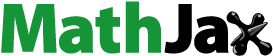
ABSTRACT
In this study, we reported the first long-term monitoring of SARS-CoV-2 in wastewater in Mainland China from November 2021 to October 2023. The city of Shijiazhuang was employed for this case study. We developed a triple reverse transcription droplet digital PCR (RT-ddPCR) method using triple primer-probes for simultaneous detection of the N1 gene, E gene, and Pepper mild mottle virus (PMMoV) to achieve accurate quantification of SARS-CoV-2 RNA in wastewater. Both the RT-ddPCR method and the commercial multiplex reverse transcription quantitative polymerase chain reaction (RT-qPCR) method were implemented for the detection of SARS-CoV-2 in wastewater in Shijiazhuang City over a 24-month period. Results showed that SARS-CoV-2 was detected for the first time in the wastewater of Shijiazhuang City on 10 November 2022. The peak of COVID-19 cases occurred in the middle of December 2022, when the concentration of SARS-CoV-2 in the wastewater was highest. The trend of virus concentration increases and decreases forming a “long-tailed” shape in the COVID-19 outbreak and recession cycle. The results indicated that both multiplex RT-ddPCR and RT-qPCR are effective in detecting SARS-CoV-2 in wastewater, but RT-ddPCR is capable of detecting low concentrations of SARS-CoV-2 in wastewater which is more efficient. The SARS-CoV-2 abundance in wastewater is correlated to clinical data, outlining the public health utility of this work.
First long-term monitoring of SARS-CoV-2 in wastewater in Mainland China
COVID-19 outbreak was tracked in Shijiazhuang City from outbreak to containment
Wastewater was monitored simultaneously using RT-ddPCR and RT-qPCR methods
Triple primer-probe RT-ddPCR detects N1 and E genes of SARS-CoV-2 and PMMoV
Highlights
Introduction
Wastewater-based surveillance (WBS) is a highly effective tool for early detection and caseload assessment of communicable diseases. One of the biggest challenges regarding infectious diseases is its unpredictability. WBS provides a complementary tool, and sometimes primary for early warning of outbreak and assessment of disease prevalence independent of clinical diagnosis. This was particularly evident during the COVID-19 pandemic. Monitoring for SARS-CoV-2 RNA in wastewater has shown great potential for detecting the virus before cases are noticed [Citation1–4]. WBS can provide crucial information, even in areas with low infection rates, making it an essential component of public health surveillance strategies [Citation5–8]. Reported COVID-19 cases usually cannot reflect the magnitude of infections in the community, primarily due to the large proportion of asymptomatic and mildly symptomatic infections [Citation9].
Previous studies have shown that SARS-CoV-2 RNA can be detected in stool samples from COVID-19 positive patients and asymptomatic carriers [Citation10,Citation11]. SARS-CoV-2 was detected in wastewater four to ten days earlier than by clinical case reporting [Citation12]. China employed wastewater monitoring of SARS-CoV-2 in early June 2020 in Hong Kong using one-step RT-qPCR. The implementation of the Zero-COVID strategy in mainland China made the need for wastewater monitoring of SARS-CoV-2 less urgent. However, with the discovery of the Omicron variant in South Africa in 2021, Shijiazhuang initiated the wastewater monitoring of SARS-CoV-2 in November 2021. This was the earliest surveillance and the first long-term surveillance established in mainland China.
Optimizing RT-ddPCR for the concurrent detection of SARS-CoV-2 N1, E genes, and the PMMoV can enhance both accuracy and precision within wastewater analyses. The N1 and E genes serve as critical marker genes for the SARS-CoV-2 virus, ensuring reliable detection even if one of the genes encounters interference, as the positive identification of the alternate gene furnishes robust evidence of the virus's presence. Concurrently, by screening for PMMoV, which is predominantly found in faecal matter, the process confirms the adequacy of human faecal content in samples, thus corroborating their integrity and bolstering the credibility of the test outcomes. Evidence suggests PMMoV's noteworthy stability in various environmental water conditions and its concentration spikes correlating with overall faecal pollution surges [Citation13–15], thus advocating its use for consistent accuracy and method efficacy. Contrarily, separating the assays for SARS-CoV-2's N1, E genes, and PMMoV could lead to increased reagent expenditure and extended analysis duration. As such, the RT-ddPCR assay can be refined through a triple primer-probe tactic, economizing on reagents and expediting the process.
The aim of this study was to optimize a quantitative RT-ddPCR method for monitoring the abundance of SARS-CoV-2 in wastewater in Shijiazhuang City. The proposed method was used in conjunction with a widely used SARS-CoV-2 RT-qPCR detection method to obtain and compare data from November 2021 to October 2023.
Materials and methods
Characteristics of the city of Shijiazhuang and Qiaodong wastewater treatment plant
The city of Shijiazhuang is the capital of Hebei province, with an urban population of 5.75 million. Qiaodong wastewater treatment plant is located south-east of Shijiazhuang and services over 1.5 million people, or approximately 26.09% of the urban population. The treatment capacity of the wastewater treatment plant is 510,000 tons/day. It is designed and operated as a modern wastewater treatment facility.
SARS-CoV-2 standards and other virus standards
SARS-CoV-2 in vitro transcribed nucleic acid standards, purchased from the China Academy of Metrology, include a high-concentration standard (Catalog No. GBW(E)091089) and a low-concentration standard (Catalog No. GBW(E)091090). These RNA transcript standards were synthesized through in vitro transcription of critical characteristic genes of the novel coronavirus: the full-length nucleocapsid protein (N) gene, the full-length envelope protein (E) gene, and a gene fragment of the open reading frame 1ab (ORF1ab) (spanning genome coordinates 13,201–15,600). The actual concentration of Catalog No. GBW(E)091089 for the N gene is 7.00 × 108 copies/mL and for the E gene is 5.03 × 108 copies/mL. The actual concentration of Catalog No. GBW(E)091090 for the N gene is 6.36 × 105 copies/mL, and for the E gene, it is 4.57 × 105 copies/mL. Positive control standards for viruses including sapovirus (SaV), astrovirus (AstV), echovirus (ECHO), enteric adenovirus (HAdV), norovirus (NoV), and rotavirus (RV) were sourced from the laboratory of the Hebei Provincial Center for Disease Control and Prevention. A SARS-CoV-2 pseudovirus (Catalog No. M591001-0001) comprising ORF1ab, E, and N genes was procured at a concentration of 6.22 × 108 copies/mL from Sangon Biotech (Shanghai) Co., Ltd.
Wastewater sampling
The HACH-AS950 Controller (HACH, Loveland, USA) was used to collect 24-hour composite wastewater samples. Sampling of the inlet of municipal wastewater in Shijiazhuang was conducted. To ensure temporal consistency in sampling, a 500 mL aliquot of wastewater is collected at 9.00am on each designated sampling date. A total of 130 wastewater samples were collected in this study between November 2021 and October 2023. For the first 11 months of the study, between November 2021 and October 2022, 66 samples were collected every five to seven days at the Qiaodong wastewater treatment plant. The other 64 samples were collected every two to seven days from November 2022 to October 2023.
Pre-treatment of wastewater and RNA extraction
First, the wastewater samples were inactivated by heating the sample at 60°C for 10 min. After mixing, take a 150 mL wastewater sample and centrifuge it at high speed (10,000 g) for 10 min at 4°C to pelletize bacteria and large particle sediment. Collect the supernatant. To enhance the adsorption on Cellulose Nitrate Filter Membranes (ACN, 0.45 μM, Sartorius), add 2.5 M MgCl2 to achieve a final concentration of 25 mM/L in the wastewater prior to filtration. Adjust the pH of the wastewater to 3.5 with 0.5 mol/L HCl. Then, pour the samples into a Lafil 400-SF 10 Vacuum Filtration System (Rocker, Taiwan, China), filter the samples, and subsequently remove and dissect the filters. Place the filter pieces into 3 mL of MagMAX™ Lysis Buffer eluate (Thermo Fisher Scientific, Massachusetts, USA) with magnetic beads. Vigorously shake for 15 min, extract the supernatant, and use a fully automated nucleic acid extractor SSNP-9600A (Jiangsu ShuoShi Biological Co., Ltd.) to extract RNA. Immediately test or store samples at −80°C. The schematic representation of the wastewater treatment process is illustrated in Supplementary Figure 1.
RT-ddPCR assay
We developed and optimized a one-step triplex RT-ddPCR method for the detection of N1, E and PMMoV. Several gene targets that are specific to SARS-CoV-2, including RNA-dependent RNA polymerase (RdRP), nucleocapsid (N1, N2), envelope protein (E), spike glycoprotein (S), membrane protein (M) and ORF1ab gene, have been used for wastewater monitoring. The primers and probe sequences used in this study were selected from the nucleocapsid protein 1 (N1) [Citation16] and envelope protein (E) genes [Citation17] designed by Centers for Disease Control and Prevention of United States(CDC) and Charité Institute of Virology, Berlin, Germany, with the aim of specifically detecting SARS-CoV-2. Primers and probes for the N1 and E gene regions were sensitive and specific for quantifying SARS-CoV-2 RNA in wastewater [Citation17–19]. But SARS-CoV-2 is a single strand RNA virus that mutates very quickly. Based on viral whole genome sequencing analysis in Hebei Province, China, we found that there were several essential single nucleotide variations (SNVs) on primer-probe regions accumulating within same Omicron Variants’ genomes. Two SNVs, T28297C, C28311T emerging on 2019-nCoV-N1 (CDC-N1) primer-probe regions, recommended by US CDC in 2020, and two SNVs, C26270T, A26275G emerging on E (Charité-E) primer-probe regions recommended by Charité, Germany. Moreover, these SNVs are stable for a long time, so update the N1 and E primer probes in time. All primers and probes were synthesized by a commercial company (Generay Biotech, Shanghai, China) (). We prepared the reaction system using the One-step RT-ddPCR Advanced Kit for Probes 200 rxns (Bio-Rad, CA, USA) kit. First, 5 μL of Supermix, 2 μL of reverse transcriptase, 1 μL of DTT (300 mmol/L), 4 μL of RNA template, and optimized primers and probes in the final concentration to a 20 μL reaction volume were added. The sealed 96-well PCR plate was placed in a C1000 Touch™ Thermal Cycler for PCR reaction and operated under the following thermal cycling conditions: reverse transcription at 45°C for 60 min, enzyme activation at 95°C for 10 min, DNA denaturation at 94°C for 30 s, annealing and extension at 55–65°C for 60 s, enzyme inactivation at 98°C for 10 min, and finally incubation at 4°C. The 96-well PCR plate amplified by thermal cycling was transferred to the QX200TM Droplet Reader for reading. After reading, we quantified the target amplification product using QuantaSoft™ Analysis Pro software.
Table 1. Forward primers, reverse primers, and probes of the assay.
RT-qPCR assay
We employed RT-qPCR, the most widely used method in China, at the same time to verify the feasibility of RT-qPCR in wastewater viral surveillance. The RT-qPCR test uses the Novel coronavirus (2019-nCoV) nucleic acid detection kit (fluorescent PCR method) (BioGerm, Shanghai, China), which is well-known and recommended by Chinese Center for Disease Control and Prevention (China CDC) and has high sensitivity with a limit of detection of 250 copies/mL. The overall reaction system was 40 μL, containing 12 μL of nucleic acid amplification reaction solution, 4 μL of enzyme mixture, 4 μL of ORF1ab/N reaction solution, and 20 μL of the tested sample nucleic acid. The reaction conditions were 50°C for 10 min, 97°C for 1 min, 45 cycles of 5 s at 97°C, and 30 s at 58°C, collecting fluorescence. The RT-qPCR results were analysed using QuantStudio 7 Flex.
Validation test
The accuracy and specificity of the RT-ddPCR method were assessed utilizing SARS-CoV-2 reference material. Replicating each assay three times allowed for a comparative analysis between the detection outcomes and the expected theoretical values. The analytical specificity of the RT-ddPCR assay was also evaluated for various key wastewater viruses, including SaV, AtV, ECHO, HAdV, NV, RV, alongside the N1, E, and PMMoV genes, using positive virus standard solutions. Furthermore, the sensitivity of the RT-ddPCR method was determined by performing serial dilutions of SARS-CoV-2 RNA and PMMoV RNA templates.
SARS-CoV-2 recovery efficiency
RT-ddPCR was firstly used to detect wastewater samples to get the concentration of SARS-CoV-2 as a background value. Three bottles of 100 mL wastewater and three bottles of 100 mL tap water were added with 10 μL of SARS-CoV-2 pseudovirus with a concentration of 6.22 × 107 copies/mL, respectively. Subsequently, wastewater and tap water were concentrated and extracted according to the pre-processing method of this study. The wastewater and tap water recovery efficiency (mean and standard deviation) was calculated based on the number of copies quantified by RT-ddPCR. The equation for the calculations is as follows:
Detection of SARS-CoV-2 in wastewater
RT-ddPCR method was used to directly quantify SARS-CoV-2 RNA, yielding results in copies per millilitre. Concurrently, RT-qPCR analyses were performed to obtain Cycle Threshold (Ct) values for each specimen. When Ct > 38, it was negative. Each wastewater underwent triplicate assays to ensure consistency and accuracy, and the result is determined when all three tests produce identical outcomes.
Statistical analysis
All statistical analyses were conducted using the SPSS software (IBM SPSS Statistics 26.0). The Cohen's Kappa consistency test and paired χ2 test (McNemar test) were used to compare the consistency of qualitative results obtained by RT-ddPCR and RT-qPCR, and to determine whether there was a difference in the detection results between the two methods. The Pearson Correlation Analysis was conducted to evaluate whether the WBS results were correlated with the temporal trend of Positive rate of COVID-19 in sentinel hospitals published by China CDC [Citation20]. Linear Regression to model the relationship between positive rate of COVID-19 in sentinel hospitals in China and SARS-CoV-2 concentrations detected in wastewater.
Results
Development and validation of RT-ddPCR assays
Optimization of the RT-ddPCR assay
To determine the optimal annealing temperature, evaluation was performed under a temperature gradient of 57 to 62°C, and the highest number of positive droplets and best separation in the 2D graph were obtained at 57°C (Supplementary Figure 2). Next, the terminal concentration of the primer was optimized within the range of 450–1800nmol/L, and the primer concentration was found to be optimal at 900 nM (Supplementary Figure 3). The probe terminal concentration was optimized within the range of 125–500 nM, and positive and negative droplets were clearly separated at 250 nM (Supplementary Figure 4). Finally, the annealing extension time was optimized for 60, 90, and 120 s, and it was found that the longer the annealing extension time, the better the clustering of positive and negative droplets. The optimal annealing extension time was determined to be 120 s (Supplementary Figure 5). The experimental results showed that the optimal annealing temperature was 57°C, and the final concentrations of primer and probe were 900 and 250 nM, respectively, with an annealing extension time of 120 s. As detailed in Supplementary Figure 5, under the optimized conditions, the clusters were separated clearly with few droplets, and satisfactory results were obtained. The formation of the optimized RT-ddPCR system and programme are detailed in .
Table 2. RT-ddPCR reaction mix preparation and thermal cycling condition.
Considering that the primer-probe concentration, annealing temperature, and annealing extension time in RT-ddPCR multiplex detection can affect amplification and separation efficiency, we optimized the reaction system and programme. Based on experimental results, we ultimately selected 900 nM–250 nM as the appropriate primer-probes concentration, 57°C as the suitable annealing temperature, and 120 s as the appropriate annealing extension time. Under these conditions, all genes detected in this study obtained satisfactory results with good performance features in terms of accuracy, sensitivity, specificity, and reproducibility, even at very low virus concentrations.
Accuracy and sensitivity of RT-ddPCR method
The accuracy of the experiment was repeated three times, and the average detection value of N1 was 5.6 × 105 copies/mL, with a deviation of 0.91% from the theoretical detection value of standard sample N1. The average detection value of E was 4.0 × 105 copies/mL, with a deviation of 3.84% from the theoretical detection value of standard sample E. The results show that the actual detection values of RT-ddPCR are consistent with the theoretical values of the positive standard material for the new coronavirus (high concentration) GBW(E)091089 standard samples N1 and E.
An evaluation of the analytical specificity for other important viruses in the wastewater samples, in addition to SARS-CoV-2, was conducted. The results showed that positive droplets were obtained using N1, E, and PMMoV gene RNA as templates, while no more than three positive droplets were observed for other viruses (Supplementary Figure 6). In fact, wells with no more than three droplets can be considered negative results due to instrument error. This was indicative of the assay having a high specificity for detecting SARS-CoV-2 in wastewater.
Based on the experimental results, the N1 detection limit of RT-ddPCR was determined to be 159 copies/mL, and the E detection limit was 228 copies/mL. Furthermore, the measured value of RT-qPCR was always lower than that of RT-ddPCR at low concentrations of simulated wastewater samples. When the measurement value of RT-ddPCR is lower than 190 copies/mL, the result of RT-qPCR is negative (Supplementary Figure 7).
Viral RNA recovery efficiency
By performing three replicate tests at different times, the SARS-CoV-2 recovery efficiency was determined to be 21.7%± 4.3% in wastewater and 36.3%± 2.7%
in tap water.
Surveillance and warning effect of wastewater
Detection of SARS-CoV-2 in wastewater
A total of 130 wastewater samples were collected from Qiaodong wastewater treatment plant serving Shijiazhuang’s south-east region from November 2021 to October 2023. Through RT-ddPCR 62 wastewater samples were detected for SARS-CoV-2 positive, while through RT-qPCR 61 samples were detected for positive. The developed SARS-CoV-2 RT-ddPCR method had similar diagnostic performance to the RT-qPCR method for actual wastewater samples ().
Table 3. RT-ddPCR and RT-qPCR McNemar test and Cohen’s Kappa statistics.
The Ct values of N gene detected by RT-qPCR in 61 positive samples were between 28.193 and 36.793, and the Ct values of ORF1ab gene were between 27.940 and 37.483. The concentration of N1 gene in 62 positive samples detected by RT-ddPCR ranged from 625 to 23,900 copies/mL, and the concentration of E gene ranged from 228 to 17,800 copies/mL (Supplementary Table 1).
In this study, a “long-tailed” pattern emerged (), suggesting continued low-level community prevalence following the initial COVID-19 wave. A significant upsurge in SARS-CoV-2 concentration in wastewater was detected after a hiatus exceeding five months, with a peak recorded on 24 May 2023. Nonetheless, the second wave's peak concentration was less pronounced than the first.
Figure 1. (a) The temporal distribution of SARS-CoV-2 in wastewater using RT-ddPCR during the COVID-19 epidemic from November 2021 to October 2023. (b) The temporal distribution of SARS-CoV-2 in wastewater Since China's Zero-COVID strategy ends from November 2022 to October 2023. And positive rate of COVID-19 in sentinel hospitals in China (%) published by China CDC.

Early warning effect of wastewater
The monitoring results of urban wastewater showed that the SARS-CoV-2 was detected for the first time in the wastewater of Shijiazhuang City in China in November 2022, after China announced optimized measures for COVID-19 prevention and control. On 10 November 2022, the concentration of N1 gene was 625 copies/mL and that of E gene was 228 copies/mL as detected by RT-ddPCR. On 11 November 2022, the Ct values of N and ORF1ab genes were 36.075 and 36.819, respectively, as detected by RT-qPCR. Subsequently, the concentration of the SARS-CoV-2 gradually increased and peaked around 15 December 2022 ((a)), which allowed for early prediction of the outbreak of the epidemic in Shijiazhuang. We also found a strong correlation between weekly wastewater data (averaged over a week of wastewater monitoring data) and positive rate of COVID-19 in sentinel hospitals in China. Wastewater SARS-CoV-2 RNA levels mirrored the positive COVID-19 trends reported by sentinel hospitals in China ((b)). The Pearson correlation coefficient between positive rate of COVID-19 in sentinel hospitals with N1 and E from wastewater was r = 0.633, P < 0.001 and r = 0.697, P < 0.001, respectively. And with N and ORF1ab from wastewater was r = 0.628, P < 0.001 and r = 0.631, P < 0.001, respectively. Linear Regression obtained R2 Linear (N1) = 0.401, R2 Linear (E) = 0.462 ((a)). R2 Linear (N) = 0.395, R2 Linear (ORF1ab) = 0.399 ((b)).
Figure 2. (a) Linear regression to model the relationship between positive rate of COVID-19 in sentinel hospitals in China and concentration of SARS-CoV-2 in wastewater. R2 Linear (N1) = 0.401, R2 Linear (E) = 0.462. (b) Linear regression to model the relationship between positive rate of COVID-19 in sentinel hospitals in China and SARS-CoV-2 N/ORF1ab cycle threshold in wastewater. R2 Linear (N) = 0.395, R2 Linear (ORF1ab) = 0.399.

Integrating wastewater data with other epidemiological indicators, such as case reports and “Baidu Trends,” (Supplementary Figure 8) can enhance public health responses by providing a multifaceted view of community transmission dynamics.
Discussion
WBS has shown great utility in estimating transmission and extent of COVID-19 in the post-epidemic era. It increases the ability to monitor populations that do not have access to healthcare. Since wastewater can provide mixed samples from the entire community and is easier to obtain compared to collecting clinical samples individually, screening and testing wastewater can more comprehensively estimate the level of infection in densely populated areas and help control the spread of epidemics. The most widely used quantitative method for SARS-CoV-2 detection in wastewater is reverse transcription quantitative polymerase chain reaction (RT-qPCR) [Citation21]. However, the complexity of the environmental sample matrix poses challenges. The RT-qPCR method requires a standard curve for quantification, and there may be PCR inhibition issues in complex matrices [Citation22,Citation23]. RT-ddPCR offers a compelling approach for the detection of SARS-CoV-2 in wastewater, owing to its exceptional consistency and the ability to provide absolute quantification without the reliance on a standard curve [Citation24]. Accumulating evidence suggests an increased detection rate of SARS-CoV-2 RNA in clinical specimens when utilizing RT-ddPCR over other methods [Citation25–27]. Notably, in this study during the second week of November 2022, RT-ddPCR yielded positive detections, in contrast to the negative or marginally negative results (Cycle Threshold values ranging between 36 and 40) observed with RT-qPCR. The quantification of the N1 gene by RT-ddPCR span from 625 to 1615 copies/mL, and the E gene was detected at levels ranging from 228 to 282 copies/mL.
To compare the sensitivity between RT-ddPCR and RT-qPCR, we added a small amount of reference materials to wastewater samples and then tested them using both methods. The results demonstrated that RT-ddPCR was capable of detecting samples that yielded negative results with RT-qPCR (Supplementary Figure 7).
In this study, the sensitivity of RT-ddPCR method was lower than in our previous work detecting for Norovirus and Hepatitis A Virus [Citation28], likely due to the one-step method we used in this study. Previously, Racˇki et al. found that the degree of inhibition of one-step RT-ddPCR amplification of RNA by undiluted raw wastewater is the same as that of RT-qPCR determination [Citation29].
Over the past three years, China’s Zero-COVID policy protected the health and lives of the country’s 1.4 billion people. After the removal of all Zero-COVID measures, a large portion of the population became infected over a very short period. In this study, SARS-CoV-2 RNA was detected in samples of wastewater before any cases were reported, suggesting that virus monitoring can provide an early warning for outbreak in a population before an outbreak is clinically apparent. The monitoring results showed that the SARS-CoV-2 was first detected in the city's wastewater on 10 November 2022. After that, a dramatically increased SARS-CoV-2 concentration was observed with rapid increase in local clinical cases reported. Moreover, it can be seen from (b) that the epidemic trend of wastewater monitoring is earlier than that reported by the Positive rate of COVID-19 in sentinel hospitals. And by using Linear Regression to model the quantitative relationship between Positive rate of COVID-19 in sentinel hospitals in China and Concentration of SARS-CoV-2 in wastewater and draw scatter plot to get fitting equation. (a,b) shows that RT-ddPCR has a higher coefficient of determination R² Linear (N1, E) compared to RT-qPCR, indicating that RT-ddPCR exhibits superior linearity in gene expression detection and ensures more accurate quantitative results. This also highlights the efficacy of wastewater surveillance in predicting the COVID-19 positivity rate. Additionally, Pearson Correlation Analysis revealed that RT-ddPCR yields a higher Pearson correlation coefficient, further supporting its robustness in monitoring trends.
Prior studies have established a clear link between the 14-day COVID-19 incidence in sewer catchments and the detection of SARS-CoV-2 RNA in influent wastewater [Citation30]. An incidence rate of 7 cases per 100,000 over two weeks is associated with a 50% likelihood of SARS-CoV-2 detection in wastewater, increasing to 95% at an incidence rate of 40 cases per 100,000. Consistent correlations between wastewater viral RNA and COVID-19 cases have been observed globally, including in Mexico, the United States, Argentina, and Canada [Citation31–33]. Despite potential variability factors, the positive rate of COVID-19 aligns with rising SARS-CoV-2 RNA concentrations in wastewater, serving as a robust pandemic-monitoring tool.
There are still various challenges such as how to detect new variants early in monitoring [Citation34], false negatives caused by low virus recovery rates in wastewater, and difficulties in fully interpreting the data obtained [Citation35]. Currently, research results show that the recovery rate of SARS-CoV-2 in wastewater is low [Citation36,Citation37]. Our own experiments on recovery efficiency suggest that impurities may adsorb viruses, complicating the detection of already minute viral concentrations within expansive and complex wastewater systems. In addition to improving the sensitivity of detection methods, it is also necessary to optimize the wastewater pretreatment method to reduce false negative results caused by virus loss during the treatment process. Further research is needed to identify the key factors that impact the recovery of SARS-CoV-2 in wastewater.
Conclusions
A 24-months long surveillance carried out in wastewater provided a perspective of the COVID-19 outbreak and mitigation in the capital city of Shijiazhuang in the Hebei province. It is the first long-term wastewater monitoring spanning the entirety of the Zero-COVID strategy implemented at the height of the COVID-19 pandemic and post-epidemic era. Our study compares the RT-ddPCR method with the RT-qPCR detection, which is currently the most widely used technique in the China CDC system, for measuring SARS-CoV-2 concentrations in wastewater samples. The results confirm and verify that long-term wastewater monitoring of SARS-CoV-2 is a useful tool for predicting, monitoring, and controlling future COVID-19 outbreaks.
Supplementary_material
Download MS Word (3 MB)Highlights
Download MS Word (15.9 KB)Declaration
Download MS Word (13.2 KB)Supplementary_Figures
Download Zip (29.2 MB)Acknowledgements
We thank LetPub (www.letpub.com) for linguistic assistance and pre-submission expert review.
Disclosure statement
No potential conflict of interest was reported by the author(s).
Additional information
Funding
References
- Ahmed W, Tscharke B, Bertsch PM, et al. SARS-CoV-2 RNA monitoring in wastewater as a potential early warning system for COVID-19 transmission in the community: a temporal case study. Sci Total Environ. 2021 Mar 20;761:144216. doi:10.1016/j.scitotenv.2020.144216
- Claro ICM, Cabral AD, Augusto MR, et al. Long-term monitoring of SARS-COV-2 RNA in wastewater in Brazil: a more responsive and economical approach. Water Res. 2021 Sep 15;203:117534. doi:10.1016/j.watres.2021.117534
- Li X, Zhang S, Sherchan S, et al. Correlation between SARS-CoV-2 RNA concentration in wastewater and COVID-19 cases in community: a systematic review and meta-analysis. J Hazard Mater. 2023 Jan 5;441:129848. doi:10.1016/j.jhazmat.2022.129848
- Zheng X, Li S, Deng Y, et al. Quantification of SARS-CoV-2 RNA in wastewater treatment plants mirrors the pandemic trend in Hong Kong. Sci Total Environ. 2022 Oct 20;844:157121. doi:10.1016/j.scitotenv.2022.157121
- Randazzo W, Truchado P, Cuevas-Ferrando E, et al. SARS-CoV-2 RNA in wastewater anticipated COVID-19 occurrence in a low prevalence area. Water Res. 2020 Aug 15;181:115942. doi:10.1016/j.watres.2020.115942
- Gonçalves J, Koritnik T, Mioč V, et al. Detection of SARS-CoV-2 RNA in hospital wastewater from a low COVID-19 disease prevalence area. Sci Total Environ. 2021 Feb 10;755(Pt 2):143226. doi:10.1016/j.scitotenv.2020.143226
- Ou G, Tang Y, Niu S, et al. Wastewater surveillance and an automated robot: effectively tracking SARS-CoV-2 transmission in the post-epidemic era. Natl Sci Rev. 2023 Jun;10(6):nwad089.
- Bivins A, North D, Ahmad A, et al. Wastewater-based epidemiology: global collaborative to maximize contributions in the fight against COVID-19. Environ Sci Technol. 2020 Jul 7;54(13):7754–7757. doi:10.1021/acs.est.0c02388
- Li R, Pei S, Chen B, et al. Substantial undocumented infection facilitates the rapid dissemination of novel coronavirus (SARS-CoV-2). Science. 2020;368(6490):489–493. doi:10.1126/science.abb3221
- He X, Lau EHY, Wu P, et al. Temporal dynamics in viral shedding and transmissibility of COVID-19. Nat Med. 2020 May;26(5):672–675. doi:10.1038/s41591-020-0869-5
- Young BE, Ong SWX, Kalimuddin S, et al. Epidemiologic features and clinical course of patients infected With SARS-CoV-2 in Singapore. Jama. 2020 Apr 21;323(15):1488–1494. doi:10.1001/jama.2020.3204
- Wu F, Xiao A, Zhang J, et al. SARS-CoV-2 RNA concentrations in wastewater foreshadow dynamics and clinical presentation of new COVID-19 cases. Sci Total Environ. 2022 Jan 20;805:150121. doi:10.1016/j.scitotenv.2021.150121
- Hamza IA, Jurzik L, Uberla K, et al. Evaluation of pepper mild mottle virus, human picobirnavirus and torque teno virus as indicators of fecal contamination in river water. Water Res. 2011 Jan;45(3):1358–1368. doi:10.1016/j.watres.2010.10.021
- Rosario K, Symonds EM, Sinigalliano C, et al. Pepper mild mottle virus as an indicator of fecal pollution. Appl Environ Microbiol. 2009 Nov;75(22):7261–7267. doi:10.1128/AEM.00410-09
- Rachmadi AT, Kitajima M, Pepper IL, et al. Enteric and indicator virus removal by surface flow wetlands. Sci Total Environ. 2016 Jan 15;542(Pt A):976–982. doi:10.1016/j.scitotenv.2015.11.001
- Lu X, Wang L, Sakthivel SK, et al. US CDC real-time reverse transcription PCR panel for detection of severe acute respiratory syndrome coronavirus 2. Emerg Infect Dis. 2020 Aug;26(8):1654–1665. doi:10.3201/eid2608.201246
- Corman VM, Landt O, Kaiser M, et al. Detection of 2019 novel coronavirus (2019-nCoV) by real-time RT-PCR. Euro Surveill. 2020;25(3):2000045. doi:10.2807/1560-7917.ES.2020.25.3.2000045
- Hamouda M, Mustafa F, Maraqa M, et al. Wastewater surveillance for SARS-CoV-2: lessons learnt from recent studies to define future applications. Sci Total Environ. 2021 Mar 10;759:143493. doi:10.1016/j.scitotenv.2020.143493
- Ho J, Stange C, Suhrborg R, et al. SARS-CoV-2 wastewater surveillance in Germany: long-term RT-digital droplet PCR monitoring, suitability of primer/probe combinations and biomarker stability. Water Res. 2022 Feb 15;210:117977. doi:10.1016/j.watres.2021.117977
- Epidemic situation of COVID-19 infection in China. Available from: https://www.chinacdc.cn/jkzt/crb/
- Flood MT, D'Souza N, Rose JB, et al. Methods evaluation for rapid concentration and quantification of SARS-CoV-2 in raw wastewater using droplet digital and quantitative RT-PCR. Food Environ Virol. 2021 Sep;13(3):303–315. doi:10.1007/s12560-021-09488-8
- Ahmed W, Simpson SL, Bertsch PM, et al. Minimizing errors in RT-PCR detection and quantification of SARS-CoV-2 RNA for wastewater surveillance. Sci Total Environ. 2022 Jan 20;805:149877. doi:10.1016/j.scitotenv.2021.149877
- Ciesielski M, Blackwood D, Clerkin T, et al. Assessing sensitivity and reproducibility of RT-ddPCR and RT-qPCR for the quantification of SARS-CoV-2 in wastewater. J Virol Methods. 2021 Nov;297:114230. doi:10.1016/j.jviromet.2021.114230
- Ahmed W, Bivins A, Metcalfe S, et al. Evaluation of process limit of detection and quantification variation of SARS-CoV-2 RT-qPCR and RT-dPCR assays for wastewater surveillance. Water Res. 2022 Feb 3;213:118132. doi:10.1016/j.watres.2022.118132
- D'Aoust PM, Mercier E, Montpetit D, et al. Quantitative analysis of SARS-CoV-2 RNA from wastewater solids in communities with low COVID-19 incidence and prevalence. Water Res. 2021 Jan 1;188:116560. doi:10.1016/j.watres.2020.116560
- Strati A, Zavridou M, Paraskevis D, et al. Development and analytical validation of a one-step five-plex RT-ddPCR assay for the quantification of SARS-CoV-2 transcripts in clinical samples. Anal Chem. 2022 Sep 13;94(36):12314–12322. doi:10.1021/acs.analchem.2c00868
- Tedim AP, Almansa R, Domínguez-Gil M, et al. Comparison of real-time and droplet digital PCR to detect and quantify SARS-CoV-2 RNA in plasma. Eur J Clin Invest. 2021 Jun;51(6):e13501. doi:10.1111/eci.13501
- Han Y, Wang J, Zhang S, et al. Simultaneous quantification of hepatitis A virus and norovirus genogroup I and II by triplex droplet digital PCR. Food Microbiol. 2022 May;103:103933. doi:10.1016/j.fm.2021.103933
- Rački N, Dreo T, Gutierrez-Aguirre I, et al. Reverse transcriptase droplet digital PCR shows high resilience to PCR inhibitors from plant, soil and water samples. Plant Methods. 2014;10(1):42. doi:10.1186/s13007-014-0042-6
- Tiwari A, Lipponen A, Hokajärvi AM, et al. Detection and quantification of SARS-CoV-2 RNA in wastewater influent in relation to reported COVID-19 incidence in Finland. Water Res. 2022 May 15;215:118220. doi:10.1016/j.watres.2022.118220
- Carrillo-Reyes J, Barragán-Trinidad M, Buitrón G. Surveillance of SARS-CoV-2 in sewage and wastewater treatment plants in Mexico. J Water Process Eng. 2021 Apr;40:101815. doi:10.1016/j.jwpe.2020.101815
- D'Aoust PM, Graber TE, Mercier E, et al. Catching a resurgence: increase in SARS-CoV-2 viral RNA identified in wastewater 48 h before COVID-19 clinical tests and 96 h before hospitalizations. Sci Total Environ. 2021 May 20;770:145319. doi:10.1016/j.scitotenv.2021.145319
- Giraud-Billoud M, Cuervo P, Altamirano JC, et al. Monitoring of SARS-CoV-2 RNA in wastewater as an epidemiological surveillance tool in Mendoza, Argentina. Sci Total Environ. 2021 Nov 20;796:148887. doi:10.1016/j.scitotenv.2021.148887
- Karthikeyan S, Levy JI, De Hoff P, et al. Wastewater sequencing reveals early cryptic SARS-CoV-2 variant transmission. Nature. 2022 Sep;609(7925):101–108. doi:10.1038/s41586-022-05049-6
- Du Toit A. Sewer biofilms and SARS-CoV-2. Nat Rev Microbiol. 2023 May;21(5):277.
- Vincent-Hubert F, Wacrenier C, Desdouits M, et al. Development of passive samplers for the detection of SARS-CoV-2 in sewage and seawater: application for the monitoring of sewage. Sci Total Environ. 2022 Aug 10;833:155139. doi:10.1016/j.scitotenv.2022.155139
- Kevill JL, Lambert-Slosarska K, Pellett C, et al. Assessment of two types of passive sampler for the efficient recovery of SARS-CoV-2 and other viruses from wastewater. Sci Total Environ. 2022 Sep 10;838(Pt 4):156580. doi:10.1016/j.scitotenv.2022.156580