ABSTRACT
Bisphenol A (BPA) is an endocrine disruptor commonly used in industry to manufacture polycarbonate plastics. This endocrine disrupting chemical has been shown to mimic estrogens and has been associated with many serious health conditions. The effects of BPA on bone marrow derived mesenchymal stem cells (BMSCs) were investigated in vitro in the present study. BMSCs were exposed to BPA and assessed for proliferation and self-renewal capacity. The impact of BPA on osteogenic and adipogenic differentiation of BMSCs were also explored. Inhibitor studies were conducted to determine whether BPA alters BMSC biology through estrogen receptor (ER) signaling or MAPK signaling pathways. Although BMSCs treated with BPA demonstrated enhanced proliferation rate, BPA-treated BMSCs demonstrated a reduction in the total number of colony forming units (CFU); however, each CFU was larger (composed of more cells). BPA enhanced adipogenic differentiation of BMSCs, which correlated with increased mRNA expression levels for LEP, PPARG, and LPL following treatment with BPA. The administration of the mitogen-activated protein kinase (MAPK) pathway inhibitor PD184,352 reversed the effects of BPA on proliferation and adipogenesis, whereas the treatment of the cells with ICI182,780 blocked the effects of BPA on self-renewal and adipogenesis. Together, these results suggest that BPA markedly impacts BMSC biology, by enhancing proliferation through MAPK signaling, inhibiting self-renewal through ER signaling, and enhancing adipogenesis through both ER signaling or MAPK signaling.
Introduction
Endocrine disruptors are chemicals that interfere with the endocrine system and produce adverse developmental, reproductive, neurological, or immune effects.Citation1 Endocrine disruptors mimic or partly mimic natural occurring hormones in the body, bind to hormone receptors within a cell, or block endogenous hormone binding.Citation2 Previous studies have shown that exposure to these endocrine disruptors are associated with an increased rate of reproductive disorders and the increased incidence of obesity, diabetes, and certain cancers.Citation3-5
Bisphenol A (BPA) is a carbon-based synthetic endocrine disruptor commonly found in a variety of consumer products, such as water bottles, canned goods, and dental sealants. BPA is also used in thermal paper, which is used in the production of cash and billing receipts.Citation6 Serum and urine analysis indicates that almost all human adults have been exposed to BPA, as free BPA in the serum and BPA metabolites in the urine were found at noticeable concentrations.Citation7-9
The mechanism of action of BPA has been attributed to its structural similarity to estrogens and its affinity to bind to membrane-associated estrogen receptors (ER). Additional studies have shown that BPA may also act through androgen receptor, thyroid hormone receptor, or other endocrine hormone receptors.Citation10-12 As such, the physiological effects of BPA are similar to those associated with the exposure to excess hormones. Animal studies have shown that BPA reduces sperm count and sperm mobility of male mice; female mice exposed to BPA demonstrate increased chromosomal abnormalities in oocytes and reduced fertility.Citation13 Other animal studies demonstrate that the effects of BPA are associated with increased adiposity, increased fasting glucose levels, reduced sensitivity to chemotherapeutic agents, and increased susceptibility to chemical carcinogens. Epidemiological studies examining the association between BPA and various aspects of human health found that exposure to BPA negatively impacts human reproduction, reducing peak ovarian estradiol and reducing incidence of embryo implantation.Citation14-16 In addition, other studies demonstrated that an increase in urinary BPA concentration was associated with increased odds of developing of obesity, cardiovascular disease, insulin resistance, and type 2 diabetes.Citation17-20
While many studies have focused on the effects of BPA on reproduction and metabolic syndrome, few studies have focused on the effects of BPA on the stem cells or progenitor cells. Previous studies have focused on adipose stromal/stem cells (ASCs) or pre-adipocytes, which are progenitor cells that have the potential to self-renew and give rise to mature adipocytes.Citation21,22 ASCs treated with BPA demonstrated enhanced adipogenesis with corresponding increases in the expression of adipogenic genes, including peroxisome proliferator-activated receptor gamma (PPARγ) and lipoprotein lipase (LPL). These findings provide a possible cellular and molecular link between BPA and obesity. To further investigate the effects of BPA on stem cells, bone marrow-derived mesenchymal stem cells (BMSCs) were chosen to determine whether BPA had similar effects on a different stem cell population. As BMSCs are directly involved in haematopoietic stem cell maturation and migration into the circulation, these cells are key regulators of the lymphohematopoietic system. Alterations to BMSCs may result in derangement of the immune response to external cues. Therefore, understanding and reducing factors that may affect BMSC biology is essential.
The mechanism(s) of action of BPA on human BMSCs were investigated in this study. BMSCs were exposed to BPA in vitro, and the impact on proliferation, self-renewal, and differentiation was assessed. The data demonstrate that BPA-treated BMSCs exhibited profound alterations in their core biological properties and indicate that BPA induces these alterations through ER and MAPK signaling pathways.
Results
BPA enhances the proliferation of BMSCs
To assess the effects of BPA on BMSC proliferation, cells were treated with 100 nM or 1 µM BPA for 1 or 5 d and analyzed by MTT assay. BPA treatment for 1 day, irrespective of the concentration of BPA, did not enhance the proliferation of BMSCs (). In contrast, BPA exposure for 5 d at 100 nM and 1 µM BPA enhanced BMSC proliferation by day 7 from 0.36 RAU to 0.42 RAU and 0.41 RAU, respectively (P < 0.05; ).
Figure 1. BPA enhances the proliferation of BMSCs. BMSCs treated with 100 nM or 1 μM BPA for 1 d or 5 d were seeded in a 96-well plate and analyzed on days 2, 4, and 7 by the MTT assay. Values represent triplicates and 3 independent experiments for each of the 3 donors. Bar ± SD. *, P < 0.05 relative to vehicle-treated BMSCs.
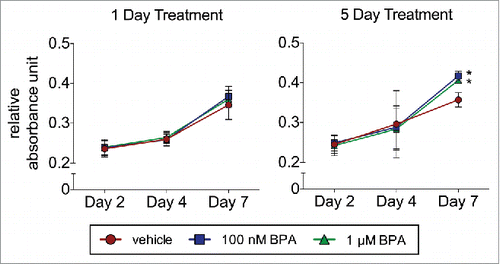
Self-renewal capacity of BMSCs is diminished by exposure to BPA
The affects of BPA on self-renewal capacity were assessed by treating BMSCs with 100 nM or 1 µM BPA for either 1 or 5 d. BMSCs treated with vehicle for one day generated 15.2 colony forming unit (CFU), whereas BPA treated cells demonstrated a reduction in self-renewal capacity to 9.3 CFU and 9.4 CFU at 100 nM BPA and 1 µM BPA, respectively (P < 0.01, ). Compared to BMSCs treated with vehicle for 5 d (15.6 CFU), BPA-treated BMSCs demonstrated diminished capacity to self-renew, resulting in 9.2 CFU when treated with 100 nM BPA and 7.2 CFU when treated with 1 µM (P < 0.01, ). Interestingly, the diameter of the CFUs was significantly larger in size after BPA exposure after 5 d of treatment ().
Figure 2. BPA reduces the self-renewal capacity of BMSCs. BMSCs treated with 100 nM or 1 μM BPA for 1 d or 5 d were plated at low density, incubated for 14 d, and fixed and stained with crystal violet. (A) Colonies greater than 2 mm2 in diameter were counted. (B) Colonies stained with crystal violet were destained, and absorbance was measured on a plate reader at 584 nm. Values represent the ratio of absorbance to the number of CFU. Values represent triplicates and 3 independent experiments for each of the 3 donors. Bar ± SD. **, P < 0.01; ***, P < 0.001 relative to vehicle-treated BMSCs.
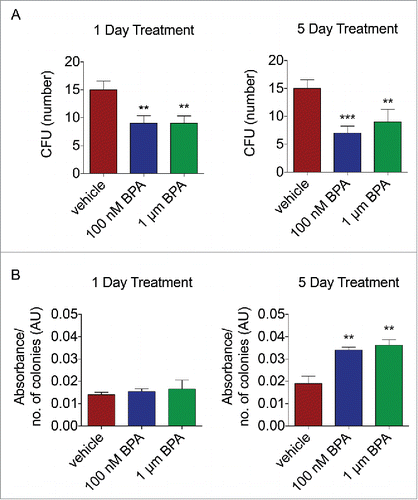
BMSCs exposed to BPA displayed enhanced adipogenic differentiation
Since BMSCs exposed to 1 µM BPA treated for 5 d demonstrated diminished proliferation and self-renewal capacity, all additional BMSC experiments were performed using this concentration. To assess the effects of BPA on BMSC differentiation, cells were exposed to 1 µM BPA for a 5 d period and then induced to differentiate in osteogenic and adipogenic differentiation media in the absence of BPA. The exposure to BPA enhanced the adipogenic differentiation of BMSC to 1.8-fold, compared to vehicle treated BMSCs (). BPA was failed to enhance osteogenic differentiation of BMSCs (). Furthermore, the analysis of adipogenic factors in BPA-treated BMSCs by RT-PCR demonstrated elevated mRNA levels for leptin (LEP), peroxisome proliferator-activated receptor gamma (PPARG), and lipoprotein lipase (LPL), with values of 70.3- (P < 0.001), 98.3- (P < 0.5), and 3984.3-fold (P < 0.001), respectively, compared with controls ().
Figure 3. BPA enhances the adipogenic differentiation of BMSCs. BMSCs treated with 1 μM BPA for 5 d. (A) Treated cells were incubated in osteogenic or adipogenic differentiation medium and stained with Alizarin Red or Oil Red O, respectively, after 14 d. Representative images showing osteogenic and adipogenic differentiation are shown. Bars represent 200 μm. (B) Stains were eluted and absorbance values were obtained on a plate reader at 584 nm. Samples were normalized to the amount of protein present. (C) Cells were assessed by qRT-PCR analysis of total cellular RNA prepared from vehicle- or DDT-treated cells. Values are normalized to β-actin. Values represent triplicates and 3 independent experiments for each of the 3 donors. Bar ± SD. *, P < 0.05; **, P < 0.01; ***, P < 0.001 relative to vehicle-treated BMSCs.
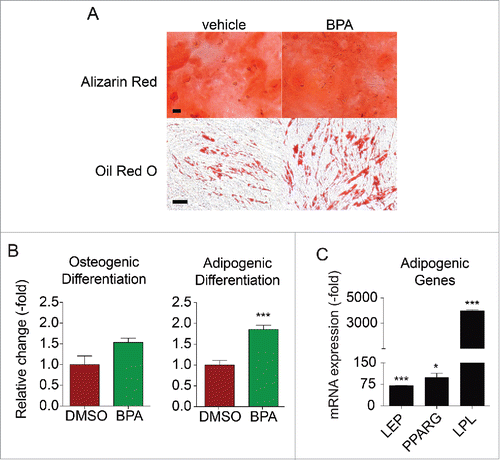
BPA exposure alters the transcriptomic profile of BMSCs
Transcriptome analysis was performed with RNA-sequencing (RNA-seq) on BMSCs exposed to BPA for a 5 d period. Whole transcriptome-wide analysis revealed 267 differentially expressed genes (Supplemental Table 1) in BPA-treated BMSCs that mediate the following canonical pathways: role of BRCA1 in DNA damage response, hereditary breast cancer signaling, mismatch repair in eukaryotes, roles of CHK proteins in cell cycle, checkpoint control, and eicosanoid signaling (). Total RNA was fractionated and small RNA (microRNA, piwi-interacting RNA) analysis of BPA-treated BMSCs revealed 604 differentially expressed genes (Supplemental Table 2) that correlated to pathways involved in the metabolism of cells: GDP-L-fucose biosynthesis II, tetrapyrrole biosynthesis II, lysine degradation II, superpathway of methionine degradation, methionine degradation I to homocysteine ().
Table 1. RNA-sequencing analysis of BMSCs exposed to BPA.
MEK1/2 inhibitor reversed the effects of BPA on BMSC proliferation and ER inhibitor reversed the effects of BPA on BMSC self-renewal capacity
To assess whether BPA affects proliferation and self-renewal capacity through ER signaling or ERK1/2 MAPK signaling pathway, BMSCs were treated with vehicle, ICI182,780 (ER inhibitor), PD184,352 (MEK1/2 inhibitor), BPA, BPA and ICI182,780, or BPA and PD184,352 for 5 d and assessed by MTT assay for proliferation or CFU formation for self-renewal capacity. PD184,352 reversed the proliferative effects of BPA on BMSCs from 0.39 RAU to 0.30 RAU, whereas ICI182,780 was unable to reduce BMSC proliferation induced by BPA by day 7 (). In contrast, ICI182,780 restored the self-renewal capacity of BMSCs, while PD184,352 treatment was unable to enhance the self-renewal capacity of BMSCs treated with BPA (). Exposure to ICI182,780 and PD184,352 alone did not affect BMSC proliferation or self-renewal. Together, these results indicate that the proliferative effects of BPA on BMSCs is mediated via MAPK signaling pathways and that the reduced self-renewal capacity induced by BPA on BMSCs is mediated through ER signaling.
Figure 4. ER inhibitor reverses the effects of BPA on BMSC proliferation and self-renewal. BMSCs were simultaneously treated with BPA and fulvestrant (ICI182,780; 100 nM) or MEK1/2 inhibitor PD184,352 (1 μM) for 5 d. (A) Treated cells were seeded in a 96-well plate and analyzed on day 7 with the MTT assay. (B) Treated BMSCs were plated at low density, incubated for 14 d, and fixed and stained with crystal violet. Colonies greater than 2 mm2 in diameter were counted. Values represent triplicates and 3 independent experiments for each of the 3 donors. Bar ± SD. *, P < 0.05 relative to vehicle-treated BMSCs.
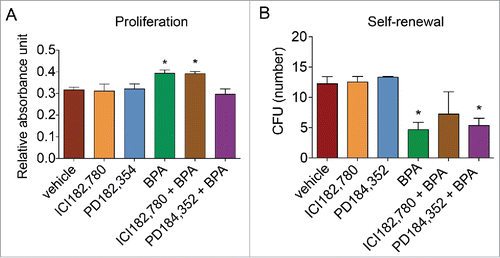
Treatment with ER and MEK1/2 inhibitor reduces the inductive effects of BPA on adipogenic differentiation
To determine whether BPA induces adipogenic differentiation through ER signaling or MAPK signaling, BMSCs were exposed to vehicle, ICI182,780, PD184,352, BPA, BPA and ICI182,780, or BPA and PD184,352 for 5 d. ICI182,780 and PD184,354 reduced the adipogenic differentiation induced by BPA back to baseline levels (). Exposure to ICI182,780 or PD184,352 alone did not affect adipogenic differentiation of BMSCs. Together, these results suggest that BPA enhances adipogenic differentiation through ER signaling and MAPK signaling.
Figure 5. ER and MEK1/2 inhibitor reduces the adipogenic inductive effects of BPA in BMSCs. BMSCs treated with BPA alone or simultaneously with ER inhibitor (ICI182,780; 100 nM) or MEK1/2 inhibitor (PD184,352; 1 μM) for 5 d were incubated in osteogenic or adipogenic differentiation medium and stained with Alizarin Red or Oil Red O, respectively, after 14 d. Stains were eluted and absorbance values were obtained on a plate reader at 584 nm. Samples were normalized to the amount of protein present. Bar ± SD. ***, P < 0.001 relative to vehicle-treated BMSCs.
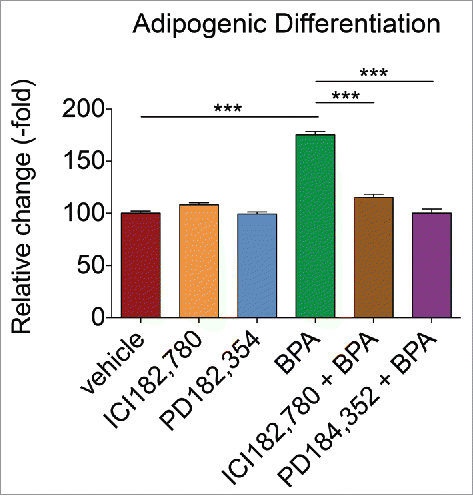
Discussion
In this study, the effects of BPA on human BMSCs were examined. The results show increased proliferation, diminished self-renewal capacity, and enhanced adipogenic differentiation following treatment with BPA. BMSCs exposed to BPA also demonstrated increased expression of several key factors that regulate adipogenic differentiation compared to BMSCs treated with the vehicle. Global transcriptome-wide changes in BMSCs following exposure to BPA was assessed by RNA-seq and revealed several key pathways involved in cell maintenance and metabolism were altered. ICI182,780, an ERα and ERβ antagonist, inhibited the effects of BPA on self-renewal and adipogenic differentiation, whereas MEK1/2 inhibitor PD184,352 reversed the effects of BPA on proliferation and adipogenic differentiation. These results indicate that BPA activates ER genomic signaling pathways as well as non-genomic signaling pathways, such as MAPK signaling, which leads to aberrant stem cell biology.
Enhanced proliferation, reduced self-renewal, increased adipogenic differentiation, and alterations to the transcriptome as a result of BPA exposure would suggest that BPA alters both the expansion and differentiation potential of BMSCs. Masuno et al.Citation22 demonstrated that BPA accelerated the terminal differentiation of pre-adipocytes into adipocytes, leading to increased triglyceride accumulation in cells and higher levels of LPL and FABP4 expression.
Several studies have also investigated the impact of BPA on adipose-derived stromal/stem cells (ASCs), a counterpart to BMSCs due to their mesenchymal origin and ability to differentiate into adipocytes and self-renew. In Ohlstein et al.,Citation21 our group demonstrated that ASCs treated with 10 nM, 100 nM, or 1 μm BPA enhanced adipogenic differentiation and increased expression of DLK, IGF-1, CEBPA, PPARG, and LPL when cells were isolated from subcutaneous depots from adults.Citation21 Additional studies have found similar increases in adipogenic differentiation and adipogenic gene expression in ASCs treated with higher dosages of BPA (25 μM and 50 μM).Citation23 ASCs isolated from visceral adipose depots also demonstrated similar increases in adipogenic differentiation at concentrations as low as 10 nM and as high as 80 uM.Citation24 Together with our findings, the results indicate that BPA induces adipogenic differentiation of several mesenchymal lineage cells.
The enhanced adipogenic differentiation of BMSCs may have significant consequences to the bone marrow compartment and in turn the lymphohematopoietic system. As a vital cellular component of the bone marrow niche, BMSCs are key regulators of haematopoietic stem cells (HSCs) by maintaining HSCs in a quiescent state in the marrow cavity. Alterations to BMSCs may result in homeostatic imbalance of HSCs, resulting in the generation and/or expansion of malignant cells.Citation25,26 Additional studies have demonstrated that BMSCs from patients with acute lymphocytic leukemia showed severe dysfunction and even loss of the suppressive immune-regulatory effects on the proliferation of immune cells suggesting aberrant functional properties of BMSCs.Citation25,26 Consistent with our study, changes in the stem cell properties of BMSCs due to exposure to BPA could play a role in altering the lymphohematopoietic system.
Studies have indicated that BPA signals through ERs. Previous investigations have demonstrated that BPA binds to and activates ERα and ERβ.Citation27 The inhibitor studies performed here indicate that BPA binds to ERα and ERβ to inhibit self-renewal and induce adipogenic differentiation of cells. However, BPA may utilize other ERs to induce proliferative effects. In recent years, the identification of G-protein coupled receptor (GPER) as a novel ER suggests new avenues by which estrogenic compounds may induce biological effects in different cell types.Citation28-32 BPA is one of several environmental estrogens that have exhibited the ability to bind to GPER and to activate signal transduction cascades involved in the biological responses of cells.Citation33 One such signaling transduction cascade is the MAPK signaling pathway. Previous studies have demonstrated a role for MAPK signaling in EDC-mediated regulation of gene expression and proliferation.Citation34-39 Consistent with these studies, the results of this study would suggest that BPA may bind to GPER and induce MAPK signaling, resulting in enhanced proliferation and adipogenic differentiation. Interesting, these results indicate that BPA may induce adipogenic differentiation through 2 independent pathways.
In summary, BPA significantly alters the core biologic properties of BMSC. While additional studies are necessary to determine the precise mechanism of action, BPA acts through both ER and MAPK signaling pathways. Future in vitro and in vivo studies investigating the precise mechanism by which BPA effects BMSC biology will identify specific transcription factors or other proteins involved in cell signaling for which we can target and reduce the negative effect of this endocrine disrupting chemical on human health.
Materials and methods
Chemicals
Bisphenol A (BPA), fulvestrant (ICI 182,780), MEK1/2 inhibitor PD184352, dexamethasone, isobuytlmethylxanthine, indomethacin, ascorbate 2-phosphate, β-glycerol phosphate, Oil Red O, Alizarin Red S, cetylpyridinium chloride (CPC), and crystal violet were all purchased from Sigma (St. Louis, MO).
Isolation of BMSCs
BMSCs from 3 normal healthy donors (mean age 25.3 ± 6.6 and mean body mass index 21.3 ± 1.7) were obtained after informed consent and under a protocol approved by Tulane University Institutional Review Board. The cells were prepared from bone marrow aspirates using standard protocols as previously described.Citation40 In brief, bone marrow aspirates were isolated from the iliac crest of normal adult donors using a density gradient (Ficol-Paque; Amersham Pharmacia Biotech; Milwaukee, WI) and cultured in complete culture media (CCM), which consisted of α-MEM (GIBCO; Grand Island, NY), 20% fetal bovine serum (FBS; Atlanta Biologicals; Lawrenceville, GA), 100 units per ml penicillin/100 µg/ml streptomycin (P/S; GIBCO), and 2 mM L-glutamine (GIBCO).
Cell culture
BMSCs (106) were thawed, plated in CCM, and incubated at 37°C with 5% humidified CO2. After 24 hours, viable cells were washed, harvested with 0.25% trypsin / 1 mM EDTA, and replated at 100 cells/cm2 in CCM. Media was changed every 3–4 d. For all experiments, sub-confluent cells (≤70 % confluent) between passages 2–6 were used. Where indicated, BMSCs from each donor were treated separately with DMSO as a vehicle control, 100 nM BPA, or 1 μM BPA for 1 day or 5 d in α-MEM supplemented with 20% charcoal dextrose stripped FBS (CDS-FBS), 2 mM L-glutamine, and P/S. Combined treatment of 1 μM BPA and 100 nM ICI182,780 or 1 μM BPA and 1 μM PD184,352 was administered for 5 d in CDS-FBS. Images were acquired on Nikon Eclipse TE200 (Melville, NY) with Nikon Digital Camera DXM1200F using Nikon ACT-1 software version 2.7.
MTT assay
Assessment of cell viability was performed according to MTT (3-(4,5-dimethylthiazol-2-yl)-2,5-diphenyltetrazolium bromide, Invitrogen) assay. BMSCs were treated for 5 d and then plated in triplicates in 96-well plates at a density of 500 cells per well. After 2, 4, and 7 days, cells were incubated with 10 mM MTT (Invitrogen; Grand Island, NY) for 4 hours at 37°C with 5% humidified CO2. A total of 100 μl of dissolving solution (10% SDS, 0.01 M HCl) was added to each well and incubated for an additional 12–16 h at 37°C with 5% humidified CO2. Absorbance was measured at 544 nm (FLUOstar optima). Inhibition studies were similarly performed on BMSCs treated for 5 d and assessed for proliferation on day 7 with 10 nM MTT for 4 hours.
Colony forming unit assay
Treated BMSCs from each donor (n = 3) were plated in triplicates at 1.8 cells/cm2 on adherent 6-well dishes (Nunc) and incubated for 14 d. Plates were washed with PBS, and stained with 3% crystal violet dissolved in methanol for 30 min. Plates were washed and colonies that were greater than 2 mm2 in diameter were counted. Plates were destained with methanol and absorbance values were measured at 584 nm (FLUOstar optima; BMG Labtech Inc.; Durham, NC). Values represent the ratio of the absorbance value to the number of colony forming units and are shown as arbitrary units (A.U.). Assays were performed 3 independent times.
Differentiation protocols
Treated BMSCs (105) from each donor (N = 3) were plated in 6-well plates in triplicates and cultured in CCM until 70% confluent. For osteogenic differentiation, CCM was replaced with media containing osteogenic supplements, which consisted of 50 µM ascorbate 2-phosphate, 10 mM β-glycerol phosphate, and 10 nM dexamethasone. For adipogenic differentiation, CCM was replaced with media containing adipogenic supplements, which consisted of 0.5 µM dexamethasone, 0.5 mM isobuytlmethylxanthine, and 50 µM indomethacin. After 2 weeks, cells were fixed, washed, and stained with 40 mM Alizarin Red to assess osteogenic differentiation or 0.5% Oil Red O to assess adipogenic differentiation. Images were acquired on the Nikon Eclipse TE200 with Nikon Digital Camera DXM1200F using Nikon ACT-1 software version 2.7. For quantification, the Alizarin Red or Oil Red O was extracted from each well with 10% CPC or isopropanol, respectively, and read at 584 nm (FLUOstar optima). To normalize to amount of protein in each sample, protein extraction with RIPA buffer (Pierce; Thermo Scientific; Waltham, MA) and protein quantification with the Bicinchoninic acid (BCA) assay (Thermo Scientific) was performed according to manufacturer's instructions.
RNA isolation and real-time RT-PCR
MSCs (106) from 3 different donors were treated separately with vehicle or 1 μM BPA for 5 d. Total cellular RNA was extracted from BMSCs using TRIzol reagent (Invitrogen), purified with DNase I digestion (Invitrogen) according to manufacturer's instructions, and reverse transcribed using the SuperScript VILO cDNA synthesis kit (Invitrogen). Quantitative real-time PCR was performed in triplicates using the EXPRESS SYBR GreenER qPCR SuperMix Kit (Invitrogen) according to the manufacturer's instructions. Primer sequences are as follows: LEP forward 5′-GGCTTTGGCCCTATCTTTTC-3′ and reverse 5′-GCTCTTAGAGAAGGCCAGCA-3′; PPARG forward 5′- GCTGTTATGGGTGAAACTCTG-3′ and reverse 5′- ATAAGGTGGAGATGCAGGTTC-3′; LPL forward 5′-GAGATTTCTCTGTATGGCACC-3′ and reverse 5′-CTGCAAATGAGACACTTTCTC-3′, and β-actin forward 5′- CACCTTCTACAATGAGCTGC-3′ and reverse 5′-TCTTCTCGATGCTCGACGGA-3′.Citation40 The expression of human β-actin was used to normalize mRNA content.
Next generation sequencing and ingenuity pathway analysis
BMSCs (106) were treated with vehicle or 1 μM BPA. After 5 d, cells were trypsinized, washed with PBS, and stored at −80°C prior to RNA isolation. RNA-seq was performed as previously described.Citation41 cDNA libraries were prepared from ribo-depleted total RNA and sequenced on an Illumina HiSeq 2000 instrument. Before RNA-seq reads were aligned to the human genome, 3 nucleotides from the 5′ end of each read were trimmed using PrinSeq [default options]. Reads were then aligned and analyzed as previously described.Citation42,43 An adjusted P < 0.05 was applied for multiple testing for identifying significant differential expression. Data was inputted into Ingenuity Pathway Analysis and top canonical pathways were analyzed.
Abbreviations
ASCs | = | adipose-derived stem cells |
BMSC | = | bone marrow-derived mesenchymal stem cells |
BPA | = | bisphenol A |
CCM | = | complete culture medium |
HSC | = | haematopoietic stem cells |
ICI182,780 | = | fulvestrant |
MAPK | = | mitogen-activated protein kinase |
PD184,352 | = | MEK1/2 inhibitor |
Disclosure of potential conflicts of interest
No potential conflicts of interest were disclosed.
KEND_1200344_Supplemental_Table_1_and_Figure_1.pdf
Download PDF (238.1 KB)References
- Schug TT, Janesick A, Blumberg B, Heindel JJ. Endocrine disrupting chemicals and disease susceptibility. J Steroid Biochem Mol Biol 2011; 127:204-15; PMID:21899826; http://dx.doi.org/10.1016/j.jsbmb.2011.08.007
- Swedenborg E, Ruegg J, Makela S, Pongratz I. Endocrine disruptive chemicals: mechanisms of action and involvement in metabolic disorders. J Mol Endocrinol 2009; 43:1-10; PMID:19211731; http://dx.doi.org/10.1677/JME-08-0132
- De Coster S, van Larebeke N. Endocrine-disrupting chemicals: associated disorders and mechanisms of action. J Environ Public Health 2012; 2012:713696; PMID:22991565; http://dx.doi.org/10.1155/2012/713696
- Stel J, Legler J. The Role of Epigenetics in the Latent Effects of Early Life Exposure to Obesogenic Endocrine Disrupting Chemicals. Endocrinology 2015; 156:3466-72; PMID:26241072; http://dx.doi.org/10.1210/en.2015-1434
- Prins GS, Calderon-Gierszal EL, Hu WY. Stem Cells as Hormone Targets That Lead to Increased Cancer Susceptibility. Endocrinology 2015; 156:3451-7; PMID:26241068; http://dx.doi.org/10.1210/en.2015-1357
- Hormann AM, Vom Saal FS, Nagel SC, Stahlhut RW, Moyer CL, Ellersieck MR, Welshons WV, Toutain PL, Taylor JA. Holding thermal receipt paper and eating food after using hand sanitizer results in high serum bioactive and urine total levels of bisphenol A (BPA). PloS one 2014; 9:e110509; PMID:25337790; http://dx.doi.org/10.1371/journal.pone.0110509
- Calafat AM, Ye X, Wong LY, Reidy JA, Needham LL. Exposure of the U.S. population to bisphenol A and 4-tertiary-octylphenol: 2003-2004. Environ Health Perspect 2008; 116:39-44; PMID:18197297; http://dx.doi.org/10.1289/ehp.10753
- Liao C, Kannan K. Determination of free and conjugated forms of bisphenol A in human urine and serum by liquid chromatography-tandem mass spectrometry. Environ Sci Technol 2012; 46:5003-9; PMID:22489688; http://dx.doi.org/10.1021/es300115a
- Vandenberg LN, Chahoud I, Heindel JJ, Padmanabhan V, Paumgartten FJ, Schoenfelder G. Urinary, circulating, and tissue biomonitoring studies indicate widespread exposure to bisphenol A. Environ Health Perspect 2010; 118:1055-70; PMID:20338858; http://dx.doi.org/10.1289/ehp.0901716
- Sheng ZG, Tang Y, Liu YX, Yuan Y, Zhao BQ, Chao XJ, Zhu BZ. Low concentrations of bisphenol a suppress thyroid hormone receptor transcription through a nongenomic mechanism. Toxicol Appl Pharmacol 2012; 259:133-42; PMID:22227104; http://dx.doi.org/10.1016/j.taap.2011.12.018
- Richter CA, Taylor JA, Ruhlen RL, Welshons WV, Vom Saal FS. Estradiol and Bisphenol A stimulate androgen receptor and estrogen receptor gene expression in fetal mouse prostate mesenchyme cells. Environ Health Perspect 2007; 115:902-8; PMID:17589598; http://dx.doi.org/10.1289/ehp.9804
- Moriyama K, Tagami T, Akamizu T, Usui T, Saijo M, Kanamoto N, Hataya Y, Shimatsu A, Kuzuya H, Nakao K. Thyroid hormone action is disrupted by bisphenol A as an antagonist. J Clin Endocrinol Metab 2002; 87:5185-90; PMID:12414890; http://dx.doi.org/10.1210/jc.2002-020209
- Vandenberg LN, Ehrlich S, Belcher SM, Ben-Jonathan N, Dolinoy DC, Hugo ER, Hunt PA, Newbold RR, Rubin BS, Saili KS, et al. Low dose effects of bisphenol A. Endocrine Disruptors 2013; 1:e26490; PMID:NOT_FOUND; http://dx.doi.org/10.4161/endo.26490
- Fujimoto VY, Kim D, Vom Saal FS, Lamb JD, Taylor JA, Bloom MS. Serum unconjugated bisphenol A concentrations in women may adversely influence oocyte quality during in vitro fertilization. Fertil Steril 2011; 95:1816-9; PMID:21122836; http://dx.doi.org/10.1016/j.fertnstert.2010.11.008
- Ehrlich S, Williams PL, Missmer SA, Flaws JA, Ye X, Calafat AM, Petrozza JC, Wright D, Hauser R. Urinary bisphenol A concentrations and early reproductive health outcomes among women undergoing IVF. Hum Reprod 2012; 27:3583-92; PMID:23014629; http://dx.doi.org/10.1093/humrep/des328
- Ehrlich S, Williams PL, Missmer SA, Flaws JA, Berry KF, Calafat AM, Ye X, Petrozza JC, Wright D, Hauser R. Urinary bisphenol A concentrations and implantation failure among women undergoing in vitro fertilization. Environ Health Perspect 2012; 120:978-83; PMID:22484414; http://dx.doi.org/10.1289/ehp.1104307
- Lang IA, Galloway TS, Scarlett A, Henley WE, Depledge M, Wallace RB, Melzer D. Association of urinary bisphenol A concentration with medical disorders and laboratory abnormalities in adults. Jama 2008; 300:1303-10; PMID:18799442; http://dx.doi.org/10.1001/jama.300.11.1303
- Carwile JL, Michels KB. Urinary bisphenol A and obesity: NHANES 2003-2006. Environ Res 2011; 111:825-30; PMID:21676388; http://dx.doi.org/10.1016/j.envres.2011.05.014
- Trasande L, Attina TM, Blustein J. Association between urinary bisphenol A concentration and obesity prevalence in children and adolescents. Jama 2012; 308:1113-21; PMID:22990270; http://dx.doi.org/10.1001/2012.jama.11461
- Melzer D, Osborne NJ, Henley WE, Cipelli R, Young A, Money C, McCormack P, Luben R, Khaw KT, Wareham NJ, et al. Urinary bisphenol A concentration and risk of future coronary artery disease in apparently healthy men and women. Circulation 2012; 125:1482-90; PMID:22354940; http://dx.doi.org/10.1161/CIRCULATIONAHA.111.069153
- Ohlstein JF, Strong AL, McLachlan JA, Gimble JM, Burow ME, Bunnell BA. Bisphenol A enhances adipogenic differentiation of human adipose stromal/stem cells. J Mol Endocrinol 2014; 53:345-53; PMID:25143472; http://dx.doi.org/10.1530/JME-14-0052
- Masuno H, Iwanami J, Kidani T, Sakayama K, Honda K. Bisphenol a accelerates terminal differentiation of 3T3-L1 cells into adipocytes through the phosphatidylinositol 3-kinase pathway. Toxicol Sci 2005; 84:319-27; PMID:15659569; http://dx.doi.org/10.1093/toxsci/kfi088
- Boucher JG, Boudreau A, Atlas E. Bisphenol A induces differentiation of human preadipocytes in the absence of glucocorticoid and is inhibited by an estrogen-receptor antagonist. Nutr Diabetes 2014; 4:e102; PMID:24418828; http://dx.doi.org/10.1038/nutd.2013.43
- Wang J, Sun B, Hou M, Pan X, Li X. The environmental obesogen bisphenol A promotes adipogenesis by increasing the amount of 11beta-hydroxysteroid dehydrogenase type 1 in the adipose tissue of children. Int J Obesity (2005) 2013; 37:999-1005; PMID:23090578; http://dx.doi.org/10.1038/ijo.2012.173
- Rodriguez-Pardo VM, Aristizabal JA, Jaimes D, Quijano SM, De los Reyes I, Herrera MV, Solano J, Vernot JP. Mesenchymal stem cells promote leukaemic cells aberrant phenotype from B-cell acute lymphoblastic leukaemia. Hematol Oncol Stem Cell Ther 2013; 6:89-100; PMID:24161606; http://dx.doi.org/10.1016/j.hemonc.2013.09.002
- Hanoun M, Zhang D, Mizoguchi T, Pinho S, Pierce H, Kunisaki Y, Lacombe J, Armstrong SA, Duhrsen U, Frenette PS. Acute myelogenous leukemia-induced sympathetic neuropathy promotes malignancy in an altered hematopoietic stem cell niche. Cell Stem Cell 2014; 15:365-75; PMID:25017722; http://dx.doi.org/10.1016/j.stem.2014.06.020
- Welshons WV, Nagel SC, Vom Saal FS. Large effects from small exposures. III. Endocrine mechanisms mediating effects of bisphenol A at levels of human exposure. Endocrinology 2006; 147:S56-69; PMID:16690810; http://dx.doi.org/10.1210/en.2005-1159
- Pupo M, Pisano A, Lappano R, Santolla MF, De Francesco EM, Abonante S, Rosano C, Maggiolini M. Bisphenol A induces gene expression changes and proliferative effects through GPER in breast cancer cells and cancer-associated fibroblasts. Environ Health Perspect 2012; 120:1177-82; PMID:22552965; http://dx.doi.org/10.1289/ehp.1104526
- Vivacqua A, Bonofiglio D, Albanito L, Madeo A, Rago V, Carpino A, Musti AM, Picard D, Ando S, Maggiolini M. 17beta-estradiol, genistein, and 4-hydroxytamoxifen induce the proliferation of thyroid cancer cells through the g protein-coupled receptor GPR30. Mol Pharmacol 2006; 70:1414-23; PMID:16835357; http://dx.doi.org/10.1124/mol.106.026344
- Albanito L, Madeo A, Lappano R, Vivacqua A, Rago V, Carpino A, Oprea TI, Prossnitz ER, Musti AM, Ando S, et al. G protein-coupled receptor 30 (GPR30) mediates gene expression changes and growth response to 17beta-estradiol and selective GPR30 ligand G-1 in ovarian cancer cells. Cancer Res 2007; 67:1859-66; PMID:17308128; http://dx.doi.org/10.1158/0008-5472.CAN-06-2909
- Maggiolini M, Picard D. The unfolding stories of GPR30, a new membrane-bound estrogen receptor. J Endocrinol 2010; 204:105-14; PMID:19767412; http://dx.doi.org/10.1677/JOE-09-0242
- Thomas P, Pang Y, Filardo EJ, Dong J. Identity of an estrogen membrane receptor coupled to a G protein in human breast cancer cells. Endocrinology 2005; 146:624-32; PMID:15539556; http://dx.doi.org/10.1210/en.2004-1064
- Thomas P, Dong J. Binding and activation of the seven-transmembrane estrogen receptor GPR30 by environmental estrogens: a potential novel mechanism of endocrine disruption. J Steroid Biochem Mol Biol 2006; 102:175-9; PMID:17088055; http://dx.doi.org/10.1016/j.jsbmb.2006.09.017
- Frigo DE, Burow ME, Mitchell KA, Chiang TC, McLachlan JA. DDT and its metabolites alter gene expression in human uterine cell lines through estrogen receptor-independent mechanisms. Environ Health Perspect 2002; 110:1239-45; PMID:12460804; http://dx.doi.org/10.1289/ehp.021101239
- Frigo DE, Tang Y, Beckman BS, Scandurro AB, Alam J, Burow ME, McLachlan JA. Mechanism of AP-1-mediated gene expression by select organochlorines through the p38 MAPK pathway. Carcinogenesis 2004; 25:249-61; PMID:14604893; http://dx.doi.org/10.1093/carcin/bgh009
- Frigo DE, Vigh KA, Struckhoff AP, Elliott S, Beckman BS, Burow ME, McLachlan JA. Xenobiotic-induced TNF-alpha expression and apoptosis through the p38 MAPK signaling pathway. Toxicology letters 2005; 155:227-38; PMID:15603917; http://dx.doi.org/10.1016/j.toxlet.2004.09.008
- Frigo DE, Basu A, Nierth-Simpson EN, Weldon CB, Dugan CM, Elliott S, Collins-Burow BM, Salvo VA, Zhu Y, Melnik LI, et al. p38 mitogen-activated protein kinase stimulates estrogen-mediated transcription and proliferation through the phosphorylation and potentiation of the p160 coactivator glucocorticoid receptor-interacting protein 1. Mol Endocrinol 2006; 20:971-83; PMID:16410316; http://dx.doi.org/10.1210/me.2004-0075
- Bratton MR, Frigo DE, Vigh-Conrad KA, Fan D, Wadsworth S, McLachlan JA, Burow ME. Organochlorine-mediated potentiation of the general coactivator p300 through p38 mitogen-activated protein kinase. Carcinogenesis 2009; 30:106-13; PMID:18791200; http://dx.doi.org/10.1093/carcin/bgn213
- Bratton MR, Frigo DE, Segar HC, Nephew KP, McLachlan JA, Wiese TE, Burow ME. The organochlorine o,p′-DDT plays a role in coactivator-mediated MAPK crosstalk in MCF-7 breast cancer cells. Environ Health Perspect 2012; 120:1291-6; PMID:22609851; http://dx.doi.org/10.1289/ehp.1104296
- Strong AL, Shi Z, Strong MJ, Miller DF, Rusch DB, Buechlein AM, Flemington EK, McLachlan JA, Nephew KP, Burow ME, et al. Effects of the Endocrine-Disrupting Chemical DDT on Self-Renewal and Differentiation of Human Mesenchymal Stem Cells. Environ Health Perspect 2014; 123(1):42-8; PMID:25014179
- Miller DFB, Yan PS, Buechlein A, Rodriguez BA, Yilmaz AS, Goel S, Lin H, Collins-Burow B, Rhodes LV, Braun C, et al. Methods http://dx.doi.org/10.1016/j.ymeth.2013.03.0232013
- Miller DF, Yan PS, Buechlein A, Rodriguez BA, Yilmaz AS, Goel S, Lin H, Collins-Burow B, Rhodes LV, Braun C, et al. A new method for stranded whole transcriptome RNA-seq. Methods 2013; 63:126-34; PMID:23557989; http://dx.doi.org/10.1016/j.ymeth.2013.03.023
- Miller DF, Yan PX, Fang F, Buechlein A, Ford JB, Tang H, Huang TH, Burow ME, Liu Y, Rusch DB, et al. Stranded Whole Transcriptome RNA-Seq for All RNA Types. Cur Protoc Hum Genet 2015; 84:11.4.1-.4.23; PMID:25599667