ABSTRACT
The current study was designed to comparatively analyse the reactions of different mouse strains in response to acellular pertussis (aP) vaccine, with attempt to further provide a reference for aP vaccine evaluation. NIH mice, ICR mice, and BALB/c mice adopted from different pharmacopoeias and studies were utilized to measure the immune protection and immunogenicity of the same batch of aP vaccine according to the Modified intracerebral challenge assay (MICA) from some Asian pharmacopoeias and the pertussis serological potency test (PTST) method from European Pharmacopoeia. Based on our results, the aP vaccine detected by NIH mice had the best potency. So the NIH mice were more suitable for detecting the immune protection of aP vaccine by the MICA method. Given that the levels of PT-IgG and FHA-IgG antibodies in ICR mice were the highest, and the levels of Th1 and Th2 cells were significantly increased (P < .01), it was more suitable for the detection of immunogenicity of aP vaccine by PSPT method. Spleen lymphocytes were stimulated by PT and FHA. And the levels of IL-4 in ICR mice and NIH mice were significantly increased, so were the levels of IL-17, IL-23, IL-27, and TNF-α in BALB/c mice. NIH mice have stronger adaptive immunity and the weakest inflammatory response, and ICR mice have enhanced adaptive immunity and inflammatory responses, both of which can be thereby used for evaluation by different pharmacopoeia methods. NIH was more suitable for the MICA method of Chinese Pharmacopoeia, and ICR for the PSPT method of European Pharmacopoeia.
Introduction
As we all know, whooping cough is a common acute respiratory disease in infectious diseases, and its epidemic cycle is three to five years [Citation1,Citation2]. Once infants and young children are infected with whooping cough, they may have spasmodic cough and even die in severe cases. Since the 1990s, the incidence of whooping cough has been on the rise all over the world, and it has gradually increased with the passage of time, including many countries with extensive vaccine coverage, such as the United States and Australia [Citation3–6]. Vaccine immunization is usually used to prevent and control whooping cough. And aP vaccine has replaced whole-cell pertussis (wP) vaccine as the main vaccine because of fewer side effects [Citation7].
When it comes to the potency evaluation of the aP vaccine, different standards exist among countries, and there are no agreed standards for methods and animal strains. A guideline of WHO provides two methods to evaluate the immunogenicity of pertussis vaccine, namely Modified intracerebral challenge assay (MICA) and mouse immunogenicity test (MIT). At the same time, new methods such as mouse nasal challenge test were developed [Citation8]. The Chinese Pharmacopoeia, the Japanese Pharmacopoeia, and the Korean Pharmacopoeia all use the MICA method, but NIH mice are recommended by the former two and ICR mice by the latter [Citation9–11]. The pertussis serological potency test (PSPT) method in the MIT method is adopted by the European Pharmacopoeia, in which ICR mice were recommended [12]. Compared with the MICA method, the number of animals in PSPT method is much less and it is more convenient to operate. The US federal regulations for aP vaccine evaluation only provide guidelines without detailed operating procedures. Meanwhile, as the established intranasal infection model, BALB/c mice have been widely used as a research model for evaluating the potency of new aP vaccines [Citation13–15]. Guiso et al. [Citation16] found that the results of aP potency test in BALB/c mice were the same as the protective effect of the vaccine, which was expected to be used as an aP vaccine efficacy evaluation model. The differences in methods and animal strains pose challenges to the scientific validity of the evaluation results. The results of in vivo potency test in animals are related to the type, concentration, mode and frequency of antigen entry into the body, as well as the age, and sex, strain or even substrain of the animal. Therefore, in order to improve the accuracy and repeatability of research results, the ARRIVE 2.0 guidelines pay great attention to the specification of materials, methods, animals and other elements [Citation17]. However, data regarding to whether the outbred stock NIH or ICR mice recommended by pharmacopoeia or inbred strain BALB/c mice can be used as suitable strains are limited.
As the touchstone of scientific research, experimental animals are very important. The difference of laboratory animal strains may destroy the scientific and reliable results. Many studies have shown that different strains of mice may have different immune response effects due to different genetic backgrounds. Poyntz reported that even the same BALB/c mice from different suppliers had significant changes in their humoral immune response spectrum [Citation18]. Mosley showed that 28 kinds of inbred mice immunized with aP vaccine produced different levels and types of antibodies [Citation19,Citation20]. These results provide insights into immune heterogeneity and suggest additional research on the genetic effects of vaccine efficacy caused by different mice. Therefore, it is urgent to evaluate the efficacy of the vaccine to determine the immune response characteristics of different mice immunized with aP vaccine, and to screen out mouse strains suitable for aP immune protection and immunogenicity evaluation.
In this study, NIH, ICR and BALB/c mouse strains were taken as research objects, and the immunoprotection and immunogenicity of the same batch of aP vaccine were determined by Chinese and European Pharmacopoeia. This study can provide the best mouse strain under different standards for the evaluation of aP vaccine.
Materials and methods
Vaccines
The vaccines tested in this study were Diphtheria, tetanus, pertussis (acellular, component), poliomyelitis (inactivated) vaccine and Haemophilus type b conjugate vaccine, adsorbed (DTaP/IPV), containing pertussis toxin (PT) and filamentous haemagglutinin antigens (FHA), 50 μg/ml respectively. All tested vaccines, reference aP vaccines, and pertussis bacillus (CMCC58030) were provided by the National Institute for Food and Drug Control (NIFDC) of China.
Animals
This experiment has been approved by the Animal Welfare Ethics Committee of China Food and Drug Administration (license number: 2020(B)012). ICR mice were purchased from Beijing Vital River Laboratory Animal Technology Co., Ltd. (production license number: SCXK (Beijing) 2016-006), NIH and BALB/c mice were provided by the laboratory animals centrer of the National Institute for Food and Drug Control of China (NIFDC) (production license number: SCXK (Beijing) 2017-005). All animals are kept under certain aseptic conditions. With the permission of the local government, animal vaccination was carried out in the negative pressure barrier facility of NIFDC (license number: SYXK (Beijing)-2017-0013). For the determination of vaccine efficacy by MICA method, 10–12 g half-sex mice of different strains were used. In order to determine the immunogenicity by PSPT method, different strains of five-week-old female mice were selected for subsequent research.
Immunoprotection test of aP vaccine
The procedure for detecting the immune protection of the vaccine followed the MICA method in Chinese Pharmacopoeia (Volume III, 2020 Edition). Taking NIH mice as an example, 170 mice were needed in a batch. Three dilutions of the reference vaccine and tested vaccine were intraperitoneally injected to mice, with 20 mice for each dilution. Vaccine-exposed mice were all challenged with 80,000 Bordetella bacteria/0.03 mL 21 days later. At the same time, a total of 50 NIH mice were injected with sterile saline and defined as the control group. The dose of intraperitoneal injection was 0.5 mL/mouse. Three weeks after immunization, the mice in the control group were challenged with 8, 80, 800, 8000, and 80,000 Bordetella bacteria/0.03 mL, respectively with 10 mice at each concentration. The number of dead mice within 14 days was observed and recorded. LD50 value was calculated by the Reed–Muench method. The potency of the vaccine was calculated as the parallel line assay relating the qualitative reaction.
Immunogenicity detection of aP vaccine
The specific operation steps for detecting the immunogenicity of the study vaccine were based on the European Pharmacopoeia (EP 10.0), namely the PSPT method. Taking NIH mice as an example, 30 mice were needed in a batch. Five-time diluted vaccine was intraperitoneally injected into 10 mice as antibody detection group and 10 mice as cytokine detection group, and with saline injection into 10 mice as a negative control. After three weeks of the first immunization, 10 mice in cytokine group were subjected to five-time diluted vaccine again. Four weeks after the initial immunization, blood samples in both antibody group and control group were collected, and the spleens in cytokine group and control group were harvested. The levels of PT antibody and FHA antibody were detected by ELISA as previously described [Citation21]. The international standard in ELISA was NIBSC 97/642 and the positive control was inhouse serum prepared in our laboratory. 1640 medium (Gibco, Cat. No. C22400500BT) with 5% foetal bovine serum was used in specific stimulation with PT or FHA antigen (10 μg/mL). One hundred microlitres of the stimulator and an equal volume concentration of 106 cells/mL of spleen lymphocyte suspension were added to a 96-well plate and then stimulated for 12 h at 37°C for the assay. The lymphocyte grouping and the levels of cytokines secreted were detected by flow cytometry. Biolegend (Cat. No. 740150) kit was used for IL-4, IL-10, IL-17A, IL-23, IL-27, IFN-γ, MCP-1, GM-CSF and TGF-α expression.
Statistical analysis
The antibody levels of different strains of mice were compared by one-way ANOVA with SPSS 21 software. The results are expressed as geometric mean standard deviation (GMT ± S). We also used Student T Test to compare the lymphocyte content and cytokine level of different strains of mice before and after immunization. The results are expressed as the average standard deviation. Statistical significance was assessed as P < .05. (two-sided)
aP vaccine evaluation
The immune protection effect of aP vaccine was evaluated by the MICA method. As clearly described in the Chinese Pharmacopoeia [Citation22],when the limit of LD50 for pertussis challenge bacteria was between 100 and 1000, the test was valid. And a qualified aP vaccine destined for use in China must meet the following requirements: the potency of aP vaccine must be ≥8 IU/ml and the lower limit of 95% confidence ≥4 IU/ml.
Immunogenicity was evaluated by antibody level according to PSPT method. When PT antibody ≥1 EU/mL and FHA antibody ≥10 EU/mL, it was considered to appear positive conversion. And if there were more than 8 mice in 10 showed positive conversion, the test was valid. The levels of PT-IgG and FHA-IgG antibodies produced by ICR, NIH and BALB/c mice were compared to analyse the immunogenicity of different mouse strains. In addition, we also measured the cytokine levels before and after aP vaccine immunization to determine the immune heterogeneity among the three strains.
Results
Immune protective results of different strains of mice
The number of LD50 for pertussis challenge bacteria in ICR, NIH, and BALB/c mice was 428.214, 995.467 and 292.139, respectively, which all met the standard of 100–1000. The immune protection efficacy of ICR, NIH and BALB/c mice was measured with the same batch of aP vaccine. As shown in , the vaccine efficacy and 95% efficacy lower limit of ICR and NIH mice are higher than the standard values and can be defined as qualified, while the results of BALB/c mice are lower than the standard values and are defined as unqualified.
Table 1. Immune protection efficacy determination.
Immunogenicity results of different strains of mice
Anti-pertussis antibody content in the serum of different strains of mice
The antibody-positive rates of PT and FHA in ICR mice were all 100%. The PT antibody positive conversion rate of NIH mice was 100%, and the FHA antibody positive conversion rate was 90%. The positive rate of PT antibody in BALB/c mice was 90%, and the positive rate of FHA antibody was 100%.
As shown in , the levels of PT-IgG antibody and FHA-IgG antibody produced by ICR mice were the highest, followed by NIH, and the level of anti-pertussis antibody in BALB/c mice was the lowest.
Figure 1. Levels of PT-IgG and FHA-IgG in different strains of mice after immunization. (A) and (B) represent the levels of PT-IgG and FHA-IgG in each strain of mice after immunization; * indicates P < .05, ** indicates P < .01. Sample size: n = 10. The antibody titre is represented by geometric mean and standard deviation.
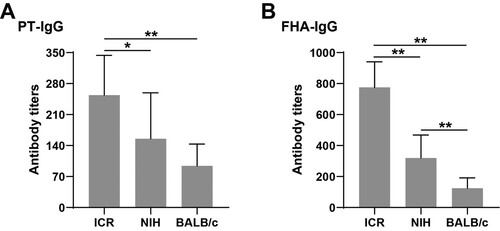
Changes of lymphocyte content in different strains of mice
In comparison to control mice, the relative content of subsets of T helper (Th) cells changed greatly before and after immunization in the determined mouse strains. The levels of Th1 and Th2 cells in ICR and NIH mice was significantly increased ((A,B), P < .01). The levels of Th17 cells failed to show statistical differences in the two strains, despite the result that there existed an upward trend in numerical value. However, an inverse result was found in BALB/c mice. Only Th17 cells in BALB/c mice increased significantly ((C), P < .01). Meanwhile, the level of Th1 cells in BALB/c mice declined after immunization ((C), P < .05).
Changes of cytokine content in different strains of mice after antigen stimulation
As presented in , the level of IL-4 in spleen lymphocytes from ICR and NIH mice were significantly increased after PT stimulation ((A,B), P < .01). Likewise, the levels of IL-4, IL-10, IL-17A, IL-23, IL-27, and TNF-α in spleen lymphocytes from BALB/c mice were significantly increased ((C)).
Figure 3. Changes of cytokines in three strains of mice after PT antigen stimulation. (A), (B), and (C) represent the changes of cytokines in ICR, NIH, and BALB/c mice after PT stimulation. * indicates P < .05, ** indicates P < .01. Sample size: n = 10.
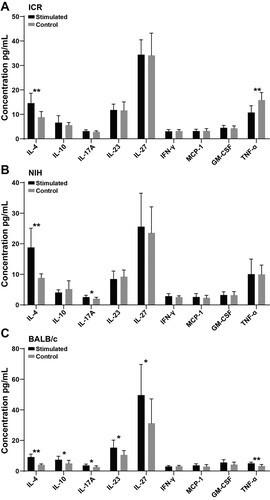
When measured in lymphocytes stimulated by FHA from mouse spleen, the changes of cytokine expression are very different. As shown in , down-regulated expressions of IL-10 and MCP-1 were determined in NIH and BALB/c mice after FHA stimulation ((A,C)). The increase of IL-4 level can only be determined in NIH and BALB/c mice stimulated by FHA ((B,C)). Interestingly, the level of IFN-γ in lymphocytes stimulated by FHA from NIH mice increased, while the level of IFN-γ in lymphocytes stimulated by FHA from BALB/c mice decreased ((B,C)).
Discussion
Under the guidance of WHO, several laboratories have taken JNIH-3 as a reference vaccine (proposed by our laboratory) for aP vaccine screening and for exploring the effect of active pertussis toxin on the vaccine [Citation23]. However, the choice of mouse strain varied from country to country [Citation24]. A previous study on 28 inbred mice showed that genetic factors in experimental animals had a significant effect on IgG subclass content and antibody longevity [Citation20]. However, they were limited to inbred or outbred mice in the selection of experimental animals. Efforts had been made in our study to reveal that by subjecting ICR, NIH, and BALB/c mice to aP vaccine. The immunoprotection and immunogenicity of the same batch of aP vaccine were compared to screen out the mouse strains that were suitable for the MICA and PSPT method in potency of acellular pertussis vaccine evaluation.
We compared the LD50 values of ICR, NIH, BALB/c mice (10–12 g) and the protective potency of the same aP vaccine to analyse the effect of genetic background. The LD50 value indicated the resistance of mice to pertussis. The potency detected by the same batch of aP vaccine reflected the applicability of different mouse strains. From the pertussis resistance data, it can be seen that the challenge protection against pertussis bacteria exists higher in NIH than that in ICR and BALB/c mice. The immune protection results detected by NIH mice and ICR mice could determine that the aP vaccine was qualified, while the results of BALB/c mice were unqualified. NIH mice were associated with the strongest resistance to Bordetella pertussis and had the best potency. Therefore, it is appropriate to use NIH mice to detect the immune protective titre of aP vaccine by the MICA method.
MICA is the only clinically relevant method in detecting the overall immune response after vaccination [Citation25]. However, the MICA method uses a large number of mice, requires high skills for operation, and well-experienced staff for results determination [Citation26,Citation27]. The PSPT method is recommended for immunogenicity detection of aP vaccine by European Pharmacopoeia. It mainly detects the content of anti-pertussis antigen-specific IgG antibodies, which reflects the humoral immune response produced by aP vaccine [Citation28,Citation29]. The PSPT method has been proven to be an effective method for estimating the potency of pertussis vaccines from different laboratories [Citation30]. Previous studies compared the results between PSPT and MICA methods and found that the pertussis effect values detected by the two methods were similar [Citation29,Citation31–33]. In these studies, it was found that there were significant differences in results among laboratories, which may be caused by different mouse strains. Currently, studies have shown that T cell subsets can be used as a supplementary parameter for the detection of aP immunogenicity by PSPT [Citation34]. Nevertheless, not all mouse strains are suitable. We found that the content of Th1 and Th2 cells in ICR and NIH mice increased significantly after immunization. The results in BALB/c mice are only Th17 cells increased significantly after immunization, while Th1 cells went down. So the BALB/c mice appear to be a model that could react with neither an induced Th1-biased nor an induced Th2-biased immune response. Some previous studies also reported the difference T cell subset results caused by different mice. Yano et al. [Citation35] have found that C57BL/6 mice were used to compare the immune responses of wP and aP, and it was found that wP was more likely to induce Th1-dominant responses, while aP vaccine was more likely to induce Th2-dominant responses. The results of NIH and ICR were consistent with the results of C57BL/6, but the BALB/c mice showed a significant difference in Th17 dominant response. Hoonakker et al. [Citation34] used NIH mice and ICR mice weighing 20–24 g to study three immunization dose groups of whole cell pertussis vaccine (wP) and found that the adaptive immune responses induced by different doses of wP vaccine were different. Low doses of vaccine produced generally lower Th1, Th2 and Th17. Adult healthy ICR mice aged approximately five weeks were immunized with a five-time diluted vaccine according to European Pharmacopoeia. According to the growth curves of the three kinds of mice in two vendors, the animal’s weights in our study were around 15 g in PSPT methods, which differed from Hoonakker’s study [Citation34]. This also suggests that we need to pay more attention to the selection and specification of animals in future research, because even different substrains may produce completely different results [Citation36–39].
The results of T cell subsets and cytokines were basically consistent in each mouse strain. In NIH mice, there was a significant increase in IL-4 after PT stimulation and the increase in IFN-γ after FHA stimulation, suggesting that the mainly activated T cells were Th1 and Th2 ((B)). In ICR mice, IL-4 significantly increased after PT antigen stimulation, which mutually corroborated by the activation of Th2 ((A)). However, the results of BALB/c showed that the increase of IL-17, IL-27 and IL-23 indicated that Th17 cells were mainly activated ((C)).
It was worth noting that there were some cytokine decreases in ICR and BALB/c, especially after FHA stimulation. IL-10 and MCP-1 were decreased in both mice, while IFN-γ was only in BALB/c. It seemed to be abnormal. However, there were several factors could influence the level of cytokine. Avila et al. [Citation40] had reported that IFN-γ expression fluctuates over time in BALB/c, and is also influenced by different antigens. From 1-12 h, the increase in IFN-γ existed all the time with smooth OMV from Brucella melitensis, but decreased in 12 h with mutant rough OMV from Brucella melitensis. And in ICR mice, Hoonakker also found that after high, middle and low dose immunization, the levels of IFN-γ were all lower than that of the control group [Citation34].
Our study has limitations. Firstly, we focused on the protective immunity after stimulated with aP vaccine using MICA and PSPT methods in the three mouse strains. So some details were ignored such as the possible effect of PT mitogenicity on cell stimulation. Moreover, not all of the typical cytokines (like IL-2) were included in this study with the limitation of commercial kit.
Nonetheless, the data in immune protection and immunogenicity strongly support that different mouse strains may be the main influence for aP evaluation. After a comprehensive analysis of the differences in cells and cytokines of each strain of mice, it was found that after immunization with aP, the adaptive immune response of ICR and NIH mice was stronger, while the adaptive immune response of BALB/c mice was weaker. ICR mice had a certain inflammatory response. NIH mice had the weakest inflammatory response, while the most severe inflammatory response was identified in BALB/c mice. This characteristic of BALB/c mice may be the reason for their poor resistance to pertussis in brain cavity attacks.
Conclusion
In this study, the immune response effects of three strains of mice commonly used in aP potency evaluation after vaccine immunization were comprehensively explored. Mouse strains suitable for evaluating vaccine efficacy by MICA method and PSPT method were screened out, which contributed to further standardizing vaccine evaluation procedures. However, our study did not involve the screening and comparison of different batches, ages and mouse sublines. Further research is needed in the follow-up study to support the evaluation and industrial development of pertussis vaccine.
Disclosure statement
No potential conflict of interest was reported by the author(s).
Additional information
Funding
References
- Kilgore PE, Salim AM, Zervos MJ, et al. Pertussis: microbiology, disease, treatment, and prevention. Clin Microbiol Rev. 2016;29(3):449-486. DOI:10.1128/CMR.00083-15
- van der Zee A, Schellekens JF, Mooi FR. Laboratory diagnosis of pertussis. Clin Microbiol Rev 2015;28(4):1005–1026. DOI:10.1128/CMR.00031-15
- Lefrancq N, Bouchez V, Fernandes N, et al. Global spatial dynamics and vaccine-induced fitness changes of Bordetella pertussis. Sci Transl Med. 2022;14(642):eabn3253. DOI:10.1126/scitranslmed.abn3253
- Boulet SL, Chamberlain AT, Biswas HH, et al. Trends in infant pertussis hospitalizations in the United States, 2009-2017. JAMA. 2019;322(21):2134–2136. DOI:10.1001/jama.2019.15577
- Domenech de Celles M, Magpantay FMG, King AA, et al. The impact of past vaccination coverage and immunity on pertussis resurgence. Sci Transl Med. 2018;10(434).
- Harmon GE. Seasonal incidence of whooping cough in the United States. Am J Public Health Nations Health. 1932;22(8):831–839. DOI:10.2105/AJPH.22.8.831
- Wood N, Nolan T, Marshall H, et al. Immunogenicity and safety of monovalent acellular pertussis vaccine at birth: a randomized clinical trial. JAMA Pediatr. 2018;172(11):1045–1052. DOI:10.1001/jamapediatrics.2018.2349
- Trs Annex P, Trs AT. Recommendations to assure the quality, safety and efficacy of live attenuated yellow fever vaccines.
- Commission CP. Pharmacopoeia of the People’s Republic of China. People’s Medical Publishing House; 2015.
- Kyokai NK. The Japanese pharmacopoeia; 2006.
- Korea. Korean pharmacopoeia. 10.0 ed. Korean Pharmacopoeia; 2012.
- Europe. European Pharmacopoeia. 10.0 ed. 2019.
- Dorji D, Graham RM, Singh AK, et al. Immunogenicity and protective potential of Bordetella pertussis biofilm and its associated antigens in a murine model. Cell Immunol 2019;337:42–47. DOI:10.1016/j.cellimm.2019.01.006
- van Beek LF, de Gouw D, Eleveld MJ, et al. Adaptation of Bordetella pertussis to the respiratory tract. J Infect Dis 2018;217(12):1987–1996. DOI:10.1093/infdis/jiy125
- Komatsu E, Yamaguchi F, Abe A, et al. Synergic effect of genotype changes in pertussis toxin and pertactin on adaptation to an acellular pertussis vaccine in the murine intranasal challenge model. Clin Vaccine Immunol CVI. 2010;17(5):807–812.
- Guiso N, Capiau C, Carletti G, et al. Intranasal murine model of Bordetella pertussis infection. I. Prediction of protection in human infants by acellular vaccines. Vaccine. 1999;17(19):2366–2376. DOI:10.1016/S0264-410X(99)00037-7
- Percie du Sert N, Hurst V, Ahluwalia A, et al. The ARRIVE guidelines 2.0: updated guidelines for reporting animal research. Br J Pharmacol 2020;177(16):3617–3624. DOI:10.1111/bph.15193
- Poyntz HC, Jones A, Jauregui R, et al. Genetic regulation of antibody responsiveness to immunization in substrains of BALB/c mice. Immunol Cell Biol 2019;97(1):39–53. DOI:10.1111/imcb.12199
- Mosley YC, Radder JE, HogenEsch H. Genetic variation in the magnitude and longevity of the IgG subclass response to a diphtheria-tetanus-acellular pertussis (DTaP) vaccine in mice. Vaccines (Basel). 2019;7(4).
- Mosley YC, Radder JE, Berndt A, et al. Genome-Wide association mapping of the antibody response to diphtheria, tetanus and acellular pertussis vaccine in mice. J Infect Dis 2017;215(3):466–474.
- Ying-Hua XU, Luo P, Wang LC. Development and validation of ELISA assays for determination of serum antibody against pertussis toxin, filamentous hemagglutinin, pertactin of Bordetella pertussis. Chin J Vaccine Immun; 2011.
- Commission CP. Pharmacopoeia of The People’s Republic of China. China Medicine Science Press; 2020.
- Knezevic I, Baca-Estrada M, Xing DK, et al. WHO working group meeting on standardization of acellular pertussis vaccines: potency assay Beijing, People’s Republic of China, 7-9 November 2007. Vaccine. 2008;26(32):3960–3968.
- Gaines-Das R, Horiuchi Y, Zhang SM, et al. Modified intra-cerebral challenge assay for acellular pertussis vaccines: comparisons among whole cell and acellular vaccines. Vaccine. 2009;27(49):6824–6832. DOI:10.1016/j.vaccine.2009.09.014
- Xing D, Asokanathan C, Xu YH, et al. Relationship of immunogenicity to protective potency in acellular pertussis vaccines. Hum Vaccin Immunother. 2014;10(7):2066–2073. DOI:10.4161/hv.28765
- Isomura S. Clinical studies on efficacy and safety of acellular pertussis vaccine. Tokai J Exp Clin Med 1988;13:39–43.
- Sato Y, Sato H. Further characterization of Japanese acellular pertussis vaccine prepared in 1988 by 6 Japanese manufacturers. Tokai J Exp Clin Med 1988;13(Suppl):79–88.
- von Hunolstein C, Gomez Miguel MJ, Pezzella C, et al. Evaluation of two serological methods for potency testing of whole cell pertussis vaccines. Pharmeuropa Bio. 2008;1:7–18.
- van der Ark A, van Straaten-van de Kappelle I, Olander RM, et al. The pertussis serological potency test. collaborative study to evaluate replacement of the mouse protection test. Biologicals. 2000;28(2):105–118.
- Li SH, Xiao ZR, Liu BK. Establishment and adaptivity of pertussis serological potency test. Chin J Biologicals. 2009;22(11):1105–1109.
- Mohammadbagher D, Noofeli M, Karimi G. Comparative assessment of the whole-cell pertussis vaccine potency using serological and intracerebral mouse protection methods. Arch Razi Inst. 2019;74(2):103–109.
- van Der Ark A, Straaten-Van De Kappelle v, Hendriksen II, et al. Pertussis serological potency test as an alternative to the intracerebral mouse protection test: development, evaluation and validation. Altex. 1998;15(5):33–36.
- van der Ark A, van Straaten-van de Kappelle I, Hendriksen C, et al. Pertussis serological potency test as an alternatively to the intracerebral mouse protection test. Dev Biol Stand 1996;86:271–281.
- Hoonakker ME, Verhagen LM, van der Maas L, et al. Adaptive immune response to whole cell pertussis vaccine reflects vaccine quality: A possible complementation to the Pertussis Serological Potency test. Vaccine. 2016;34(37):4429–4436. DOI:10.1016/j.vaccine.2016.07.011
- Yano A, Komatsu T, Ishibashi M, et al. Potent CTL induction by a whole cell pertussis vaccine in anti-tumor peptide immunotherapy. Microbiol Immunol 2007;51(7):685–699. DOI:10.1111/j.1348-0421.2007.tb03957.x
- Treger RS, Pope SD, Kong Y, et al. The lupus susceptibility locus Sgp3 encodes the suppressor of endogenous retrovirus expression SNERV. Immunity. 2019;50(2):334–347.e9. DOI:10.1016/j.immuni.2018.12.022
- Ulland TK, Jain N, Hornick EE, et al. Nlrp12 mutation causes C57BL/6J strain-specific defect in neutrophil recruitment. Nat Commun. 2016;7:13180. DOI:10.1038/ncomms13180
- Kaushansky A, Austin LS, Mikolajczak SA, et al. Susceptibility to Plasmodium yoelii preerythrocytic infection in BALB/c substrains is determined at the point of hepatocyte invasion. Infect Immun 2015;83(1):39–47. DOI:10.1128/IAI.02230-14
- Farkas B, Boldizsar F, Tarjanyi O, et al. BALB/c mice genetically susceptible to proteoglycan-induced arthritis and spondylitis show colony-dependent differences in disease penetrance. Arthritis Res Ther 2009;11(1):R21), DOI:10.1186/ar2613
- Avila-Calderon ED, Lopez-Merino A, Jain N, et al. Characterization of outer membrane vesicles from Brucella melitensis and protection induced in mice. Clin Dev Immunol 2012;2012:352493.