ABSTRACT
Vaginal transmission from semen of male Ebola virus (EBOV) survivors has been implicated as a potential origin of Ebola virus disease (EVD) outbreaks. While EBOV in semen must traverse cervicovaginal mucus (CVM) to reach target cells, the behaviour of EBOV in CVM is poorly understood. CVM contains substantial quantities of IgG, and arrays of IgG bound to a virion can develop multiple Fc-mucin bonds, immobilizing the IgG/virion complex in mucus. Here, we measured the real-time mobility of fluorescent Ebola virus-like-particles (VLP) in 50 CVM specimens from 17 women, with and without ZMapp, a cocktail of 3 monoclonal IgGs against EBOV. ZMapp-mediated effective trapping of Ebola VLPs in CVM from a subset of women across the menstrual cycle, primarily those with Lactobacillus crispatus dominant microbiota. Our work underscores the influence of the vaginal microbiome on IgG-mucin crosslinking against EBOV and identifies bottlenecks in the sexual transmission of EBOV.
Introduction
Ebola virus (EBOV) causes severe disease, with an estimated case fatality ratio ranging from 50% to 90% [Citation1]. While the major mode of EBOV transmission is direct contact with blood or other bodily fluids, there is increasing evidence implicating the possibility of sexual transmission of EBOV from survivors to their uninfected discordant partners. Indeed, an infectious virus has been isolated from semen up to 119 days post-disease onset after recovery from initial infection [Citation2,Citation3], and EBOV RNA is detectable in semen up to 2 years after the initial disease onset [Citation4,Citation5]. Purported sexual transmission has been reported [Citation6], where the wife fell ill with acute EBOV 199 days after her partner recovered from the initial infection, was found to be positive for EBOV RNA in semen, and no other known exposure. Sexual transmission from survivors has been accepted as the likely transmission route in several post-outbreak EBOV flare-ups [Citation4,Citation7].
To date, male-to-female appears to be the primary route of sexual transmission, with no suspected case of female-to-male sexual transmission. Infectious virus has not been isolated from the vaginal secretions of EBOV survivors, and RNA found only up to 36 days after symptom onset [Citation8,Citation9]. Currently, the WHO recommends that male survivors maintain either abstinence or condom usage for at least one year post-discharge, or until receiving two negative semen tests. However, up to 46% of male survivors report engaging in condomless intercourse within a year of discharge, and receiving positive semen test results appeared to have little impact on safe-sex behaviours [Citation10]. Thus, there remains a need to understand how EBOV behaves in vaginal secretions, and to explore strategies for preventing vaginal transmission of EBOV.
Cervicovaginal mucus (CVM) represents the first line of defence in the female reproductive tract (FRT). CVM not only minimizes physical trauma to the vaginal epithelium during coitus, but also creates a diffusional barrier that limits immediate pathogen contact with the underlying cells [Citation11,Citation12]. Since viruses in semen must traverse CVM to reach and infect the vaginal epithelium, reinforcing the CVM barrier by trapping viruses in CVM can directly reduce the flux of viruses arriving at target cells, allow for more thorough inactivation of viruses prior to contact, or facilitate more effective elimination of viruses via natural mucosal clearance. We previously found that IgG can trap Herpes Simplex Virus (HSV-1) with strong avidity in CVM [Citation13–15] even at sub-neutralizing concentrations, unaffected by the composition of vaginal microbiota or menstrual cycle phase [Citation16], and afforded effective protection against vaginal HSV-1 transmission in mice [Citation14]. Here, using high-resolution multiple-particle tracking that allowed us to quantify the real-time mobility of thousands of individual virions, we investigated the behaviour of Ebola VLP in fresh native CVM as well as in CVM supplemented with ZMapp, a cocktail of 3 monoclonal IgG monoclonal antibody (mAb).
Materials and methods
Ethics statement
Written informed consent was obtained from all participants after the nature and potential consequences of the study were explained. All studies were performed according to a protocol as approved by the Institutional Review Board of the University of North Carolina at Chapel Hill (IRB 10-1817).
CVM collection and characterization
CVM was collected and characterized using a self-sampling protocol as previously described [Citation17]. Additional details are provided in supplementary materials.
VLP production and purification
Ebola pseudoviruses were produced by transfecting HEK293T cells with plasmids encoding Gag-mCherry and Zaire strain Ebola glycoproteins, plasmids for which are generously provided by Dr. Suryaram Gummuluru (Department of Microbiology, Boston University School of Medicine) and Dr Ronald N. Harty (Department of Pathobiology, School of Veterinary Medicine, University of Pennsylvania), respectively. The amino acid sequence for the EBOV glycoprotein used corresponds to that in Genbank accession number L11365.1. Details on expression and purification are provided in supplementary materials.
Multiple-particle tracking of Ebola VLPs in CVM
Ebola VLPs (108-109 particles/mL, 1 µl) and Abs (2 µl, to a final concentration of 5 µg/mL), were mixed into 20 µl of pH-neutralized CVM. Samples were incubated for ∼15 min at 37°C prior to microscopy. Particle motion was captured using MetaMorph imaging software, at 66.7 ms temporal resolution. Particle trajectories were obtained using convolutional neural network-based tracking software [Citation18]. Additional details are available in the supplementary materials.
16S rRNA gene amplicon sequencing and analysis
DNA extractions for microbiota analysis were performed as previously described [Citation19,Citation20] on aliquots of the same CVM samples used for microscopy. The method of Fadrosh et al [Citation21] was used to analyse the vaginal microbiota composition and structure. Additional details are available in the supplementary materials.
Statistical analysis
Statistical comparisons were performed using ANOVA with multiple comparisons between matched samples (Tukey's multiple comparison's test or Holm-Sidak corrections) or mixed-effects analysis with multiple comparisons between matched samples using GraphPad Prism version 8.0 (GraphPad Software, La Jolla, CA). Differences were deemed significant at an alpha of 0.05.
Results
Ebola VLPs readily diffuse through fresh human CVM
Few laboratories have access to the Biosafety Level 4 containment required to study wild-type EBOV. Therefore, to investigate EBOV mobility in CVM, we prepared non-infectious virus-like-particles (VLPs) by pseudo-typing fluorescent human immunodeficiency virus type 1 (HIV-1) Gag-mCherry capsid proteins with Zaire strain EBOV glycoprotein, as previously described [Citation15]. These VLPs were mixed with CVM pH-neutralized to mimic the buffering effects of semen. High-resolution video microscopy was performed on 50 individual CVM specimens from 17 unique donors, from which particle trajectories were extracted to quantify the real-time mobility of the EBOV VLPs.
Ebola VLPs displayed rapid motion in CVM, with mobility typically spanning many microns within a 20s video (A, Supp Video S1). Consistent with the rapid mobility, the slope of their geometrically-averaged ensemble mean squared displacement (MSD) vs. timescale on a log–log scale (α) was ∼0.7 (for pure Brownian motion, α = 1, and α approaches 0 as a hindrance of Brownian diffusion increases) (B). Their geometrically-averaged mean diffusivity (Deff) was 0.6 ± 0.6 µm2/s (mean ± SD), only ∼10-fold reduced compared to its theoretical diffusivity in buffer (C). The measured mobility was comparable to those for ∼100 nm polyethylene glycol coated polystyrene (PS-PEG) nanoparticles engineered with exhibit minimal adhesion to mucins [Citation22–24] (supplementary material, Figure S1). In contrast, similar-sized carboxyl-modified polystyrene (PS-COOH) beads were effectively immobilized in the same CVM samples (Supplementary material, Figure S1), as reflected by their ∼300-fold reduced Deff (0.002 ± 0.005 µm2/s) compared to Ebola VLPs (Supplementary material, D). We also compared the measured mobility of Ebola VLPs to those of viral-like particles pseudotyped with HIV Env; both exhibited similar diffusivities in matched CVM samples (Supplementary material, F). These results indicate Ebola VLPs can effectively evade adhesion to mucins and undergo rapid diffusion through the interstitial fluids between mucins, similar to other sexually transmitted viruses [Citation16,Citation17,Citation25,Citation26].
Figure 1. Diffusion of Ebola VLPs in pH-neutralized human CVM that is untreated or treated with ZMapp. (A) Representative traces with effective diffusivities within 1 standard error of the mean of the ensemble average at a time scale of 0.2667 s (B) Ensemble-averaged geometric mean square displacements (MSD) as a function of timescale. Statistical significance is evaluated by two-way repeated measures ANOVA with correction by Holm-Šidák test. (C) Ensemble-averaged geometric Deff at a timescale of 0.2667 s for individual CVM specimens, with geometric mean and standard deviation indicated by solid lines. Statistical significance is evaluated by repeated measures mixed effects analysis with correction by Holm-Šidák test on log-transformed data. (D) Fraction of fast-moving particles (average 〈Deff〉 ≥ 0.347 µm2/s, capable of crossing a 50 µm mucus barrier within 1 h) with geometric mean and standard deviation indicated by solid lines. Evaluated by repeated measures mixed-effects analysis followed by Holm-Šidák test. (E) Estimated time for 50% and (F) 10% of pseudovirus particles to diffuse through a 50 µm layer. Data represent the ensemble-averaged mean of samples in each treatment. Error bars represent the standard error of the mean. Evaluated by repeated measures mixed-effect analysis on log-transformed data with correction by Holm-Šidák test (****p < 0.0001).
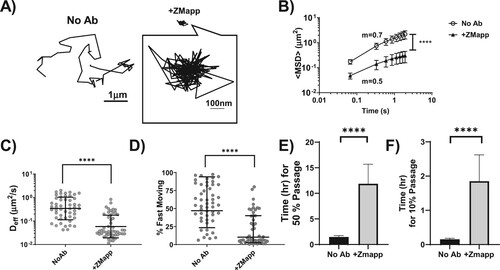
ZMapp markedly reduces the mobility of Ebola VLPs in CVM
We next evaluated whether virion-binding IgG can hinder Ebola VLP diffusion in CVM. Since CVM from individuals vaccinated against Ebola is not readily available, we utilized ZMapp as a surrogate for Ebola-binding IgG, at a final concentration of 5 µg/mL in CVM. We observed substantial heterogeneity in virion mobility in the presence of ZMapp. In some CVM, Ebola VLPs were nearly uniformly immobilized (A; Supplementary Video S2); in other specimens, there were both immobilized particles and diffusive particles in the same videos (Supplementary Video S3). ZMapp reduced the 〈Deff〉 of Ebola VLPs by ∼6-fold, from 0.6 ± 0.6 µm2/s to 0.1 ± 0.2 µm2/s (C). Each individual mAb (c2G4, c13C6, and c4G7) in the ZMapp cocktail appeared to reduce 〈Deff〉 to a comparable extent (Supplementary material, Figure S2).
The observed heterogeneity prompted an evaluation of ZMapp's effect on faster-moving fractions that are more likely to diffuse through the CVM layer before either removal by natural mucosal clearance and/or inactivation by re-acidification of the vaginal lumen or other innate defence mechanisms. We quantitatively defined a “fast-moving” virus population as those with sufficient mobility to pass through an estimated physiological CVM layer of 50 µm within an hour, i.e. those with a minimum 〈Deff〉 of >0.347µm2/s. Among the 50 CVM samples tested, ZMapp reduced the fast-moving virion population by ∼2.5 fold, from ∼ 56% ± 27% to ∼21% ± 22 (D). We further performed a first passage time analysis based on the measured diffusivities of the individual virions., and found that ZMapp increased the predicted time for half of the VLPs to cross a 50 µm layer of CVM from ∼0.7 [0.5, 1] hrs (geometric mean [Lower CI, Upper CI]) to ∼4.8 [3.1, 7.3] hrs (E). ZMapp also increased the time needed for the fastest 10% of virions to cross a 50 µm thick CVM layer, from ∼0.09 [0.07,0.1] hrs to ∼0.5 [0.3, 0.8] hrs (F).
ZMapp-mediated trapping of Ebola VLPs is consistent within select individuals
While the presence of ZMapp induced a clear reduction in the average mobility of Ebola VLPs in CVM, there was substantial inter-specimen variability. In certain samples, ZMapp did not appreciably reduce the 〈Deff〉 or fast-moving populations, whereas the effects were more pronounced and consistent across CVM specimens from the same donors. This led us to classify the donors into two categories: those whose CVM specimens supported a statistically significant trapping of Ebola VLPs (“trappers”), and those whose specimens did not lead to a statistically significant reduction in the mobility of Ebola VLPs vs untreated control (“non-trappers”). ZMapp induced effective trapping of Ebola VLPs in CVM from 9 of 17 donors enrolled in our study, including 4 donors (F13, F65, F66, and F70) whom we were able to collect 3–6 CVM specimens spanning 1–3 menstrual cycles (A,B). Across 31 CVM specimens from the 9 “trapping” donors, ZMapp mediated more effective virion-trapping than the overall average, with 〈Deff〉 reducing by ∼12-fold from 0.6 ± 0.6 µm2/s to 0.05 ± 0.06 µm2/s. Similarly, ZMapp reduced the fast-moving VLP fraction by ∼5-fold, from ∼56 ± 28% in CVM with no mAb to 11 ± 13% in CVM treated with ZMapp (C). In contrast, ZMapp did not induce trapping in nearly all CVM from the “non-trappers”: among the 19 CVM samples from Donors F36, F64, F67 and F68, the measured 〈Deff〉 was only reduced ∼2-fold from 0.4 ± 0.4 µm/s with no mAb to ∼0.2 ± 0.2 µm/s following addition of ZMapp (D). Similarly, the reduction in fast-moving virion populations was marginal, from 53 ± 25% with no mAb to 38 ± 26% with ZMapp.
Figure 2. Diffusion of Ebola VLPs in pH-neutralized CVM that is untreated or treated with ZMapp, segregated by whether ZMapp induced a statistically significant reduction in the fast-moving populations of Ebola VLPs in CVM from the same donor. (A) Fraction of fast-moving virus (average 〈Deff〉 ≥ 0.347 µm2/s, capable of crossing a 50 µm mucus barrier within one hour in each donor with and without ZMapp treatment. Statistical difference is determined by two-way ANOVA followed by post-hoc uncorrected Fisher’s LSD test. (B) Ensemble-averaged geometric Deff at a timescale of 0.2667 s for Ebola VLPs in untreated and ZMapp-treated CVM within the same donors. (C) Fraction of fast-moving virus in untreated or ZMapp-treated CVM samples separated by trapping status. Statistical difference is determined by one-way repeated measures ANOVA corrected by post-hoc Sidak test. Solid lines represent geometric mean and standard deviation. (D) Ensemble-averaged geometric Deff at a timescale of 0.2667 seconds for Ebola VLPs in untreated or ZMapp-treated CVM samples separated by trapping status. Solid lines represent geometric mean and standard deviation. Trapping samples are those from donors with a significant decrease in fast-moving particles before and after treatment with ZMapp. Statistical difference is determined by one-way repeated measures ANOVA corrected by post-hoc Sidak test (*p < 0.05, **p < 0.01, ***p < 0.001, ****p < 0.0001).
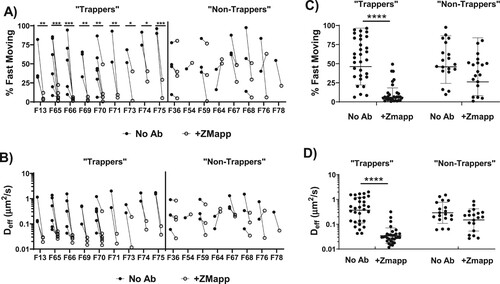
mAb-mediated trapping of Ebola VLPs in CVM may be influenced by the vaginal microbiota
Vaginal microbiota with a low prevalence of highly protective L. crispatus generally correlates with increased susceptibility to various STIs [Citation27–30]. While there are multiple mechanisms that likely contribute to the clinical observations, we have previously shown that the native barrier properties of CVM against HIV differ based on the dominant microbial species present [Citation26]. Thus, we next sought to investigate whether the vaginal microbiome may be associated with the observed variations in mAb-mediated trapping of Ebola VLPs in different CVM specimens. We were able to characterize the microbial composition in 47 of 50 CVM samples via 16S rRNA gene amplicon sequencing, and classified the specimens into five community state types (CSTs) according to the dominant bacterial species, following the standards developed by Ravel, et al [Citation20]. The vast majority of samples in the present study (∼70%) were CST I (L. crispatus dominant, n = 33), with a very limited number in CST II (L. gasseri dominant, n = 3), CST III (L. iners dominant, n = 3), CST IV (G. vaginalis and other anaerobes dominant, n = 5), or CST V (L. jensenii dominant, n = 3).
ZMapp-mediated trapping of Ebola VLPs was statistically significant only among samples classified as CST I. This is attributed in part to the small number of samples belonging to CSTs II-V (Supplementary material, Table S1, A,B). Of the samples classified as “trapping” for which microbiome data were attained, 26 out of 31 (∼84%) were classified as CST I. In contrast, only 7 of 16 non-trapping samples (∼43%) were classified as CST I, suggesting that microbial compositions of CST I may more consistently support Ab-mediated trapping in CVM.
Figure 3. Ab-mediated trapping of Ebola VLPs by Community State Type (CST). (A) Ensemble-averaged 〈Deff〉 or (B) percent fast-moving viruses (Deff ≥ 0.347 µm2/s) in pH-neutralized CVM treated or untreated with ZMapp. Samples are grouped by microbial CST. Samples were sequenced with 16s rRNA whole-genome sequencing analysis and classified into CSTs depending on the predominant microbial species, according to the following groups: Class I (L. crispatus, n = 34), Class II (L.gasseri, n = 3), Class III (L.iners, n = 3), Class IV (G.vaginalis, n = 5), or Class V (L.Jensenii, n = 3). Solid lines represent geometric mean and standard deviation. Statistical significance is measured by repeated measures mixed effects analysis with post-hoc Sidak test (***p < 0.001, ****p < 0.0001).
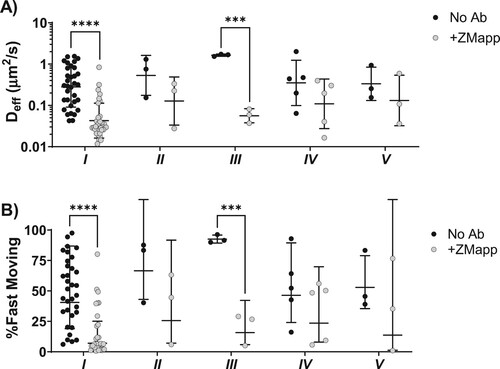
One challenge with utilizing only the dominant microbial species to define the vaginal microbial community is that the substantial variability within specific CSTs is masked (Supplementary material, Figure S3). It is possible that the activity of certain species (e.g. G. vaginalis) can influence the observed potencies of Ab-mediated trapping, even if they are not the dominant species present. We further classified each specimen according to whether its population consisted of >5% of each of the five most common bacteria in the vaginal microbiota. According to this analysis, Ebola VLP mobility in CVM was reduced in all Ab treatment conditions compared to untreated CVM regardless of the bacteria being considered (A,B). We were unable to identify differences in muco-trapping potencies based on either the presence of a minority population of L. iners and anaerobes such as G. vaginalis, or microbial diversity (see supplementary materials).
Figure 4. Ab-mediated Trapping of Ebola VLPs based on presence of substantial bacterial populations. (A) Ensemble-averaged 〈Deff〉 and (B) percent fast-moving viruses (〈Deff〉 ≥ 0.347 µm2/s) in CVM samples whose microbiome contained at least 5% of each bacteria, treated or untreated with ZMapp. (C) Ensemble-averaged 〈Deff〉 and (D) percent fast-moving viruses in pH-neutralized CVM, where samples are sorted according to whether the sum of L. Iners, G. Vaginalis, and other anaerobes composed greater than 5% of the sample’s bacterial population. Solid lines represent geometric mean and standard deviation. Analysis was performed by two-way ANOVA on log-transformed data with post-hoc Holm-Sidak’s test. (*p < 0.05, **p < 0.01, ***p < 0.001, ****p < 0.0001).
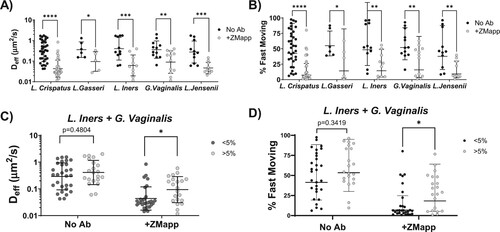
Ab-mediated trapping of Ebola VLPs is consistent across the menstrual cycle and independent of birth control status
Finally, the menstrual cycle can exert a substantial influence on the viscoelastic properties of vaginal CVM [Citation31–33]. We thus assessed whether the menstrual cycle phase may influence the observed variability in the Ab-mediated trapping of Ebola. We normalized all CVM specimens to a 28-day cycle from the end of the last reported menstrual cycle, then classified them as follicular or luteal phase. We included women using hormonal birth control (Supplementary material, Table S1), as many hormonal contraceptives reduce but do not eliminate hormonal fluctuations, and many women using hormonal birth control still exhibit ovarian activity and follicular development to varying degrees [Citation33–35]. In agreement with our previous studies on IgG-mediated trapping of HSV, neither menstrual cycle phase nor birth control status was observed to influence Ab-mediated trapping potency (A,B, Supplementary Figure S6). We also investigated the consistency of muco-trapping potencies across the full 28-day menstrual cycle by plotting either the fast-moving population percentage or 〈Deff〉 as a function of the cycle day and testing for linearity using Pearson's correlation. No significant trend between virus mobility and cycle day was observed for untreated Ebola VLPs (C) or those treated with ZMapp (D), further indicating that Ab-mediated trapping of Ebola VLPs is not influenced by the menstrual cycle phase. Additionally, no relationship was seen between cycle day and the overall Ab-induced mobility change expressed as a ratio of the measurement in the presence of ZMapp to the untreated condition (E).
Figure 5. Effect of the menstrual cycle phase and birth control status on Ab-mediated trapping of Ebola VLPs in pH-neutralized CVM. Cycles were normalized to 28 days based on the donor reported number of days post-menses. CVM samples were grouped by the cycle phase and separated by birth control status. Samples classified as follicular phase were those collected 6–14 days post-menses, while those classified as luteal phase were from 15 to 28 days post-menses. (A) Average 〈Deff〉 of virions in follicular versus luteal phase in normally cycling versus donors on birth control. (B) Percentage of fast-moving virions (average 〈Deff〉 ≥ 0.347 µm2/s) by phase in follicular versus luteal phase in normally cycling versus donors on birth control. Solid lines represent geometric mean and standard deviation. Data were analysed using Two-Way repeated measures ANOVA with Sidak’s multiple comparisons test. A p ≤ 0.05 was considered statistically significant (***p < 0.001, ****p < 0.0001). (C) Average 〈Deff〉 and percent fast-moving of untreated Ebola VLPs in pH-neutralized CVM as a function of cycle day. (D) Average 〈Deff〉 and percent fast-moving of Ebola VLPs treated with ZMapp in pH-neutralized CVM as a function of cycle day. (E) Trapping effect size as a ratio of either average 〈Deff〉 or percent fast-moving virions in a treated sample over the untreated control. The dotted line represents the point at which there was no decrease in mobility in the antibody condition compared to control, with the shaded area representing a decrease in mobility upon addition of Ab. Data were analysed using Spearman’s Correlation. Alpha of p ≤ 0.05 was considered statistically significant; there was no relationship between trapping potency and cycle day.
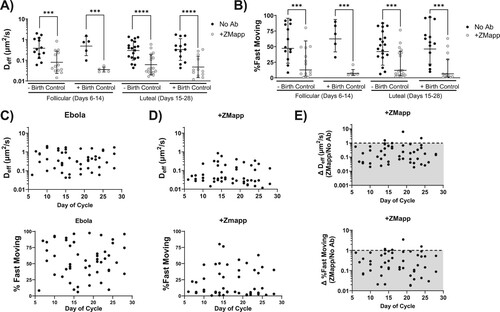
Discussion
Human vaginal epithelium is permissive to EBOV infection [Citation36,Citation37], and vaginal transmission appeared to be a likely transmission route in at least one outbreak [Citation38]. Coupled with the long-term persistence of EBOV in some male EBOV survivors, we believe there is a need to both better understands vaginal transmission of EBOV, and whether vaginal transmission can be blocked. Here, we demonstrate for the first time that Ebola VLPs can readily diffuse across human CVM. This ability to evade adhesion to mucins and undergo rapid diffusion across CVM, is similar to our prior investigations of other viruses that can transmit mucosally (HSV-1 [Citation14,Citation16], HIV-1 [Citation17,Citation39], SARS-CoV-2 [Citation40]), clearly substantiates the feasibility of vaginal EBOV transmission. We also found here that Ebola-binding IgG can markedly hinder the rapid diffusion of Ebola VLPs in the same CVM secretions, consistent with the “muco-trapping” effector function of IgG in CVM we have previously observed for other sexually transmitted viruses [Citation14,Citation16,Citation39]. It is also consistent with our previous observation that ZMapp could mediate effective trapping of Ebola VLP in airway mucus (AM) [Citation15], despite substantial differences in the mucin mesh microstructure and microbial composition in human AM vs. CVM. Our findings with ZMapp underscore muco-trapping via Fc-mucin bonds as a potentially universal effector function of IgG in mucus secretions [Citation15,Citation16,Citation39,Citation41]. Since Ab content in CVM often reflects transudates from blood [Citation42], our work suggests that vaccine-induced IgG, if sustained at sufficient levels in vaginal secretions, may potentially reduce the likelihood of initial vaginal transmission of Ebola.
The inter-sample variability in trapping Ebola VLPs stands in contrast to our previous observations of IgG-mediated trapping of HSV, which was highly consistent across individuals regardless of microbiome class or menstrual phase [Citation16], and also to ZMapp-mediated trapping in human AM [Citation15]. The latter suggests the variations observed here are unlikely related to any inherent heterogeneity with the VLPs. Instead, we conjecture that the difference could be our strategy to generate Ebola VLPs, which incorporated EBOV GP onto a retroviral chassis, as preparations using a filovirus matrix protein chassis yielded insufficient titres for microscopy. While the Gag-GFP protein confers a strong fluorescence intensity that allows us to track the real-time motion of individual virions, the system typically only incorporates a very limited number of glycoprotein spikes on its surface, with HIV Env ranging between 7 and 14 per virion [Citation43]. Such antigen incorporation is markedly lower than the antigenic epitopes that are typically present per virion, which ranges from hundreds for HSV-1 [Citation44] to potentially more than 1000 GP per Ebola virus [Citation45]. The reduced epitope density should proportionally reduce the number of IgG that can bind per Ebola VLP, leading to a gross underestimate of the actual muco-trapping potencies of ZMapp against wild-type EBOV. Indeed, with fewer IgG bound per virion, the overall avidity between virion/IgG complexes may be less consistently achieved in some CVM specimens at the threshold necessary to effectively trap in CVM. This is less likely an issue in human AM, as the tighter mesh spacings in AM (D ∼ 120 nm [Citation23,Citation24]) likely ensure a greater probability of Fc crosslinking with mucins and thus more complete immobilization. In contrast, as the pore sizes of CVM are considerably higher (D ∼ 340 nm, [Citation22,Citation46]), the number of Fc-mucin bonds at any instance in time is likely limiting. This bottleneck can likely be overcome with larger viruses with a greater number of antigen sites leading to greater high overall avidity. We found no appreciable difference in trapping potency between the 3 different mAbs comprising ZMapp, which supports the idea that trapping viruses in mucus depends more on the overall number of Ab-mucin crosslinks rather than the specific epitope on the viral glycoprotein that the Ab targets.
The vaginal microbiome plays an influential role in shaping the overall native barrier properties of CVM. We have previously found that native CVM with microbiota dominant in L. crispatus were able to immobilize HIV, while it was readily diffusive in native specimens containing substantial fractions of L. iners or G. vaginalis [Citation26]. It would not be surprising if other aspects of the CVM barrier, such as Fc-mucin crosslinking, may also be impacted by the vaginal microbiome. Indeed, we recently found that the muco-trapping potencies for broadly neutralizing Ab (bnAb) against HIV-1 is considerably enhanced in CVM from women with L. crispatus dominant microbiota, and considerably reduced in women with G. vaginalis dominated microbiota. Consistent with those findings, we observed here a trend toward reduction in muco-trapping potencies in CVM specimens with substantial populations of G. vaginalis, and a trend towards increased trapping in those with a high relative abundance of L. crispatus. These results highlight the importance of further investigating the vaginal microbiota when assessing the potential efficacy of Ab-mediated interventions against EBOV transmission, regardless of whether the Ab is induced by vaccine or delivered topically for localized passive immunization. These results also suggest methods that can promote low-diversity monocultures of non-iners Lactobacillus may synergistically enhance the potencies of EBOV vaccines in preventing sexual transmission.
The muco-trapping function of IgG remains underexamined in the search for novel preventative strategies against the transmission of STIs. Trapping viruses in mucus via IgG-mucin bonds offers the potential for sterilizing immunity, by blocking infectious agents at the portal of entry and preventing the initiation of infections altogether, as has been previously observed in mouse studies of HSV transmission [Citation14]. Reducing virion mobility through the mucin mesh should proportionally increase the time it takes a virus to reach and infect target cells, allowing other innate and adaptive immune mechanisms more time for complete inactivation, and facilitating elimination via natural mucus clearance mechanisms [Citation47].
Supplemental Material
Download MS Word (6 MB)Disclosure statement
Intellectual property associated with harnessing antibody-mucin interactions described in part in this publication was developed at the University of North Carolina – Chapel Hill (UNC-CH), and has been licensed to Mucommune, LLC and Inhalon Biopharma. SKL is a founder of Mucommune and currently serves as its interim CEO, board of director, and scientific advisory board. SKL is also founder and Chief Scientific Officer of Inhalon Biopharma. SKL owns stock in both companies; SKL’s relationships with both companies are subject to certain restrictions under University policy and are managed by UNC-CH in accordance with its conflict-of-interest policies. JR is co-founder of LUCA Biologics, a biotechnology company focusing on translating microbiome research into live biotherapeutics drugs for women’s health.
Additional information
Funding
References
- Lefebvre A, Fiet C, Belpois-Duchamp C, et al. Case fatality rates of Ebola virus diseases: a meta-analysis of World Health Organization data. Med Mal Infect. 2014;44:412–416. doi:10.1016/j.medmal.2014.08.005
- Rodriguez LL, De Roo A, Guimard Y, et al. Persistence and genetic stability of Ebola virus during the outbreak in Kikwit, Democratic Republic of the Congo, 1995. J Infect Dis. 1999;179(Suppl. 1):S170–S176. doi:10.1086/514291
- Sissoko D, Duraffour S, Kerber R, et al. Persistence and clearance of Ebola virus RNA from seminal fluid of Ebola virus disease survivors: a longitudinal analysis and modelling study. Lancet Glob Health. 2017;5:e80–e88. doi:10.1016/S2214-109X(16)30243-1
- Subissi L, Keita M, Mesfin S, et al. Ebola virus transmission caused by persistently infected survivors of the 2014–2016 outbreak in West Africa. J Infect Dis. 2018;218:S287–S291. doi:10.1093/infdis/jiy280
- Fischer WA, Brown J, Wohl DA, et al. Ebola virus ribonucleic acid detection in semen more than two years after resolution of acute Ebola virus infection. Open Forum Infect Dis. 2017;4:ofx155.
- Mate SE, Kugelman JR, Nyenswah TG, et al. Molecular evidence of sexual transmission of Ebola virus. N Engl J Med. 2015;373:2448–2454. doi:10.1056/NEJMoa1509773
- Boon S D, Marston BJ, Nyenswah TG, et al. Ebola virus infection associated with transmission from survivors. Emerging Infect Dis. 2019;25:249–255. doi:10.3201/eid2502.181011
- Vetter P, Fischer WA, Schibler M, et al. Ebola virus shedding and transmission: review of current evidence. J Infect Dis. 2016;214:S177–S184. doi:10.1093/infdis/jiw254
- Liu WJ, Sesay FR, Coursier A, et al. Comprehensive clinical and laboratory follow-up of a female patient with Ebola virus disease: Sierra Leone Ebola virus persistence study. Open Forum Infect Dis. 2019;6:ofz068.
- Tompkins K, Brown J, Tozay S, et al. The impact of semen testing for Ebola virus RNA on sexual behavior of male Ebola survivors in Liberia. PLoS Negl Trop Dis. 2020;14:e0008556. doi:10.1371/journal.pntd.0008556
- Cone RA. Barrier properties of mucus. Adv Drug Deliv Rev. 2009;61:75–85. doi:10.1016/j.addr.2008.09.008
- Schaefer A, Lai SK. Innate and adaptive barrier properties of airway mucus. In: Hickey AJ, Mansour HM, editors. Inhalation aerosols: physical and biological basis for therapy. 3rd ed. New York (NY): CRC Press; p. 257–274.
- Newby J, Schiller JL, Wessler T, et al. A blueprint for robust crosslinking of mobile species in biogels with weakly adhesive molecular anchors. Nat Commun. 2017;8, 833.
- Wang YY, Kannan A, Nunn KL, et al. Igg in cervicovaginal mucus traps HSV and prevents vaginal herpes infections. Mucosal Immunol. 2014;7:1036–1044. doi:10.1038/mi.2013.120
- Yang B, Schaefer A, Wang Y-Y, et al. Zmapp reinforces the airway mucosal barrier against Ebola virus. J Infect Dis. 2018;218:901–910. doi:10.1093/infdis/jiy230
- Schroeder HA, Nunn KL, Schaefer A, et al. Herpes simplex virus-binding IgG traps HSV in human cervicovaginal mucus across the menstrual cycle and diverse vaginal microbial composition. Mucosal Immunol. 2018;11:1477–1486. doi:10.1038/s41385-018-0054-z
- Lai SK, Hida K, Shukair S, et al. Human immunodeficiency virus type 1 is trapped by acidic but not by neutralized human cervicovaginal mucus. J Virol. 2009;83:11196–11200. doi:10.1128/JVI.01899-08
- Newby JM, Schaefer AM, Lee PT, et al. Convolutional neural networks automate detection for tracking of submicron-scale particles in 2D and 3D. Proc Natl Acad Sci USA. 2018;115:9026–9031. doi:10.1073/pnas.1804420115
- Gajer P, Brotman RM, Bai G, et al. Temporal dynamics of the human vaginal microbiota. Sci Transl Med. 2012;4:132r, a52. doi:10.1126/scitranslmed.3003605
- Ravel J, Gajer P, Abdo Z, et al. Vaginal microbiome of reproductive-age women. Proc Natl Acad Sci USA. 2011;108(Suppl 1):4680–4687. doi:10.1073/pnas.1002611107
- Fadrosh DW, Ma B, Gajer P, et al. An improved dual-indexing approach for multiplexed 16S rRNA gene sequencing on the Illumina MiSeq platform. Microbiome. 2014;2:6.
- Lai SK, O’Hanlon DE, Harrold S, et al. Rapid transport of large polymeric nanoparticles in fresh undiluted human mucus. Proc Natl Acad Sci USA. 2007;104:1482–1487. doi:10.1073/pnas.0608611104
- Lai SK, Suk JS, Pace A, et al. Drug carrier nanoparticles that penetrate human chronic rhinosinusitis mucus. Biomaterials. 2011;32:6285–6290. doi:10.1016/j.biomaterials.2011.05.008
- Schuster BS, Suk JS, Woodworth GF, et al. Nanoparticle diffusion in respiratory mucus from humans without lung disease. Biomaterials. 2013;34:3439–3446. doi:10.1016/j.biomaterials.2013.01.064
- Wang Y-Y, Schroeder HA, Nunn KL, et al. Diffusion of immunoglobulin G in shed vaginal epithelial cells and in cell-free regions of human cervicovaginal mucus. PLoS One. 2016;11:e0158338.
- Nunn KL, Wang Y-Y, Harit D, et al. Enhanced trapping of HIV-1 by human cervicovaginal mucus is associated with Lactobacillus crispatus-dominant microbiota. MBio. 2015;6:e1008236.
- Watts DH, Fazzari M, Minkoff H, et al. Effects of bacterial vaginosis and other genital infections on the natural history of human papillomavirus infection in HIV-1-infected and high-risk HIV-1-uninfected women. J Infect Dis. 2005;191:1129–1139. doi:10.1086/427777
- Allsworth JE, Lewis VA, Peipert JF. Viral sexually transmitted infections and bacterial vaginosis: 2001–2004 national health and nutrition examination survey data. Sex Transm Dis. 2008;35:791–796. doi:10.1097/OLQ.0b013e3181788301
- Borgdorff H, Gautam R, Armstrong SD, et al. Cervicovaginal microbiome dysbiosis is associated with proteome changes related to alterations of the cervicovaginal mucosal barrier. Mucosal Immunol. 2016;9:621–633. doi:10.1038/mi.2015.86
- Brotman RM, Klebanoff MA, Nansel TR, et al. Bacterial vaginosis assessed by gram stain and diminished colonization resistance to incident gonococcal, chlamydial, and trichomonal genital infection. J Infect Dis. 2010;202:1907–1915. doi:10.1086/657320
- Chappell CA, Rohan LC, Moncla BJ, et al. The effects of reproductive hormones on the physical properties of cervicovaginal fluid. Am J Obstet Gynecol. 2014;211(226):e1–e7.
- Wira CR, Fahey JV, Rodriguez-Garcia M, et al. Regulation of mucosal immunity in the female reproductive tract: the role of sex hormones in immune protection against sexually transmitted pathogens. Am J Reprod Immunol. 2014;72:236–258. doi:10.1111/aji.12252
- Aksoy M, Guven S, Tosun I, et al. The effect of ethinyl estradiol and drospirenone-containing oral contraceptives upon mucoprotein content of cervical mucus. Eur J Obstet Gynecol Reprod Biol. 2012;164:40–43. doi:10.1016/j.ejogrb.2012.05.002
- Birtch RL, Olatunbosun OA, Pierson RA. Ovarian follicular dynamics during conventional vs. continuous oral contraceptive use. Contraception. 2006;73:235–243. doi:10.1016/j.contraception.2005.09.009
- Han L, Taub R, Jensen JT. Cervical mucus and contraception: what we know and what we don’t. Contraception. 2017;96:310–321. doi:10.1016/j.contraception.2017.07.168
- Escaffre O, Juelich TL, Freiberg AN. Polyphenylene carboxymethylene (PPCM) in vitro antiviral efficacy against Ebola virus in the context of a sexually transmitted infection. Antiviral Res. 2019;170:104567.
- Escaffre O, Popov V, Hager E, et al. Characterization of an air-liquid interface primary human vaginal epithelium to study Ebola virus infection and testing of antivirals. Antiviral Res. 2023;211:105551.
- 2020 Democratic Republic of the Congo, Equateur Province | Democratic Republic of Congo | Outbreaks | Ebola (Ebola Virus Disease) | CDC.
- Schaefer A, Yang B, Schroeder HA, et al. Broadly neutralizing antibodies consistently trap HIV-1 in fresh cervicovaginal mucus from select individuals. Acta Biomater. 2023;169:387–397. doi:10.1016/j.actbio.2023.07.031
- McSweeney MD, Stewart I, Richardson Z, et al. Stable nebulization and muco-trapping properties of regdanvimab/IN-006 support its development as a potent, dose-saving inhaled therapy for COVID-19. Bioeng Transl Med. 2022;8:e10391.
- Wang Y-Y, Harit D, Subramani DB, et al. Influenza-binding antibodies immobilise influenza viruses in fresh human airway mucus. Eur Respir J. 2017;49:1601709.
- Mkhize NN, Durgiah R, Ashley V, et al. Broadly neutralizing antibody specificities detected in the genital tract of HIV-1 infected women. AIDS. 2016;30:1005–1014. doi:10.1097/QAD.0000000000001038
- Zhu P, Liu J, Bess J, et al. Distribution and three-dimensional structure of AIDS virus envelope spikes. Nature. 2006;441:847–852. doi:10.1038/nature04817
- Grünewald K, Desai P, Winkler DC, et al. Three-dimensional structure of herpes simplex virus from cryo-electron tomography. Science. 2003;302:1396–1398. doi:10.1126/science.1090284
- Beniac DR, Melito PL, Devarennes SL, et al. The organisation of Ebola virus reveals a capacity for extensive, modular polyploidy. PLoS One. 2012;7:e29608. doi:10.1371/journal.pone.0029608
- Lai SK, Wang Y-Y, Hida K, et al. Nanoparticles reveal that human cervicovaginal mucus is riddled with pores larger than viruses. Proc Natl Acad Sci USA. 2010;107:598–603. doi:10.1073/pnas.0911748107
- Nguyen PV, Kafka JK, Ferreira VH, et al. Innate and adaptive immune responses in male and female reproductive tracts in homeostasis and following HIV infection. Cell Mol Immunol. 2014;11:410–427. doi:10.1038/cmi.2014.41