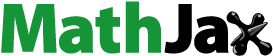
Abstract
Despite its rapid growth and adaptability, Andropogon (Andropogon gayanus 12,465) was not preserved to address the severe feed deficit in the prolonged dry season in Ethiopia. Determining how the harvesting stage and additives (AD) affect the silage quality, animal preference, and digestibility of Andropogon was the objective of the current study. Three stages of harvesting (vegetative, boot, and full bloom) of the grass were used, and four AD (untreated (WO), with molasses (WM), with urea (WU), and with urea + molasses (WUM)) were factorially combined with a completely randomized design. With WO and molasses-based additives, the vegetative and boot-stage silage had a pleasant smell. The full-bloom silage scored lower than the other two stages for its odor, color, texture, and moldiness. The silage pH value with WM shows that it was lower in the vegetative (4.31) and boot (4.10) stages than in the full bloom (5.08) stages. At the boot stage, WM and WU showed higher silage crude protein (CP) content than WO silages. Based on dry matter (DM) intake, the Arab goat showed a higher preference for boot-stage silages with WM followed by WUM, while the lowest was with WO and WU. When compared to the other additives, goats fed WM and WU digested more DM, OM, and CP. The result also showed that the pH of the silage was strongly associated with texture. According to our findings, Andropogon, which was harvested at the boot stage and ensiled with WM has the potential to produce high-quality silage.
1. Introduction
Livestock productivity is mainly affected by feed-related issues due to seasonal variations in green feed availability, the poor nutritional quality of feed, an inability to afford concentrate feed, and lower adoption of forage preservation techniques like making hay and silage. In Ethiopia, green fodder is abundant during the wet season of the year, but during the dry season, there is severe feed scarcity (Abebe et al., Citation2017). Particularly in the western part of Ethiopia, feed shortage in the dry season is a critical problem due to the lower forage preservation practices like making hay and silage. According to the study, natural pastures only contribute 2.8% of the hay, and no silage is used to feed livestock in Benishangul Gumuz regional state, western Ethiopia (CSA Authority central statistics, Citation2010). In this region, the lack of such practices is the key factor contributing to feed scarcity. And, the native pasture in the area is dominated by Hypernia rufa grass which quickly loses its quality, especially during the dry season (Abebe et al., Citation2017). In addition to these, smallholder farmers also burn pasture fields and bushes from December to April, which aggravates the feed shortage in the area (AsARC 2006, unpublished data).
To solve the major livestock production constraints, recently different improved forages were introduced into the region by governmental and non-governmental organizations. From the introduced improved forage, Andropogon (Andropogon gayanus 12,465) Dirk Ayifera variety (locally known as drought tolerant) was released in 2009. The grass is perennial, adaptive to the lowland area, fast-growing, has high biomass production (10–12 tons DM/ha), drought tolerant, and favorable growth on low fertility acidic soils (Getnet et al., Citation2020). At 50% heading, the grass contains 90.76, 8.69, 91.30, 6.34, 77.31, 50.02, and 8.61 percent of dry matter (DM), ash, OM (organic matter), CP (crude protein), NDF (Neutral detergent fiber), ADF (acid detergent fiber), and ADL (acid detergent lignin), respectively (Arega et al., Citation2020).
However, due to the region’s high precipitation during the rainy season (May–November), haymaking at the recommended growth stage of the grass is not possible in the area. So, ensiling of this improved grass during the rainy season is one management strategy to avail feed in the dry season.
But to prepare good-quality grass silage, identifying the best time of harvesting is critical. It is well known that early harvest of grass generally produces highly digestible silage that promotes high feed intake and milk yield (Pang et al., Citation2021). Harvesting grass for a combined high productivity and nutritive value of silage is difficult due to the low DM content (15 to 20 g/kg) (Da Silva et al., Citation2019). A previous study showed thatAndropogon gayanus silages ruminal degradation kinetics ensiled at 56 days of regrowth had higher nutritional value than 84 and 112 days (Ribeiro et al., Citation2014)
In addition to harvesting stages in East Africa, the use of chemical or microbial additives in the preservation of forages is not common (Balehegn et al., Citation2022). Tropical grasses showed lower CP and water-soluble carbohydrates (WSC) which reduces the quality of the silage (Bernardes et al., Citation2018). So, additives like molasses, cereals, beet, citrus pulp, and urea for grasses are necessary to improve silage quality, nutritive value, preference, and digestibility (Da Silva et al., Citation2017). From those additives, molasses is added to provide fast fermentable carbohydrates for the ensilage of tropical grasses. Urea treatment increases the crude protein content of tropical grass and is effective in increasing the digestibility of fibrous feed (Ahmed et al., Citation2013). Moreover, in Ethiopia, both molasses and urea additives are easily accessible to smallholder livestock producers.
Furthermore, in Ethiopia, silage feeding of indigenous cattle is not common. So, assessing the preference of silage by Arab goats (Capra hircus) and generally for small ruminants is important. A study showed that due to poor silage properties such as pungent smell and mold growth, the silage could be rejected by the animals (Falola et al., Citation2013).
There is little information available about the best time to harvest Andropogon for silage production by using urea and molasses as additives to improve silage quality, nutritive value, preference, and digestibility to fill the feed gap during the dry season. As a result, the current study was aimed to determine the effect of the harvesting stage and additives on the silage quality of Andropogon grass, as well as the animal preference, feed intake, and digestibility of silages made from this grass.
2. Materials and methods
2.1. Field experiment layout and design
Andropogon (Andropogon gayanus 12,465) Dirki Ayifera variety was established at Assosa Agricultural Research Center in Benishangul Gumuz Regional State, western Ethiopia, in 27 July 2019 for silage production, preference, and digestibility study. The experimental area (402.5 m2) was prepared at the start of the experiment and divided into six blocks (11.5 × 5 m2) and three plots per block (3.5 × 5 m2) for a total of 18 plots for laboratory silage making. The distance between the blocks was 1 m and 0.5 m between the plots. Using the lottery method of randomization, each harvesting stage (Vegetative, Boot, and Full bloom) was assigned to each plot at random. Then for silage preparation, the assigned six random plots from 18 plots (one replicate in each block) were used at each harvesting stage. Regarding the preference test and digestibility experiment, an additional area of 450 m2 (12 × 36 m2) was prepared similarly to harvest Andropogon at the boot stage.
2.2. Plot preparation and experimental materials
The layout was prepared as per the specification and each plot was leveled and ridged manually. To ensure adequate emergence, Andropogon grass seed was broadcast at 15 kg seed/ha onto the appropriate plots and lightly covered with soil. At sowing, 100 and 50 kg/ha diammonium phosphate (DAP) and urea were broadcast onto the plots, respectively. Weeding and related management practices were carried out following the management practices.
3. Silage experiment
3.1. Treatment and design for laboratory evaluation of silage
The laboratory experiment to assess silage quality was set in a 3 harvesting stages × 4 additives (AD) in a factorial arrangement with 3 replications per treatment, making a total of 36 (Table ). The silage AD was without additives (WO), with 4% molasses (WM), 0.6% urea (fertilizer grade, 46% N) (WU), and both urea (0.6%) and molasses (4%) (WUM) in a completely randomized design. A 10-kg capacity plastic bag was used for each replicate. At each harvesting stage, Andropogon was immediately chopped into about 2–3 cm lengths using a manual chopper. In the replicate bag, 5 kg of the sample was weighed and treated with the respective AD treatment as a weighted % of the grass after chopping. To avoid viscosity, molasses was diluted with warm tap water. Urea was also dissolved with warm tap water at a ratio of 1:1 for a uniform distribution (Oladosu et al., Citation2016). When WM or WUM were applied, the amount of warm tap water used for dilution equals the amount of molasses used by weight (Suárez et al., Citation2011). For WUM, molasses was diluted first and urea was added to it and mixed thoroughly. Then the chopped grass was placed on a polyethylene sheet laid on a concrete floor, sprinkled with the respective AD treatment, and thoroughly mixed, after which the chopped grasses were filled into their plastic bag layer by layer, compacted, pressed with a wooden stick and immediately, the bags were tied carefully with rope and placed under ambient temperature in a room. After 21 days of ensiling, the bags were opened and subsamples from each bag were taken for the assessment of the physical quality, chemical composition, and fermentation characteristics of silages.
Table 1. Treatment layout
3.2. Preference and apparent digestibility study of silages
In these feeding experiments, testing all 12 treatment feed types was very challenging. So, only the boot stage of Andropogon (four treatments) was chosen based on a previous study in which the grass showed optimum DM yield and nutrient content before ensiling (Ribeiro et al., Citation2014). The levels of AD were exactly equal percentage and applied with the same procedure of ensiling as the laboratory experiment but the chopped grass was ensiled using 350 kg capacity plastic bags for each treatment.
3.3. Assessment of the physical quality of silages
The observation of mold formation and extent was done during the opening of the silos. After observation of the mold, by removing the top part of the silages, several hand-grabbed samples from the middle part of each bag (replication) were mixed thoroughly, and triplicate 500 g subsamples (from each) were taken where one sample was used for fermentative quality evaluation, one for physical characteristics assessment and one for chemical analysis.
For physical characteristics determination, 4 panelists were used for assessment by avoiding any possible pain, distress, or discomfort of assessors according to Hawassa University Research Ethics guideline. The panelists were from the Department of Livestock Research with different professional backgrounds, volunteers, and experienced in silage-making. They were trained practically on how to apply the criteria set (subjective score 1–4; Table ) and exercised independently before commencing the actual evaluation. Observation for mold formation was done starting from the opening of the silages, while color, smell, and texture were evaluated immediately after silo being opened . The visual observation for color assessment was also aided by standard color charts. Each individual scores for all attributes was used in the statistical analysis.
Table 2. Description of the scales used as indices of silage quality assessment
3.4. Fermentation characteristics
For pH determination, about 20 g of silage sample per replication was taken in a beaker to which 100 ml of distilled water was added (Piltz & Law, Citation2007). The samples were blended using a glass stirrer manually for 30 minutes and then kept for 1 hour and filtered through a cheesecloth. Then silage pH was measured from the extract using a conventional digital pH meter (PH-016, Graigar, China), calibrated with buffer solutions (pH 4, 7, and 9).
3.5. Chemical analysis of grass and silages
Before ensiling, from each harvesting stage, representative sub-samples of 300 g of chopped grasses and about 500 g of fresh silage sub-samples were taken from each bag (triplicates per treatment). Then to determine the DM content, the sub-samples were dried in a forced air drying oven (BOV-T50F, Biobase, Shandog, China) at 65°C for 72 hours, milled through a 1 mm screen (Thomas-Wiley, Philadelphia, USA), and stored in airtight plastic bags for chemical analysis (AOAC Association of Official Analytical Chemists, Citation1990). The total Nitrogen (N) and ash content of the samples was determined according to AOAC (Association of Official Analytical Chemists), Citation1990). The neutral detergent fiber (NDF), acid detergent fiber (ADF), and acid detergent lignin (ADL) were analyzed by Fibertec® (M 1020 Hot Extractor and 1021 Cold Extraction, Fibertec, Tecator, Hoganiis, Sweden) according to the procedure described by Van Soest and Robertson (Citation1985).
3.6. Preference for silages
Four intact male Arab goats (averaging 26 months of age) with an initial body weight of 25.32 ± 0.84 kg (mean ± SD) were selected from the Assosa Agricultural Research Center goat farm for the preference experiment of boot-stage silage using the AD treatments previously described in Table (Number 5, 6, 7, and 8). The experiment was carried out according to the guidelines of the Hawassa University Research Ethics guideline. The experiment was conducted for a total period of 23 days consisting of a 15-day adaptation period to the silage followed by 8 days of preference study. During the adaptation period, animals were vaccinated with lyophilized virus vaccine PPR virus strain cultured on VERO—cells 1 ml diluted in 100 ml of cool and sterile saline water for peste des petits ruminants (PPR) through subcutaneous injection at mid-neck region (NVI, Bishoftu, Ethiopia). And for the prevention of external parasites, we used 1.6 liters of acarmic for every 800 litter of water and sprayed using a knapsack sprayer (Amitraz 12,5%, Canelones, Uruguay). Moreover, the goats were dewormed with 1 bolus for 250 kg body weight broad-spectrum Albendazole 2500 mg (Albentong 2500, China), using a balling gun, against internal parasites as prescribed by the manufacturer. The experiment was conducted in the room by dividing it into four individual pens. Four feeding troughs (plastic boxes) were provided for each goat at each pen and each treatment silage was provided ad libitum (≈20% refusal) within a pen so that each goat had free access to the four silages and randomized the feed trough every day to avoid habit reflexes. A cafeteria feeding approach was used as described by Larbi et al. (Citation1993). No other feed was provided during the preference study.
The weight of the silages was determined 10 hours after the offer (8 am) to calculate the total DM intake. Silage offered and refused per goat was weighed and recorded daily and DM intake was determined for each animal for each silage. Water and mineral licks (common salt) were provided ad libitum. Representative samples of the offered silages were taken daily and dried at 105°C to determine the daily intake of DM for each animal. Finally, the DM intake data were used to determine the relative preference of the silage. The relative preference index (RPI) was calculated by dividing the intake of silage by the total intake of the most consumed silage and the results obtained were then multiplied by 100 (Larbi et al., Citation1993).
3.7. Apparent digestibility trial
After a preference study of the selected silage (Table : Number 5, 6, 7, and 8), 20 intact male Arab goats (average 23 months of age) with an initial body weight of 24.46 ± 1.05 kg (mean ± SD) were selected from the Assosa Agricultural Research Center goat farm to determine the apparent digestibility of experimental feed.
Randomized complete block design (RCBD) was used with four treatments consisting of five replications and four goats in a block which was based on initial live weight. Goats within a block were assigned to one of the four dietary treatments randomly. The treatment feeds were introduced gradually over the 2 weeks adaptation period and 3 days of adaptation to carrying the fecal collection bags. The animal care and management during the experimental period were the same as previously described in the preference study. After adaptation to fecal bags, the daily feed offered and refusal was measured and recorded. The daily total fecal output was also weighed and 20% was subsampled and stored at a temperature of −20°C in a medical freezer (MDF-235, Sanyo, Japan) for seven consecutive days. Silages were offered twice a day at 0800 and 1600 hours. Silage was provided to all animals ad libitum by adding a 20% allowance of the previous day’s intake. The experimental goats had free access to water and common salt licks. No other feed was provided except grass silage during the entire digestibility experiment.
On the last day of the collection period, fecal samples were thawed and thoroughly mixed, and 20% was subsampled from a fecal composite sample for each animal. Then the subsampled feed, refusal, and feces were dried at 60°C for 72 hours and ground to pass through a 1-mm sieve screen and stored in a plastic bag pending chemical analysis as previously described. Finally, the digestibility of DM and nutrient was calculated using the following equations:
3.8. Statistical analyses
The data were analyzed using the General Linear Model (GLM) and multivariate analysis of the R software (Version, R4.0.0). Treatment means indicating significant differences at the probability level of p < 0.05 were compared using Duncan’s new multiple-range test.
The following statistical models were used to analyze the data
Daily RPI values obtained for each treatment were subjected to analysis of variance with feeds as treatment and individual animals as replicates in a completely randomized design.
Response (silage physical, fermentative and chemical composition) as affected by harvesting stage and additives: Yijk = µ + Hi + Aj + HAij + eijk, Where; Yijk is the response variable, µ is the overall mean, Hi is the fixed effect of the HS, i= VEG, BT, and FB stage; Aj is the fixed effect of treatment AD, j= additives; HAij is the interaction of HS i and silage additive AD j and eijk is a random error.
Apparent digestibility trial includes diet and error effects: Yij = μ + ti + bj + eijk, where, Yij = is the response variable, μ= is the overall mean, ti= is the treatment effect, bj =is the block effect and eijk is the random error.
Multivariate analysis procedure of correlation through Agricolae R software package (version 1.3.5) a statistical procedure for agricultural research (De mandiburu, Citation2021), and FactoMineR (version 2.8) of an R Software Package for principal component analysis (Sebastien et al., Citation2008)
4. Results and discussion
4.1. Chemical composition of the grasses before ensiling
The chemical compositions of Andropogon grass harvested at different stages of maturity before ensiling is shown Table . The main challenge of the production of tropical grasses silages is the low moisture at harvest (Da Silva et al., Citation2019). Andropogon grass the DM% was greater (P < 0.05) without wilting and the DM content is outside of the optimal range for silage production (30% to 35% DM) during the full bloom stage of harvest. Only grass ensiled at the boot stage had DM content within these limits in our investigation. Higher value of DM was recorded in the 100% heading cutting stage of Andropogon, whereas lower DM was recorded before heading but ready to heading stage of cutting (Arega et al., Citation2020). An increase in ADF and a reduction in CP were observed as grass maturity increased in the current study. The findings are consistent withthe results of Ribeiro et al. (Citation2014) and Arega et al. (Citation2020), who discovered that the nutritional value of Andropogon decreases with physiological maturity. The CP concentration decreased dramatically from vegetative to full bloom in the current study. At the full bloom stage of harvest, CP concentrations was very low (P < 0.05), which is too low to sustain optimal activity by microorganisms for efficient ruminal fermentation (Van Soest, Citation1994). The present study showed that the CP content of Andropogon at the full bloom stage is lower than the value reported by Arega et al. (Citation2020), with an overall CP content of Andropogon is 6.66% obtained at different stages of cutting. The ADF and ADL contents increased (P < 0.05) from the vegetative to the full bloom stage in the current study. Another study also indicates the higher mean value of NDF, ADF, and ADL percentages 77.14%, 49.79%, and 8.56% respectively was recorded from Andropogon grass harvested before heading and at 10%, 25%, 50%, 75% and 100% heading (Arega et al., Citation2020).
Table 3. Nutrient composition (% DM) of the grass at different harvesting stages before ensiling
4.2. Andropogon silage physio-chemical properties
The effects of the harvesting stage and AD on the physical and fermentative characteristics of Andropogon grass silages are shown in Table . There was an interaction effect between the harvesting stage and AD on the smell, color, and pH of silage. The smell of WO (without additives) silages shows similarity (P > 0.05) with WUM (with urea plus molasses) at the vegetative and boot stages. But at the full bloom stage, a lower smell score (P < 0.05) was observed and AD had no effect compared to the WO silages. The lower score in physical attributes (smell, color, and moldiness) of silages at the full bloom stage may be related to the grass nature showing higher stem and dead materials proportion at the late stage of harvest which makes it difficult to pack during ensiling processes. A study by Ribeiro et al. (Citation2014) indicates a higher stem-to-leaf ratio (1.30) recorded on the regrowth of Andropogon gayanus harvested after 140 days.
Table 4. Andropogon grass silage physio-chemical properties in a different stage of harvest and additives
Silage with a pleasant smell was produced from WO and molasses-based additives (WM and WUM) during the vegetative and boot stages. But WU (urea additive) gave an offensive smell implying that urea might have created undesirable fermentation (growth of clostridia bacteria in the silage, organisms produce butyric acid). In contrast to our results, Mehtap et al. (Citation2007) observed that sorghum treated with the addition of 0.5%, 1%, and 1.5% of urea resulted in good, smell which indicates an excellent class.
The urea-based additives silages retain the original color (excellent scores) of the grass at the vegetative and boot stages. However, studies showed urea change in the color of silage from yellow to greenish yellow which may be attributed to the mode and extent of fermentation that occurred during ensiling (Saeed, Citation2012). In the current experiment, the full bloom stage of the grass has lower scores in color (dark) both with additives-based and WO silages. There was no significant difference among AD in the texture of the silages but silage at the boot stage had loose, soft, and firm texture. High (P < 0.05) moldiness was recorded at the full bloom stage of harvest and without AD silages, indicating that late harvesting and lack of AD can result in silage with moderate quality. The mold-free silage prepared during the vegetative compared to boot and full bloom indicates that the plant had higher leaves and soluble carbohydrates during these stages, which can easily be compacted during the process of silage making. Studies showed low level of lactic acid bacteria at late-stage silage of maturity which is responsible in improving silage fermentation and aerobic stability and reducing fungal growth (Ogunade et al., Citation2018)
The highest (P < 0.05) pH was observed at full bloom with WO, and with AD silages, while the lowest (P < 0.05) was recorded at the boot stage treated WM silage (Table ). This result is consistent with Ribeiro et al. (Citation2014) who found the pH of Andropogon silage was lower (pH = 4.6, 4.5, and 4.1) at the early stage harvested after 56, 84, and 82, respectively, as compared to the late stage (pH = 5.1) after 140 days of regrowth. Increasing forage DM content reduced lactic acid levels and increased silage pH without additives but not for additives-based silages (Da Silva et al., Citation2019). A study by Van Niekerk et al. (Citation2010) also indicated that the concentrations of water-soluble carbohydrates and buffering capacity of the late stage of Panicum maximum were lower than the vegetative stage. In this study, all harvesting stages of Andropogon silages prepared with WO had higher pH values (5.37) than the range suggested by Kebede Yadete (Citation2019) (3.7–4.5). This is probably due to the low WSC content of the Andropogon not being adequate for satisfactory fermentation without additives like molasses. In addition to this, WU-treated silage had also the highest pH value. This may be related to the production of ammonia released from urea being slightly basic, which could not restrain urease activity causing a delay in pH and had a pH value of more than 4.6 (Rong et al., Citation2013). Similar to the current study, high pH was observed in Napier grass ensiled with 0.4% urea, and 4% molasses with 0.4% urea leading to poor fermentation of silage (Rong et al., Citation2013). Another study also showed that silage made from urea treatment of Hypernia rufa grass had higher pH (6.5–7.9) due to higher buffering capacity of urea (Abebe et al., Citation2017). And Kebede Yadete (Citation2019) showed that the sole urea additive raised the pH significantly over untreated sugarcane top silage. However corn silage with urea additives had lower pH (3.8–4.09) but the hydrolysis of urea occurs in the first hours of the fermentation process, raising the corn silage pH and resistance to fast and intense acidification (Santos et al., Citation2020).
The result indicates that the pH of silage-treated WM was lower (P < 0.05) than WO, WU, and WUM. According to Chen et al. (Citation2020), molasses additive provides sufficient substrates for the rapid accumulation of lactic acid and sharp reduction of pH value. But in the current study, a mixture of urea and molasses did not improve the fermentation quality of the silage. This result is in line with Kebede Yadete (Citation2019) which indicates that when molasses was used in combination with urea it failed to significantly counteract the effect of urea alone to reduce the pH of silage from sugar cane tops. The result indicates that the addition of a soluble carbohydrate source improves the quality of silage and further additives are used to overcome the low content of sugars in Andropogon grasses.
4.3. Silages chemical composition
The effects of harvesting stages, AD, and their interaction on the chemical composition of silages are presented in Table . The result shows that with an increase in the harvesting stages, the DM, NDF, ADF, and ADL contents were increased (P < 0.05). There was no significant effect of silage AD on DM and ADL contents. The DM content of the silage increased with increasing stages of maturity and lost quality, which is consistent with the study by Xie et al. (Citation2012) who showed that DM increases with the delay of harvesting in tropical grasses. Harvesting Andropogon grass at a higher DM content may restricts compaction and fermentation, causing deterioration of silages and nutrient loss. Andropogon stem elongation with increasing maturity is consistent with a reduction in leafiness with increasing maturity (Ribeiro et al., Citation2014). The current result indicates DM content of the silage is not affected by AD. This result is contrary to the finding of Getahun et al.,(Citation2018) who indicated that addition of molasses increased the DM content of fermented green sugar cane tops silages as compared with control silage, or that treated with urea alone.
Table 5. Nutrient composition (% DM) of Andropogon silage
The Andropogon silage at the vegetative stage had higher (P < 0.05) CP content (Table ). Further, the experiment showed that the CP content of WO in the vegetative stages not difference (P > 0.05) from that of the additives-based silages (WM, WU, and WUM). Silage made from grass harvested at boot-stage and treated with WM and WU alone had higher (P < 0.05) CP content than that of the WO. Rong et al. (Citation2013) showed that the CP content determined based on oven-dried samples, could lead to the loss of volatile nitrogen compounds from urea-treated silage indicating that high ammonia might be lost during drying, while the mixture of urea and molasses improved fermentation quality and reduced the loss of ammonia. However, another study indicate that ensiling with urea-based additives (WU and WUM) resulted in higher CP content than the treatments without additive in sugar cane top silage (Getahun et al., Citation2018). In this study, the full bloom silages with AD had lower (P < 0.05) CP content than the vegetative and boot stages with AD silages. The CP content of the silage at the full bloom stage had lower than 60 g/kg DM, a level that is too low to sustain optimal activity by microbes for efficient ruminal fermentation (Van Soest, Citation1994).
The decrease in NDF of the silage compared with the NDF content before ensiling in the current study could probably be because hemicellulose was utilized for fermentation so as hemicellulose constitutes a portion of NDF, it was expected that NDF would also decrease during fermentation (Salamone et al., Citation2012). It has been reported that in WU treated silage the clostridial fermentation caused more loss in easily degradable nutrients than in structural carbohydrates, which indirectly increased the proportion of structural carbohydrates but the decrease structural carbohydrate with molasses-based additives might be attributed to the lower fiber contents of molasses, and enhancement of cell wall hydrolysis by increased organic acids production due to the sugars in molasses (Rong et al., Citation2013). A study by Bezabih and Tamir (Citation2014) also indicated molasses levels lowered pH, ADF, and NDF percentages and increased in vitro DM digestibility in Bermuda grass silages.
4.4. Preference and digestibility of Andropogon grass silages
4.4.1. Chemical composition of Andropogon silages
The lowest (P < 0.05) DM content was recorded in WO (Table ). The CP content was highest in both WM and WU. This result is in line the study by Getahun et al. (Citation2018) who indicated that the CP content of sugarcane top silage increased with urea addition, but not with molasses alone. While in fiber (NDF and ADL) content, there was no significant difference among the ADs. The highest (P < 0.05) ADF content was recorded in silage-made from WO treatment.
Table 6. The chemical composition (% DM basis) of the boot stage with different additives of Andropogon silage offered during preference and apparent digestibility study
4.5. Preference and apparent digestibility of silage
The highest (P < 0.05) DM intake and RPI was recorded for silage treated with WM followed by WUM, while the lowest (P < 0.05) was recorded in WO and WU during the preference study (Table ). Silage-treated WM was more preferred by indigenous goats, which indicates that the addition of molasses to Andropogon grasses silages enhanced its acceptability. Pratama and Jayanegara (Citation2021) indicated that feed consumption might be stimulated by higher residual water-soluble carbohydrate content in the molasses-treated silage. Silage prepared using urea additives was the least preferred and strongly avoided by Arab goats in cafeteria feeding. The low preference for silage WO and WU may be related to the end‐products of fermentation in silage that can affect the intake by animals through its effect on palatability (Keskin et al., Citation2005). And possibly the result of both sensory characteristics, especially due to a lower smell score associated with urea addition and negative post-ingestive feedback eventually derived from higher amine concentrations or other unidentified silage characteristics (Gerlach et al., Citation2014). This study indicates that the WO and the use of urea as an additive for Andropogon silage resulted in higher pH values which may contain large concentrations of undesirable compounds not preferred by goats which explains the low silage intake in these groups.
Table 7. Preference and apparent digestibility of Andropogon silage at the boot stage of harvest
The observed similarity in DM intake of WO silage and WM in the apparent digestibility study may be related to the smell of the WO silage . Compared to WM silages, WU silage had lower DM intake, which may be related to the influnce of silage fermentative profile on animal intake. This result contradicted with Bureenok et al. (Citation2012), who reported that the silage from elephant grass with the addition of molasses resulted in higher feed intake (1.4 times) than silage without any additional treatment. A study showed that the DM intake of dairy cattle was reduced by feeding a higher pearly millet silage diet due to an increase in acetic acid concentration and a change in the odor, taste, and texture of silage (Rodríguez et al., Citation2015). Goats in WM and WU digested more (P < 0.05) DM, OM, and CP compared to the other treatments, while the least (P < 0.05) was recorded in WO. The lowest digestibility of silages without the use of additives may be due to the lack of supply of energy from molasses and/or nitrogen from urea. But the high digestibility of CP for WM and WU treatment may be due to the high CP content in these two treatments. Silage treated with urea alone had the highest (P < 0.05) NDF digestibility.
Ensiling of Napier grass (harvested at the vegetative stage) with molasses resulted in increased in vitro DM digestibility coefficients (Keady et al., Citation2008). In addition, the increase in DM and OM digestibility for silages ensiled with molasses are in line with the observed lower NDF content of this silage. The improvement in the apparent digestibility of NDF in urea-treated silages shows that addition of urea to silages affects DM digestibility in the rumen positively. Ammonolysis causes lysis of the bonds between the structural carbohydrates releasing and increasing the contact surface with the rumen microorganisms (Da Silva et al., Citation2017).
4.6. Correlations between different grass silage composition factors
Among all investigated grass silage factors, texture had the greatest correlation to pH (r = −0.63, P < 0.05, Figure ). This is because the moisture content of the Andropogon silage material was too dry for optimal fermentation process. In a previous study by Fahmi et al. (Citation2019) silage texture was influenced by the water content of the material at the beginning of the silage process. The Andropogon silage showed a low moisture content (<30%) with increasing harvesting stage and a dry texture which affects the silage pH. The results also showed a strong positive correlation between the content of CP and the physical quality (color, texture, smell, and moldiness) of the silage (P < 0.05). The silage DM was negatively correlated with the color, moldiness, crude protein, and smell of silage (P < 0.05).
5. Principal component analysis
The principal component analysis grouped the 12 silage types over the four quadrants based on the physical characteristics, fermentative, and chemical composition measures (Figure ). The silage remained scattered in all four quadrants, showing large variability in silage characteristics. The silage at a vegetative stage with urea and molasses additives in the top-left quadrant was closely related to crude protein and moldiness content. The right top quadrant consisted of the silage with related contents of ADF and fermentation characteristics. The right bottom quadrant comprised the silage associated with the DM, NDF, and ADL content.
Figure 2. Biplot graph of principal component analysis for physical characteristics, fermentative, and chemical composition measures of silage harvested at different stages and treated with molasses and urea additives, NDF: neutral detergent fiber, ADF: acid detergent fiber. ADL: acid detergent lignin. Dim: Dimensionality Reduction. 1–12: the treatment number in Table .

6. Conclusion
This study demonstrated that good quality silage can be made from Andropogongrass in terms of acceptable smell, color, texture, and low moldiness at the vegetative and boot stages of harvest with the use of molasses or a mixture of molasses and urea. Undesirable pH was obtained with urea additive and control silage at all harvesting stages. The silage crude protein content at the boot stage was better with the addition of urea and molasses. Grass harvested at boot stage and treated with 4% molasses is the most preferred which shows the highest DM intake by Arab goats. The digestibility of the grass silages also improves due to the addition of molasses compared to silages without additives. The DM, OM, and CP were the lowest digestibility of silages without treatment but the highest when molasses and urea alone were used. In summary, the physicochemical characteristics and the nutrient composition indicate that Andropogon grass at the full bloom stage is not suitable for silage production. But, silage smell, texture, moldiness, color, fermentative characteristics, DM, and CP content, and their preference indicates harvesting at the boot stage and ensiling with 4% molasses is optimum for silage conservation practice in the study area. However, further research is needed which evaluates Andropogon grass silage harvested at the boot stage with 4% molasses additive for feeding Arab goats as basal feed.
Author contributions
AT: Conceptualization, Formal analysis, Investigation, Data collection, Visualization, Writing—Original draft, Writing—Review & editing; AN: Methodology, Validation, Supervision, Data Analysis, Writing, Review & editing; MB: Data analysis, Supervision, Writing, Review & editing;
Availability of data and materials
The datasets analyzed during the current study are available from the corresponding author on reasonable request
Ethical statements
The manuscript has not been published, nor is it under consideration by any other journal.
ABOUT THE AUTHOR.docx
Download MS Word (12.2 KB)Acknowledgments
This work was funded by the Ethiopian Institute of Agricultural Research. The authors thank the institute for its support in the study. And the authors have declared that no competing interests exist.
Disclosure statement
No potential conflict of interest was reported by the author(s).
Supplemental data
Supplemental data for this article can be accessed online at https://doi.org/10.1080/23311932.2023.2293516
Additional information
Funding
Notes on contributors

Alayu Tarekegn
Alayu Tarekegn completed his first Degree in Animal Science and Range land management from Debre Brihan University and a Master’s Degree in Animal Nutrition from Hawassa University, Ethiopia. He had been a researcher of feed and nutrition for six years at the Ethiopian Institute of Agricultural Research, and he has three years of expertise in forage science, tropical animal production systems, animal feeds, and nutrition, and the sustainability of livestock production systems in the Agricultural and Rural Development office. He has published more than seven articles in both national and international journals. He is now an Associate Researcher in the animal feed and nutrition program.
References
- Abebe, A., Alebachew, H., Faji, M., Almaw, D., & Tesfamariam, W. (2017). Effect of growth stage at harvest and type of additives on nutritional quality and fermentative characteristics of Hypernia rufa silage. African Journal of Basic & Applied Sciences, 9(5), 286–18.
- Ahmed, M. H., Babiker, S. A., Fadel Elseed, A. E. M. A., & Mohammed, A. M. (2013). Effect of urea-treatment on nutritive value of sugarcane bagasse. ARPN Journal of Science and Technology, 3(8), 834–838.
- AOAC (Association of Official Analytical Chemists). (1990). Official methods of analysis. Association of Official Analytical Chemists.
- Arega, H., Shitaneh, E., Getnet, M., & Worku, B. (2020). Determination of appropriate cutting time of perennial elite lowland adaptive forage grass species of dirk Ayifera/Andropogon gayanus/for optimum yield and quality of hay in Metekel Zone of Benishangul Gumuz. International Journal of Scientific Engineering and Science, 4(9), 41–46.
- Balehegn, M., Ayantunde, A., Amole, T., Njarui, D., Nkosi, B. D., Müller, F. L., Meeske, R., Tjelele, T. J., Malebana, I. M., Madibela, O. R., Boitumelo, W. S., Lukuyu, B., Weseh, A., Minani, E., & Adesogan, A. T. (2022). Forage conservation in sub‐Saharan Africa: Review of experiences, challenges, and opportunities. Agronomy Journal, 114(1), 75–99. https://doi.org/10.1002/agj2.20954
- Bernardes, T. F., Daniel, J. L. P., Adesogan, A. T., McAllister, T. A., Drouin, P., Nussio, L. G., Huhtanen, P., Tremblay, G. F., Bélanger, G., & Cai, Y. (2018). Silage review: Unique challenges of silages made in hot and cold regions. Journal of Dairy Science, 101(5), 4001–4019. https://doi.org/10.3168/jds.2017-13703
- Bezabih, M., & Tamir, B. (2014). Silage Additives: Review. Open Journal Applied Science, 4(5), 258–274. https://doi.org/10.4236/ojapps.2014.45026
- Bureenok, S., Yuangklang, C., Vasupen, K., Schonewille, J. T., & Kawamoto, Y. (2012). The effects of additives in napier grass silages on chemical composition, feed intake, nutrient digestibility and rumen fermentation. Asian-Australasian Journal of Animal Sciences, 25(9), 1248. https://doi.org/10.5713/ajas.2012.12081
- Chen, S. W., Chang, Y. Y., Huang, H. Y., Kuo, S. M., & Wang, H. T. (2020). Application of condensed molasses fermentation solubles and lactic acid bacteria in corn silage production. Journal of the Science of Food and Agriculture, 100(6), 2722–2731. https://doi.org/10.1002/jsfa.10304
- CSA (Authority central statistics). (2010). Ethiopian Agricultural sample enumeration. Addis Ababa.
- Da Silva, T. C., Da Silva, L. D., Santos, E. M., Oliveira, J. S., & Perazzo, A. F. (2017). Importance of the Fermentation to Produce High-Quality Silage. Fermentation Processes (pp. 1–20). IntechOpen Rijeka, Croatia. https://doi.org/10.5772/64887
- Da Silva, T., Santos, R., Santos, E., Rodrigues, J. P., & Rêgo, A. (2019). Challenges and perspectives of tropical grasses silages. Conference: VI International Symposium on Forage Quality and Conservation (ISFQC) at Piracicaba, SP.
- de Mendiburu, F. (2021). Agricolae: Statistical Procedures for Agricultural Research_. R package version 1.3-5.
- Fahmi, M., Utomo, R., & Umami, N. (2019, November). Physical and chemical quality of silage from two Pennisetum purpureum sp varieties supplemented with molasses at different levels. IOP Conference Series: Earth and Environmental Science, 387(1), 012059. https://doi.org/10.1088/1755-1315/387/1/012059
- Falola, O. O., Alasa, M. C., & Babayemi, O. J. (2013). Assessment of silage quality and forage acceptability of vetiver grass (chrysopogon zizanioides L. Roberty) ensiled with cassava peels by WAD goat. Pakistan Journal of Nutrition, 12(6), 529. https://doi.org/10.3923/pjn.2013.529.533
- Gerlach, K., Roß, F., Weiß, K., Büscher, W., & Südekum, K. H. (2014). Aerobic exposure of grass silages and its impact on dry matter intake and preference by goats. Small Ruminant Research, 117(2–3), 131–141. https://doi.org/10.1016/j.smallrumres.2013.12.033
- Getahun, K., Ashenafi, M., Getnet, A., & Getachew, A. (2018). Nutritional and fermentative quality of sugarcane (saccharum officinarum) top ensiled with or without urea and molasses. African Journal of Agricultural Research, 13(20), 1010–1017. https://doi.org/10.5897/AJAR2017.12888
- Getnet, M., Arega, H., Shitaneh, E., & Worku, B. (2020). Determination of appropriate cutting date of perennial elite lowland adaptive forage grass species: Chifir Bequa (Pennisetum Polystachion). International Journal of Scientific Engineering and Science (Online): 2456. 7361, 4(8), 1–3.
- Keady, T. W. J., Kilpatrick, D. J., Mayne, C. S., & Gordon, F. J. (2008). Effects of replacing grass silage with maize silages, differing in maturity, on performance and potential concentrate sparing effect of dairy cows offered two feed value grass silages. Livestock Science, 119(1–3), 1–11. https://doi.org/10.1016/j.livsci.2008.02.006
- Kebede Yadete, G., 2019. Sugarcane by products as feed resources and performance of sheep fed on sugarcane tops based diets [ Doctoral dissertation], Addis Ababa University.
- Keskin, B., Yilmaz, İ. H., Karsli, M., & Nursoy, H. (2005). Effects of urea or urea plus molasses supplementation to silages with different sorghum varieties harvested at the milk stage on the quality and in vitro dry matter digestibility of silages. Turkish Journal of Veterinary & Animal Sciences, 29(5), 1143–1147.
- Larbi, A., Osakwe, I. I., & Lambourne, J. W. (1993). Variation in relative palatability to sheep amongGliricidia sepium provenances. Agroforestry Systems, 22(3), 221–224. https://doi.org/10.1007/BF00705235
- Mehtap, G., Murat, D., & Sibel, C. (2007). Effect of urea plus molasses supplementation to sorghum silage on the quality, in vitro organic matter digestibility and metabolic energy contents. The Journal of Biological Sciences, 7(2), 401–404. https://doi.org/10.3923/jbs.2007.401.404
- Ogunade, I. M., Martinez-Tuppia, C., Queiroz, O. C. M., Jiang, Y., Drouin, P., Wu, F., Vyas, D., & Adesogan, A. T. (2018). Silage review: Mycotoxins in silage: Occurrence, effects, prevention, and mitigation. Journal of Dairy Science, 101(5), 4034–4059. https://doi.org/10.3168/jds.2017-13788
- Oladosu, Y., Rafii, M. Y., Abdullah, N., Magaji, U., Hussin, G., Ramli, A., & Miah, G. (2016). Fermentation quality and additives: A case of rice straw silage. BioMed Research International, 2016, 1–14. https://doi.org/10.1155/2016/7985167
- Ososanya, T. O., & Olorunnisomo, O. A. (2015). Silage characteristics and preference of sheep for wet brewer’s grain ensiled with maize cob. Livestock Research for Rural Development, 27(1). http://www.lrrd.org/lrrd27/1/osos27012.htm
- Pang, D., Yan, T., & Krizsan, S. (2021). Effect of strategy for harvesting regrowth grass silage on performance in dairy cows. Journal of Dairy Science, 104(1), 367–380. https://doi.org/10.3168/jds.2020-18888
- Piltz, J., & Law, D. (2007). AFIA-laboratory methods manual. Australian Fodder Industry Association Inc Balwyn Victoria.
- Pratama, A., & Jayanegara, A. (2021, May). Nutrient digestibility of dairy cows fed with chopped and ensiled elephant grass. IOP Conference Series: Earth and Environmental Science, 756(1), 012061. https://doi.org/10.1088/1755-1315/756/1/012061
- Ribeiro, G. O., Jr., Teixeira, A. M., Velasco, F. O., Faria, W. G., Pereira, L. G. R., Chaves, A. V., Gonçalves, L. C., & McAllister, T. A. (2014). Production, nutritional quality and in vitro methane production from Andropogon gayanus grass harvested at different maturities and preserved as hay or silage. Asian-Australasian Journal of Animal Sciences, 27(3), 330–341. https://doi.org/10.5713/ajas.2013.13161
- Rodríguez, A. A., Luciano, D., Galván, M., & Solórzano, L. C., 2015. Effects of aerobic exposure on fermentation end-products of corn and sugarcane silages and intake by goats.In: Proceedings of the XVII International Silage Conference. Piracicaba, São Paulo, Brazil.
- Rong, H., Yu, C., Li, Z. H., Shimojo, M., & Shao, T. (2013). Evaluation of fermentation dynamics and structural carbohydrate degradation of napiergrass ensiled with additives of urea and molasses. Pakistan Veterinary Journal, 33(3), 374–377.
- Saeed, A. A. (2012). The effect of addition urea and ensiling period on the quality and chemical composition of wheat straw silages. Al-Qadisiya Journal for Agricultural Sciences, 2(2), 1–14. https://doi.org/10.33794/qjas.2012.68150
- Salamone, A. M., AbuGhazaleh, A. A., & Stuemke, C. (2012). The effects of maturity and preservation method on nutrient composition and digestibility of master graze. Journal of Animal Research and Technology, 1(1), 13–19. https://doi.org/10.5147/jart.v1i1.104
- Santos, A. P. M. D., Santos, E. M., Araújo, G. G. L. D., Oliveira, J. S. D., Zanine, A. D. M., Pinho, R. M. A., Cruz, G. F. D. L., Ferreira, D. D. J., Perazzo, A. F., Pereira, D. M., & Santos, F. N. D. S. (2020). Effect of inoculation with preactivated lactobacillus buchneri and urea on fermentative profile, aerobic stability and nutritive value in corn silage. Agriculture, 10(8), 335. https://doi.org/10.3390/agriculture10080335
- Sebastien, L., Josse, J., & Husson, F. (2008). FactoMineR: An R package for multivariate analysis. Journal of Statistical Software, 25(1), 1–18. https://doi.org/10.18637/jss.v025.i01
- Suárez, R., Mejía, J., González, M., García, D. E., & Perdomo, D. A. (2011). Evaluation of mixed silages of saccharum officinarum and gliricidia sepium using additives. Pastures and forages, 34(1), 69–85. http://ref.scielo.org/kft4qd
- Van Niekerk, W. A., Hassen, A., & Bechaz, F. M. (2010). Influence of growth stage at harvest on fermentative characteristics of Panicum maximum silage. South African Journal of Animal Science, 40(4), https://doi.org/10.4314/sajas.v40i4.65251
- Van Soest, P. J. (1994). Nutritional ecology of the ruminant. Cornell university press.
- Van Soest, P. J., & Robertson, J. B. (1985). Analysis of forage and fibrous feeds: A laboratory manual for animal sciences 613. Cornell University, Ithaca.
- Xie, Z. L., Zhang, T. F., Chen, X. Z., Li, G. D., & Zhang, J. G. (2012). Effects of maturity stages on the nutritive composition and silage quality of whole crop wheat. Asian-Australasian Journal of Animal Sciences, 25(10), 1374. https://doi.org/10.5713/ajas.2012.12084