Abstract
It is well-established that Morus alba and its derivatives have been clinically proven to have antioxidant and anti-inflammatory properties. Although several studies have mentioned the beneficial effect of Morus alba on kidney disorders, the role of Morus alba in CKD still needs to be explored. Thus, this study investigated the effect of Morus alba and chlorogenic acid treatment on renal fibrosis in mice induced with unilateral urethral obstruction (UUO). Twenty-five Balb/c mice were randomly divided into five groups: the sham-operated group, the UUO untreated group, and the UUO treated with enalapril (ENA), Morus alba leaves extract (MLE) and chlorogenic acid (CGA) groups. Urine, blood, and organ samples were collected to evaluate urinalysis, haematology, biochemistry, and histopathology. The result indicated that the UUO group had significantly higher levels of creatinine (p < 0.001) and urea (p < 0.05), as well as hydronephrosis and urine crystal sedimentation (p < 0.05). In comparison to the UUO group, the MLE and CGA groups displayed improved urine profiles, ameliorated anaemia (p < 0.05), and urine crystals (p < 0.05). The administration of CGA also significantly reduced serum creatinine (p < 0.05) and protein-creatinine ratio (UPCr) levels. In addition, MLE and CGA could prevent hydronephrosis and delay renal damage, particularly in the dilation of Bowman’s space, tubular injury, loss of tubular epithelium, and cellular inflammation. In conclusion, Morus alba and CGA had a therapeutic effect in minimising the progression of renal damage. As a result, it can be promoted as a complementary renoprotective product based on natural products, as it has a potentially similar effect to enalapril.
PUBLIC INTEREST STATEMENT
Morus alba is a plant that originated in China and has spread worldwide. This plant has been used in many civilisations for animal feed, culinary uses, and even traditional medicine. Indeed, several studies have shown that the leaves of Morus alba contain various phytochemicals that may positively affect health. Morus alba leaves contain a variety of phytochemicals, including phenolic acids, sterols, coumarins, anthocyanins, flavonoids, and flavonols. Several scientific studies have found that certain phytochemicals, particularly chlorogenic acid, have a beneficial effect on renal disease. In fact, Morus alba contains diuretic and free radical scavenging properties that may help prevent kidney damage. Thus, using the mice animal model, this study aimed to provide evidence of the effects of Morus alba leaf extract and chlorogenic acid on CKD symptoms.
REVIEWING EDITOR:
1. Introduction
CKD is a severe health problem in humans and animals that affects more than 10% of the general population worldwide (Kovesdy, Citation2022). The leading causes of CKD are diabetes and hypertension, followed by ageing, male gender, and lifestyle (Webster et al., Citation2017). According to a meta-analysis study conducted in eleven different nations, two out of every three CKD cases in adult populations go misdiagnosed and untreated (Sundström et al., Citation2022). Consequently, over the last two decades, CKD has been related to a high death rate and morbidity worldwide (Rhee & Kovesdy, Citation2015). Therefore, more outstanding efforts for better prevention and treatment are necessary due to the large number of people affected and the significant negative impact of CKD.
CKD may be advanced to end-stage renal disease, which usually considers hemodialysis and transplantation as replacement therapy. However, due to the high cost of treatment and the adverse effects on the patient’s quality of life, hemodialysis is not an ideal treatment option (Abecassis et al., Citation2008). A similar situation exists for kidney transplantation, which has drawbacks due to limited organ donors and the possibility of organ rejection (Liu et al., Citation2020). Furthermore, it is well known that systemic inflammation and oxidative stress play key roles in the pathogenesis of CKD (Rapa et al., Citation2020). On the other hand, a systematic review article mentioned that numerous plants and their extracts have been shown to enhance kidney function through antioxidant action, which positively prevents inflammation and kidney dysfunction (Khan et al., Citation2022). Thus, discovering a therapeutic agent through plants could be promising in preventing end-stage renal disease by inhibiting the development of CKD.
Traditional herbal medicine Morus alba is widely cultivated in tropical countries like Southeast Asia to treat ailments (Ma et al., Citation2022; Zakaria & Mohd, Citation2015). It is well known that Morus alba is rich in bioactive compounds, such as flavonoids, a group of phenolic compounds abundant in Morus alba leaves (Chen et al., Citation2021). This compound appears to be a natural antioxidant that protects various organs from oxidation (Stefanucci et al., Citation2020). Benzofurans, phenolic acid, alkaloids, coumarins, chalcones, and stilbenes are other classes of compounds found in Morus alba leaves (Chen et al., Citation2021). One of the major phenolic acid compounds with antioxidant and antidiabetic properties is chlorogenic acid (CGA) (Jan et al., Citation2022). CGA also acts as an anti-fibrosis and anti-inflammatory agent, which can prevent kidney damage progression (Hwang et al., Citation2014; Yuan et al., Citation2017; Yunus et al., Citation2020).
Moreover, several studies have reported that Morus alba leaves have anti-inflammatory effects (Gryn-Rynko et al., Citation2016) and powerful antioxidant activities (Kim et al., Citation2014), protecting cells undergoing a pathological state. Indeed, studies have reported that Morus alba could protect against nephrotoxicity due to medicine treatment (Nematbakhsh et al., Citation2013), diabetic nephropathy (Swathi et al., Citation2017), and prevent nephrolithiasis (Maya & Pramod, Citation2014). However, detailed studies on the effect of Morus alba and the CGA compound on inhibiting the progression of chronic kidney disease are not available. Therefore, the present study aimed to evaluate whether Morus alba leaf extract and CGA could prevent the progression of renal damage in UUO-induced mice based on urinalysis, biochemical and haematological profiles, and histology. To examine fibrosis-attenuating treatment by Morus alba leaves and CGA, the UUO method was utilised in this study to establish a progressive kidney disease in experimental animals (Martínez-Klimova et al., Citation2019). In the meantime, the angiotensinogen-converting enzyme inhibitor enalapril served as the study’s positive control medication due to its nephroprotective properties, which may have reduced tubulointerstitial fibrosis through the TGF-beta signalling pathway downregulation (Huang et al., Citation2014; Sun et al., Citation2016).
2. Materials and methods
2.1. Morus alba leaves extraction, enalapril, and chlorogenic acid
White Mulberry (Morus alba) leaves were collected from the garden of Universiti Putra Malaysia, Selangor, Malaysia. The leaves samples were cleaned from dust and dirt, then chopped into small pieces and stored at −80 °C. The dried samples of white mulberry were ground into a coarse powder and prepared for the next extraction process. Morus alba dried powdered leaves were extracted with 60% ethanol. The solvent for the extraction of Morus alba leaves, 60% ethanol (v/v), was chosen based on the results of previous studies showing that it can provide a high content of phenolic acid, especially chlorogenic acid (Chen et al., Citation2022). The powdered extract was mixed with solvent at a 1:15 ratio in a clean glass flask. Approximately 100 g of sample powdered Morus alba leaves were dissolved in 1500 mL of 60% ethanol/water (v/v) (Zhou et al., Citation2022). The extract was filtered through no.1 filter paper and concentrated using a rotary evaporator (Buchi Rotavapor R-200, Switzerland). Subsequently, the semisolid extract was kept at 4 °C and utilised for experimental study. In our previous work, phenolic compounds in the MLE were evaluated using the Folin-Ciocalteu, aluminium chloride colourimetric, Diphenyl-1-Picrylhydrazyl (DPPH), and HPLC assays. This MLE contains a total phenolic content of 11 mg GAE/g dry weight (DW), a total flavonoid content of 257 mg QE/g DW, and antioxidant activity (602 IC50 μg/mL). The chlorogenic acid as the main compound in the MLE was 306 mg/100 g DW. Furthermore, Enapril®, produced by Malaysian company YSP Industries Sdn Bhd and contains enalapril maleate, was utilised as a standard reference medication for the renal illness. Meanwhile, Tokyo Chemical Industry (TCI) Co Ltd, Tokyo, Japan, provided pure chlorogenic acid hydrate (HPLC >98.0%).
2.2. Animal model
2.2.1. Experimental animal
The experiment used 25 male Balb/C mice aged eight weeks with an average body weight of 25 g. Male mice were used for the study as they are more susceptible to UUO-induced renal fibrosis than females, particularly regarding fibrosis gene expression (Cho et al., Citation2012). The mice were acclimatised for seven days and given feed and drink ad libitum. The animals were housed at the Faculty of Veterinary Medicine animal research facility. The cages are placed in locations free from noise and pollutants with ambient temperatures of around 20–24 °C. The university’s ethical clearance committee reviewed and approved the protocols under number UPM/IACUC/AUP-R024/2022. Experimental animals were divided into five groups: sham operation (SO) as a negative control group; UUO as a positive control group; UUO treated with 15 mg/kg enalapril (ENA) group; UUO treated with 125 mg/kg Morus alba leaves extract (MLE) group; UUO treated with 25 mg/kg chlorogenic acid (CGA) group. The enalapril dose of 15 mg/kg was chosen based on previous animal studies (Ham et al., Citation2018; Yang et al., Citation2013). The dose of 125 mg/kg of Morus alba leaf extract was selected based on the no observed adverse effect level (NOAEL) dose of our previous MLE toxicity study. Besides, the chlorogenic acid dose in mice (25 mg/kg) was adapted from animal study reports (Owumi et al., Citation2021). The mice were orally administered the treatment for 14 days, starting from the second day after the UUO induction.
2.2.2. Unilateral urethral obstruction procedure
The mice induced renal fibrosis by ligating a single ureteral in the urinary tract. All operations are carried out according to the guidelines and regulations applied to animals (Hesketh et al., Citation2014). Surgical equipment was sterilised by autoclave. Ketamine hydrochloride (100 mg/kg), xylazine (10 mg/kg), and acepromazine (5 mg/kg) cocktail were injected intraperitoneally to anesthetise the mice (Hedenqvist, Citation2021). UUO surgery was performed when animals were anaesthetised. Hair around the flank incision area was shaved with a clipper and cleaned with chlorhexidine. The right kidney was exposed with angle forceps. The right ureter was exposed and ligated twice with a 5/0 monofilament polyamide suture (Brilon®, Malaysia) between the bladder and kidney. The flank cavity was closed with a simple continuous suture with a 5/0 monofilament polyamide suture. The mice were monitored until consciousness was recovered.
2.3. Urinalysis and urine sedimentation
Mice’s urine was collected using metabolic cages for 24 h on the last day of the experimental period. The urine analysis was conducted using the Combur10 Test urine stick and the Pro-DocUReader2 (Budapest, Hungary) semi-automatic urine chemistry analyser. The urine samples were measured for specific gravity, pH, nitrite, ketone, protein, leukocytes, erythrocytes, glucose, urobilinogen, and bilirubin. Meanwhile, the urine sedimentation was performed by centrifuging the urine sample in Eppendorf 5424R microcentrifuges at 1000 × g for 5 min. Urine supernatant was discharged, and residual urine sediment was mixed using Methylene blue. Crystal urine was evaluated under the light microscope (Nikon Eclipse 80i, Japan).
2.4. Haematology, urine protein creatinine, and biochemical analysis
Celltac Alpha VET® MEK-6550K (Nihon Kohden, Japan) was applied to the blood sample to measure haematological parameters, including total erythrocytes, haemoglobin (Hb), packed cell volume (PCV), mean corpuscular volume (MCV), mean corpuscular haemoglobin concentration (MCHC), and platelet count. Additionally, a blood smear was performed by utilising the Wright stain to differentiate leukocytes by counting 100 cells and multiplying by the total leukocyte count from the automated haematology analyser (von Konigslow et al., Citation2019). Furthermore, blood serum was evaluated for biochemical analyses, including urea, creatinine, creatinine kinase (CK), alanine transaminase (ALT), aspartate transaminase (AST), total protein, and albumin, with an automated clinical chemistry analyser (Biolis 24i Premium® chemistry analyser, Tokyo, Japan) (Lim et al., Citation2019). The urine protein creatinine ratio (UPCr) was also evaluated using a Biolis 24i automated machine.
2.5. Relative kidney weight analysis
The kidney organs were collected and weighed. Each animal’s relative organ was determined by the mice’s organ weight divided by body weight and multiplied by 100% (Nurul et al., Citation2018).
2.6. Histopathological analysis
A gross and histopathological examination of animal kidneys in each group was conducted. The animals’ kidneys were collected and preserved in 10% neutral buffered formalin. Tissues were trimmed, embedded in paraffin, sectioned, and stained with Haematoxylin and Eosin (Rowley et al., Citation2020). The kidney histology was observed under a light microscope with 400x magnification. The scoring system follows Gibson-Corley (Gibson-Corley et al., Citation2013). Scoring refers to the distribution of kidney lesions, where no histological damage (score 0), <25% damage (score 1), 26–50% damage (score 2), 51–75% damage (score 3), and 76–100% damage (score 4).
2.7. Statistical analysis
Data expressed as mean ± standard error (SE) was performed using IBM SPSS Statistics version 26 for Windows, and graphs were created using GraphPad Prism version 9. Relative kidney-to-weight ratio, urine profile, UPCr, haematology, and biochemistry were analysed using one-way analysis of variance (ANOVA) followed by Duncan’s post-hoc test. Meanwhile, a Kruskal-Wallis nonparametric test followed by Dunn’s test was performed to analyse urine sedimentation and the histological evaluation of the kidneys. p-Values that were ≤0.05 (p ≤ 0.05) were considered statistically significant (Riffenburgh & Gillen, Citation2020).
3. Results
3.1. Effect of MLE and CGA in UUO-induced CKD mice on urinalysis result
The results of urine analysis in the UUO mice given MLE and CGA are shown in . The urine analysis parameters had significant differences between groups. The urinalysis results showed that the UUO-induced mice exhibited changes in the urine profile, including increased ketones, proteins, leukocytes, and erythrocytes (p < 0.05). Meanwhile, the administration of enalapril could reduce leukocytes in the urine. Interestingly, the mice treated with CGA or MLE indicated reduced ketones, proteins, and erythrocytes in the urine but not in leucocytes. On the other hand, the urine sedimentation observation displayed that the UUO group had more different types of urine crystals than the SO group (). Kruskal Wallis test result presented less urine crystal formation in MLE and CGA administration than in the UUO group (). In addition, depicts urine crystals found in fresh urine samples, such as triple phosphate, calcium oxalate, calcium carbonate, and epithelial. Meanwhile, treatment with enalapril revealed significant (p < 0.05) improvement in kidney function by a decline in UPCr compared to the UUO group (). Also, the CGA treatment had significantly (p < 0.05) lower UPCr than the UUO group. Meanwhile, although insignificant, the MLE treatment group indicated a decrease in UPCr.
Figure 1. (A) types of urine sedimentation on all groups n = 5; (B) Kruskal-Wallis’s result on urine crystal formation (Significance *p < 0.05 vs. UUO group, n = 5); (C) Urine crystal image with 40–400× magnification: (i) Calcium oxalate (black arrow) and calcium carbonate (arrowhead) (40×); (ii) calcium oxalate (400×); (iii) calcium carbonate (blue arrowhead) (100×); (iv) triple phosphate (red arrow head) (100×); (v) triple phosphate (red arrow head) (400×); (vi) epithelial squamous (red arrowhead) and triple phosphate (yellow arrowhead) (100×). SO: sham operation; UUO: unilateral urethral obstruction; ENA: enalapril; MLE: Morus alba leaves extract; CGA: chlorogenic acid; Significance: ns: not significant; *p < 0.05 vs. UUO group.
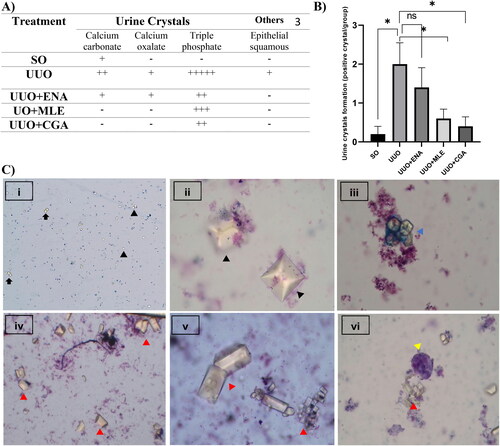
Figure 2. The urine protein creatinine ratio on enalapril, Morus alba leaves extract, and chlorogenic acid administration in the UUO mice. SO: sham operation; UUO: unilateral urethral obstruction; ENA: enalapril; MLE: Morus alba leaves extract; CGA: chlorogenic acid; Significance: ns: not significant; *p < 0.05 vs. UUO group.
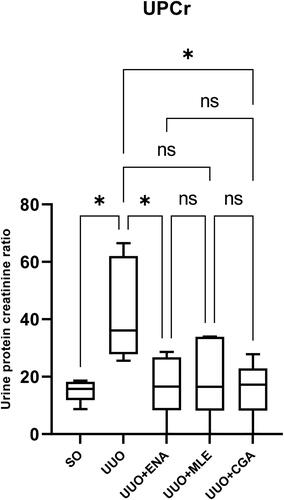
Table 1. The urine analysis result of UUO mice administrated with enalapril, Morus alba leaf extract, and chlorogenic acid for 14 days.
3.2. Effect of MLE and CGA in UUO-induced CKD mice on haematology and kidney biochemistry result
A complete blood count of UUO mice treated with MLE and CGA for 14 days is presented in . Haematology parameters in the UUO group had a significant decrease (p < 0.05) in red blood cell count, haemoglobin, and haematocrit compared to the SO group. The mean MCV in the UUO group was higher than in the SO group, while MCHC was lower than in the SO group. The UUO group eventually revealed mild macrocytic hypochromic anaemia. Compared to the UUO group, the ENA, MLE, and CGA groups improved anaemia, as evidenced by increasing total red blood cell count, haemoglobin, and haematocrit. The total number of white blood cells (WBC) was raised in the UUO group compared to the SO group (p < 0.05). In addition, treatment with ENA, MLE, and CGA revealed a trend towards a decrease in WBC, neutrophils, and lymphocytes compared to the UUO group, although this was not significant. Meanwhile, there were no variations in platelet count among all groups.
Table 2. Haematology of the UUO mice administrated with enalapril, Morus alba leaf extract, and chlorogenic acid for 14 days.
The serum biochemistry results revealed that the UUO group significantly raised the creatinine (p < 0.001) and urea (p < 0.05) levels in comparison to the SO group (). In contrast, the CGA group dramatically lowered creatinine levels (p < 0.05), but ENA and MLE treatments only exhibited a trend of decreasing creatinine levels when compared to the UUO group. Moreover, although not statistically significant (p > 0.05), the ENA, MLE, and CGA groups demonstrated a decreasing trend in urea levels compared to the UUO group. Total protein, albumin, and globulin were significantly higher (p < 0.05) in the UUO group compared to the SO group. Conversely, total protein, albumin, and globulin values in UUO-induced mice treated with ENA, MLE, or CGA were barely lower than in the UUO group. Yet the muscle enzyme CK and the liver enzymes AST and ALT did not significantly differ between the groups.
Figure 3. Biochemistry analyses of the UUO Mice treated with enalapril, Morus alba Leaf extract and chlorogenic acid. Data values are presented as mean ± SEM; Asterisks symbol (a–c) shows statistical significance between the groups (n = 5). SO: sham operation; UUO: unilateral urethral obstruction; ENA: enalapril; MA: Morus alba; CGA: chlorogenic acid; AST: aspartate aminotransferase; ALT: alanine aminotransferase; CK: creatine kinase; MLE: Morus alba leaves extract.
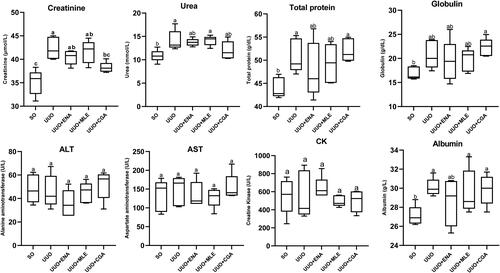
3.3. Effect of MLE and CGA in UUO-induced CKD mice on kidney morphology and histopathology
The gross anatomy of the mice’s kidneys treated with MLE and CGA on the UUO mice is illustrated in . Gross anatomy showed that the UUO mice had an enlarged kidney (), paler kidney colour, and hydronephrosis around the renal pelvis. The UUO mice treated with ENA, MLE, and CGA had an improved kidney structure with less hydronephrosis than the UUO non-treatment group. A collateral kidney, which was not blocked by an obstruction, displayed normal colour in each group. The UUO group appeared to have a larger kidney volume.
Figure 4. Gross anatomy of mice kidneys treated with enalapril, Morus alba leaves extract, and chlorogenic acid for 14 days in the UUO mice. (A) Sham operation (negative control), (B) UUO (positive control), (C) UUO treated with enalapril, (D) UUO treated with Morus alba leaves extract, (E) UUO treated with chlorogenic acid, (F) UUO Kidney to body weight ratio in Morus alba and chlorogenic acid treatment in the mice. (A,B) Collateral unobstructed kidney to body weight ratio in Morus alba and chlorogenic acid treatment in the mice. SO: sham operation; UUO: unilateral urethral obstruction; ENA: enalapril; MLE: Morus alba leaves extract; CGA: chlorogenic acid; Significance: ns: not significant; *p < 0.05; ****p < 0.0001.
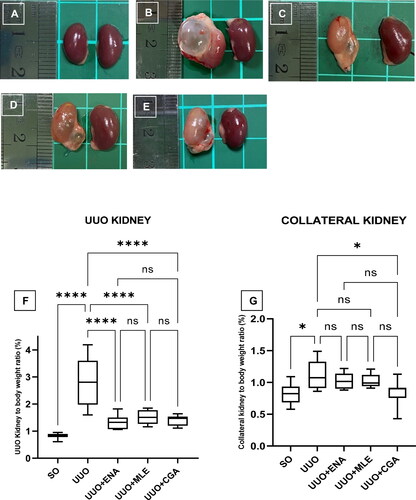
The kidney-to-body weight ratio in the UUO kidney and collateral unblocked kidney mice is shown in . The kidney-relative organ ratio was highest in the UUO group, and it increased significantly (p < 0.0001) in comparison to the SO, ENA, and MLE groups (). Similarly, the collateral kidney also exhibited a significant increase in kidney relative organ (p < 0.05) in the UUO group compared to the SO and CGA groups (). Meanwhile, the collateral kidney in the ENA and MLE groups had no significance compared to the UUO group.
Figure 5. Histology of kidney mice administrated with enalapril, Morus alba leaves extract, and chlorogenic acid in Haematoxylin and Eosin (H&E) staining. (A) Sham operation group (10 µm scale bar) shows the normal structure of glomerulus and tubules; (B) UUO group depicts interstitial fibrosis, Bowman’s space dilatation, and tubular epithelial cell loss with the intralobular eosinophilic cast (50 µm scale bar); (C) UUO group with higher magnification of group figure B (10 µm scale bar); (D) UUO group shows interstitial fibrosis, dilatation of tubules and infiltration of the inflammatory cell (10 µm scale bar); (E) UUO treated with enalapril group (10 µm scale bar); (F) UUO treated with Morus alba leaves extract (10 µm scale bar); (G) UUO treated with chlorogenic acid (10 µm scale bar). G: glomerulus; P: proximal tubule; D: distal tubule; yellow arrow: intralobular cast; blue arrow: fibrosis; red arrow: cellular inflammatory; black arrow: tubular dilation.
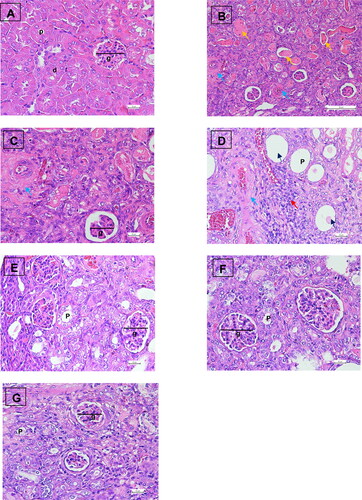
A photomicrograph of the kidney histopathology of UUO mice treated with MLE and CGA in Haematoxylin and Eosin (H&E) staining is illustrated in . The kidney histology demonstrated the normal structure of the SO group’s kidney parenchyma, glomeruli, and tubules. On the other hand, the UUO group had tubulointerstitial fibrosis, Bowman’s capsule dilatation, tubular epithelial cell loss with eosinophilic cast, and infiltrations of inflammatory cells. The kidney histology observation of the ENA, MLE, and CGA treatment groups also improved due to fewer areas of Bowman’s space dilatation, tubular dilatation, tubular epithelial loss, cellular inflammation, and eosinophilic intralobular cast.
The kidney scoring result using the nonparametric Kruskal Wallis test is presented in . In the SO group, histopathological examination of the renal tissue revealed that the glomeruli and tubules were normal, without cellular inflammation or protein cast. The histopathological scores in the UUO group were significantly higher than in the other groups. Treatment with ENA inhibited tissue damage, as evidenced by a reduction in Bowman’s space enlargement, cellular inflammation, and intralobular cast. Meanwhile, treatment with MLE showed fewer tubular dilatations than the UUO groups. Interestingly, CGA treatment indicates the best positive effect on renal tissue damage, as reflected by a reduction in Bowman’s space dilatation, tubular dilatation, epithelial cell loss, cellular inflammation, and intralobular protein cast.
Table 3. Kidney histology scoring of the UUO mice administrated with enalapril, Morus alba leaf extract, and chlorogenic acid for 14 days.
4. Discussion
In this current study, UUO was performed by ligating the right kidney to induce progressive renal fibrosis. According to urinalysis results, UUO-induced renal fibrosis in mice led to alterations to urine composition, including ketonuria, proteinuria, leukocyturia, and haematuria. This urine abnormality occurred due to renal obstruction injury and increased activity on non-obstructive collateral renal during blood filtration. There was also evidence of enlargement in the kidney morphology on a macroscopic observation. In the meantime, studies have revealed that high proteinuria has been associated with interstitial fibrosis (Lee et al., Citation2019; Yu et al., Citation2022). The UUO group also exhibited higher quantities of crystalline normal urine, which included calcium carbonate, calcium oxalate, and triple phosphate. Based on daily observations of the UUO group, it was assumed that the reduced urine volume was the cause of this finding. Indeed, according to a previous study, low urine production causes higher urine saturation, which increases the risk of crystal urine sedimentation (Travers et al., Citation2023).
Furthermore, this study used enalapril as a positive control drug because it has been reported to prevent renal damage in renal fibrosis (Liao et al., Citation2022). In this study, the treatment with enalapril in UUO mice significantly decreased the number of leukocytes in the urine. It may also preserve normal urine content. Based on urine sedimentation tests, enalapril counteracts the formation of urine crystals in renal fibrosis, such as calcium carbonate, calcium oxalate, and triple phosphate. It is well-known that enalapril belongs to the class of angiotensin-converting enzyme inhibitors (ACE -I), a class of drugs that lower blood pressure, fluid retention, and sodium levels (Smeets et al., Citation2020). A study found that ACE inhibitors could decrease urine crystal formation in kidney stone disease (Khan & Canales, Citation2023).
On the other hand, treatment with MLE and CGA in this study improved the urine profiles, i.e. urine proteins, ketones, and erythrocytes. The urine sedimentation data demonstrated that administering MLE and CGA to the UUO animal model suppressed urine crystal formation. The results are consistent with an earlier study reporting that the leaves of Morus sp. have an anti-urolithic effect (Bolda Mariano et al., Citation2023). Indeed, according to Kant et al., the pharmacological activity of plants as anti-urolithiasis is based on their anti-hypercalciuria, diuretic, antioxidant, anti-inflammatory, and crystal aggregation-suppressive properties (Kant et al., Citation2020).
Moreover, a study has demonstrated that urine protein creatinine ratio (UPCr) will be increased in obstructed kidneys (Wu et al., Citation2012). In fact, spot urinary protein: creatinine ratios (UPCr) are commonly used to evaluate the quantitative determination of proteinuria (Kamińska et al., Citation2020). In this study, the UUO group had the highest UPCr value compared to other groups. Meanwhile, the CGA group displayed the best prevention in reducing proteinuria rate in kidney damage, followed by the enalapril and Morus alba groups. Presumably, this is because the ability of CGA to maintain stable renal function may be due to its blood pressure-lowering effect, which in turn keeps the filtration rate of the glomeruli. Indeed, it is well-established that CGA has a blood pressure-lowering effect (Zhu et al., Citation2022). The same result was observed with MLE, which could preserve renal function as demonstrated by a drop in UPCr levels. It has been suggested that the MLE's capability is related to the mice’s blood pressure-lowering action. Indeed, according to Nade et al., the leaves of Morus alba have cardioprotective and antihypertensive effects (Nade et al., Citation2013).
In this study, the haematology result indicated mild anaemia due to renal obstruction in the UUO group, characterised by a decrease in erythrocyte, haemoglobin, and haematocrit. Our results are consistent with previous studies demonstrating that urethral ligation causes anaemia due to decreased haematocrit in the mice (Tasanarong et al., Citation2017). The UUO group in this study indicated mild macrocytic hypochromic anaemia, which might be linked with urethral obstruction in mice. Urethral obstruction has been associated with kidney damage, leading to decreased erythropoietin production and contributing to anaemia in affected animals (Chevalier et al., Citation2009). There was also an inflammatory reaction in the UUO group, as seen by leukocytosis, neutrophilia, and lymphocytosis. It was because of the ureteral obstruction, which increases oxidative stress due to the accumulation of urinary toxins in the renal pelvis. A study has revealed that obstructive nephropathy induces leukocyte recruitment and interstitial fibrosis (Grande et al., Citation2010). Interestingly, ENA, MLE, and CGA treatments reduced the risk of anaemia in UUO-induced mice, resulting in normal red blood cells, haemoglobin, and haematocrit levels. Moreover, MLE-treated UUO mice could also attenuate inflammatory responses by decreasing the total leukocyte count in the haematology assay. The findings supported a prior study that found Morus alba leaves can increase haematological levels, reduce anaemia, and heal inflammation in animal trials (Neamat-Allah et al., Citation2021).
In this study, biochemical analysis demonstrated that ureteral obstruction in the UUO group was related to reduced renal function, as evidenced by higher urea and creatinine levels. On the other hand, enalapril improved renal function as measured by a decrease in urea and serum creatinine. Surprisingly, CGA outperformed ENA and MLE regarding therapeutic efficacy, as indicated by its capacity to maintain normal creatinine levels. The results were consistent with a study that found a CGA dose of 20–40 mg/kg could lower blood urea nitrogen and creatinine in mice with lead-induced kidney injury (Zhang et al., Citation2019). It is widely acknowledged that CGA's capacity to preserve renal function results from its ability to prevent oxidative stress-related kidney damage. Indeed, CGA has a high antioxidant and anti-inflammatory activity, contributing to redox imbalance (Yin et al., Citation2022).
Furthermore, the total protein, globulin, and albumin characteristics in the UUO group were increased significantly compared to the SO group. It is presumably associated with hydronephrosis in the kidney and increases cellular inflammation, which can be seen in macroscopic and microscopic observations. According to Washino et al., in hydronephrosis, the filtration rate of the blood is impaired, and kidney injury and inflammation can increase serum protein levels in the blood (Washino et al., Citation2020). However, all UUO-induced mice showed no significant difference in ALT and AST results compared to the SO group. It is believed that the renal obstruction induced by UUO had a local impact on the urinary tract, so it did not affect liver enzyme activity (Wang et al., Citation2022). This finding is consistent with recent studies showing that UUO-induced experimental animals can exacerbate kidney damage without affecting liver function (Xu et al., Citation2021; Zhang et al., Citation2013). On the other hand, treatment with enalapril, MLE, and CGA could not restore the total protein, albumin, and globulin in UUO-induced mice despite a lowering trend.
This study’s examination of renal gross anatomy revealed that ureter ligase can increase urine volume in the renal pelvis, resulting in hydronephrosis. The UUO group’s kidney samples displayed hydronephrosis, indicating that the renal pelvic area was filled with fluid (). It is well known that hydronephrosis can induce haemodynamic abnormalities and injury to the corticomedullary region. This damage was supported by the finding in this study that showed enlargement in renal volume and a higher kidney weight-to-organ ratio. In addition, hydronephrosis also could increase blood pressure with an increase in the ACE (Zhang et al., Citation2015). Furthermore, this study found that UUO mice administered enalapril had fewer hydronephrosis events, as revealed by renal morphology with low fluid deposition in the renal pelvic area. Enalapril’s ability to suppress hydronephrosis is due to the drug’s antihypertensive action, which affects filtration rate and intraglomerular blood pressure. This was similar to prior research, which found that enalapril dramatically lowered ACE, blood pressure, and the incidence of hydronephrosis (Ferri et al., Citation2022; Zhang et al., Citation2013).
The treatment of MLE and CGA indicated a tendency to minimise the occurrence of hydronephrosis, with less fluid deposition noticed in the renal pelvic area than in the UUO group. According to a prior study, the efficacy of MLE and CGA to prevent hydronephrosis may be related to a blood pressure-lowering action (Nade et al., Citation2013; Zhu et al., Citation2022). The lower incidence of hydronephrosis was consistent with the results of renal volume and weight measurements in this study. Based on the results of the kidney-to-body weight ratio measurements in the UUO kidney (right kidney) and collateral kidney (left kidney), the UUO group had an increase in the kidney-to-body weight ratio compared to the control group. Meanwhile, the MLE and CGA therapy displayed improved renal morphology and normalised the renal volume and weight in UUO-induced mice, similar to enalapril.
The histological examination of the kidneys with HE staining in the UUO group showed renal damage characterised by increased infiltration of inflammatory cells and damage to the renal tubules. Tubular epithelium loss, tubular dilatation, intralobular eosinophil loss, and inflammatory cell infiltration were all observed in the UUO group. Based on the results of the Kruskal-Wallis evaluation of the kidney histological scoring, the UUO group had a greater width of Bowman’s space and tubular damage followed by tubular epithelial necrosis, increased infiltration of inflammatory cells, and intralobular cast accumulation. Meanwhile, in renal histology observation, UUO mice treated with enalapril exhibited prevention of tubular damage and inflammation. Indeed, enalapril effectively prevented kidney damage by lowering glomerular capillary filtration pressure and inhibiting the synthesis of profibrotic molecules (Sun et al., Citation2016).
Despite this, renal histology improved more with CGA treatment. In particular, Bowman’s space dilatation, tubular dilatation, tubular epithelium loss, and cellular inflammation were all improved. This result was in line with earlier research that showed CGA dramatically enhanced renal histopathology in kidney ischemia injury studies by lowering tubular damage and enhancing epithelial cell proliferation (Arfian et al., Citation2019). Additionally, another study reported that CGA has an anti-inflammatory effect that could reduce inflammation and apoptosis in renal nephrotoxic renal tissue by arsenic (Al-Megrin et al., Citation2020).
It is well known that the ability of MLE to inhibit the progression of kidney damage is due to the high chlorogenic acid content in the extract. Chlorogenic acid is one of the major phenolic acid compounds in the leaves of Morus alba, followed by gallic acid, vanillic acid, hydroxybenzoic acid, syringic acid, p-coumaric acid, protocatechuic acid, ferulic acid and m-coumaric acid (Sánchez-Salcedo et al., Citation2015). Our previous study showed that MLE has a total phenolic content of 11 mg GAE/g dry DW, a total flavonoid content of 257 mg QE/g DW, and antioxidant activity (602 IC50 μg/mL) and a high chlorogenic acid content (306 mg/100g dry weight). As a result, it could be linked to the current study’s prevention findings on the progression of renal damage in UUO-induced mice. Indeed, as the major phenolic compound in plants, CGA has been reported to cure organ damage by alleviating inflammation and injury in various targets (Nwafor et al., Citation2022). CGA also has high antioxidant and anti-inflammatory activity to cure metabolic diseases via modulating Nrf2/HO-1 and NF-ĸB signalling pathways (Bao et al., Citation2018). Thus, the abilities of MLE and CGA to protect the kidneys in UUO mice may provide an alternative treatment to delay the onset of renal insufficiency.
5. Conclusion
In conclusion, the administration of MLE and CGA had a therapeutic effect by improving renal anatomy with less hydronephrosis, normalising renal weight, and preventing crystal sedimentation, UPCr, creatinine, and histopathological changes. The MLEs, especially the CGA derivatives, have the potential to inhibit the kidney damage and are promoted as therapies that can reduce the progression of kidney damage.
Author contributions
Ahmad Fauzi: performing experiment, data curation, formal analysis, methodology, validation, writing–original draft; Nurina Titisari: performing experiment, review and editing; Mohd. Hezmee Mohd. Noor: methodology, supervision; Hazilawati Hamzah: conceptualization, validation, methodology, writing–review, and editing; Azrina Azlan: conceptualisation, funding acquisition, validation, project administration, supervision, resources, review, and editing. All data were generated in-house, and no paper mill was used. Authors agree to be accountable for all aspects of work, ensuring integrity and accuracy.
Public interest statement.docx
Download MS Word (13.3 KB)About the author.docx
Download MS Word (13.4 KB)Acknowledgements
The authors would like to thank the Clinical Pathology Laboratory, Histology Laboratory, Faculty of Veterinary Medicine, Universiti Putra Malaysia (UPM), and the Nutrition Laboratory, Faculty of Medicine and Health Sciences, UPM, for providing the facilities for this study. All staff of Universiti Putra Malaysia were thanked for their technical assistance in conducting this study.
Disclosure statement
No potential conflict of interest was reported by the author(s).
Data availability statement
Data will be made available on request.
Additional information
Funding
Notes on contributors
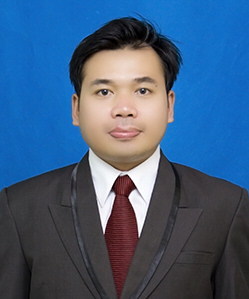
Ahmad Fauzi
Ahmad Fauzi is a veterinarian and an assistant professor within the Faculty of Veterinary Medicine at the University of Brawijaya in East Java, Indonesia. He is pursuing a doctoral degree at the Faculty of Veterinary Medicine, Universiti Putra Malaysia, with a special interest in clinical pathology. His research pursuits centre around herbal medicine and kidney diseases. Accumulating 20 research and community service grants, Dr. Fauzi has authored and co-authored 33 publications encompassing books, journals, papers, and technical guidelines. Furthermore, he engaged in clinical work at an animal hospital, concurrently serving as the director of the diagnostic laboratory at Brawijaya University Animal Teaching Hospital. In his capacity as a veterinarian, he maintains active membership within the Indonesian Small Animal Practitioner Veterinary Association (ISAPVA) and the Indonesian Association for Laboratory Animal Sciences (IALAS).
Nurina Titisari
Nurina Titisari is an assistant professor in veterinary physiology at the Faculty of Veterinary Medicine, University of Brawijaya.
Mohd. Hezmee Mohd. Noor
Mohd. Hezmee Mohd. Noor is a lecturer at the Faculty of Veterinary Medicine, Universiti Putra Malaysia. His areas of expertise are clinical pharmacology and toxicology.
Azrina Azlan
Azrina Azlan is a professional technologist recognised by the Malaysian Board of Technologists (MBOT), and she is a professor in the Department of Nutrition at the Faculty of Medicine and Health Sciences, Universiti Putra Malaysia.
Hazilawati Hamzah
Hazilawati Hamzah is a lecturer at the Faculty of Veterinary Medicine, Universiti Putra Malaysia. Her areas of expertise are cytopathology, blood vessel disease, blood cancer, and ethnopharmacology.
References
- Abecassis, M., Bartlett, S. T., Collins, A. J., Davis, C. L., Delmonico, F. L., Friedewald, J. J., Hays, R., Howard, A., Jones, E., Leichtman, A. B., Merion, R. M., Metzger, R. A., Pradel, F., Schweitzer, E. J., Velez, R. L., & Gaston, R. S. (2008). Kidney transplantation as primary therapy for end-stage renal disease: A National Kidney Foundation/Kidney Disease Outcomes Quality Initiative (NKF/KDOQI™) conference. Clinical Journal of the American Society of Nephrology, 3(2), 1–15. https://doi.org/10.2215/CJN.05021107
- Al-Megrin, W. A., Metwally, D. M., Habotta, O. A., Amin, H. K., Abdel Moneim, A. E., & El-Khadragy, M. (2020). Nephroprotective effects of chlorogenic acid against sodium arsenite-induced oxidative stress, inflammation, and apoptosis. Journal of the Science of Food and Agriculture, 100(14), 5162–5170. https://doi.org/10.1002/jsfa.10565
- Arfian, N., Wahyudi, D. A. P., Zulfatina, I. B., Citta, A. N., Anggorowati, N., Multazam, A., Romi, M. M., & Sari, D. C. R. (2019). Chlorogenic acid attenuates kidney ischemic/reperfusion injury via reducing inflammation, tubular injury, and myofibroblast formation. BioMed Research International, 2019, 5423703. https://doi.org/10.1155/2019/5423703
- Bao, L., Li, J., Zha, D., Zhang, L., Gao, P., Yao, T., & Wu, X. (2018). Chlorogenic acid prevents diabetic nephropathy by inhibiting oxidative stress and inflammation through modulation of the Nrf2/HO-1 and NF-ĸB pathways. International Immunopharmacology, 54, 245–253. https://doi.org/10.1016/j.intimp.2017.11.021
- Bolda Mariano, L. N., Cechinel-Zanchett, C. C., Nazário, D., Lupatini, K., & de Souza, P. (2023). Dose-dependent dual effect of Morus nigra L. extract on urinary parameters of hypertensive rats. Chemistry & Biodiversity, 20(5), e202300017. https://doi.org/10.1002/cbdv.202300017
- Chen, C., Mokhtar, R., A., M., Sani, M., S. A., Noor, & N. Q. I., M. (2022). The effect of maturity and extraction solvents on bioactive compounds and antioxidant activity of mulberry (Morus alba) fruits and leaves. Molecules, 27(8), 1–20. https://doi.org/10.3390/molecules27082406
- Chen, C., Razali, U. H. M., Saikim, F. H., Mahyudin, A., & Noor, N. Q. I. M. (2021). Morus alba L. plant: Bioactive compounds and potential as a functional food ingredient. Foods, 10(3), 1–28. https://doi.org/10.3390/foods10030689
- Chevalier, R. L., Forbes, M. S., & Thornhill, B. A. (2009). Ureteral obstruction as a model of renal interstitial fibrosis and obstructive nephropathy. Kidney International, 75(11), 1145–1152. https://doi.org/10.1038/ki.2009.86
- Cho, M. H., Jung, K.-J., Jang, H.-S., Kim, J. I., & Park, K. M. (2012). Orchiectomy attenuates kidney fibrosis after ureteral obstruction by reduction of oxidative stress in mice. American Journal of Nephrology, 35(1), 7–16. https://doi.org/10.1159/000334598
- Ferri, N., Corsini, A., & Pontremoli, R. (2022). Antihypertensive treatment with calcium channel blockers and renal protection: Focus on lercanidipine and lercanidipine/enalapril. European Review for Medical and Pharmacological Sciences, 26, 7482–7492. https://doi.org/10.26355/eurrev_202210_30018
- Gibson-Corley, K. N., Olivier, A. K., & Meyerholz, D. K. (2013). Principles for valid histopathologic scoring in research. Veterinary Pathology, 50(6), 1007–1015. https://doi.org/10.1177/0300985813485099
- Grande, M. T., Pérez-Barriocanal, F., & López-Novoa, J. M. (2010). Role of inflammation in tbulo-interstitial damage associated to obstructive nephropathy. Journal of Inflammation, 7, 19. https://doi.org/10.1186/1476-9255-7-19
- Gryn-Rynko, A., Bazylak, G., & Olszewska-Slonina, D. (2016). New potential phytotherapeutics obtained from white mulberry (Morus alba L.) leaves. Biomedicine & Pharmacotherapy, 84, 628–636. https://doi.org/10.1016/j.biopha.2016.09.081
- Ham, O., Jin, W., Lei, L., Huang, H. H., Tsuji, K., Huang, M., Roh, J., Rosenzweig, A., & Lu, H. A. J. (2018). Pathological cardiac remodeling occurs early in CKD mice from unilateral urinary obstruction, and is attenuated by Enalapril. Scientific Reports, 8(1), 16087. https://doi.org/10.1038/s41598-018-34216-x
- Hedenqvist, P. (2021). Handbook of laboratory animal science: Essential principles and practices (pp. 343–378). CRC Press.
- Hesketh, E. E., Vernon, M. A., Ding, P., Clay, S., Borthwick, G., Conway, B., & Hughes, J. (2014). A murine model of irreversible and reversible unilateral ureteric obstruction. Journal of Visualized Experiments, (94), 52559. https://doi.org/10.3791/52559
- Huang, Y.-R., Wei, Q.-X., Wan, Y.-G., Sun, W., Mao, Z.-M., Chen, H.-L., Meng, X.-J., Shi, X.-M., Tu, Y., & Zhu, Q. (2014). Ureic clearance granule, alleviates renal dysfunction and tubulointerstitial fibrosis by promoting extracellular matrix degradation in renal failure rats, compared with enalapril. Journal of Ethnopharmacology, 155(3), 1541–1552. https://doi.org/10.1016/j.jep.2014.07.048
- Hwang, S. J., Kim, Y. W., Park, Y., Lee, H. J., & Kim, K. W. (2014). Anti-inflammatory effects of chlorogenic acid in lipopolysaccharide- stimulated RAW 264.7 cells. Inflammation Research, 63(1), 81–90. https://doi.org/10.1007/S00011-013-0674-4/FIGURES/7
- Jan, B., Zahiruddin, S., Basist, P., Irfan, M., Abass, S., & Ahmad, S. (2022). Metabolomic profiling and identification of antioxidant and antidiabetic compounds from leaves of different varieties of Morus alba Linn grown in Kashmir. ACS Omega, 7(28), 24317–24328. https://doi.org/10.1021/acsomega.2c01623
- Kamińska, J., Dymicka-Piekarska, V., Tomaszewska, J., Matowicka-Karna, J., & Koper-Lenkiewicz, O. M. (2020). Diagnostic utility of protein to creatinine ratio (P/C ratio) in spot urine sample within routine clinical practice. Critical Reviews in Clinical Laboratory Sciences, 57(5), 345–364. https://doi.org/10.1080/10408363.2020.1723487
- Kant, R., Singh, T. G., & Singh, S. (2020). Mechanistic approach to herbal formulations used for urolithiasis treatment. Obesity Medicine, 19, 100266. https://doi.org/10.1016/j.obmed.2020.100266
- Khan, M. A., Kassianos, A. J., Hoy, W. E., Alam, A. K., Healy, H. G., & Gobe, G. C. (2022). Promoting plant-based therapies for chronic kidney disease. Journal of Evidence-Based Integrative Medicine, 27, 2515690X221079688. https://doi.org/10.1177/2515690X221079688
- Khan, S. R., & Canales, B. K. (2023). Proposal for pathogenesis-based treatment options to reduce calcium oxalate stone recurrence. Asian Journal of Urology, 10(3), 246–257. https://doi.org/10.1016/j.ajur.2023.01.008
- Kim, D.-S., Kang, Y. M., Jin, W. Y., Sung, Y.-Y., Choi, G., & Kim, H. K. (2014). Antioxidant activities and polyphenol content of Morus alba leaf extracts collected from varying regions. Biomedical Reports, 2(5), 675–680. https://doi.org/10.3892/BR.2014.294
- Kovesdy, C. P. (2022). Epidemiology of chronic kidney disease: An update 2022. Kidney International Supplements, 12(1), 7–11. https://doi.org/10.1016/j.kisu.2021.11.003
- Lee, J., An, J. N., Hwang, J. H., Lee, H., Lee, J. P., & Kim, S. G. (2019). P38 MAPK activity is associated with the histological degree of interstitial fibrosis in IgA nephropathy patients. PLOS One, 14(3), e0213981. https://doi.org/10.1371/journal.pone.0213981
- Liao, X., Lv, X., Zhang, Y., Han, Y., Li, J., Zeng, J., Tang, D., Meng, J., Yuan, X., Peng, Z., Tao, L., & Xie, Y. (2022). Fluorofenidone inhibits UUO/IRI-induced renal fibrosis by reducing mitochondrial damage. Oxidative Medicine and Cellular Longevity, 2022, 2453617. https://doi.org/10.1155/2022/2453617
- Lim, K. C., Yusoff, F. M., Shariff, M., Kamarudin, M. S., & Nagao, N. (2019). Dietary supplementation of astaxanthin enhances hemato-biochemistry and innate immunity of Asian seabass, Lates calcarifer (Bloch, 1790). Aquaculture, 512, 734339. https://doi.org/10.1016/j.aquaculture.2019.734339
- Liu, D., Cheng, F., Pan, S., & Liu, Z. (2020). Stem cells: A potential treatment option for kidney diseases. Stem Cell Research & Therapy, 11, 1–20. https://doi.org/10.1186/s13287-020-01751-2
- Ma, G., Chai, X., Hou, G., Zhao, F., & Meng, Q. (2022). Phytochemistry, bioactivities and future prospects of mulberry leaves: A review. Food Chemistry, 372, 131335. https://doi.org/10.1016/j.foodchem.2021.131335
- Martínez-Klimova, E., Aparicio-Trejo, O. E., Tapia, E., & Pedraza-Chaverri, J. (2019). Unilateral ureteral obstruction as a model to investigate fibrosis-attenuating treatments. Biomolecules, 9(4), 141. https://doi.org/10.3390/biom9040141
- Maya, S., & Pramod, C. (2014). Evaluation of anti-nephrolithiatic activity of ethanolic leaf extract of Morus alba L. in animal models. International Research Journal Of Pharmacy, 5, 427–433. https://doi.org/10.7897/2230-8407.050588
- Nade, V. S., Kawale, L. A., Bhangale, S. P., & Wale, Y. B. (2013). Cardioprotective and antihypertensive potential of Morus alba L. in isoproterenol-induced myocardial infarction and renal artery ligation-induced hypertension. Journal of Natural Remedies, 13, 54–67.
- Neamat-Allah, A. N. F., Mahmoud, E. A., & Mahsoub, Y. (2021). Effects of dietary white mulberry leaves on hemato-biochemical alterations, immunosuppression and oxidative stress induced by Aeromonas hydrophila in Oreochromis niloticus. Fish & Shellfish Immunology, 108, 147–156. https://doi.org/10.1016/j.fsi.2020.11.028
- Nematbakhsh, M., Hajhashemi, V., Ghannadi, A., Talebi, A., & Nikahd, M. (2013). Protective effects of the Morus alba L. leaf extracts on cisplatininduced nephrotoxicity in rat. Research in Pharmaceutical Sciences, 8(2), 71–77.
- Nurul, S. A. S., Hazilawati, H., Mohd, R. S., Mohd, F., H., R., Noordin, M. M., & Norhaizan, M. E. (2018). Subacute oral toxicity assesment of ethanol extract of Mariposa Christia vespertilionis leaves in male Sprague Dawley rats. Toxicological Research, 34(2), 85–95. https://doi.org/10.5487/TR.2018.34.2.085
- Nwafor, E. O., Lu, P., Zhang, Y., Liu, R., Peng, H., Xing, B., Liu, Y., Li, Z., Zhang, K., Zhang, Y., & Liu, Z. (2022). Chlorogenic acid: Potential source of natural drugs for the therapeutics of fibrosis and cancer. Translational Oncology, 15(1), 101294. https://doi.org/10.1016/j.tranon.2021.101294
- Owumi, S. E., Olusola, J. K., Arunsi, U. O., & Oyelere, A. K. (2021). Chlorogenic acid abates oxido-inflammatory and apoptotic responses in the liver and kidney of Tamoxifen-treated rats. Toxicology Research, 10(2), 345–353. https://doi.org/10.1093/toxres/tfab002
- Rapa, S. F., Di Iorio, B. R., Campiglia, P., Heidland, A., & Marzocco, S. (2020). Inflammation and oxidative stress in chronic kidney disease—Potential therapeutic role of minerals, vitamins and plant-derived metabolites. International Journal of Molecular Sciences, 21(1), 263. https://doi.org/10.3390/IJMS21010263
- Rhee, C. M., & Kovesdy, C. P. (2015). Spotlight on CKD deaths—Increasing mortality worldwide. Nature Reviews. Nephrology, 11(4), 199–200. https://doi.org/10.1038/NRNEPH.2015.25
- Riffenburgh, R. H., & Gillen, D. L. (2020). Statistics in medicine. Academic Press.
- Rowley, J. E., Rubenstein, G. E., Manuel, S. L., Johnson, N. L., Surgnier, J., Kapitsinou, P. P., Duncan, F. E., & Pritchard, M. T. (2020). Tissue-specific fixation methods are required for optimal in situ visualization of hyaluronan in the ovary, kidney, and liver. The Journal of Histochemistry and Cytochemistry, 68(1), 75–91. https://doi.org/10.1369/0022155419884879
- Sánchez-Salcedo, E. M., Mena, P., García-Viguera, C., Hernández, F., & Martínez, J. J. (2015). (Poly)phenolic compounds and antioxidant activity of white (Morus alba) and black (Morus nigra) mulberry leaves: Their potential for new products rich in phytochemicals. Journal of Functional Foods, 18, 1039–1046. https://doi.org/10.1016/j.jff.2015.03.053
- Smeets, N. J. L., Schreuder, M. F., Dalinghaus, M., Male, C., Lagler, F. B., Walsh, J., Laer, S., & De Wildt, S. N. (2020). Pharmacology of enalapril in children: a review. Drug Discovery Today, 25(11), 1957–1970. https://doi.org/10.1016/j.drudis.2020.08.005
- Stefanucci, A., Zengin, G., Llorent-Martinez, E. J., Dimmito, M. P., Della Valle, A., Pieretti, S., Ak, G., Sinan, K. I., & Mollica, A. (2020). Chemical characterization, antioxidant properties and enzyme inhibition of Rutabaga root’s pulp and peel (Brassica napus L.). Arabian Journal of Chemistry, 13, 7078–7086. https://doi.org/10.1016/j.arabjc.2020.07.013
- Sun, N., Zhai, L., Li, H., Shi, L., Yao, Z., & Zhang, B. (2016). Angiotensin-converting enzyme inhibitor (ACEI)-mediated amelioration in renal fibrosis involves suppression of mast cell degranulation. Kidney & Blood Pressure Research, 41, (1), 108–118. https://doi.org/10.1159/000368549
- Sundström, J., Bodegard, J., Bollmann, A., Vervloet, M. G., Mark, P. B., Karasik, A., Taveira-Gomes, T., Botana, M., Birkeland, K. I., Thuresson, M., Jäger, L., Sood, M. M., VanPottelbergh, G., & Tangri, N. (2022). Prevalence, outcomes, and cost of chronic kidney disease in a contemporary population of 2.4 million patients from 11 countries: The CaReMe CKD study. The Lancet Regional Health. Europe, 20, 100438. https://doi.org/10.1016/J.LANEPE.2022.100438
- Swathi, P., Gana Manjusha, K., Vivekanand, M., Ramkishan, A., & Bhavani, B. (2017). Effect of Morus alba against hyperglycemic and hyperlipidemic activities in streptozotocin induced diabetic nephropathy. Biosciences Biotechnology Research Asia, 14, 1441–1447. https://doi.org/10.13005/bbra/2589
- Tasanarong, A., Kongkham, S., & Khositseth, S. (2017). Corrigendum to “Dual inhibiting senescence and epithelial-to-mesenchymal transition by erythropoietin preserve tubular epithelial cell regeneration and ameliorate renal fibrosis in unilateral ureteral obstruction”. BioMed Research International, 2017, 1357109. https://doi.org/10.1155/2017/1357109
- Travers, S., Prot-Bertoye, C., Daudon, M., Courbebaisse, M., & Baron, S. (2023). How to monitor hydration status and urine dilution in patients with nephrolithiasis. Nutrients, 15(7), 1642. https://doi.org/10.3390/nu15071642
- von Konigslow, T. E., Renaud, D. L., Duffield, T. F., Higginson, V., & Kelton, D. F. (2019). Validation of an automated cell counter to determine leukocyte differential counts in neonatal Holstein calves. Journal of Dairy Science, 102(8), 7445–7452. https://doi.org/10.3168/jds.2019-16370
- Wang, M., Qin, T., Zhang, Y., Zhang, T., Zhuang, Z., Wang, Y., Ding, Y., & Peng, Y. (2022). Toll-like receptor 4 signaling pathway mediates both liver and kidney injuries in mice with hepatorenal syndrome. American Journal of Physiology. Gastrointestinal and Liver Physiology, 323(5), G461–G476. https://doi.org/10.1152/ajpgi.00048.2022
- Washino, S., Hosohata, K., & Miyagawa, T. (2020). Roles played by biomarkers of kidney injury in patients with upper urinary tract obstruction. International Journal of Molecular Sciences, 21(15), 1–18. https://doi.org/10.3390/ijms21155490
- Webster, A. C., Nagler, E. V., Morton, R. L., & Masson, P. (2017). Chronic kidney disease. Lancet, 389(10075), 1238–1252. https://doi.org/10.1016/S0140-6736(16)32064-5
- Wu, M. T., Lam, K. K., Lee, W. C., Hsu, K. T., Wu, C. H., Cheng, B. C., Ng, H. Y., Chi, P. J., Lee, Y. T., & Lee, C. T. (2012). Albuminuria, proteinuria, and urinary albumin to protein ratio in chronic kidney disease. Journal of Clinical Laboratory Analysis, 26(2), 82–92. https://doi.org/10.1002/jcla.21487
- Xu, L., Tan, B., Huang, D., Yuan, M., Li, T., Wu, M., & Ye, C. (2021). Remdesivir inhibits tubulointerstitial fibrosis in obstructed kidneys. Frontiers in Pharmacology, 12, 626510. https://doi.org/10.3389/fphar.2021.626510
- Yang, Z., Yu, X., Cheng, L., Miao, L., Li, H., Han, L., & Jiang, W. (2013). Effects of enalapril on the expression of cardiac angiotensin-converting enzyme and angiotensin-converting enzyme 2 in spontaneously hypertensive rats. Archives of Cardiovascular Diseases, 106(4), 196–201. https://doi.org/10.1016/j.acvd.2013.01.004
- Yin, X., He, X., Wu, L., Yan, D., & Yan, S. (2022). Chlorogenic acid, the main antioxidant in coffee, reduces radiation-induced apoptosis and DNA damage via NF-E2-related factor 2 (Nrf2) activation in hepatocellular carcinoma. Oxidative Medicine and Cellular Longevity, 2022, 4566949. https://doi.org/10.1155/2022/4566949
- Yu, Y., Chen, Y., Shi, X., Ye, C., Wang, J., Huang, J., Zhang, B., & Deng, Z. (2022). Hepatoprotective effects of different mulberry leaf extracts against acute liver injury in rats by alleviating oxidative stress and inflammatory response. Food & Function, 13(16), 8593–8604. https://doi.org/10.1039/d2fo00282e
- Yuan, Y., Gong, X., Zhang, L., Jiang, R., Yang, J., Wang, B., & Wan, J. (2017). Chlorogenic acid ameliorated concanavalin A-induced hepatitis by suppression of Toll-like receptor 4 signaling in mice. International Immunopharmacology, 44, 97–104. https://doi.org/10.1016/j.intimp.2017.01.017
- Yunus, J., Salman, M., Bamba Ratih Lintin, G., Muchtar, M., Cahyani Ratna Sari, D., Arfian, N., & Mansyur Romi, M. (2020). Chlorogenic acid attenuates kidney fibrosis via antifibrotic action of BMP-7 and HGF. Medical Journal of Malaysia, 75, 5–9.
- Zakaria, M., & Mohd, M. A. (2015). Traditional Malay medicinal plants. ITBM.
- Zhang, T., Chen, S., Chen, L., Zhang, L., Meng, F., Sha, S., Ai, C., & Tai, J. (2019). Chlorogenic acid ameliorates lead-induced renal damage in mice. Biological Trace Element Research, 189(1), 109–117. https://doi.org/10.1007/s12011-018-1508-6
- Zhang, Y., Ma, L., Wu, J., & Chen, T. (2015). Hydronephrosis alters cardiac ACE2 and Mas receptor expression in mice. Journal of the Renin-Angiotensin-Aldosterone System, 16(2), 267–274. https://doi.org/10.1177/1470320314568439
- Zhang, Y., Wu, J., Li, B., & Wang, B. (2013). GW24-e3532 effects of hydronephrosis on cardiac ACE/ACE2 gene expression in mice. Heart, 99, A63–A64. https://doi.org/10.1136/heartjnl-2013-304613.176
- Zhang, Z., Wang, G., Ma, J., Liu, H., Zhang, X., & Zhu, G. (2013). Effect of Herba centellae on the expression of HGF and MCP-1. Experimental and Therapeutic Medicine, 6(2), 427–434. https://doi.org/10.3892/etm.2013.1146
- Zhou, Q. Y. J., Liao, X., Kuang, H. M., Li, J. Y., & Zhang, S. H. (2022). LC-MS metabolite profiling and the hypoglycemic activity of Morus alba L. extracts. Molecules, 27(17), 1–14. https://doi.org/10.3390/molecules27175360
- Zhu, Q., Zhu, Y., Liu, Y., Tao, Y., Lin, Y., Lai, S., Liang, Z., Chen, Y., Chen, Y., & Wang, L. (2022). Moderation of gut microbiota and bile acid metabolism by chlorogenic acid improves high-fructose-induced salt-sensitive hypertension in mice. Food & Function, 13(13), 6987–6999. https://doi.org/10.1039/d2fo00038e