Abstract
The present study aimed to investigate the in-vitro antidiabetic activity of collagen hydrolysate (CH) from Asian bullfrog skin and its application as a bioactive compound in dark chocolate bars. Papain (3% [w/w]) was used to obtain CH with a simultaneous conjunction of ultrasound. CH possessed α-amylase inhibitory activity with an IC50 value of 3.60 mg/mL, while results from inhibition reaction kinetics revealed that CH inhibited α-amylase in a non-competitive mode. Different concentrations (0.5, 1.0, and 2.0% [w/w]) of CH were then fortified into dark chocolate bars, and the physicochemical characteristics, breaking strength, and rheological behaviour of the resulting chocolate were investigated and compared with a control (without CH addition). A higher level of CH significantly enhanced antidiabetic activity against α-amylase (p ≤ 0.05) in chocolate bar. Chocolate bar with 2% CH complied with the nutritional claim requirement for ‘source of protein’ with lower fat content and energy value, while sensory evaluation revealed good organoleptic properties.
REVIEWING EDITOR:
Introduction
The global chocolate market has experienced major growth and is valued at more than $87.5 billion (Gallo et al., Citation2018). In the United States alone, the five-year retail market projection for chocolate has been estimated to be worth at least $19 billion. The US chocolate market is significantly influenced by “craft” or “bean-to-bar” chocolate, which has drawn the attention of multinational confectionary companies for further business partnerships (Brown et al., Citation2020). Craft chocolate has been categorized as premium chocolate based on its distinctive flavour attributes, quality, and origin of the cacao (Theobroma cacao L.) used (Cadby et al., Citation2021). As a functional food, chocolate has health-beneficial features, including in vitro antioxidant and anti-inflammatory properties, in addition to lowering the risk of cardiovascular disease rand regulating blood pressure (Oliveira et al., Citation2022; Shah et al., Citation2017; Valussi & Minto, Citation2016). However, owing to its high sugar content, excessive consumption of chocolate increases the risk of being overweight and having diabetes. Therefore, some chocolate products have been created as sugar-free, fat-reduced, and/or calorie-reduced products for people with diabetes and other health concerns using sweeteners or fat replacers (Aidoo et al., Citation2017; Bahari & Akoh, Citation2018; Mohammadi et al., Citation2022; Oliveira et al., Citation2022; Shah et al., Citation2017). Nevertheless, a reduction in the calorific content of foods, containing sugar and/or fat, along with other food additives may compromise certain essential qualities (Auerbach & Dedman, Citation2012).
Collagen hydrolysates (CHs) have been extracted from various marine and terrestrial animal sources and possess excellent physicochemical and bioactive properties (i.e. antioxidant, antimicrobial, anti-inflammatory, and antidiabetic) (Offengenden et al., Citation2018; Rabiei et al., Citation2019; Rahimi et al., Citation2022; Zamorano-Apodaca et al., Citation2020; Zhang et al., Citation2020). Recent studies have shown that CH from yellowfin tuna, a wild marine fish, exhibits antidiabetic activity (Harris et al., Citation2021; Natsir et al., Citation2019). The Asian bullfrog (Rana tigerina) has been widely utilized for human consumption in North Africa and Southeast Asia (Sai et al., Citation2001), where the frog farming and its culture have been extensively studied (Diesmos et al., Citation2004). According to Karnjanapratum and Benjakul (Citation2020), the collagenous derivative obtained from the skin of Asian bullfrog showed no cytotoxicity to all cell lines tested (Raw264.7 (epithelial), Caco-2 (macrophage), and L929 (fibroblast)) at concentration ranging from 100 to 800 μg/mL. Previously, CH prepared from Asian bullfrog skin (Rana tigerina) with simultaneous ultrasound assistance displayed superior antioxidant activity against free radicals (Indriani et al., Citation2022). Because of these activities, Asian bullfrog skin CHs may be used effectively as multifunctional additives in both the food and pharmaceutical industries (Zamorano-Apodaca et al., Citation2020). However, there is still limited information regarding its bioactivity, especially its antidiabetic activity, and its potential as a functional food additive in food systems. Therefore, this study aimed to evaluate the antidiabetic activity of CH from Asian bullfrog skin, in which its application as a bioactive compound in a food model system was studied by fortifying dark chocolate bars. The α-amylase inhibitory activity and its reaction kinetics of CH were conducted to study inhibition modes. Dark chocolate bars with different CH concentrations were characterized for their antidiabetic activity and physicochemical, thermal, and rheological properties. A sensory evaluation and nutritional value of the selected antidiabetic chocolate bars were also determined.
Materials and methods
Materials
Cocoa (T. cacao) was supplied by a local farm (Thung Song Cocoa Community Enterprise, Thung Song, Nakhon Si Thammarat, Thailand). Cocoa butter (Chocolásia, Bangkok, Thailand), powdered sugar (Lin, Thai Roong Ruang Sugar Group, Bangkok, Thailand), soy lecithin (Krungthepchemi Co. Ltd., Bangkok, Thailand), and vanilla powder (Best Foods, Upfield Co. Ltd., Bangkok, Thailand) were food grade and purchased from a local baking shop in Ladkrabang, Bangkok, Thailand. Asian bullfrog (R. tigerina) skin was procured from a local market in Ladkrabang, Bangkok, Thailand. Papain from Carica papaya latex (EC 3.4.22.2, 3,000 U/g) and porcine pancreatic α-amylase (EC 3.2.1.1, 13 U/mg) was purchased from Sigma–Aldrich (St. Louis, MO, USA). All chemicals used in this study were of analytical grade.
Preparation of collagen hydrolysate
CH from Asian bullfrog skin was prepared according to the method described by Indriani et al. (Citation2022). Prior to hydrolysis, the skins were pretreated with a 0.3 M NaOH solution, followed by swelling with 1.0 M acetic acid. The swollen skins were conditioned by soaking in distilled water at a ratio of 1:5 (w/v) at 40 °C for 60 min. Thereafter, 3% (w/w) papain was added to the suspension to obtain CH. Hydrolysis was carried out using an ultrasound instrument (Sonics, Model VC750, Sonica & Materials, Inc., Newton, USA) coupled with a flat-tip probe (∅ 25 mm), under simultaneous mode (80% amplitude, 20 kHz, 750 W, 5-s active and 5-s resting pulse mode, 40 °C, 30 min) and the total hydrolysis time was 2 h. The reaction was terminated by heating the mixture at 90 °C for 20 min followed by centrifugation (9,000 rpm, EppendorfTM 5920 R, Thermo Fisher Scientific Inc., Massachusetts, USA) at 4 °C for 30 min. The supernatant was collected and lyophilized using a freeze-dryer (Scanvac CoolSafe 55–4 Pro, Labogene, Denmark). The CH was stored at –20 °C and used within one month.
In vitro antidiabetic assay
α-Amylase inhibitory activity
The α-amylase inhibitory activity of CH was determined according to the method of Ademiluyi et al. (Citation2015), with minor modifications. Briefly, 200 μL of the CH solution (0–50 mg/mL) was mixed with 500 μL of 0.02 M sodium phosphate buffer (pH 6.9, 0.006 M NaCl) containing porcine pancreatic α-amylase (EC 3.2.1.1, 1 mg/mL), and incubated at 25 °C for 10 min. Then, 500 μL of 1% (w/w) starch solution in 0.02 M sodium phosphate buffer (pH 6.9 with 0.006 M NaCl) was added, followed by incubation at 25 °C for 10 min. The reaction was terminated by adding 1.0 mL of dinitrosalicylic acid colour reagent, then incubated in a boiling water bath for 5 min followed by cooling to 26–28 °C. The reaction mixture was then diluted with 5 mL of distilled water, and optical absorbance was measured at 540 nm using a spectrophotometer (UV-1200, Shimadzu, Japan). The results are expressed as percentages of enzyme inhibition and plotted against sample concentrations. The CH concentration providing the greatest inhibitory effect within the initial velocity (2.88 mg/mL) was selected for further study.
α-Amylase activity inhibition reaction kinetics
The kinetics of α-amylase activity were determined using the Lineweaver-Burk equation. The concentration of the starch substrate was varied (0.125, 0.25, 0.5, 0.75, 1, 1.5, and 2% [w/v]), and the concentrations of enzyme and CH were fixed at 1 mg/mL and 2.88 mg/mL, respectively. Reactions were performed at 25 °C for 10 min. After reaction termination, following the method described above, the optical absorbance of the reaction mixture was measured at 540 nm. A double reciprocal curve was drawn by plotting 1/V against 1/[S], where V and [S] represent the reaction rate and substrate concentration, respectively.
Development of dark chocolate bars with CH at different addition levels
Preparation of dark chocolate bar
Dark chocolate bars were prepared according to the method described by Fibrianto et al. (Citation2021) with modifications. The ingredients of the dark chocolate bars were cocoa (88 g), cocoa butter (8 g), powdered sugar (52.8 g), lecithin (1 g), and vanilla powder (0.34 g). Briefly, the cocoa mass was ground into a paste using a kitchen mill chopper (HR2223/01, ProBlend Crush 700 W, Philips, Netherlands), followed by the addition of powdered sugar for a total milling time of 10 min. CH, cocoa butter, lecithin, and vanilla powder were then added to the mixture and blended for an additional 5 min. The chocolate blend was then tempered (45–50 °C) in a water bath (WNB 45, Memmert GmbH + Co. KG, Schwabach, Germany). The temperature was gradually reduced to 28–29 °C followed by immediate heating to 32 °C. The tempered chocolate blend was poured into a polycarbonate mold (27.5 × 13.5 × 2.5 cm3) and allowed to solidify in a refrigerator at 10 °C for 60 min. Thereafter, the chocolate bar was removed from the mold and packed in a zip-lock aluminium foil bag (12 × 18 cm2). The resulting chocolate bars were then conditioned in an air-conditioned room at 25 °C for 24 h prior to characterization, which was done within seven days. Chocolate bars prepared with different levels of CH addition at 0, 0.5, 1.0, and 2.0% ([w/w], based on the total weight of chocolate blend) were referred to as ‘Control’, ‘C-0.5’, C-1.0’, and ‘C-2.0’, respectively.
Characterization
Moisture content (Mc), water activity (aw), and colour. The Mc of the chocolate bars was determined using a hot-air oven following the AOAC method and expressed as a % (w/w) wet basis (AOAC Citation2012). The aw was measured using an aw-meter (AQUA–LAB 4TEV, Decagon Devices, USA). The colour of the chocolate bars was determined using a colourimeter (CR-400 Chroma Meter, Konica Minolta Sensing America, Inc., USA). The colour was measured as L* (lightness), a* (redness), and b* (yellowness) (Fibrianto et al., Citation2021).
Breaking strength. Hardness was measured using a texture analyser (TA.XT.plus®, Texture Technologist Corp., USA) with a three-point bend rig, according to the method of Bahari and Akoh (Citation2018), with slight modifications. Briefly, samples were conditioned by holding them at 20 °C for 1 h prior to measurement. The parameters were set for pre-test speed (1 mm/s), test speed (1.1 mm/s), post-test speed (10 mm/s), distance (10 mm), load cell (50 kg), and trigger force (20 g). The breaking strength was calculated as the ratio of hardness to distance (kg/cm).
Rheological behaviour
The rheological behaviour of the chocolate bar samples was evaluated using a rheometer (Anton Paar, MCR302, Graz, Austria) according to the method described by Ashrafie et al. (Citation2014) with a slight modification. A double-gapped concentric cylinder with a radius ratio of 1.035, an internal gap of 0.43 mm, an external gap of 0.47 mm and a gap of 1 mm between the rotational cylinder and the bottom of the cup, was used. Briefly, the sample was melted at 50 °C for 60 min then transferred to the cup. The molten sample was cooled to 40 °C. The rheological behaviour was evaluated through four segments: (1) pre-shearing for 500 s at 5 s−1 to homogenize and control the temperature of the sample; (2) shear rate ramp of 2 s−1 to 50 s−1 with 18 points in 180 s; (3) constant shearing at 50 s−1 for 60 s; and (4) shear rate ramp of 50 s−1 to 2 s−1, duration 180 s. The results were analysed using Rheoplus/32 v.3.21 software (Anton Paar). Rheological behaviour was measured in terms of plastic viscosity (Pa.s), yield stress (Pa), flow behaviour index, apparent viscosity (Pa.s), and thixotropy (Pa.s−1). Viscosity at a shear rate of 40 s−1 was considered the apparent viscosity, and differences in viscosity during ramp-up and ramp-down were determined as thixotropy.
Thermal behaviour
The thermal behaviour of the dark chocolate bars was evaluated using differential scanning calorimetry (DSC2, PerkinElmer, Norwalk, CA, USA), according to the method described by Joshi et al. (Citation2020), with a slight modification. The chocolate bar was incubated in the 40 μL aluminium crucible (8 mg) at 22 °C for 24 h before measurement. An empty aluminium crucible was used as a reference cell. Nitrogen gas (30 mL/min) was used for cooling. The experiment consisted of four segments employing isothermal and dynamic processes. The sample was heated to 90 °C and maintained there for 20 min. Thereafter, the sample was cooled to –50 °C followed by immediate reheating to 90 °C before the next segment. The heating and cooling rates were set at 5 °C/min and evaluation of graphs was performed using STARe software.
α-Amylase inhibitory activity of dark chocolate bars
The α-amylase inhibitory activity of the dark chocolate bars was determined as described in ‘α-amylase inhibitory activity’ section. Chocolate bar samples (1 g) were melted and homogenized in distilled water (5 mL) at 40 °C for 15 min. Thereafter, this suspension was used for subsequent assay, following the method described above. The results are expressed as percent inhibition of α-amylase activity.
Based on essential physicochemical characteristics and α-amylase inhibitory activity, the dark chocolate bar added with CH was then selected for further characterization, in comparison with those of a control.
Nutritional composition and energy value
Moisture, protein, fat, ash, and total carbohydrate contents of the selected chocolate were determined following AOAC methods and expressed in % wet basis (AOAC Citation2012). The energy value was calculated using the Atwater system (Merrill & Watt, Citation1955).
Sensory evaluation
Sensory evaluation of the selected dark chocolate bar samples was conducted according to the method described by Mohammadi et al. (Citation2022) with slight modifications. Prior to evaluation, potential panellists were screened to select those who were familiar with dark chocolate bars. Briefly, the samples were labelled with a three-digit random number, which was then presented along with a questionnaire, to the 30 panellists. The panellists were asked to evaluate the sensory attributes of the samples (appearance, colour, taste, mouthfeel, sweetness, hardness, texture, aftertaste, and overall acceptance). A hedonic liking score of 5-point was used for the evaluation (1: disliked very much, 2: disliked moderately, 3: neither liked nor disliked, 4: liked moderately, 5: liked very much). The panellists were instructed to refresh their palates between each sample evaluation with drinking water at room temperature.
Statistical analysis
The experimental design in this study was a completely randomized design (CRD), except for the sensory evaluation, for which a randomized complete block design (RCBD) was used. Experiments were conducted in triplicate, and one-way analysis of variance (ANOVA) was performed. Duncan’s multiple range test and independent t-test were used to compare means between samples and between two samples, respectively. All statistical analyses were performed using SPSS statistical software (SPSS Statistics, IBM, NY, USA). Statistically, significant differences in all comparisons were reported at p ≤ 0.05.
Results and discussion
α-Amylase inhibitory activity of CH
The in vitro antidiabetic activity of CH was evaluated using α-amylase inhibitory activity assays (). α-Amylase is a calcium metalloenzyme secreted by salivary glands and the pancreas, that is essential in breaking down polysaccharides into glucose and maltose, leading to an elevation of postprandial blood glucose levels (Tiwari et al., Citation2015). Inhibition of α-amylase activity prompts a delay in carbohydrate digestion and hydrolysis, resulting in lower blood glucose levels and control of diabetes (Kaur et al., Citation2021). Therefore, before using CH as a functional food additive, it is necessary to study the potential inhibitory activity of CH against α-amylase and its inhibitory mechanism by enzymatic kinetics. The inhibitory activity of CH against α-amylase was gradually increased in a dose-dependent manner (0.4–23.0 mg/mL of CH) ((a)). Based on suppression curve calculations, the IC50 value (half of maximum inhibitory concentration) of CH in terms of α-amylase inhibition was 3.60 ± 0.32 mg/mL. There have been no previous reports regarding the antidiabetic activity of CH obtained from frog skin, particularly against α-amylase. In contrast, bioactive peptides from frog skin secretions such as brevinins, esculentins, magainin, ranateurins, temporins, and tigerinins have been reported to exhibit antidiabetic activity by enhancing the release of insulin and lactate dehydrogenase in vitro (Indriani et al., Citation2023; Soltaninejad et al., Citation2021). This result was comparable with previous a report on α-amylase inhibitory peptide fractions isolated from the Chinese giant salamander that showed IC50 values ranging from 2.9 to 13.8 mg/mL (Ramadhan et al., Citation2017).
Figure 1. Inhibitory effect of collagen hydrolysate on α-amylase. (a) α-amylase inhibitory activities of collagen hydrolysate. (b) Lineweaver–Burk plot for determining the kinetic constants for α-amylase.
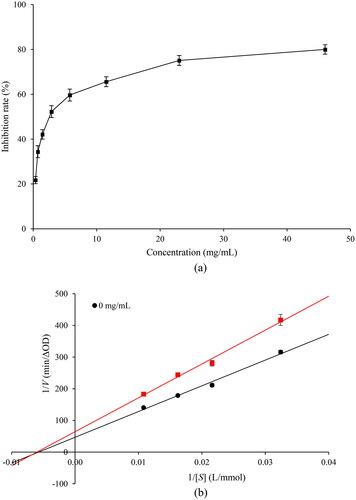
The mechanism of action of CH on α-amylase was evaluated using the double-reciprocal Lineweaver-Burk kinetic curve ((b)). Based on the linear relationship between the substrate and CH concentrations (Michaelis-Menten plot), the intersecting lines along the x-axis and intersections on the y-axis corresponded to the Km and Vmax, respectively. In the absence of CH, the Km and Vmax of α-amylase were 173.39 mmol/L and 0.021 ΔOD/min, respectively. The presence 2.88 mg/mL CH was found to reduce Vmax to 0.016 ΔOD/min, whereas Km remained unchanged. This indicated that CH possessed a non-competitive inhibitor for α-amylase. Non-competitive inhibition explains the peptide (CH) binding at α-amylase sites other than the active site without competing with the substrate, whereas both enzyme-CH and enzyme-substrate-CH complexes could be enzymatically inactive. Nevertheless, the substrate could still bind to the enzyme with unchanged affinity for the substrate thus possibly create an unstable conformation (Awosika & Aluko, Citation2019). The type of protease governed various hydrolysis patterns with different molecular weight of protein hydrolysate, thus influenced its bioactivity in food matrices (Karimi et al., Citation2021; Rivero-Pino, Citation2023). The molecular weight of CH used in this study was ranging from 20–110 kDa (Indriani et al., Citation2022). Moreover, the length and proportion of alkyl side chains present in amino acids (i.e. Val, Ala, Leu, Iso, and Phe) are essential for the polarity of proteins owing to their inhibitory activity. The use of papain hydrolysis in conjunction with simultaneous ultrasonication produced CH containing approximately 34.6% hydrophobic amino acids (Indriani et al., Citation2022). Similarly, peptides derived from oat meal (NINAHSVVY) containing 44.4% hydrophobic amino acids inhibited α-amylase at an IC50 of 67.3 μM. Furthermore, the presence of Ser in the last two positions of the N-terminus in antidiabetic peptides from soft-shelled turtle egg (SGTLLHK) possessed antidiabetic activity at a lower inhibition (IC50: 289 μM), especially against α-glucosidase (Berraquero-García et al., Citation2023). It has been reported that interactions between the aromatic chain of α-amylase and CH (by hydrogen bonds, electrostatic interactions, and Van der Waals interactions) could also hinder inhibitory activity (Gong et al., Citation2020). Branched amino acids (such as Lys, Phe, Tyr, and Trp) and cationic residues in CH can potentially bind to α-amylase (Arise et al., Citation2019; Indriani et al., Citation2022). Similarly, a non-competitive inhibition pattern against α-amylase was also reported for protein hydrolysates from red seaweed laver and watermelon seeds (Admassu et al., Citation2018; Arise et al., Citation2016). Therefore, CH could be considered a potential antidiabetic agent exhibiting non-competitive inhibition of α-amylase for further applications in dark chocolate bars.
Characteristics of dark chocolate bars with addition of CH
The physicochemical characteristics of dark chocolate bars prepared by adding different concentrations of CH from Asian bullfrog skin were investigated, including the moisture content (Mc), water activity (aw), and colour (). Under the processing conditions used in the present study, it was noted that dark chocolate bars fortified with CH at higher than 2.0% (w/w) could not form a homogenous molten chocolate blend without lumps of CH, resulting in under-tempered chocolate bars (data not shown). Mc contributes to the texture of chocolate bars as well as to various properties, including their shelf-life (Aidoo et al., Citation2015). Mc (1.27–1.41 g/100 g) increased in a dose-dependent manner with increasing CH levels (p ≤ 0.05). This was plausibly due to the presence of hydrophilic groups in CH, which could trap or hold water in chocolate bar. Water could be lost during chocolate bar preparation and bound to CH (Aidoo et al., Citation2015). This result was in accordance with the aw of CH fortified chocolate bars. An increase in aw was found with increased CH levels. Nevertheless, at ≥1.0% (w/w) CH, C-2.0 presented an aw–value that was higher than C-1.0. This most likely indicates that moisture in the CH itself increases the Mc of chocolate bars increasing their water content (Ashrafie et al., Citation2014; Konar et al., Citation2018; Mohammadi et al., Citation2022). The aw is important in determining the shelf-life of chocolate bars, with a higher aw leading to a shorter shelf-life.
Table 1. Characteristics of dark chocolate bars prepared with collagen hydrolysate from Asian bullfrog skin at different addition levels.
The colours of chocolate bar samples were evaluated for lightness (L*), redness (a*), and yellowness (b*) (). All samples displayed a distinctive dark brown colour with a shiny appearance (). However, a white spot on the chocolate bar surface was observed only in the C-0.5 sample. This suggests the occurrence of chocolate blooms because of the low stability of the emulsion system in the chocolate bar. With emulsion activity (EAI) and emulsion stability index (ESI) at 17.34 m2/g and 11.32 min, respectively, CH is a good emulsifier (data not shown). With a comparable Mc as the CH-added samples, C-0.5 contained the lowest CH, which might not be sufficient to act as an emulsifying agent to stabilize water droplets in the emulsion system. The cocoa mass strongly contributes to the brown colour, which is a combination of a* (red–green) and b* (yellow–blue) (Nizori et al., Citation2021). All samples showed a similar appearance, with no significant difference in chromatic colour (data not shown). The control sample displayed the darkest appearance (L*: 27.45), with the highest intensity of redness (a*: 8.34) and yellowness (b*: 6.03). Notably, the addition of CH to chocolate bars resulted in a lighter colour (L*: 30.62–30.82) than that of control bars, regardless of the amounts of added CH. This might be due to the dilution effect of the CH fortification. The yellowish-white colour of the CH powder used in this study (L*: 87.14, a*: 0.68, b*: 12.04) reduced the cocoa mass concentration in the chocolate bar to a lighter colour. In addition, redness and yellowness decreased with a decrease in a* and b* values. However, many processes and reactions, such as homogenization, tempering, and Schiff base formation, were plausibly affected by CH addition (Afoakwa & Afoakwa, Citation2016; Praseptiangga et al., Citation2019). Different amounts of CH addition may have different effects on these phenomena. Nevertheless, these colour changes did not result in a significant difference in the appearance of the chocolate, regardless of the CH amounts used (). This result was comparable to a previous report, in which the colour of dark chocolate bars was 25.44–27.40, 8.02–8.63, and 4.38–5.32 for L*, a*, and b* values, respectively, after the addition of cinnamon bark oleoresin microcapsules (Praseptiangga et al., Citation2019). In addition, the colour changes of the fortified chocolate were acceptable to consumers. Therefore, it is possible to produce chocolate bars that are fortified by CH addition while maintaining colour and appearance of regular chocolate bars.
Figure 2. Appearance of dark chocolate bars prepared with collagen hydrolysate from Asian bullfrog skin at different addition levels. Control: Dark chocolate bar prepared without collagen hydrolysate addition. C-0.5, -1.0, -2.0: Dark chocolate prepared with collagen hydrolysate addition at 0.5, 1.0, and 2.0% (w/w), respectively.
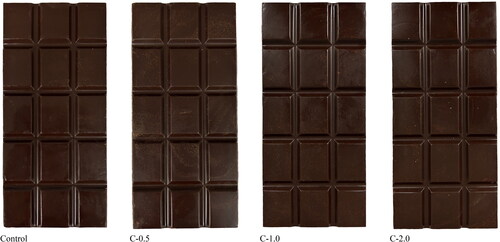
Textural properties
Texture is the combination of the physical structure of a material and its mechanical surface properties. Textural changes, such as an increase in hardness, affect the melting profiles of chocolate bars, lead to a prolonged melting time (Nightingale et al., Citation2009). The breaking strength (BS) is a key textural property of chocolate that is predominantly influenced by the type of fat, its content, type of sweetener, particle size distribution, crystal structure, and polymorphisms of cocoa butter (CB) (Afoakwa & Afoakwa, Citation2016). shows the BS values of the dark chocolate bars. BS increased significantly in a dose-dependent manner with an increase in CH levels (p ≤ 0.05). This was in accordance with the increase in Mc and the increase in CH amount (). This process changes the proportion of Mc and fat in the chocolate systems, which strongly governs the phase transition that occurs during emulsion crystallization (Ashrafie et al., Citation2014). CH might surround water droplets, directly impacting the polymorphic evolution and changing the structural re-arrangement of CB triglycerides (di Bari et al., Citation2017). Different formulations of chocolate bars could possibly change their emulsion systems. In addition, a proportional change in moisture and fat content is attributed to particle size distribution and rheological properties, thus affecting the textural properties of chocolate bars (Caparosa & Hartel, Citation2020; Feichtinger et al., Citation2020; Mohammadi et al., Citation2022). Similar results were found for reduced-fat and sugar-free chocolate bars using various CB (CH and cinnamon bark oleoresin microcapsule) and sucrose replacers (inulin and polydextrose), respectively (Aidoo et al., Citation2017; Ashrafie et al., Citation2014; Mohammadi et al., Citation2022; Praseptiangga et al., Citation2019).
Figure 3. Breaking strength of dark chocolate bars prepared with collagen hydrolysate from Asian bullfrog skin at different addition levels. Control: Dark chocolate bar prepared without collagen hydrolysate addition. C-0.5, -1.0, -2.0: Dark chocolate prepared with collagen hydrolysate addition at 0.5, 1.0, and 2.0% (w/w), respectively. Different letters on the bars indicate significant differences between the samples (p ≤ 0.05).
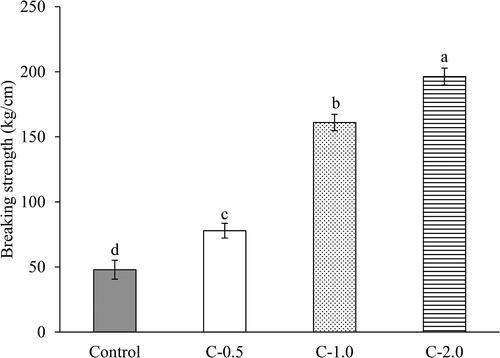
Rheological behaviour
The rheological properties of molten chocolate correspond to the manufacturing and product quality of chocolate bars (Taylor et al., Citation2008). As shown in , higher levels of CH addition increased the plastic viscosity, yield stress, flow behaviour index, apparent viscosity, and thixotropy in a dose-dependent manner (p ≤ 0.05). The plastic viscosity (η) increased from 5.30 to 14.46 Pa.s. CH addition proportionally lowered the free fat content in the system, thus hindering the flowability and lubricating tendency of the molten chocolate as η increased (Ashrafie et al., Citation2014). Free fat enhanced the movement of particles passing each other as they flowed. In liquid form, most of the fat is attached to particle surfaces, which significantly affected lubrication of the flow (Beckett, Citation2019). In addition, both higher Mc and solid content (particularly by CH addition) with a lower continuous phase of CB were reported to increase η of chocolate bars (Mohammadi et al., Citation2022).
Yield stress (σy) is the stress required for chocolate to decay and initiate flow, which displays low shear-rate properties, particularly in chocolate (Aidoo et al., Citation2015; Ardakani et al., Citation2014). This parameter has a linear dependency on particle-particle interactions and the amount and specific surface area of the particles, emulsifiers, and Mc (Afoakwa et al., Citation2008; Aidoo et al., Citation2015; Ashrafie et al., Citation2014; Feichtinger et al., Citation2020). The σy was increased significantly by adding CH in a dose-dependent manner () (p ≤ 0.05). It has been reported that a higher Mc with a higher CB replacement level leads to an increase in the solid content of the continuous phase (CB). This proportional reduction in CB content resulted in an increase in σy (Mohammadi et al., Citation2022). Moreover, a higher σy is predominantly influenced by the formation of CH micelles and moisture within fat multilayers around the solid particles (i.e. sugar), which prevents flow (Beckett, Citation2009; de Graef et al., Citation2011). Moreover, the addition of CH might have induced interparticle interactions between particle surfaces, hindering the flow of molten chocolate (Afoakwa, Citation2010). Our results were in accordance with the flow behaviour index of chocolate bars (). The flow behaviour index significantly increased with increasing CH addition (p ≤ 0.05). The flow behaviour index of all chocolate samples was <1 (0.86–0.89), indicating shear-thinning behaviour. This result was consistent with previous reports, in which the flow behaviour index ranged from 0.81 to 0.90 (Ashrafie et al., Citation2014; Mohammadi et al., Citation2022).
The apparent viscosity (ηap) of all the samples was recorded at a shear rate of 40 s−1 considering its relative reproducibility. As a higher CH level was used, a higher ηap was obtained () (p ≤ 0.05). This result aligned with the plastic viscosity and flow behaviour indexes. Due to its emulsification properties, CH addition could influence the formation of micelles and induce interactions between CH and chocolate particles in molten chocolate. This rheological behaviour is possibly explained by the alignment of the constituent molecules, particularly CH (Konar et al., Citation2018). The CH used in this study contained a distinctive quantity of peptides with various functional groups (Indriani et al., Citation2022) that could interact with other molecules and induce particle-particle interactions between the chocolate components. Higher interactions of CH with sugar and cocoa mass could occur in a hydrophobic environment owing to its amphiphilic ability, and could be obtained with higher CH amounts. Generally, these CH-coated particles can reduce particle-particle interactions, particularly between hydrophilic particles, with a decrease in viscosity (Caparosa & Hartel, Citation2020; Feichtinger et al., Citation2020). Nevertheless, interactions between these CH-coated particles might be induced, with the hydrophilic patch of CH favouring the hydrophilicity of sugar, and the cocoa solid being expected for hydrophobic patches. In contrast, CH-coated particles can also form complex molecules and undergo protein-protein interactions or protein aggregation on their surfaces (Mezzenga & Fischer, Citation2013). Apart from the proportional changes in fat and solid contents, this hypothesis could better explain the enhancement of CH molecular interaction in the chocolate matrix with increasing chocolate viscosity, particularly ηap. Thus, the different CH addition amounts used in the present study had a significant impact on chocolate flow behaviour, which contributed to its thixotropy.
Thixotropy is a time-dependent behaviour during shearing, implying an extensive structural breakdown of the sample. In general, properly conched chocolate should not exhibit thixotropic or very low thixotropy values (Ashrafie et al., Citation2014). shows a significant increase in thixotropy for chocolate bar samples after CH addition (0.5–2.0% [w/w]) compared with the control (4.11 Pa.s−1) (p ≤ 0.05). Increases in CH increased thixotropy in a dose-dependent manner (p ≤ 0.05). This indicated a dilution effect of CB by the addition of CH, which could hamper conching and tempering during chocolate production. The control group had a higher and more available proportion of the continuous fat phase to disperse the particles properly compared to those in the CH addition group. The thixotropies are governed by fat content and particle size distribution (Afoakwa et al., Citation2008). Notably, lower thixotropy values indicate a lower degree of network formation with fewer structural changes or rearrangements (Feichtinger et al., Citation2020). This result was consistent with the higher molecular interactions induced by CH with increasing plastic viscosity, yield stress, and apparent viscosity as the CH addition amount increased (). A similar result was reported by Mohammadi et al. (Citation2022), in which a higher CB replacement with instant gel schoko (IGS) resulted in a significantly higher thixotropy. Therefore, the rheological behaviour of chocolate bars with different amounts of added CH was varied significantly because of the proportional change in the CB as well as the induction of molecular interactions involving CH. This could influence the melting and sensory properties of chocolate bars.
Thermal behaviour
The thermal behaviour changes of chocolate bar samples were evaluated under three different segments of DSC thermogram after a storage period of 24 h at 22 °C: (1) melting, (2) crystallization, and (3) re-melting (). shows the effect of isothermal crystallization on the melting behaviour of chocolate bar samples. Tonset corresponds to the temperature at which a particular crystal form begins to melt, and Tmax is the temperature at the highest melting rate (end of melting or completion of liquefaction) (Aidoo et al., Citation2015). Here, Tendset represents the temperature at which the melting process is complete. CB displays several crystal polymorphisms, such as α, β, and β′ forms whereas its crystallization consistency and sensorial profiles are determined by the composition of triacylglycerol (TAG) (Afoakwa, Citation2010; Beckett, Citation2019). Moreover, the phase transitions may be correlated with hydrocarbon chains or molecular bending in the glycerol region. All chocolate bar samples exhibited similar Tonset (28.56–29.05 °C). Tmax (34.52 to 34.83 °C) with an insignificant difference in enthalpies (ΔH: 54.69 to 50.55 J/g) influenced crystal melting (p > 0.05). However, a significant increase in Tendset was observed for CH-supplemented samples, particularly at the highest concentration tested (p ≤ 0.05). CH particles embedded in the chocolate matrix could hinder CB crystallization by reducing the creation of large crystalline bulk regions, thus increasing the melting temperature (Castro-Alayo et al., Citation2023; Lapčíková et al., Citation2022), whereas at higher-ordered dense crystalline cocoa fat matrix could be generated to a greater extent (Feichtinger et al., Citation2020; Lapčíková et al., Citation2022; Nakano et al., Citation2014). The results from the present study showed Tmax was within the range of 29–34 °C, the chocolate sample produced a smooth and refreshing sensation in the mouth, where it occurred in solid form at room temperature and melted immediately as the mouth’s latent heat was absorbed (Castro-Alayo et al., Citation2023). Thus, all chocolate samples contained similar types and proportions of polymorphisms, which could favour their melting characteristics.
Figure 4. Thermal behaviour of dark chocolate bars prepared with collagen hydrolysate from Asian bullfrog skin at different addition levels. Control: Dark chocolate bar prepared without collagen hydrolysate addition. C-0.5, -1.0, -2.0: Dark chocolate prepared with collagen hydrolysate addition at 0.5, 1.0, and 2.0% (w/w), respectively. (a) Melting process after crystallization for 24 h at 22 °C. (b) Dynamic crystallization from 90 °C to – 50 °C. (c) Re-melting of crystals formed in segment (b). Arrow indicates the S-L-S (Solid–Liquid–Solid) transition.
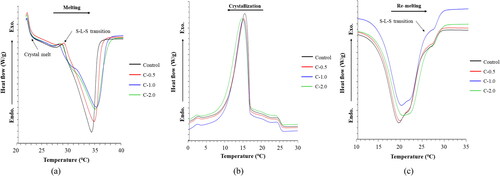
The dynamic crystallization of the chocolate bar samples is shown in (b), and the exothermic peaks were studied. This segment demonstrates the co-crystallization of the high melting fraction (HMF), medium melting fraction (MMF), and low melting fraction (LMF) of TAG after the melting segment (Joshi et al., Citation2020). A single sharp peak was observed because the predominant TAGs in CB were SSS (saturated-saturated-saturated), which constitute nearly 96% of total TAGs (da Silva et al., Citation2017). di Bari et al. (Citation2017) suggested that the system might experience a heterogeneous pathway of nucleation and emulsions containing a larger number of ‘crystallization seeds’. As an emulsifier, CH may modify crystallization during crystal nucleation and/or growth, and act as a crystal promoter (Rosales et al., Citation2017). A slight shifting of crystallization peak to lower Tmax and Tendset was observed from CH-added samples, especially C-2.0 (p ≤ 0.05). Nevertheless, all samples displayed similar crystallization curves with a slight change in the Tonset and exothermic peak areas (p > 0.05). The dilution and solubilization of TAGs and fatty acids in chocolate blends were presumed to cause differences in crystallization temperatures (Jahurul et al., Citation2014). Moreover, a higher degree of particle-particle interactions could occur because of the presence of CH particles and additional heating during the melting segment. In addition, the presence of solid particles (non-fat solids from cocoa, sugar, and CH) might contribute to the crystallization behaviour of chocolate bars because they have specific crystallization characteristics (i.e. temperature and structure) (Slavyanskiy et al., Citation2021), which might influence the homogeneity of the crystal forms in the chocolate bar.
The re-melting profiles of the chocolate samples after crystallization were studied and are presented in (c). C-2.0 showed less sharp or overlapping endothermic peaks with wider shoulders compared to the others, with sharp and slightly steep shoulder peaks. This was in accordance with the significantly higher Tmax and Tendset found for C-2.0 (p ≤ 0.05). This result indicated the eutectic mixture in C-2.0, as it exhibited a broader peak in the re-melting profile. di Bari et al. (Citation2017) reported the Tmax of polymorphism II (α: 18 ± 2.0 °C), III (β′2: 23 ± 1.5 °C), IV (β′′1: 26 ± 1.5 °C), and V (β2: 30 ± 1.0 °C), in which chocolate bars could contain crystal forms in various proportions. According to the present results, a peak ranging from 19.66–20.31 °C was observed, indicating the presence of polymorphism III. This indicated the possibility of the α polymorphism undergoing crystallization at first, followed by its evolution to β′2 prior/during re-heating. Consequently, polymorphism III appeared predominantly in the re-melting curve (di Bari et al., Citation2017). The re-melting of chocolate samples could influence particle-particle interactions that subsequently drive nucleation into a new arrangement by a mechanism of ‘interfacial heterogeneous nucleation’ (di Bari et al., Citation2017; Feichtinger et al., Citation2020; Fernandes et al., Citation2013). In addition, the presence of CH could interfere with the enlargement of fat crystals and act as a nucleating agent, thus inducing the nucleation of smaller fat crystals with a more ordered structure.
α-Amylase inhibitory activity
The inhibitory activity of chocolate bars supplemented with CH against α-amylase is listed in . A control sample prepared without CH showed no potential for α-amylase inhibitory activity, whereas samples with higher levels of CH exhibited significantly higher α-amylase inhibitory activity in a dose-dependent manner (p ≤ 0.05). This result was consistent with the α-amylase inhibitory activity of CH (), with an IC50 of 3.60 mg/mL. Kababie-Ameo et al. (Citation2022) reported that different types of cocoa (chocolate, beverage, cocoa extract, and its bioactive compound isolate) can increase or decrease the bioavailability of bioactive compounds. Dark chocolate bars have been formulated and/or used as drug delivery tools using bioactive compounds (Chaudhari et al., Citation2018; Naqvi et al., Citation2022; Paul et al., Citation2021). In the present study, C-0.5, C-1.0, and C-2.0 contained CH at 4.98, 9.90, and 14.78 mg/g sample with 14.74, 22.97, and 36.44% inhibitory activity, respectively. This suggests an interfering effect by chocolate bar components and/or the production process used, could reduce the bioavailability of CH to some degree compared to CH in its original form. Nevertheless, CH retained its bio-functionality as an antidiabetic agent in chocolate bars. Thus, the addition of CH could effectively produce an antidiabetic dark chocolate bar with α-amylase inhibitory activity, which would be expected to prevent the digestion of starch and sugar to control and/or reduce blood sugar levels after consumption.
In comparison with the control, C-2.0 showed comparable physicochemical characteristics, rheological, and thermal behaviours, with the highest α-amylase inhibitory activity, making it an ideal candidate for further characterization.
Characteristics of selected dark chocolate bar with addition of CH
Nutritional characteristics and energy value
The nutritional characteristics and energy values of chocolate bars with 2% (w/w) CH addition are shown in in comparison with those of the control (without CH). CH addition significantly increased the protein content from 8.31 to 9.59 g/100 g (p ≤ 0.05). The nutrient reference value (NRV) of protein for adults and children (≥4 years old) is defined at 50 g according to caloric intake reference at 2,000 calories with 0.75–0.8 g of protein per kg body weight per day (FDA Citation2016; WHO Citation2007). Based on the Codex Alimentarius Commission (CAC), C-2.0 can be claimed as a ‘source of protein’ that contained at least 10% NRV per 100 g (Lewis, Citation2019). C-2.0 had a lower fat content (34.37 g/100 g), which was plausibly due to proportional changes in fat and solid content caused by CH addition compared to that of the control (37.53 g/100 g) (p ≤ 0.05). Similarly, Ashrafie et al. (Citation2014) found that 15% of CB replacement by using IGS resulted in 8.7% fat reduction. Moreover, a higher level of CB replacement with CH was found to lower the fat content of chocolate bars (Ashrafie et al., Citation2014; Mohammadi et al., Citation2022). The addition of CH, particularly at 2%, did not significantly affect ash (2.05–2.16 g/100 g) and total carbohydrate (50.44–51.12 g/100 g) contents, and the solid content in chocolate bars was increased (p > 0.05). C-2.0 displayed a lower energy value owing to its increased protein content and decreased fat content by 15.40% and 8.42%, respectively, compared to that of the control (p ≤ 0.05). The approximate energy value of chocolate samples was predominantly dependent on protein and fat content. It was worthwhile to use CH from Asian bullfrog skin as a bioactive compound, particularly as an antidiabetic agent, in dark chocolate bars. The resulting product did not only exhibit α-amylase inhibitory activity, but also realized an improvement in nutritional value, particularly protein content with lowered calorific content.
Table 2. Nutritional compositions, energy value, and sensory attributes of selected dark chocolate bars prepared with collagen hydrolysate from Asian bullfrog skin at different addition levels.
Sensory characteristics
Sensory characteristics of dark chocolate bars prepared with 2.0% (w/w) addition of CH were evaluated using a 5-point hedonic scale and reported as a ‘liking score’, in comparison with the control (no CH addition) (). Notably, all attributes tested in C-2.0 did not significantly differ from the control, with liking scores >3.00 (p ≤ 0.05), except for mouthfeel and hardness. Although a small difference in colour was found between the control and C-2.0 (), the panellists did not notice this as a distinct characteristic. Hamdan et al. (Citation2020) found that the unique odour of microalgae (Spirulina platensis) was not detected in a fortified chocolate bar. Nonetheless, it enhanced the intensity of the sweet and chocolate flavours and decreased the bitter and sour flavours without giving off an unpleasant odour. Given the plain taste and flavour of the CH used in the present study, the addition of CH did not have a detrimental impact on taste and aftertaste of the chocolate bars. The sweetness of the dark chocolate bars was mainly due to sucrose and fructose (McShea et al., Citation2008). A similar liking score for sweetness coincided with a similar total carbohydrate content in both samples () (p > 0.05). As a continuous phase in the chocolate system, CB governs the melting profiles and mouthfeel (Mohammadi et al., Citation2022). Thus, a reduction in the proportion of CB and a change in its rearrangement upon the addition of CH led to an increase in hardness () and delayed chocolate melting in the mouth by increasing the melting temperature (Tmax) (). C-2.0 presumably displayed a slower melting rate and had a slightly lower liking score than the control (p ≤ 0.05). Konar et al. (Citation2018) reported that an unpleasant texture can occur due to the presence of coarse particles, resulting in gritty chocolate and coarse mouthfeel. These factors lead to difficulties in swallowing, poor melting profiles, and hardness. However, although fat reduction in chocolate bars (up to 5%) was reported to reduce the liking score for all sensory attributes tested, it remained acceptable to panellists (FarzanMehr et al., Citation2008). Here, a similar result was observed for C-2.0, with an 8.42% fat reduction, compared to that of the control (). Nevertheless, the C-2.0 sample had good acceptance among the panellists, as indicated by liking scores ≤4.00, particularly for overall acceptance. Therefore, CH from the skin of Asian bullfrogs showed potential as a functional fortifier in dark chocolate bars with antidiabetic activity and good sensory characteristics, particularly at 2% CH addition.
Conclusion
CH from Asian bullfrog skin exhibited antidiabetic activity against α-amylase, possessing a non-competitive inhibition mode. The addition of CH to dark chocolate bars affected the characteristics of the chocolate samples. By adding CH, the BS increased, whereas α-amylase inhibitory activity also increased in a dose-dependent manner (p ≤ 0.05). The thermal behaviour of the chocolate bars changed with the addition of CH, particularly at the highest concentration used. The addition of CH could effectively yield chocolate bars with α-amylase inhibitory activity, especially at 2% (w/w) CH addition (C-2.0). C-2.0 showed higher protein content with lower fat content and energy values than the control. Moreover, C-2.0 had good sensory acceptance among the panellists, indicating good palatability. Thus, CH has the potential to be used as a bioactive food additive to produce functional foods, particularly antidiabetic dark chocolate bars.
Authors’ contributions
S.I.: conceptualization, methodology, software, data curation, writing –original draft preparation, visualization, investigation, writing – review & editing. S.B.: writing – review & editing. A.B.S.: writing – review & editing. S.K.: conceptualization, methodology, writing – original draft preparation, visualization, investigation, writing – review & editing. S.N.: writing – review & editing.
Ethical approval
All experiments this study were performed with the approval of the Research Ethics Committees of King Mongkut’s Institute of Technology Ladkrabang (EC-KMITL_64_039).
Disclosure statement
The authors declare no conflict of interest.
Additional information
Funding
References
- Ademiluyi, A. O., Oboh, G., Aragbaiye, F. P., Oyeleye, S. I., & Ogunsuyi, O. B. (2015). Antioxidant properties and in vitro α-amylase and α-glucosidase inhibitory properties of phenolics constituents from different varieties of Corchorus spp. Journal of Taibah University Medical Sciences, 10(3), 1–15. https://doi.org/10.1016/j.jtumed.2014.11.005
- Admassu, H., Gasmalla, M. A. A., Yang, R., & Zhao, W. (2018). Identification of bioactive peptides with α-amylase inhibitory potential from enzymatic protein hydrolysates of red seaweed (Porphyra spp). Journal of Agricultural and Food Chemistry, 66(19), 4872–4882. https://doi.org/10.1021/acs.jafc.8b00960
- Afoakwa, E. O. (2010). Chocolate science and technology. Wiley-Blackwell.
- Afoakwa, E. O., & Afoakwa, E. O. (2016). Tempering and fat crystallization effects on chocolate quality (pp. 317–344). Chocolate Science and Technology.
- Afoakwa, E. O., Paterson, A., & Fowler, M. (2008). Effects of particle size distribution and composition on rheological properties of dark chocolate. European Food Research and Technology, 226(6), 1259–1268. https://doi.org/10.1007/s00217-007-0652-6
- Aidoo, R. P., Afoakwa, E. O., & Dewettinck, K. (2015). Rheological properties, melting behaviours and physical quality characteristics of sugar-free chocolates processed using inulin/polydextrose bulking mixtures sweetened with stevia and thaumatin extracts. LWT – Food Science and Technology, 62(1), 592–597. https://doi.org/10.1016/j.lwt.2014.08.043
- Aidoo, R. P., Appah, E., van Dewalle, D., Afoakwa, E. O., & Dewettinck, K. (2017). Functionality of inulin and polydextrose as sucrose replacers in sugar-free dark chocolate manufacture – effect of fat content and bulk mixture concentration on rheological, mechanical and melting properties. International Journal of Food Science & Technology, 52(1), 282–290. https://doi.org/10.1111/ijfs.13281
- [AOAC] Association of Official Analytical Collaboration (US). (2012). Official methods for analysis. Association of Official Analytical Chemists.
- Ardakani, H. A., Mitsoulis, E., & Hatzikiriakos, S. G. (2014). Capillary flow of milk chocolate. Journal of Non-Newtonian Fluid Mechanics, 210, 56–65. https://doi.org/10.1016/j.jnnfm.2014.06.001
- Arise, R., Yekeen, A., & Ekun, O. (2016). In vitro antioxidant and α-amylase inhibitory properties of watermelon seed protein hydrolysates. Environmental and Experimental Biology, 14, 163–172.
- Arise, R. O., Idi, J. J., Mic-Braimoh, I. M., Korode, E., Ahmed, R. N., & Osemwegie, O. (2019). In vitro Angiotesin-1-converting enzyme, α-amylase and α-glucosidase inhibitory and antioxidant activities of Luffa cylindrical (L.) M. Roem seed protein hydrolysate. Heliyon, 5(5), e01634. https://doi.org/10.1016/j.heliyon.2019.e01634
- Ashrafie, N. T., Azizi, M. H., Taslimi, A., Mohammadi, M., Neyestani, T. R., & Mohammadifar, M. A. (2014). Development of reduced-fat and reduced-energy dark chocolate using collagen hydrolysate as cocoa butter replacement agent. Journal of Food and Nutrition Research, 53(1), 13–21.
- Auerbach, M., & Dedman, A-k (2012). Chapter 20 bulking agents – multi-functional ingredients. In O’Donnell, K., & Kearsley, M.W. (Eds.), Sweeteners and sugar alternatives in food technology, Second Edition (pp. 433–470). Wiley Online Library.
- Awosika, T. O., & Aluko, R. E. (2019). Inhibition of the in vitro activities of α-amylase, α-glucosidase and pancreatic lipase by yellow field pea (Pisum sativum L.) protein hydrolysates. International Journal of Food Science & Technology, 54(6), 2021–2034. https://doi.org/10.1111/ijfs.14087
- Bahari, A., & Akoh, C. C. (2018). Texture, rheology and fat bloom study of ‘chocolates’ made from cocoa butter equivalent synthesized from illipe butter and palm mid-fraction. LWT, 97, 349–354. https://doi.org/10.1016/j.lwt.2018.07.013
- Beckett, S. T. (2009). Chocolate flow properties. In Beckett S. T. (Ed.), Industrial chocolate manufacture and use (pp. 224–246). Wiley-Blackwell.
- Beckett, S. T. (2019). The science of chocolate. Royal Society of Chemistry.
- Berraquero-García, C., Rivero-Pino, F., Ospina, J. L., Pérez-Gálvez, R., Espejo-Carpio, F. J., Guadix, A., García-Moreno, P. J., & Guadix, E. M. (2023). Activity, structural features and in silico digestion of antidiabetic peptides. Food Bioscience, 55, 102954. https://doi.org/10.1016/j.fbio.2023.102954
- Brown, A. L., Bakke, A. J., & Hopfer, H. (2020). Understanding American premium chocolate consumer perception of craft chocolate and desirable product attributes using focus groups and projective mapping. PloS One, 15(11), e0240177. https://doi.org/10.1371/journal.pone.0240177
- Cadby, J., Araki, T., & Villacis, A. H. (2021). Breaking the mold: Craft chocolate makers prioritize quality, ethical and direct sourcing, and environmental welfare. Journal of Agriculture and Food Research, 4, 100122. https://doi.org/10.1016/j.jafr.2021.100122
- Caparosa, M. H., & Hartel, R. W. (2020). Characterizing lecithin interactions in chocolate using interfacial properties and rheology. Journal of the American Oil Chemists’ Society, 97(12), 1309–1317. https://doi.org/10.1002/aocs.12419
- Castro-Alayo, E. M., Balcázar-Zumaeta, C. R., Torrejón-Valqui, L., Medina-Mendoza, M., Cayo-Colca, I. S., & Cárdenas-Toro, F. P. (2023). Effect of tempering and cocoa butter equivalents on crystallization kinetics, polymorphism, melting, and physical properties of dark chocolates. LWT, 173, 114402. https://doi.org/10.1016/j.lwt.2022.114402
- Chaudhari, S. A., Devare, R., Dewang, P. S., Patil, V. B., Patil, A. M., & Pawar, S. P. (2018). Chocolate formulation as drug delivery system. Indian Journal of Drugs, 6(2), 136–141.
- da Silva, T. L. T., Grimaldi, R., Calligaris, G. A., Cardoso, L. P., & Gonçalves, L. A. G. (2017). Crystallinity properties and crystallization behavior of chocolate fat blends. Journal of Food Science and Technology, 54(7), 1979–1989. https://doi.org/10.1007/s13197-017-2634-4
- de Graef, V., Depypere, F., Minnaert, M., & Dewettinck, K. (2011). Chocolate yield stress as measured by oscillatory rheology. Food Research International, 44(9), 2660–2665. https://doi.org/10.1016/j.foodres.2011.05.009
- di Bari, V., Macnaughtan, W., Norton, J., Sullo, A., & Norton, I. (2017). Crystallisation in water-in-cocoa butter emulsions: Role of the dispersed phase on fat crystallisation and polymorphic transition. Food Structure, 12, 82–93. https://doi.org/10.1016/j.foostr.2016.10.001
- Diesmos, A., Brown, R., & Gee, G. (2004). Preliminary report on the amphibians and reptiles of Balbalasang-Balbalan National Park, Luzon Island, Philippines. Sylvatrop, the Technical Journal of Philippine Ecosystems and Natural Resources, 13(1&2), 63–80.
- FarzanMehr, H., Abbasi, S., & Sahari, M. A. (2008). Effects of sugar replacers on physicochemical, rheological and sensory properties of milk chocolate. Iranian Journal of Nutrition Science and Food Technology, 3(3), 65–82.
- [FDA] Food and Drug Administration (US). (2016). Food labeling: Revision of the nutrition and supplement facts labels (2016-11867). http://federalregister.gov/a/2016-11867
- Feichtinger, A., Scholten, E., & Sala, G. (2020). Effect of particle size distribution on rheological properties of chocolate. Food & Function, 11(11), 9547–9559. https://doi.org/10.1039/d0fo01655a
- Fernandes, V. A., Müller, A. J., & Sandoval, A. J. (2013). Thermal, structural and rheological characteristics of dark chocolate with different compositions. Journal of Food Engineering, 116(1), 97–108. https://doi.org/10.1016/j.jfoodeng.2012.12.002
- Fibrianto, K., Azhar, L. O. M. J., Widyotomo, S., & Harijono, H. (2021). Effect of cocoa bean origin and conching time on the physicochemical and microstructural properties of Indonesian dark chocolate. Brazilian Journal of Food Technology, 24, e2019249.
- Gallo, P. J., Antolin-Lopez, R., & Montiel, I. (2018). Associative sustainable business models: Cases in the bean-to-bar chocolate industry. Journal of Cleaner Production, 174, 905–916. https://doi.org/10.1016/j.jclepro.2017.11.021
- Gong, L., Feng, D., Wang, T., Ren, Y., Liu, Y., & Wang, J. (2020). Inhibitors of α-amylase and α-glucosidase: Potential linkage for whole cereal foods on prevention of hyperglycemia. Food Science & Nutrition, 8(12), 6320–6337. https://doi.org/10.1002/fsn3.1987
- Hamdan, A. B., Riaty, C., Fitriya, W., & Ekantari, N. (2020). Effects of nonencapsulated carotenoid of Spirulina platensis on the sensory profiles of dark and milk chocolate. E3S Web of Conferences, 147, 03022. https://doi.org/10.1051/e3sconf/202014703022
- Harris, M., Potgieter, J., Ishfaq, K., & Shahzad, M. (2021). Developments for collagen hydrolysate in biological, biochemical, and biomedical domains: A comprehensive review. Materials (Basel, Switzerland), 14(11), 2806. https://doi.org/10.3390/ma14112806
- Indriani, S., Karnjanapratum, S., Nirmal, N. P., & Nalinanon, S. (2023). Amphibian skin and skin secretion: An exotic source of bioactive peptides and its application. Foods (Basel, Switzerland), 12(6), 1282. https://doi.org/10.3390/foods12061282
- Indriani, S., Sae-Leaw, T., Benjakul, S., Quan, H. T., Karnjanapratum, S., & Nalinanon, S. (2022). Impact of different ultrasound-assisted processes for preparation of collagen hydrolysates from Asian bullfrog skin on characteristics and antioxidative properties. Ultrasonics Sonochemistry, 89, 106163. https://doi.org/10.1016/j.ultsonch.2022.106163
- Jahurul, M., Zaidul, I., Norulaini, N. N., Sahena, F., Abedin, M., Mohamed, A., & Omar, A. M. (2014). Hard cocoa butter replacers from mango seed fat and palm stearin. Food Chemistry, 154, 323–329. https://doi.org/10.1016/j.foodchem.2013.11.098
- Joshi, B. L., Zielbauer, B. I., & Vilgis, T. A. (2020). Comparative study on mixing behavior of binary mixtures of cocoa butter/tristearin (CB/TS) and cocoa butter/coconut oil (CB/CO). Foods (Basel, Switzerland), 9(3), 327. https://doi.org/10.3390/foods9030327
- Kababie-Ameo, R., Rabadán-Chávez, G. M., Vázquez-Manjarrez, N., & Gutiérrez-Salmeán, G. (2022). Potential applications of cocoa (Theobroma cacao) on diabetic neuropathy: Mini-review. Frontiers in Bioscience, 27(2), 57.
- Karimi, A., Gavlighi, H. A., Sarteshnizi, R. A., & Udenigwe, C. C. (2021). Effect of maize germ protein hydrolysate addition on digestion, in vitro antioxidant activity and quality characteristics of bread. Journal of Cereal Science, 97, 103148. https://doi.org/10.1016/j.jcs.2020.103148
- Karnjanapratum, S., & Benjakul, S. (2020). Asian bullfrog (Rana tigerina) skin gelatin extracted by ultrasound-assisted process: Characteristics and in-vitro cytotoxicity. International Journal of Biological Macromolecules, 148, 391–400. https://doi.org/10.1016/j.ijbiomac.2020.01.150
- Kaur, N., Kumar, V., Nayak, S. K., Wadhwa, P., Kaur, P., & Sahu, S. K. (2021). Alpha-amylase as molecular target for treatment of diabetes mellitus: A comprehensive review. Chemical Biology & Drug Design, 98(4), 539–560. https://doi.org/10.1111/cbdd.13909
- Konar, N., Toker, O. S., Pirouzian, H. R., Oba, S., Polat, D. G., Palabiyik, İ., Poyrazoglu, E. S., & Sagdic, O. (2018). Enrichment of milk chocolate by using EPA and DHA originated from various origins: Effects on product quality. Sugar Tech, 20(6), 745–755. https://doi.org/10.1007/s12355-018-0611-5
- Lapčíková, B., Lapčík, L., Salek, R., Valenta, T., Lorencová, E., & Vašina, M. (2022). Physical characterization of the milk chocolate using whey powder. LWT, 154, 112669. https://doi.org/10.1016/j.lwt.2021.112669
- Lewis, J. (2019). Codex nutrient reference values. FAO and WHO.
- McShea, A., Ramiro-Puig, E., Munro, S. B., Casadesus, G., Castell, M., & Smith, M. A. (2008). Clinical benefit and preservation of flavonols in dark chocolate manufacturing. Nutrition Reviews, 66(11), 630–641. https://doi.org/10.1111/j.1753-4887.2008.00114.x
- Merrill, A. L., & Watt, B. K. (1955). Energy value of foods: Basis and derivation: Human nutrition research branch. Agricultural Research Service.
- Mezzenga, R., & Fischer, P. (2013). The self-assembly, aggregation and phase transitions of food protein systems in one, two and three dimensions. Reports on Progress in Physics. Physical Society (Great Britain), 76(4), 046601. https://doi.org/10.1088/0034-4885/76/4/046601
- Mohammadi, M., Zoghi, A., & Fazeli, F. (2022). Influence of collagen hydrolysate as cocoa butter replacement agent on the chemical, rheological, and sensory properties of reduced-fat and reduced-calorie milk chocolate. Journal of Food Processing and Preservation, 46(2), e16267.
- Nakano, S-i., Miyoshi, D., & Sugimoto, N. (2014). Effects of molecular crowding on the structures, interactions, and functions of nucleic acids. Chemical Reviews, 114(5), 2733–2758. https://doi.org/10.1021/cr400113m
- Naqvi, R. F., Khan, A., Umer, M. F., Malik, O., & Shahwani, N. A. (2022). Design and optimization of novel taste-masked medicated chocolates of dextromethorphan with in vitro and in vivo taste evaluation. Journal of Pharmaceutical Innovation, 17(2), 376–390. https://doi.org/10.1007/s12247-020-09511-8
- Natsir, H., Dali, S., Arif, A. R., Sartika, & Leliani. (2019). Activity and kinetics of α-glucosidase inhibition by collagen hydrolysate from Thunnus albacares bone. Journal of Physics: Conference Series, 1341(3), 032015. https://doi.org/10.1088/1742-6596/1341/3/032015
- Nightingale, L., Lee, S.-Y., & Engeseth, N. (2009). Textural changes in chocolate characterized by instrumental and sensory techniques. Journal of Texture Studies, 40(4), 427–444. https://doi.org/10.1111/j.1745-4603.2009.00190.x
- Nizori, A., Adinda, V., Arzita, L., & Suseno, R. (2021). Antioxidant activity and physico-chemical of dark chocolate made with cocoa butter substitute (Cbs) from virgin coconut oils. Proceedings of the 3rd Green Development International Conference (GDIC 2020).
- Offengenden, M., Chakrabarti, S., & Wu, J. (2018). Chicken collagen hydrolysates differentially mediate anti-inflammatory activity and type I collagen synthesis on human dermal fibroblasts. Food Science and Human Wellness, 7(2), 138–147. https://doi.org/10.1016/j.fshw.2018.02.002
- Oliveira, B., Falkenhain, K., & Little, J. P. (2022). Sugar-free dark chocolate consumption results in lower blood glucose in adults with diabetes. Nutrition and Metabolic Insights, 15, 11786388221076962. https://doi.org/10.1177/11786388221076962
- Paul, M. P., Ranabhat, P. R., Khatiwara, D. K., & Bagchi, A. (2021). Review on medicated chocolate takes a patient-centered approach to drug delivery. Journal of Applied Pharmaceutical Research, 9(4), 16–22. https://doi.org/10.18231/j.joapr.2021.16.22
- Praseptiangga, D., Invicta, S. E., & Khasanah, L. U. (2019). Sensory and physicochemical characteristics of dark chocolate bar with addition of cinnamon (Cinnamomum burmannii) bark oleoresin microcapsule. Journal of Food Science and Technology, 56(9), 4323–4332. https://doi.org/10.1007/s13197-019-03901-8
- Rabiei, S., Rezaei, M., Asgharzade, S., Nikoo, M., & Rafieia-Kopai, M. (2019). Antioxidant and cytotoxic properties of protein hydrolysates obtained from enzymatic hydrolysis of Klunzinger’s mullet (Liza klunzingeri) muscle. Brazilian Journal of Pharmaceutical Sciences, 55, e18304.
- Rahimi, R., Gavlighi, H. A., Sarteshnizi, R. A., Barzegar, M., & Udenigwe, C. C. (2022). In vitro antioxidant activity and antidiabetic effect of fractionated potato protein hydrolysate via ultrafiltration and adsorption chromatography. LWT, 154, 112765. https://doi.org/10.1016/j.lwt.2021.112765
- Ramadhan, A. H., Nawas, T., Zhang, X., Pembe, W. M., Xia, W., & Xu, Y. (2017). Purification and identification of a novel antidiabetic peptide from Chinese giant salamander (Andrias davidianus) protein hydrolysate against α-amylase and α-glucosidase. International Journal of Food Properties, 20(sup3), S3360–S3372. https://doi.org/10.1080/10942912.2017.1354885
- Rivero-Pino, F. (2023). Bioactive food-derived peptides for functional nutrition: Effect of fortification, processing and storage on peptide stability and bioactivity within food matrices. Food Chemistry, 406, 135046. https://doi.org/10.1016/j.foodchem.2022.135046
- Rosales, C. K., Klinkesorn, U., & Suwonsichon, S. (2017). Effect of crystal promoters on viscosity and melting characteristics of compound chocolate. International Journal of Food Properties. 20(1), 119–132. https://doi.org/10.1080/10942912.2016.1147458
- Sai, K. P., Jagannadham, M. V., Vairamani, M., Raju, N. P., Devi, A. S., Nagaraj, R., & Sitaram, N. (2001). Tigerinins: Novel antimicrobial peptides from the Indian frog Rana tigerina. Journal of Biological Chemistry, 276(4), 2701–2707. https://doi.org/10.1074/jbc.M006615200
- Shah, S. R., Alweis, R., Najim, N. I., Dharani, A. M., Jangda, M. A., Shahid, M., Kazi, A. N., & Shah, S. A. (2017). Use of dark chocolate for diabetic patients: A review of the literature and current evidence. Journal of Community Hospital Internal Medicine Perspectives, 7(4), 218–221. https://doi.org/10.1080/20009666.2017.1361293
- Slavyanskiy, A., Lebedeva, N., & Voitsekhovsky, V. (2021). Formation of sucrose crystallization centers and their growth in a vacuum apparatus. BIO Web of Conferences, 32, 03016. https://doi.org/10.1051/bioconf/20213203016
- Soltaninejad, H., Zare-Zardini, H., Ordooei, M., Ghelmani, Y., Ghadiri-Anari, A., Mojahedi, S., & Hamidieh, A. A. (2021). Antimicrobial peptides from amphibian innate immune system as potent antidiabetic agents: A literature review and bioinformatics analysis. Journal of Diabetes Research, 2021, 2894722–2894710. https://doi.org/10.1155/2021/2894722
- Taylor, T., Fasina, O., & Bell, L. (2008). Physical properties and consumer liking of cookies prepared by replacing sucrose with tagatose. Journal of Food Science, 73(3), S145–S151.
- Tiwari, S. P., Srivastava, R., Singh, C. S., Shukla, K., Singh, R. K., Singh, P., & Sharma, R. (2015). Amylases: An overview with special reference to alpha amylase. Journal of Global Bioscience, 4(1), 1886–1901.
- Valussi, M., & Minto, C. (2016). Cacao as a globalised functional food: Review on cardiovascular effects of chocolate consumption. Open Agriculture Journal, 10(1), 36–51. https://doi.org/10.2174/1874331501610010036
- [WHO] World Health Organization (EU). (2007). Protein and amino acid requirements in human nutrition: Report of a joint FAO/WHO/UNU expert consultation. World Health Organization.
- Zamorano-Apodaca, J. C., García-Sifuentes, C. O., Carvajal-Millán, E., Vallejo-Galland, B., Scheuren-Acevedo, S. M., & Lugo-Sánchez, M. E. (2020). Biological and functional properties of peptide fractions obtained from collagen hydrolysate derived from mixed by-products of different fish species. Food Chemistry, 331, 127350. https://doi.org/10.1016/j.foodchem.2020.127350
- Zhang, L., Shan, Y., Hong, H., Luo, Y., Hong, X., & Ye, W. (2020). Prevention of protein and lipid oxidation in freeze-thawed bighead carp (Hypophthalmichthys nobilis) fillets using silver carp (Hypophthalmichthys molitrix) fin hydrolysates. LWT, 123, 109050. https://doi.org/10.1016/j.lwt.2020.109050