonen R-P Urpo A, editors Bioceramics. Elsevier Ltd; 1994. pp. 389–394.
- Tateishi T, Yunoki H. Research and development of alumina and zirconia artificial hip joint. Clin Mater. 1993;12(4):219–225. doi: 10.1016/0267-6605(93)90076-J
- Lughi V, Sergo V. Low temperature degradation-aging-of zirconia: a critical review of the relevant aspects in dentistry. Dent Mater. 2010;26(8):807–820. doi: 10.1016/j.dental.2010.04.006
- Chevalier J, Gremillard L, Deville S. Low-temperature degradation of zirconia and implications for biomedical implants. Ann Rev Mater Res. 2007;37(1):1–32. doi: 10.1146/annurev.matsci.37.052506.084250
- Muddle B, Hannink RH. Crystallography of the tetragonal to monoclinic transformation in partially stabilized zirconia. J Am Ceram Soc. 1986;69:547–555.
- Deville S, Guénin G, Chevalier J. Martensitic transformation in zirconia: Part I. Nanometer scale prediction and measurement of transformation induced relief. Acta Mater. 2004;52(19):5697–5707. doi: 10.1016/S1359-6454(04)00525-7
- Kobayashi K, Kuwajima H, Masaki T. Phase change and mechanical properties of ZrO2-Y2O3 solid electrolyte after ageing. Solid State Ion. 1981;3:489–493. doi: 10.1016/0167-2738(81)90138-7
- Schneider J, Begand S, Kriegel R, et al. Low‐temperature aging behavior of alumina‐toughened zirconia. J Am Ceram Soc. 2008;91(11):3613–3618. doi: 10.1111/j.1551-2916.2008.02700.x
- Matsui K, Nakamura K, Kumamoto A, et al. Low-temperature degradation in yttria-stabilized tetragonal zirconia polycrystal doped with small amounts of alumina: effect of grain-boundary energy. J Eur Ceram Soc. 2016;36(1):155–162. doi: 10.1016/j.jeurceramsoc.2015.09.016
- Matsui K, Hojo J. Initial sintering mechanism and additive effect in zirconia ceramics. J Am Ceram Soc. 2022;105:5519–5542.
- Matsumoto RL. Aging behavior of ceria stabilized tetragonal zirconia polycrystals. J Am Ceram Soc. 1988;71(3):C128–C129. doi: 10.1111/j.1151-2916.1988.tb05031.x
- Tsukuma K, Shimada M. Strength, fracture toughness and vickers hardness of CeO2-stabilized tetragonal ZrO2 polycrystals (ce-TZP). J Mater Sci. 1985;20:1178–1184.
- Wu S-X, Brook RJ. Kinetics of densification in stabilized zirconia. Solid State Ion. 1984;14(2):123–130. doi: 10.1016/0167-2738(84)90086-9
- Tanaka K, Tamura J, Kawanabe K, et al. Phase stability after aging and its influence on pin-on-disk wear properties of Ce-TZP/Al2O3 nanocomposite and conventional Y-TZP. J Biomed Mater Res. 2003;67A(1):200–207. doi: 10.1002/jbm.a.10006
- Ban S, Sato H, Suehiro Y, et al. Biaxial flexure strength and low temperature degradation of Ce-TZP/Al2O3 nanocomposite and Y-TZP as dental restoratives. J Med Mater Res, Part B Appl Biomater. 2008;87B(2):492–498. doi: 10.1002/jbm.b.31131
- Sim I-G, Shin Y, Shim JS, et al. Effects of artificial aging on the biaxial flexural strength of Ce-TZP/Al2O3 and Y-TZP after various occlusal adjustments. Ceram Int. 2017;43(13):9951–9959. doi: 10.1016/j.ceramint.2017.05.005
- Guo X. Hydrothermal degradation mechanism of tetragonal zirconia. J Mater Sci. 2001;36(15):3737–3744. doi: 10.1023/A:1017925800904
- Lange F, Dunlop D, Davis BI. Degradation during aging of transformation-toughened ZrO2-YO3 materials at 250°C. Journal Of The American Ceramic Society. 1986;69(3):237–240. doi: 10.1111/j.1151-2916.1986.tb07415.x
- Lawson S. Environmental degradation of zirconia ceramics. J Eur Ceram Soc. 1995;15(6):485–502. doi: 10.1016/0955-2219(95)00035-S
- Pandoleon P, Kontonasaki E, Kantiranis N, et al. Aging of 3Y-TZP dental zirconia and yttrium depletion. Dent Mater. 2017;33(11):e385–e392. doi: 10.1016/j.dental.2017.07.011
- Masonis JL, Bourne RB, Ries MD, et al. Zirconia femoral head fractures: a clinical and retrieval analysis. J Arthroplasty. 2004;19(7):898–905. doi: 10.1016/j.arth.2004.02.045
- Palmero P, Fornabaio M, Montanaro L, et al. Towards long lasting zirconia-based composites for dental implants. Part I: innovative synthesis, microstructural characterization and in vitro stability. Biomaterial. 2015;50:38–46. doi: 10.1016/j.biomaterials.2015.01.018
- Mendelson MJ. Average grain size in polycrystalline ceramics. J Am Ceram Soc. 1969;52(8):443–446. doi: 10.1111/j.1151-2916.1969.tb11975.x
- Garvie RC, Nicholson PS. Phase analysis in zirconia systems. J Am Ceram Soc. 1972;55(6):303–305. doi: 10.1111/j.1151-2916.1972.tb11290.x
- Cheng YS, Tuan WH, Chen JR, et al. Phase transformation induced fatigue of Ce-TZP/Al2O3 composite. J Am Ceram Soc. 2023;106:7670–7679. doi: 10.1111/jace.19396
- Pronk A. Theory of the four-point dynamic bending test, part I: general theory. Report-DWW-96-008. 1996.
- Rauchs G, Fett T, Munz D, et al. Tetragonal-to-monoclinic phase transformation in CeO2-stabilized zirconia under multiaxial loading. J Eur Ceram Soc. 2002;22:841–849.
- Touaiher I, Saâdaoui M, Chevalier J, et al. Fracture behavior of Ce-TZP/alumina/aluminate composites with different amounts of transformation toughening. Influence of the testing methods. J Eur Ceram Soc. 2018;38(4):1778–1789. doi: 10.1016/j.jeurceramsoc.2017.09.052
- Ritter JE. Statistical reproducibility of the dynamic and static fatigue experiments. Am Ceram Soc Bull. 1981;60:798–806.
- Gaillard Y, Jiménez-Piqué E, Soldera F, et al. Quantification of hydrothermal degradation in zirconia by nanoindentation. Acta Mater. 2008;56(16):4206–4216. doi: 10.1016/j.actamat.2008.04.050
- Nowicka A, El-Maghraby H, Švančárková A, et al. Corrosion and Low Temperature Degradation of 3Y-TZP dental ceramics under acidic conditions. J Eur Ceram Soc. 2020;40(15):6114–6122. doi: 10.1016/j.jeurceramsoc.2020.06.019
- Hunziker S, Thorpe L, Zitzmann NU, et al. Evaluation of diamond rotary instruments marketed for removing zirconia restorations. J Prosthet Dent. in press.
- Chevalier J, Cales B, Drouin JM. Low-temperature aging of Y-TZP ceramics. J Am Ceram Soc. 1999;82(8):2150–2154. doi: 10.1111/j.1151-2916.1999.tb02055.x
- Pezzotti G, Affatato S, Rondinella A, et al. In vitro versus in vivo phase instability of zirconia-toughened alumina femoral heads: a critical comparative assessment. Mater. 2017;10(5):466. doi: 10.3390/ma10050466
- Muñoz-Tabares J, Jiménez-Piqué E, Anglada M. Subsurface evaluation of hydrothermal degradation of zirconia. Acta Mater. 2011;59:473–484.
- Ruhle M, Evans AG. High toughness ceramics and ceramic-matrix composites. Prog Mater Sci. 1989;33(2):85–167. doi: 10.1016/0079-6425(89)90005-4
- Ruiz L, Ready MJ. Effect of heat treatment on grain size, phase assemblage, and mechanical properties of 3 mol% Y-TZP. J Am Ceram Soc. 1996;79(9):2331–2340. doi: 10.1111/j.1151-2916.1996.tb08980.x
- Kitano Y, Mori Y, Ishitani A, et al. Rhombohedral phase in Y2O3-partially-stabilized ZrO2. J Am Ceram Soc. 1988;71:c34–c36. doi: 10.1111/j.1151-2916.1988.tb05776.x
- Liens A, Swain M, Reveron H, et al. Development of transformation bands in ceria-stabilized-zirconia based composites during bending at room temperature. J Eur Ceram Soc. 2021;41:691–705. doi: 10.1016/j.jeurceramsoc.2020.08.062
- Liens A, Reveron H, Douillard T, et al. Phase transformation induces plasticity with negligible damage in ceria-stabilized zirconia-based ceramics. Acta Mater. 2020;183:261–273.
- Vekinis G, Ashby MF, Beaumont PWR. R-curve behaviour of Al2O3 ceramics. Acta Metallurgica et Materialia. 1990;38(6):1151–1162. doi: 10.1016/0956-7151(90)90188-M