ABSTRACT
In order to prepare for the twindemic of influenza and SARS-CoV-2 infection, we investigated the association between influenza infection and subsequent severity of SARS-CoV-2 infection. A population-based nationwide cohort study was performed using data from the National Health Insurance Service (NHIS) in the Republic of Korea. This study included 274,126 individuals who underwent SARS-CoV-2 PCR testing between 20 January 2020 and 1 October 2020. Among these patients, 28,338 tested positive for SARS-CoV-2, and 4,003 of these individuals had a history of influenza. The control group was selected through 1:1 propensity score matching. In the group of 4,003 COVID-19-positive individuals with no history of influenza, 192 (4.8%) experienced severe illness from COVID-19 infection. In the group of 4,003 COVID-19-positive individuals with a history of influenza, 260 (6.5%) had severe illness from COVID-19, and the overall adjusted odds ratio (aOR) was 1.29 (95% confidence interval 1.04–1.59). Among the 4,003 COVID-19-positive individuals with a history of influenza, severe COVID-19 infection was experienced by 143 of 1,760 (8.1%) with an influenza history within 1 year before the onset of COVID-19, 48 of 1,129 (4.3%) between 1 and 2 years, and 69 of 1,114 (6.2%) between 2 and 3 years before COVID-19 onset, and the aORs were 1.54 (1.20-1.98), 1.19 (0.84-1.70), and 1.00 (0.73-1.37), respectively. In conclusion, individuals who had an influenza infection less than 1 year before COVID-19 infection were at an increased risk of experiencing severe illness from the SARS-CoV-2 infection. To control the public health burden, it is essential that effective public health control measures, which include influenza vaccination, hand washing, cough etiquette, and mask use are in place.
Introduction
Influenza virus infection has been a global public health priority for many years [Citation1]. It is still a major cause of morbidity and mortality in many countries, and is frequently associated with respiratory symptoms [Citation1,Citation2]. In December 2019, a novel coronavirus, known as severe acute respiratory syndrome coronavirus 2 (SARS-CoV-2), emerged as the causative pathogen of COVID-19. The clinical spectrum of SARS-CoV-2 infection ranges from asymptomatic infection to critical illness [Citation3]. A global pandemic was declared in March 2020 [Citation4]. On 1 January 2022, approximately 300 million confirmed cases and 5.4 million deaths had been reported worldwide [Citation5]. In particular, individuals who died because of COVID-19 experienced severe illness from this infection, and various risk factors for severe outcomes from COVID-19 have been reported [Citation3,Citation6,Citation7].
The COVID-19 pandemic has placed onerous demands on public health systems [Citation8]. Patients with severe COVID-19 infection should be admitted to hospital for careful monitoring because progressive respiratory failure develops in many patients with severe COVID-19 [Citation8,Citation9]. Severe COVID-19 may also lead to acute cardiac, renal, and hepatic injuries, cardiac arrhythmias, rhabdomyolysis, shock, thromboembolic complications as well as disorders of the central or peripheral nervous system [Citation9]. This can overwhelm local healthcare resources resulting in shortages of trained staff, ventilators, renal-replacement treatment, and intensive care unit beds [Citation8,Citation9]. Therefore, it is necessary to evaluate and control the risk factors for the progression of COVID-19 to severe illness and prevent that progression.
Recent studies have suggested the possibility of influenza as a risk factor for severe illness from COVID-19 [Citation10–15]. Sequential infection or co-infection with influenza virus and SARS-CoV-2 virus was reported to be associated with a severe course of SARS-CoV-2 [Citation10–13]. Influenza vaccination was reported to lower susceptibility to SARS-CoV-2 infection and prevent progression to severe COVID-19 [Citation14,Citation15], making it possible to infer that influenza infection may be associated with severe COVID-19.
Very few studies have investigated the association between influenza infection and the severity of COVID-19 in the general population [Citation16]. We hypothesized that influenza virus infection within 1 year of COVID-19 onset would be a risk factor for the development of severe COVID-19. The purpose of this population-based nationwide cohort study was to investigate whether influenza infection less than 1 year before COVID-19 onset was a risk factor that may contribute to the severity of COVID-19.
Methods
Patient and public involvement, data sources
This large-scale retrospective cohort study was conducted using the Korean National Health Insurance claim-based database. The National Health Insurance Service (NHIS) is a single insurer managed by the government and contains data from approximately 97% of the Korean population [Citation17]. The Korean government covers the costs of diagnosis and treatment for individuals who meet the relevant criteria associated with COVID-19, encouraging the public to be tested for COVID-19 without delay. During the COVID-19 pandemic, the Korean government offered health insurance services to all Koreans with COVID-19. The NHIS provides a set of health information that includes the following: an eligibility database including data such as age, sex, socioeconomic status, level of income, and type of eligibility; a medical care institution database including data such as medical installation and institution; a medical treatment database with accounts submitted by medical practitioners for medical service expenditure; a health examination database with the results of general health examinations and questionnaires on lifestyle. The medical data for all patients who underwent SARS-CoV-2 testing were provided by the NHIS. In this dataset, we selected hospital records for COVID-19 patients, including death records, hospital visits, prescriptions, diagnostic records, and treatment records for the 3 years from 2017 to 2019. All patient records were anonymized before use.
Study population
We enrolled all patients who underwent SARS-CoV-2 testing between 20 January 2020 and 1 October 2020. An additional 3-month follow-up period was applied after October 1, 2020 to confirm whether severe illness from COVID-19 would develop in patients with confirmed COVID-19 within this period. Laboratory diagnosis of SARS-CoV-2 infection was defined as a positive result from real-time RT–PCR assay of nasal or pharyngeal swabs from patients with a history SARS-CoV-2 (U071) as defined using the International Classification of Disease, Tenth Revision (ICD-10). For each patient who underwent the SARS-CoV-2 test, the cohort entry date was the date of the first SARS-CoV-2 test. We combined claims data from the NHIS (NHIS -2022-1-382) and extracted age, sex, region of residence, and economic status from the insurance eligibility data. Using codes from the International Classification of Disease, Tenth Revision (ICD-10), patient data also included information on history of angina (I20), cancer (any C code), congestive heart failure (CHF, I43 and I50), chronic kidney disease (CKD, N18 and N19), myocardial infarction disease (MI, I21 and I22), diabetes mellitus (DM, E10–14), hypertension (HTN, I10–13, and I15), chronic lung disease (asthma or chronic obstructive pulmonary disease (COPD), J43–46), and chronic liver disease (K74, K703, and B18) where patients received at least two diagnoses within 1 year during the previous 3 years.
Definition
Based on the NHIS data, a history of influenza was defined as a person whose medical history included the diagnostic codes for influenza (J09–J11) during the 3-year period before the diagnosis of SARS-CoV-2. When individuals had a history of two or more influenza infections, the latest infection was included in the analysis. From the NHIS data, severe illness from COVID-19 infection was defined as an end result with one of the following conditions: 1) admission to an intensive care unit (ICU); 2) extracorporeal membrane oxygenation (ECMO) treatment; 3) use of a mechanical ventilator; or 4) death. Data on mortality (death) from COVID-19 were obtained from the NHIS data which included mortality data from the Korea Disease Control and Prevention Agency and Statistics Korea. Complicated influenza was defined as the diagnosis of influenza with any of the following: hospitalization, oxygen supply, dyspnoea, pneumonia, or acute exacerbation of the respiratory system.
Statistical analysis
Descriptive statistics included frequency analysis (percentages) for categorical variables and mean ± standard deviation or median and range for continuous variables. Categorical variables were compared using the Chi-squared test unless >20% of cells had a count <5, in which case Phi and Cramer’s V test was used. Categorical and continuous variables were compared using standard mean difference (SMD).
To examine the association between severe illness from COVID-19 infection and history of influenza, we analyzed the data for the COVID-19 patients categorized into two groups. The first group had a history of influenza infection and the second had no history of influenza infection, with both groups having a positive SARS-CoV-2 PCR result during the COVID-19 pandemic. After performing PS matching with a 1:1 ratio between the influenza-positive group and the influenza-negative group, the influenza-positive group was classified into three subgroups based on history of influenza infection within 1 year, 1–2 years, and 2–3 years before the onset of the COVID pandemic (). In addition, the influenza-positive group was divided into complicated and uncomplicated influenza groups, which were also classified into three subgroups based on a history of influenza infection within 1 year, 1–2 years, and 2–3 years before COVID-19 infection. Odds ratios (OR) for severe illness due to COVID-19 associated with influenza infection were estimated by year and complication. Among the SARS-CoV-2-tested patients, validated propensity score (PS) matching was performed to eliminate baseline characteristics and potential confounders. Age, sex, economic status, cancer type, hypertension, chronic kidney disease, diabetes mellitus, myocardial infarction, angina, congestive heart failure, chronic lung disease, and chronic liver disease were considered for PS matching using a “greedy nearest neighbour” algorithm with a 1:1 ratio. Confirmation of whether PS matching was successful was judged by the presence or absence of major imbalances at the baseline and standardized mean differences (SMDs) in each group. SMD values that were <0.1 indicated low levels of covariate imbalances, which implies that the imbalance between covariates had been nullified [Citation18].
Figure 1. The influenza-positive group was classified into five subgroups according to history of influenza infection within 1 year, 1–2 years, 2–3 years before the onset of the COVID-19 pandemic.
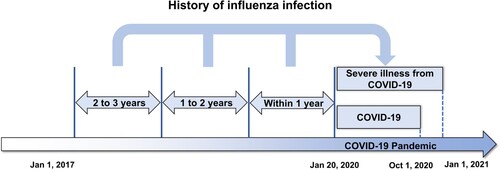
The ORs were calculated using a multivariate logistic regression model. Unadjusted OR is a method of analysis that considers only two variables (independent and dependent variables) without considering any others, whereas adjusted OR (aOR) is an analytical procedure that considers all other variables. The OR and 95% confidence interval (CI) were calculated. A two-sided P < 0.05 was considered statistically significant. SAS 9.4 (SAS Institute Inc., Cary, NC, USA) and R 4.0.3 (R Foundation for Statistical Computing, Vienna, Austria) were used to analyze the results.
Sensitivity analysis
Sensitivity analysis was performed according to 1) matching ratio, and 2) matching variables. The matching ratio (influenza group vs non-influenza group) was set to 1:3, 1:2, or 1:1. We evaluated the robustness of the odds ratio according to the matching variables (minimally: age + sex + demography, 3 underlying diseases: minimally + HTN + DM + CKD matching, 4 underlying diseases: minimally + HTN + DM + CKD + lung diseases matching, 5 underlying diseases: minimally + HTN + DM + CKD + lung diseases + liver diseases matching, fully matching: minimally + HTN + DM + CKD + lung diseases + liver diseases + cardiac diseases (CHF + angina + MI) + any type of cancer matching). An additional sensitivity analysis was performed to assess the robustness of the results to unmeasured confounding using the E-value methodology [Citation19]. This estimates what the relative risk would have to be for any unmeasured confounder to overcome the observed association of exposure to influenza with severe COVID-19 infection in our study.
Results
The final dataset included 274,126 patients tested for SARS-CoV-2, of whom 28,338 patients tested positive (, ). The baseline characteristics of the study cohort are presented in . Of the 28,338 patients with a positive SARS-CoV-2 test result, 4,003 had a history of influenza. For the remaining 24,335 patients with no history of influenza but with a positive SARS-CoV-2 test result, 4,003 were selected through 1:1 propensity score matching, and the two groups were compared (, ). After propensity score matching, there were no significant imbalances in demographics or clinical characteristics between the influenza-positive and influenza-negative groups (all SMDs < 0.1, ).
Figure 2. Distribution and flow chart of influenza and SARS-CoV-2 patients in a nationwide Korean cohort.
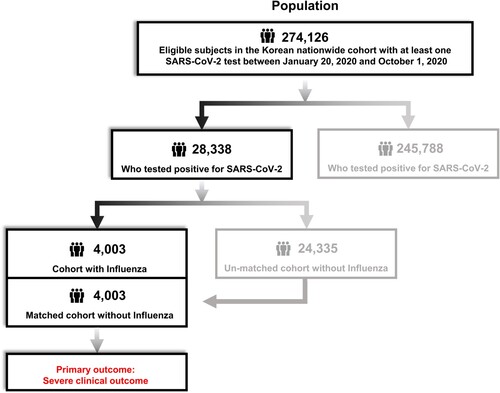
Table 1. Demographic characteristics of patients in the Korean nationwide cohort who underwent SARS-CoV-2 testing.
Table 2. Propensity score-matched baseline characteristics associated with severe illness from COVID-19 infection
There was a severe clinical outcome for COVID-19 infection in 260 patients (6.5%) with a history of influenza and 192 patients (4.8%) with no history of influenza (aOR 1.29, 95% confidence interval (CI) 1.04–1.59) (, ). In subgroup analysis, severe COVID-19 occurred in 143 cases (8.1%) with a history of influenza infection less than 1 year before COVID-19 infection ; no influenza vs influenza within 1 year: aOR 1.54 (95% CI 1.20–1.98). A history of influenza infection in other periods was not significant for severe illness due to COVID-19 (). The aOR of complicated influenza infection was 3.00 (95% CI, 2.27–3.97): aOR of complicated influenza within 1 year before COVID-19 onset was 3.45 (95% CI, 2.49–4.79); aOR within 1–2 years was 2.73 (95% CI, 1.45–5.14); aOR within 2–3 years before COVID19 onset was 2.06 (95% CI, 1.18–3.58) (). In addition to a history of influenza infection within 1 year, age (aOR 7.08, 95% CI 5.33–9.40), male sex (aOR 1.54, 95% CI 1.24–1.90), cancer (aOR 1.95, 95% CI 1.52-2.51), HTN (aOR 1.78, 95% CI 1.37–2.32), COPD (aOR 1.77, 95% CI 1.26-2.48), diabetes mellitus (aOR 1.65, 95% CI 1.29–2.12), congestive heart failure (aOR 1.58, 95% CI 1.18–2.12), and myocardial infarction (aOR 0.60, 95% CI 0.39–0.92) were independently associated with severe illness from COVID-19 infection ().
Figure 3. Propensity score-matched association between influenza and severe clinical outcomes of COVID-19 in patients who tested positive for SARS-CoV-2. Sensitivity analysis was performed according to 1) matching ratio, 2) matching variables (minimally: age + sex + demography, 3 underlying diseases: minimally + HTN + DM + CKD matching, 4 underlying diseases: minimally + HTN + DM + CKD + lung diseases matching, 5 underlying diseases: minimally + HTN + DM + CKD + lung diseases + liver diseases matching, fully matching: minimally + HTN + DM + CKD + lung diseases + liver diseases + cardiac diseases (CHF + angina + MI) matching). (Abbreviations: HTN, hypertension; DM, diabetes mellitus; CKD, chronic kidney disease; CHF, congestive heart failure; MI, myocardial infarction).
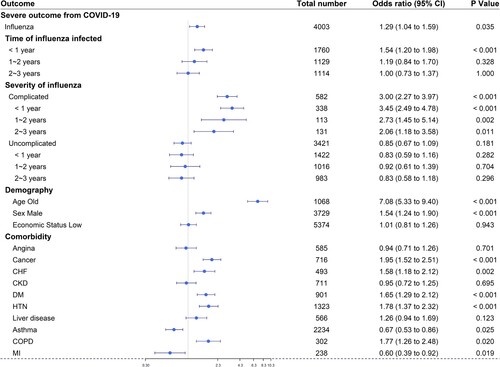
Table 3. Propensity score-matched adjusted ORs for the risk of severe illness from COVID-19 in patients with previous influenza infection
Sensitivity analysis showed the robustness of our results regarding influenza within 1 year before the onset of COVID-19, which did not change according to matching ratio or matching variables (). The E-values and lower CI limit for unmeasured confounders between influenza within 1 year and severe illness from COVID-19 were 2.45 and 1.69, respectively.
Figure 4. Sensitivity analysis was performed according to 1) matching ratio, 2) matching variables (minimally: age + sex + demography, 3 underlying diseases: minimally + HTN + DM + CKD matching, 4 underlying diseases: minimally + HTN + DM + CKD + lung diseases matching, 5 underlying diseases: minimally + HTN + DM + CKD + lung diseases + liver diseases matching, fully matching: minimally + HTN + DM + CKD + lung diseases + liver diseases + cardiac diseases (CHF + angina + MI) matching). (Abbreviations: HTN, hypertension; DM, diabetes mellitus; CKD, chronic kidney disease; CHF, congestive heart failure; MI, myocardial infarction)
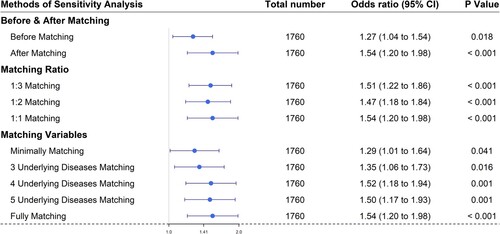
Discussion
Principal findings
The objective of this population-based nationwide cohort study was to investigate whether a history of influenza infection within 1 year before COVID-19 infection was a risk factor for severe illness from COVID-19. This is the first attempt to evaluate the impact of recent influenza viral infections on the severity of COVID-19 infection in the general population. Our study showed that influenza infection within the year before COVID-19 infection was a risk factor for severe COVID-19 illness. Our study suggests that recent influenza viral infection may contribute to the disease burden of COVID-19.
Findings in relation to other studies
Several studies have reported co-infection or sequential infection from SARS-CoV-2 and influenza during the COVID-19 pandemic. In animal studies using mice, ferrets, and hamsters, sequential SARS-CoV-2 infection after influenza A/H1N1 resulted in the aggravation of pneumonia and increased mortality compared with mono-infection, which demonstrated that host immunity-dependent COVID-19 symptoms could be aggravated by prior influenza A/H1N1 infection [Citation11,Citation12]. Simultaneous co-infection or sequential short-term interval co-infection with influenza before SARS-CoV-2 infection resulted in a lower SARS-CoV-2 viral load, and it was thought that there may be viral interference [Citation11,Citation12]; however, in sequential long-term interval co-infection with influenza before coronavirus infection, some coronavirus replication was detectable. Ultimately, simultaneous or sequential co-infection with influenza and SARS-CoV-2 leads to more severe disease [Citation11,Citation12,Citation20]. This could be explained by the fact that influenza virus increases the expression of ACE2 in small airway epithelial cells and alveolar type II cells, and SARS-CoV-2 infection in small airways and alveolar epithelium results in compromised respiratory states such as pneumonia or ARDS [Citation21].
ACE2 acts as a receptor for SARS-CoV-2 to enter host cells [Citation22]. Therefore, higher ACE2 expression plays a significant causal role in the susceptibility and severity of COVID-19 [Citation23]. Influenza virus increases the expression of ACE2 in nasal epithelium and lung tissue, such as small airway epithelial cells and alveolar type 2 cells, through the interferon (IFN) signalling response associated with type 1 IFN (IFN α and β) and type 2 IFN (IFN γ) [Citation21,Citation24–26]. In addition, influenza viral infection can progress beyond the acute phase inflammation of a self-limited course recovering in 3–7 days to the post-influenza chronic pulmonary inflammation state. The following research results provide evidence for this: cells that have survived for a long period after acute influenza viral infection showed a robust transcriptional response to viral infection, increased interferon stimulation and response, and increased levels of pro-inflammatory cytokines despite viral clearance [Citation27]; the acute phase of viral infection switched to a form of chronic lung disease (airway inflammation, mucus production, hyper-reactivity, fibrosis, and emphysema) associated with long-term persistence (105 days) of remnant active viral RNA in lung tissue [Citation28]; long-term IFN-γ/IL-10 producing T cells were observed for more than 95 days after influenza infection [Citation29]. Post-influenza chronic viral inflammation presents not only type 2 inflammation related to IL-13, which reduces the expression of ACE2 [Citation24,Citation28], but also type 1 inflammation associated with IL-10 and IFN-γ, which increases the expression of ACE2 [Citation29–32]. Other studies have reported that epithelial cells expressing interferon stimulating genes (ISG) survive infection in both influenza A and B infections [Citation27,Citation33,Citation34]. Therefore, post-influenza viral chronic inflammation is estimated to be similar to the type 1 inflammatory signature [Citation35]. The switch from acute influenza viral infection to post-viral chronic inflammation is dependent on the initial viral dose, so the more severe the acute infection, the more likely it was to progress to chronic lung disease [Citation28,Citation35]. In our data, the significant aOR value of 1.54 (95% CI: 1.20–1.98) for influenza infection within the 1 year before COVID-19 onset supports the above mechanism.
In comorbidities associated with severe SARS-CoV-2 infection, ACE2 levels increase in the lungs of patients with COPD and smokers [Citation36,Citation37]. Immune cell alterations, such as the increase in type 1 and type 2 IFN receptors, and the epithelial immune response, such as the increase in type 1 and type 2 IFN and ISG, were observed in patients with COPD [Citation38]. The IFN response associated with the ACE2 increase in COPD is similar to that observed in influenza viral infections [Citation26]. Therefore, ACE2 expressed in the respiratory tract by the influenza virus might be maintained for a relatively long period together with post-viral chronic pulmonary inflammation, similar to what occurs in patients with COPD. In the presence of increased ACE2 levels caused by influenza infection, patients are more susceptible to SARS-CoV-2 infection and more likely to progress to severe COVID-19 [Citation23]. Considering that the above processes are viral dose-dependent, even with influenza viral clearance, severe COVID-19 is more likely to occur in patients with severe influenza infection than in those with asymptomatic or mild influenza infection, accompanied by a chronic inflammatory process with increased ACE2. This is supported by our finding that the complicated influenza group, particularly those infected less than 1 year before COVID-19 infection, had a higher incidence of severe COVID-19 than the uncomplicated influenza group. However, in the context of viral infection, the role of IFN signalling has been the subject of much debate, with both protective and deleterious effects documented in different experimental systems and clinical settings [Citation39]. At present, the interaction between type 1 and type 2 inflammation with respect to influenza infection is not understood, and how type 1 and type 2 inflammation change and progress over time in lung tissue. It is also not known whether the increase in ACE2 caused by influenza in the respiratory tract can be sustained for a long period or continue chronically beyond the acute phase. Further studies are required to identify the relationship between recent influenza infection and severe COVID-19.
Implications for policy and practice
SARS-CoV-2 and influenza viruses will co-exist or co-circulate in the general population in the future [Citation40]. If this is the case, the control of influenza infection could be an important method for preventing severe illness from SARS-CoV-2 infection [Citation40]. It is already known that influenza vaccination is one of the best interventions to prevent influenza. Recently, it was reported that influenza vaccination can help prevent severe COVID-19, although the mechanism is unclear [Citation14,Citation15]. Considering the results of our study, direct prevention of influenza virus infection by vaccination may be another mechanism for preventing the progression of COVID-19 to severe illness. The results of our study serve as important evidence confirming the results from other studies that showed that influenza vaccination may reduce the mortality from COVID-19 and may prevent the progression to severe COVID-19 illness [Citation14,Citation15]. Therefore, it is necessary to maintain and encourage policies to increase influenza vaccination rates in the elderly, especially chronically ill or immunocompromised patients.
In addition to influenza vaccination, public health measures are required to effectively control concurrent influenza and SARS-CoV-2 viral infections [Citation40]. Multiple public health interventions such as hand hygiene, cough etiquette, staying at home with respiratory symptoms, and universal mask use in public places, already implemented in Korea to prevent the spread of COVID-19 during the 2019–2020 influenza season, resulted in early termination of influenza and a substantial decrease in seasonal influenza activity along with containment of the spread of COVID-19 [Citation41]. Non-pharmaceutical interventions (NPIs) and behavioural changes in China and the United States play a role in reducing influenza activity [Citation42]. Co-circulating influenza and SARS-CoV-2 may result in a greater health burden on hospital and community capacities and resources than has been experienced during the current COVID-19 pandemic [Citation40]. Therefore, beyond the current epidemic wave of COVID-19, public health measures to control influenza activity, such as hand washing, cough etiquette, mask wearing, and vaccination, are important tools to reduce the risk of progression to severe illness from COVID-19 infection, which increases the burden on the public healthcare system.
Strengths and weaknesses of this study
The strength of this study is that it includes a large cohort formed from the general population in Korea within 10 months before the onset of the COVID-19 pandemic so that the relationship between influenza and SARS-CoV-2 can be evaluated. The 3 T strategy (testing, tracing, and treatment) has been established as an important measure for COVID-19 prevention and control in the Republic of Korea. In this strategy, the COVID-19 diagnostic test plays a major role because early and rapid detection is important to prevent the SARS-CoV-2 infection from spreading [Citation43,Citation44]. The Korean government has made efforts to increase access to diagnostic tests by opening up screening stations where suspected cases could be promptly diagnosed. The maximum daily testing capacity increased from 3000 individuals in February 2020 to approximately 20,000 individuals in April 2020, and real-time RT-PCR tests for SARS-CoV-2 were performed by national central laboratories and 95 non-governmental clinical laboratories [Citation43,Citation45]. Therefore, rapid and widespread PCR testing has been conducted to detect confirmed cases at a relatively early stage of the pandemic. The results of this study were derived from a cohort of approximately 300,000 individuals from the NHIS during the first 10 months of the COVID-19 pandemic.
This study had several limitations. First, we did not evaluate whether influenza vaccination reduced the severity of COVID-19 illness. As we could not access individual histories of influenza vaccination in the Korean NHIS claim-based database, we could not confirm the influenza vaccination history. However, the influenza vaccination rate in the entire population of Korea was low at less than 40%, and the prevalence in elderly patients aged ≥65 who had a > 80% rate of influenza vaccination was less than 20% in this study [Citation46]. Therefore, it is estimated that the influence of influenza vaccination did not significantly affect the results of our study.
Second, we did not fully address selection bias because we did not include patients with asymptomatic or mild influenza infection who did not receive health care. We retrospectively analysed data from individuals diagnosed with influenza held by the National Health Insurance Service. There was a limitation in confirming whether influenza was present in individuals who did not receive medical care. As yet, there have been no research results on the incidence of asymptomatic influenza patients in Korea; however, the seasonal incidence of symptomatic influenza in the United States was reported to be approximately 8% and varied from 3% to 11% among seasons [Citation47]. Therefore, almost 90% of patients with influenza may be asymptomatic. In a prospective cohort study conducted in South Africa, approximately half of the influenza infections were asymptomatic [Citation48]. In addition, in a meta-analysis, estimates of the asymptomatic fraction in outbreak investigations fell within the range of 4% to 28%, with a mean of 16% (95% CI: 13%, 19%) [Citation49]. Estimates of the asymptomatic fraction based on cohort studies without adjustment for other illnesses were spread over a wide range from 0% to 100%, and estimates from studies adjusted for background illnesses ranged from 65% to 85% [Citation49]. Therefore, at present, there is no established range for the proportion of asymptomatic patients with influenza viral infection.
The actual asymptomatic fraction of influenza virus infections can be affected by the study design, the definitions of infection and symptomatic illness, the influenza type, the vaccination status, and the age stratification of patients [Citation49]. There may have been a selection bias in which asymptomatic patients were not included in this study, but there will be limitations in assessing the extent to which this has influenced the results of our study. Almost all Koreans (97%) are enrolled in the National Health Insurance Service, and most receive medical treatment at least once a year [Citation17]. The greatest advantage of these data is that they include virtually the entire population [Citation17]. According to a domestic study on the patient's perspective of common cold and health care utilization, when Koreans detected the symptoms of upper respiratory infection (URI), 90% of them took medication, and approximately 60% of all patients visited the primary care clinic and 40% visited the pharmacy [Citation50]. In particular, people were more likely to visit a physician if they experienced typical URI-related symptoms such as fever, cough, sore throat, rhinorrhea, or if any URI-related symptoms were prolonged [Citation50]. Therefore, although our study did not include asymptomatic individuals or some patients with mild symptoms who did not receive medical care, it was judged that most patients with influenza symptoms were included in this study, even if they had mild symptoms.
Third, we did not adjust for additional unmeasured confounding factors, such as smoking, immunosuppression, and pregnancy, although we included histories of malignancy, diabetes mellitus, angina, myocardial infarction, and chronic kidney disease, which are associated with these factors. Therefore, we calculated the E-value for unmeasured confounders. We found that the observed OR of 1.54 with a 95% CI of 1.20-1.98 could be explained by an unmeasured confounder that was associated with both influenza infection less than 1 year before COVID-19 onset and risk of incident severe illness from COVID-19 by a risk ratio of 2.45 (CI of 1.69), above and beyond the measured confounders, but weaker confounding would not do so. The CI could be moved to include the null by an unmeasured confounder that was associated with both influenza infection less than 1 year before COVID-19 onset and risk of incident severe illness from COVID-19 by a risk ratio of 1.69-fold, above and beyond the measured confounders, but weaker confounding would not do so. In this study, it seems plausible to have an unmeasured confounder with a strong association with both influenza infection less than 1 year before COVID-19 onset and risk of incident severe illness from COVID-19, especially given that the E-value of 2.45 (CI of 1.69) is very similar to or less than that observed for well-known risk factors for severe illness from COVID-19, such as old age, cancer, and HTN. Because the OR of old age is 7.08, which is greater than 2.45, and the OR values of cancer, HTN, and COPD are greater than 1.69, the E-value of 2.45 is relatively modest.
Conclusions
Influenza infection less than a year before SARS-CoV-2 infection is a risk factor for severe illness from SARS-CoV-2 infection. Therefore, effective public health control strategies to minimize the influenza burden, such as hand washing, cough etiquette, and mask wearing, as well as influenza vaccination, should be in place to minimize the enormous impact on the healthcare system at a time of co-existence of influenza and SARS-CoV-2 infections.
Ethics statements
This study was approved by the Institutional Review Board (IRB) of Jeonbuk National University Hospital (No. 2020-04-067).
Acknowledgments
This research was supported by a grant of the Korea Health Technology R&D Project through the Korea Health Industry Development Institute (KHIDI), funded by the Ministry of Health & Welfare, Republic of Korea (grant number : HI22C1124) and by the fund of the Biomedical Research Institute, Jeonbuk National University Hospital.
Data availability statement
The datasets analyzed in this study are stored in the Korean National Health Insurance claim-based database. The data will be made available for research on reasonable request and with permission from the National Health Insurance Service (NHIS) (NHIS-2022-1-382).
Disclosure statement
No potential conflict of interest was reported by the author(s).
Additional information
Funding
References
- Bresee J, Fitzner J, Campbell H, et al. Progress and remaining gaps in estimating the global disease burden of influenza. Emerg Infect Dis. 2018;24(7):1173–1177. doi:10.3201/eid2407.171270. [published Online First: 2018/06/19].
- Sullivan SG, Cowling BJ. Reconciling estimates of the global influenza burden. Lancet Respir Med. 2019;7(1):8–9. doi:10.1016/S2213-2600(18)30511-3. [published Online First: 2018/12/17].
- Gandhi RT, Lynch JB, Del Rio C. Mild or Moderate Covid-19. N Engl J Med. 2020;383(18):1757–1766. doi:10.1056/NEJMcp2009249. [published Online First: 2020/04/25].
- Cucinotta D, Vanelli M. WHO declares COVID-19 a pandemic. Acta Biomed. 2020 Mar 19;91(1):157–160.
- WHO. Weekly epidemiological update on COVID-19 - 6 January 2022. https://www.who.int/publications/m/item/weekly-epidemiological-update-on-covid-19—6-january-2022.
- Kompaniyets L, Pennington AF, Goodman AB, et al. Underlying medical conditions and severe illness among 540,667 adults hospitalized with COVID-19, 2021. Prev Chronic Dis. 2021;18:E66, doi:10.5888/pcd18.210123. [published Online First: 2021/07/02].
- Harrison SL, Fazio-Eynullayeva E, DA L, et al. Comorbidities associated with mortality in 31,461 adults with COVID-19 in the United States: a federated electronic medical record analysis. PLoS Med. 2020;17(9):e1003321, doi:10.1371/journal.pmed.1003321. [published Online First: 2020/09/11].
- Emanuel EJ, Persad G, Upshur R, et al. Fair allocation of scarce medical resources in the time of COVID-19. N Engl J Med. 2020;382(21):2049–2055. doi:10.1056/NEJMsb2005114. [published Online First: 2020/03/24].
- Berlin DA, Gulick RM, Martinez FJ. Severe Covid-19. N Engl J Med. 2020;383(25):2451–2460. doi:10.1056/NEJMcp2009575. [published Online First: 2020/05/16].
- Zheng J, Chen F, Wu K, et al. Clinical and virological impact of single and dual infections with influenza A (H1N1) and SARS-CoV-2 in adult inpatients. PLoS Negl Trop Dis. 2021;15(11):e0009997, doi:10.1371/journal.pntd.0009997. [published Online First: 2021/11/30].
- Bao L, Deng W, Qi F, et al. Sequential infection with H1N1 and SARS-CoV-2 aggravated COVID-19 pathogenesis in a mammalian model, and co-vaccination as an effective method of prevention of COVID-19 and influenza. Signal Transduct Target Ther. 2021;6(1):200, doi:10.1038/s41392-021-00618-z. [published Online First: 2021/05/22].
- Zhang AJ, Lee AC, Chan JF, et al. Coinfection by severe acute respiratory syndrome coronavirus 2 and influenza A(H1N1)pdm09 virus enhances the severity of pneumonia in golden Syrian hamsters. Clin Infect Dis. 2021;72(12):e978–e992. doi:10.1093/cid/ciaa1747. [published Online First: 2020/11/21].
- Yue H, Zhang M, Xing L, et al. The epidemiology and clinical characteristics of co-infection of SARS-CoV-2 and influenza viruses in patients during COVID-19 outbreak. J Med Virol. 2020;92(11):2870–2873. doi:10.1002/jmv.26163. [published Online First: 2020/06/13].
- Stańczak-Mrozek KI, Sobczak A, Lipiński L, et al. The potential benefits of the influenza vaccination on COVID-19 mortality rate—a retrospective analysis of patients in poland. Vaccines (Basel). 2022;10(1):5.
- Hosseini-Moghaddam SM, He S, Calzavara A, et al. Association of influenza vaccination with SARS-CoV-2 infection and associated hospitalization and mortality among patients aged 66 years or older. JAMA Netw Open. 2022;5(9):e2233730.
- Guan Z, Chen C, Li Y, et al. Impact of coinfection with SARS-CoV-2 and influenza on disease severity: a systematic review and meta-analysis. Front Public Health. 2021;9:773130.
- Kyoung DS, Kim HS. Understanding and utilizing claim data from the Korean national health insurance service (NHIS) and health insurance review & assessment (HIRA) database for research. J Lipid Atheroscler. 2022 May;11(2):103–110.
- Zhang Z, Kim HJ, Lonjon G, et al. Written on behalf of AME big-data clinical trial collaborative group. balance diagnostics after propensity score matching. Ann Transl Med. 2019 Jan;7(1):16.
- VanderWeele TJ, Ding P. Sensitivity analysis in observational research: introducing the E-value. Ann Intern Med. 2017;167(4):268–274.
- Peng JY, Shin DL, Li G, et al. Time-dependent viral interference between influenza virus and coronavirus in the infection of differentiated porcine airway epithelial cells. Virulence. 2021;12(1):1111–1121. doi:10.1080/21505594.2021.1911148. [published Online First: 2021/05/27].
- Schweitzer KS, Crue T, Nall JM, et al. Influenza virus infection increases ACE2 expression and shedding in human small airway epithelial cells. Eur Respir J. 2021 Jul 1;58(1):2003988.
- Ni W, Yang X, Yang D, et al. Role of angiotensin-converting enzyme 2 (ACE2) in COVID-19. Crit Care. 2020;24(1):422.
- Zheng M. ACE2 and COVID-19 susceptibility and severity. Aging Dis. 2022 Apr 1;13(2):360–372.
- Sajuthi SP, DeFord P, Li Y, et al. Type 2 and interferon inflammation regulate SARS-CoV-2 entry factor expression in the airway epithelium. Nat Commun. 2020;11(1):5139.
- Wang J, Nikrad MP, Phang T, et al. Innate immune response to influenza a virus in differentiated human alveolar type II cells. Am J Respir Cell Mol Biol. 2011;45(3):582–591.
- Ziegler CGK, Allon SJ, Nyquist SK, et al. SARS-CoV-2 receptor ACE2 Is an interferon-stimulated gene in human airway epithelial cells and Is detected in specific cell subsets across tissues. Cell. 2020;181(5):1016–1035.e19.
- Heaton NS, Langlois RA, Sachs D, et al. Long-term survival of influenza virus infected club cells drives immunopathology. J Exp Med. 2014;211:1707–1714.
- Keeler SP, Agapov EV, Hinojosa ME, et al. Influenza a virus infection causes chronic lung disease linked to sites of active viral RNA remnants. J Immunol. 2018;201(8):2354–2368.
- Sun J, Madan R, Karp CL, et al. Effector T cells control lung inflammation during acute influenza virus infection by producing IL-10. Nat Med. 2009;15:277–284.
- Heuberger J, Trimpert J, Vladimirova D, et al. Epithelial response to IFN-γ promotes SARS-CoV-2 infection. EMBO Mol Med. 2021 Apr 9;13(4):e13191.
- Mauad T, Hajjar LA, Callegari GD, et al. Lung pathology in fatal novel human influenza a (H1N1) infection. Am J Respir Crit Care Med. 2010;181(1):72–79.
- Wiley JA, Cerwenka A, Harkema JR, et al. Production of interferon-γ by influenza hemagglutinin-specific CD8 effector T cells influences the development of pulmonary immunopathology. Am J Pathol. 2001;158(1):119–130.
- Hamilton JR, Sachs D, Lim JK, et al. Club cells surviving influenza A virus infection induce temporary nonspecific antiviral immunity. Proc Natl Acad Sci U S A. 2016;113:3861–3866.
- Dumm RE, Fiege JK, Waring BM, et al. Non-lytic clearance of influenza B virus from infected cells preserves epithelial barrier function. Nat Commun. 2019;10:779.
- Garcia GL, Valenzuela A, Manzoni T, et al. Distinct chronic post-viral lung diseases upon infection with influenza or parainfluenza viruses differentially impact superinfection outcome. Am J Pathol. 2020;190(3):543–553.
- Zamorano Cuervo N, Grandvaux N. ACE2: Evidence of role as entry receptor for SARS-CoV-2 and implications in comorbidities. Elife. 2020 Nov 9;9:e61390.
- Leung JM, Yang CX, Tam A. ACE-2 expression in the small airway epithelia of smokers and COPD patients: implications for COVID-19. Eur Respir J. 2020 May 14;55(5):2000688.
- Bui LT, Winters NI, Chung MI, et al. Chronic lung diseases are associated with gene expression programs favoring SARS-CoV-2 entry and severity. Nat Commun. 2021;12(1):4314.
- McNab F, Mayer-Barber K, Sher A, et al. Type I interferons in infectious disease. Nat Rev Immunol. 2015;15(2):87–103.
- Chotpitayasunondh T, Fischer TK, Heraud JM, et al. Influenza and COVID-19: what does co-existence mean? Influenza Other Respir Viruses. 2021;15(3):407–412. doi:10.1111/irv.12824. [published Online First: 2020/11/01].
- Lee H, Lee H, Song KH, et al. Impact of public health interventions on seasonal influenza activity during the COVID-19 outbreak in Korea. Clin Infect Dis. 2021;73(1):e132–e140.
- Feng L, Zhang T, Wang Q, et al. Impact of COVID-19 outbreaks and interventions on influenza in China and the United States. Nat Commun. 2021;12(1):3249.
- Ministry of Health and Welfare. Coronavirus (COVID-19), Republic of Korea: about COVID-19: COVID-19 response. http://ncov.mohw.go.kr/en/baroView.do?brdId=11&brdGubun=111&dataGubun=&ncvContSeq=&contSeq=&board_id=.
- Shin HS, Park H, Kwon JS, et al. National academy of medicine of Korea (NAMOK) Key statements on COVID-19. J Korean Med Sci. 2021;36(41):e287, doi:10.3346/jkms.2021.36.e287. [published Online First: 2021/10/27].
- Kim YJ, Sung H, Ki CS, et al. COVID-19 testing in South Korea: current status and the need for faster diagnostics. Ann Lab Med. 2020;40(5):349–350. doi:10.3343/alm.2020.40.5.349. [published Online First: 2020/04/03].
- Shin GS, Choi SE. Influenza vaccination status and influencing factors in chronic patients in Korea. Korean Public Health Res. 2020;46(3):59–74. doi:10.22900/kphr.2020.46.3.005.
- Tokars JI, Olsen SJ, Reed C. Seasonal incidence of symptomatic influenza in the United States. Clin Infect Dis. 2018;66(10):1511–1518.
- Cohen C, Kleynhans J, Moyes J, et al. PHIRST Group. asymptomatic transmission and high community burden of seasonal influenza in an urban and a rural community in South Africa, 2017-18 (PHIRST): a population cohort study. Lancet Glob Health. 2021 Jun;9(6):e863–e874.
- Leung NHL, Xu C, Ip DKM, et al. Review Article: The Fraction of Influenza Virus Infections That Are Asymptomatic: A Systematic Review and Meta-analysis. Epidemiology. 2015;26(6):862–872.
- Lee SR, Park EW, Cheong YS, et al. Patient's perspective of common cold and health care utilization. Korean J Fam Med. 2009;30(6):440–448.