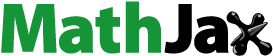
Abstract
The avocado (Persea americana) is a fruit that possesses a high nutritional content and is abundant in phytochemical compounds. However, there is a lack of research conducted on the processing and preservation methods of this fruit. Application of innovative drying such as infrared drying could create a potential product from avocado for the food and cosmetics industry. In this study, two important factors, including temperature of infrared radiation (65, 70, 75 and 80 °C) and formulation (avocado pulp with and without maltodextrin (9 g/100 g pulp)), were investigated to determine the characteristic of drying behavior. The infrared drying was found to removed moisture from avocado pulp effectively. The drying kinetics in infrared drying of avocado pulp could be determined by the Weibull model. Deff values were estimated to range between 5.24 × 10−10 m2/s and 8.41 × 10−10 m2/s for avocado pulp with maltodextrin and between 6.10 × 10−10 m2/s to 9.18 × 10−10 m2/s for avocado pulp without maltodextrin. The activation energy for infrared drying of avocado pulp was found to be 30.77 and 26.74 kJ/mol corresponding to formulation with and without maltodextrin. It takes a short time in drying avocado pulp using infrared radiation showed high potential application in the production of high-quality dried avocado such as avocado powder.
1. Introduction
Drying technology is the most critical unit operation in food processing and preservation. Therefore, this technique has been continuously improved to produce the best quality dried product with more economic benefits (Inyang et al., Citation2018). However, due to the complexation of food in type, composition, and structure, many studies on drying characteristics were performed. Empirical parameters of drying kinetics were essential to apply for developing drying systems and producing commercial products (Inyang et al., Citation2018). The avocado is a fascinating object of investigation within the category of food materials. Avocado fruits are abundant in many nutrients and phytochemicals that are commonly found in sugar-rich fruits and bitter-tasting veggies (Comerford et al., Citation2016). The addition of avocado into dietary could improve the immune system and protect the body from oxidation risks (Ortiz-Avila et al., Citation2015). Recently, avocado fruits are mainly used as fresh with limited shelf life due to the properties of climatic fruit. Still, there are a few studies on the drying of avocado flesh, such as freeze-drying (Souza et al., Citation2015), oven drying (Temu, Citation2013), heat-pump drying (Ceylan et al., Citation2007), superheated-steam (Rafidah et al., Citation2014). However, it was noted that the duration required for the drying process was excessively prolonged, ranging from 5 hours to over 20 hours. During the process of drying, the time of drying is also the time of the reaction (Nguyen et al., Citation2020). Therefore, prolonged drying time could result in more loss in quality of the dried product and more cost of operation. Among innovative drying techniques, infrared drying was considered a suitable method for drying avocado. The advantages of infrared drying, as summarised in the review report by Riadh et al. (Citation2015) and (Riadh et al., Citation2015), include reduced drying time, improved quality of the final dried product, lower capital costs, and increased energy efficiency in the drying process. Some previous studies reported that infrared drying effectively removed moisture from some fruits and vegetables (Doymaz, Citation2012, Citation2015; Nasiroglu & Kocabiyik, Citation2009; Sadin et al., Citation2014; Wen et al., Citation2020; Wu et al., Citation2019). Furthermore, the incorporation of hydrocolloids, such as maltodextrin, into fruit pulp has been demonstrated to enhance the overall quality of dried products, including mango leather (Singh Gujral & Singh Brar, Citation2003), murta (Ugni molinae Turcz) berry leather (Gómez-Pérez et al., Citation2020), tomato (Goula & Adamopoulos, Citation2008), avocado pulp (Nguyen et al., Citation2021), and others. According to a study by Osorio et al. (Citation2011) and (Osorio et al., Citation2011), maltodextrin functioned as a coating material that created a physical barrier, preventing the interaction of phytochemicals with external elements such as oxygen. Therefore, drying fruit pulp with an appropriate maltodextrin concentration will result in a better-quality dried product, characterised by increased total phenolic content and reduced total colour difference when using infrared drying for avocado pulp (Nguyen et al., Citation2021).
Recently, there have no reports on the kinetics of infrared drying of avocado published yet. Hence, the primary objective of this research is to examine the attributes associated with the infrared drying process of pulp avocado. Specifically, the study focuses on two key parameters that significantly influence this process: the infrared temperature and the formulations of the avocado pulp. The drying behavior and kinetic characteristics of pulp avocado in infrared drying were characterized using experimental data. The findings were applied to evaluate the potential implementation of infrared radiation in the processing of avocado pulp.
2. Materials and methods
2.1. Material preparation
For this study, the mature avocado fruit (Persea americana) sourced from a local farm in DakLak, Vietnam, was used as the main resource. The avocado pulp was prepared as shown in .
2.2. Infrared drying apparatus
A laboratory-scale infrared drying system was created at Nguyen Tat Thanh University’s Department of Food Technology. The apparatus comprised a drying chamber measuring 64 x 23 x 27 cm, an infrared radiation lamp module with a power of 1000 W positioned atop the chamber, as well as blowers and a temperature controller. In order to control the drying medium’s temperature, a thermal sensor is employed, which possesses an absolute error of ± 0.5° C. The material was spread onto the polyester film with a 2 mm thickness and positioned within the drying chamber at a distance of 20 cm from the far infrared radiation bulbs.
2.3. Experimental design
The experiment was carried out in three replications using a full factorial design with two variables: formulations and IR temperature. The formulations were 2-levels, including avocado pulp with maltodextrin (9 g/100 g pulp) (APM) and without maltodextrin (AP). The temperature with 4-levels was varied from 65 to 80 °C. To determine the drying kinetics associated with infrared drying of avocado pulp, the moisture content of each sample (100 g) was monitored at 5-minute intervals using the moisture analyzer with infrared heating (MB23, Ohaus, Parsippany, NJ, USA) until it reached approximately 0.04 g/g d.b.
2.4. Modelling mathematically the drying curves of thin layers
The subsequent equation was employed to calculate the moisture ratio (MR) (Pala et al., Citation1996):
(1)
(1)
Where Mt, and M0 are the moisture content at drying time (t, min), and the initial moisture content, respectively, expressed as g/g d.b.
The use of mathematical models enables the determination of the drying kinetics of food products. The drying curves for the nine semi-theoretical and empirical models presented in were fitted in this study.
Table 1. The tested mathematical models for infrared drying of avocado pulp with different formulations.
Table 2. The results of non-linear regression analysis in infrared drying of avocado pulp with different formulations at different drying temperatures.
The determination coefficient (R2) and the root mean square (RMS) was calculated to evaluated the predicted power of the tested models.
(2)
(2)
(3)
(3)
The drying rate of avocado pulp (g/g d.b./min) was calculated utilizing the subsequent equation:
(4)
(4)
2.5. The effective diffusivity
The effective diffusivity (Deff) of fruit pulp was determined as previous study (Nguyen et al., Citation2022). The equation below represents the variation in moisture ratio (MR) during the infrared drying process of avocado pulp due to lengthy drying periods:
(5)
(5)
2.6. Determination of activation energy
The activation energy (Ea) of moisture removal is estimated from an Arrhenius equation, and it was given as:
(6)
(6)
2.7 Statistical analysis
Every experiment was performed in triplicate. The computation of the mean and its standard deviation was performed using WPS Spreadsheets Software. The coefficients of mathematical models were calculated employing the Levenberg-Marquardt algorithm-based non-linear least squares method.
3. Results and discussion
3.1. Drying curves and drying rate
illustrates the variations in moisture content that transpire during the infrared drying process of avocado pulp using distinct formulations. The outcome demonstrated that as the infrared temperature increased, the drying time decreased. In particular, for both formulations with and without maltodextrin, the time required to dry avocado pulp decreased from 55 to 35 minutes, respectively, as the temperature rose from 65 to 80 °C.
Figure 2. The changes of moisture content in the avocado pulp during the infrared drying at different temperatures; (a) formulations with maltodextrin (9 g/ 100 g pulp); (b) formulations without maltodextrin.

According to a number of prior studies on infrared drying of fruits and vegetables, drying times were shortened when the infrared radiation power or temperature was increased (Doymaz, Citation2015; Onwude et al., Citation2019; Wu et al., Citation2019). There have not been published on infrared drying of avocado in the literature yet. While freeze-drying of avocado pulp required 13.3 to 16.7 hours in a previous study (Souza et al., Citation2015) and 7 hours for oven drying of slices (Temu, Citation2013). While infrared drying and oven drying both result in high temperatures for the drying material, the moisture removal efficiency was enhanced when the material is dried in puree form as compared to slices. By disrupting the structure of avocado flesh, the surface area available for evaporation would be expanded and moisture was more convenient to diffuse and evaporate. Reducing drying time would bring significant benefits in retaining the quality of the dried product (see ). In thermal processing techniques such as infrared drying, the duration of the drying process aligned with the reaction time, resulting in an extended drying period that negatively impacted the quality of the final dried items such as Origanum majorana L (Calín-Sánchez et al., Citation2015)., herbs (Orphanides et al., Citation2016), Piper borbonense (Weil et al., Citation2017), bitter melon (Nguyen et al., Citation2020), etc.
Figure 3. The photographs of avocado flesh, avocado slices, avocado pulp and avocado powder via infrared drying.

The drying rate profiles for avocado pulp with various formulations during infrared drying were indicated in . As the infrared temperature increased, so did the rate of drying, according to the findings. The increased absorption of radiation energy at the material’s surface was evidently correlated with the elevated infrared temperature (Hashimoto et al., Citation1993; Sakai & Hanzawa, Citation1994). Additionally, for any given material, the rate of internal and external moisture diffusion increases with the degree of heat gain. The increased temperature of the infrared would consequently reduce drying time by a substantial amount. Dehydration was observed primarily during the period of falling rate; the period of constant rate was undetectable. In practical food dehydration, it is reported that all biological products are dried during the falling rate period (Madamba et al., Citation1996). Similar observations regarding infrared drying have been documented in the literature (Doymaz, Citation2012; Citation2015; Wen et al., Citation2020). Besides, in this study, the drying process occurred very fast due to the state of the drying material. When the material was ground, more free water would be released from the vacuole and the xylem, causing an increased ratio of free water to bound water. A rapid increase in heat would be occured when the pulp was layered thinly. As a result, the drying process exclusively transpired during the falling-rate period, as the infrared drying of avocado pulp involved a rapid evaporation process.
Figure 4. The drying rate curves in the infrared drying of the avocado pulp at different temperatures; (a) formulations with maltodextrin (9%, w/w); (b) formulations without maltodextrin.

Additionally, the initial drying rate of the APs was considerably greater than that of the AMPs at every drying temperature. It could be because maltodextrin binds water, decreasing the evaporation rate. Furthermore, maltodextrin reduced the percentage of the water content of the pulp. Therefore, the quantity of water removed per minute in APs was significantly greater than in APMs for samples of the same initial weight. Even though maltodextrin has a negative impact on the rate of evaporation, its capacity to protect bioactive compounds from degradation reactions (Osorio et al., Citation2011) could help retain high-quality dried products.
3.2. The mathematical model for infrared drying of avocado pulp
In order to determine which model best described the behaviour of avocado pulp dried with infrared radiation, variations in the moisture ratio throughout the drying process were utilized. The thin-layer model has been determined to be the best one due to its smallest RMS and greatest R2 value (closer to 1.00000) ().
Among nine investigating models, the result showed that the value R2 had very high (most of larger than 0.97). The R2 values decrease as follows: Weibull, Midiili, Page, Modified Page (II), and Modified Page (IV) models surpass Page. The model proposed by Wang and Singh is superior to the logarithmic model, Henderson and Pabis, and Newton. It was observed that the model lacked an empirical term or a dimensionless empirical constant (n), which would have reduced its predictive potential in comparison to the Henderson and Pabis models and the Newton model. Some previous studies could confirm this conclusion (Doymaz, Citation2012, Citation2015; Sadin et al., Citation2014; Wen et al., Citation2020). Wen et al. (Citation2020) and (Wen et al., Citation2020) determined that the Page model seemed to fit the data better than the Newton, two-term, Henderson, and Pabis models, with an R2 between 0.996 and 0.997. According to a study by Sadin et al. (Citation2014), the Midilli model was deemed appropriate for the infrared drying of tomato slices in comparison with Page, Lewis, Logarithmic. In the works of Doymaz, Logarithmic (R2 ranging from 0.9985 to 0.9996) and Midilli model (R2 varied from 0.9989 to 0.9999) was chosen to represent the drying kinetics of sweet potato (Doymaz, Citation2012) and carrot slices (Doymaz, Citation2015), respectively.
The experimental data in this study indicated that the Weibull and Midilli models provided the most accurate fit with R2 value of more than 0.999 and RMS less than 3% for all runs. And, there are very small differences in values of R2 and RMS between Weibull and Midilli models. Even so, the Weibull model had better values in R2 and RMS than Midilli for most of the runs, except for the sample without maltodextrin dried at 65 °C. Several prior investigations have demonstrated that the Weibull model could be employed to describe the dehydration characteristics of food substances (Corzo et al., Citation2010; Ju et al., Citation2018; Nguyen et al., Citation2019; Tzempelikos et al., Citation2015; Uribe et al., Citation2011). As a result, the Weibull model best described the infrared drying characteristics of avocado pulp in the present study.
3.3. The effective diffusivity and activation energy
Numerous prior investigations have assessed and examined the effective diffusivity in the context of drying kinetics. The effective diffusivity was estimated using experimental data in this investigation. The linear correlation between ln(MR) and drying time (t, min) under various drying conditions was illustrated in . Employing the outcomes of linear regression analysis, the effective diffusivity was computed. The values of effective diffusivity obtained for APs and APMs at different infrared temperatures were showed in with R2 values, respectively. also presented values of activation energy calculating from Eq. (9).
Table 3. The effective diffusivity and activation energy in infrared drying of avocado pulp with different formulations.
The result revealed that when the infrared temperature changed from 65 to 80 °C, Deff would change from 5.24 × 10−10 m2/s to 8.41 × 10−10 m2/s for APMs, and from 6.10 × 10−10 m2/s to 9.18 × 10−10 for APs. It was reported that Deff values ranged from 10−11 to 10−8 m2/s for thin-layer food dehydration (Onwude et al., Citation2016). With a coefficient of determination of 0.9787 and a root mean square of 4.29%, the linear regression method revealed a correlation of linearity between the drying constant (1/s) and the effective moisture diffusivity. This equation was followed as:
(10)
(10)
Research was reported on the infrared dehydration of fruits and vegetables including tomato, carrot, red pepper, and star anises. The Deff values for infrared drying of tomato (Sadin et al., Citation2014), carrot (Wu et al., Citation2014), red pepper (Nasiroglu & Kocabiyik, Citation2009), and star anises (Wen et al., Citation2020) were found as 1.094 × 10−9 to 4.468 × 10−9 at from 60 to 80 °C, 2.38 × 10−9 to 10.30 × 10−9 at from 60 to 80 °C, 1.75 × 10−10 to 8.97 × 10−10 at from 50 to 80 °C, and 5.022 × 10−10 to 9.557 × 10−10 at from 60 to 80 °C, respectively. In a similar range of investigated infrared temperature, the results showed that the Deff values of avocado pulp were like to star anises and more a little than red pepper, but significantly less than tomato and carrot in infrared drying. Although avocado was dried in a pulp state with more released free water, the high content of lipid in the pulp could cause resistance in moisture removal. The activation energy for moisture evaporation of avocado was 30.77 and 26.74 kJ/mol in infrared drying of APMs and APs, respectively. On the drying of fruits and vegetables, Ea values were more prevalent, but there are few publications on the drying of avocados. 90% of published Ea values for the dehydration of fruits and vegetables varied between 14.42 and 43.26 kJ/mol (Onwude et al., Citation2016). The findings revealed that the energy consumption of APMs for moisture removal was considerably higher than that of APs. Thus, the water-binding capacity of maltodextrin significantly impacted the evaporation and diffusion of water within the cellulose (Adhikari et al., Citation2004). Therefore, in addition to supporting moisture diffusion from the material’s center to its surface and evaporation at its surface, radiation energy was utilized to disrupt the bond between water and maltodextrin, thereby releasing free water. Some reports on infrared drying of fruits and vegetables were found that Ea values were 14.07 – 30.31 kJ/mol for tomato slices (Sadin et al., Citation2014), 35.74 – 39.06 kJ/mol for carrot slices (Wu et al., Citation2014), and 50.90 kJ/mol for red pepper (Nasiroglu & Kocabiyik, Citation2009). Therefore, the avocado needed less energy for removing moisture than some slices of fruits and vegetables. As above mention, more free water was released after avocado flesh was ground leading to reducing energy for evaporation of moisture during drying.
4. Conclusion
This study determined that the drying process of avocado pulp using infrared technology occurred exclusively during the falling rate period. According to the findings, the Weibull model has shown the highest degree of correlation in characterizing the behavior of avocado pulp that undergoes infrared radiation drying. The enhanced absorption of radiation energy could raise the infrared temperature, hence increasing the rate of drying. Using maltodextrin in avocado pulp is associated with a decreased drying rate and effective diffusivity while exhibiting higher activation energy than pulp without maltodextrin. The infrared drying had effectiveness in removing moisture from avocado pulp with a short time for completing the drying process. The reduced operation time could result in higher product quality. Consequently, in order to identify the most suitable drying conditions that yield dried products of superior quality, subsequent investigations needs to focus on examining the impacts of infrared drying on the physicochemical properties.
Authors’ contributions
The investigation, Thi-Van-Linh Nguyen and Phuoc-Bao-Duy Nguyen; Methodology, Thi-Van-Linh Nguyen; Data Analysis, Phuoc-Bao-Duy Nguyen and Thi Tuong Vi Tran; Writing – original draft, Thi-Van-Linh Nguyen; Review & editing, Thi-Van-Linh Nguyen and Thi Tuong Vi Tran.
Acknowledgments
The authors would like to thank Nguyen Tat Thanh University for permission and for providing facilities during the research period, and thank DAKADO GROUP for supplying avocado fruits during the research period.
Disclosure statement
The authors declare no conflict of interest.
Data availability statement
The data used to support the findings of this study are included within the article and are also available from the corresponding author upon request.
Additional information
Funding
Notes on contributors
Thi-Van-Linh Nguyen
Dr. Nguyen Thi Van Linh is a researcher, lecturer and Head of Food Technology Department of Institute of Applied Technology and Sustainable Development – Nguyen Tat Thanh University (NTTU). Her study focuses on the application of novel drying technology to dehydrate materials containing high bioactive compounds. Her research interests include the application of novel technology to retain the highest content of compounds containing high antioxidant capacity; kinetics of quality change during processing or storage; drying fruit, vegetables, mushroom and herbs; development of new products from dried food materials.
Phuoc-Bao-Duy Nguyen
Dr. Tran Thi Tuong Vi is currently an Acting Head Department of Chemical Engineering at Nguyen Tat Thanh University. Her main research interests are in developing nanomaterials and conversion of biomass to valuable chemicals and biofuel via chemical reactions using heterogeneous catalysts. Currently, her research focuses mainly on: Heterogeneous catalysts for chemical production from biomass-based such as carbohydrate, glucose and xylose, synthesis of nanocomposite materials with electromagnetic shielding properties, and adsorptive removal of antibiotics and/or dyes from wastewater.
Thi Tuong Vi Tran
M. Eng Phuoc-Bao-Duy Nguyen is a lecturer in Department Of Basic Engineering, Faculty of Electrical and Electronics Engineering, Ho Chi Minh City University of Technology (HCMUT), Vietnam National University Ho Chi Minh City. His majors are automation and applied mathematics in engineering. One of his research fields are modeling kinetic processes and designing of equipment in food engineering.
References
- Adhikari, B., Howes, T., Bhandari, B. R., & Troung, V. (2004). Effect of addition of maltodextrin on drying kinetics and stickiness of sugar and acid-rich foods during convective drying: experiments and modelling. Journal of Food Engineering, 62(1), 1–10. https://doi.org/10.1016/S0260-8774(03)00171-7
- Calín-Sánchez, Á., Figiel, A., Lech, K., Szumny, A., Martínez-Tomé, J., & Carbonell-Barrachina, Á. A. (2015). Dying methods affect the aroma of Origanum majorana L. analyzed by GC–MS and descriptive sensory analysis. Industrial Crops and Products, 74, 218–227. https://doi.org/10.1016/j.indcrop.2015.04.067
- Ceylan, İ., Aktaş, M., & Doğan, H. (2007). Mathematical modeling of drying characteristics of tropical fruits. Applied Thermal Engineering, 27(11-12), 1931–1936. https://doi.org/10.1016/j.applthermaleng.2006.12.020
- Comerford, K. B., Ayoob, K. T., Murray, R. D., & Atkinson, S. A. (2016). The role of avocados in complementary and transitional feeding. Nutrients, 8(5), 316. https://doi.org/10.3390/nu8050316
- Corzo, O., Bracho, N., & Alvarez, C. (2010). Weibull model for thin-layer drying of mango slices at different maturity stages. Journal of Food Processing and Preservation, 34(6), 993–1008. https://doi.org/10.1111/j.1745-4549.2009.00433.x
- Doymaz, İ. (2012). Infrared drying of sweet potato (Ipomoea batatas L.) slices. Journal of Food Science and Technology, 49(6), 760–766. https://doi.org/10.1007/s13197-010-0217-8
- Doymaz, İ. (2015). Infrared drying kinetics and quality characteristics of carrot slices. Journal of Food Processing and Preservation, 39(6), 2738–2745. https://doi.org/10.1111/jfpp.12524
- Gómez-Pérez, L. S., Navarrete, C., Moraga, N., Rodríguez, A., & Vega-Gálvez, A. (2020). Evaluation of different hydrocolloids and drying temperatures in the drying kinetics, modeling, color, and texture profile of murta (Ugni molinae Turcz) berry leather. Journal of Food Process Engineering, 43(2), e13316.
- Goula, A. M., & Adamopoulos, K. G. May (2008). Effect of maltodextrin addition during spray drying of tomato pulp in dehumidified air: II. Powder properties. Drying Technology, 26(6), 726–737. https://doi.org/10.1080/07373930802046377
- Hashimoto, A., Igarashi, H., & Shimizu, M. (1993). Irradiation power effect on IR pasteurization below lethal temperature of bacteria. Journal of Chemical Engineering of Japan, 26(3), 331333.
- Inyang, U. E., Oboh, I. O., & Etuk, B. R. (2018). Kinetic models for drying techniques—food materials. Advances in Chemical Engineering and Science, 08(02), 27–48. https://doi.org/10.4236/aces.2018.82003
- Ju, H.-Y., Zhao, S.-H., Mujumdar, A. S., Fang, X.-M., Gao, Z.-J., Zheng, Z.-A., & Xiao, H.-W. (2018). Energy efficient improvements in hot air drying by controlling relative humidity based on Weibull and Bi-Di models. Food and Bioproducts Processing, 111, 20–29. https://doi.org/10.1016/j.fbp.2018.06.002
- Madamba, P. S., Driscoll, R. H., & Buckle, K. A. (1996). The thin-layer drying characteristics of garlic slices. Journal of Food Engineering, 29(1), 75–97. https://doi.org/10.1016/0260-8774(95)00062-3
- Nasiroglu, S., & Kocabiyik, H. (2009). Thin-layer infrared radiation drying of red pepper slices. Journal of Food Process Engineering, 32(1), 1–16. https://doi.org/10.1111/j.1745-4530.2007.00195.x
- Nguyen, T. V., Nguyen, M. D., Nguyen, D. C., Bach, L. G., & Lam, T. D. (2019). Model for thin layer drying of lemongrass (Cymbopogon citratus) by hot air. Processes, 7(1), 21. https://doi.org/10.3390/pr7010021
- Nguyen, T., Nguyen, Q., Nguyen, P., Tran, B., & Huynh, P. T. (2020). Effects of drying conditions in low-temperature microwave-assisted drying on bioactive compounds and antioxidant activity of dehydrated bitter melon (Momordica charantia L.). Food Science & Nutrition, 8(7), 3826–3834. https://doi.org/10.1002/fsn3.1676
- Nguyen, T.-V.-L., Ngo, P.-T., Huynh, T.-T.-N., Vo, P.-N.-T., Hoang, T.-N.-A., & Nguyen, P.-B.-D. (2022). Refractance window drying of mango pulp (Mangifera indica): Impact of hydrocolloids on drying characteristics and color parameters [Paper presentation]. AIP Conference Proceedings, in, AIP Publishing LLC, p. 060022. https://doi.org/10.1063/5.0100826
- Nguyen, T.-V.-L., Nguyen, Q.-D., Nguyen, T.-T.-D., & Nguyen, P.-B.-D. (2021). Effects of Infrared Drying Conditions and Maltodextrin Addition on Some Physicochemical Characteristics of Avocado (Persea americana) Pulp Powder. Applied Sciences, 11(24), 11803. https://doi.org/10.3390/app112411803
- Onwude, D. I., Hashim, N., Abdan, K., Janius, R., & Chen, G. (2019). The effectiveness of combined infrared and hot-air drying strategies for sweet potato. Journal of Food Engineering, 241, 75–87. https://doi.org/10.1016/j.jfoodeng.2018.08.008
- Onwude, D. I., Hashim, N., Janius, R. B., Nawi, N. M., & Abdan, K. May (2016). Modeling the thin-layer drying of fruits and vegetables: A review. Comprehensive Reviews in Food Science and Food Safety, 15(3), 599–618. https://doi.org/10.1111/1541-4337.12196
- Orphanides, A., Goulas, V., & Gekas, V. (2016). Drying technologies: Vehicle to high-quality herbs. Food Engineering Reviews, 8(2), 164–180. https://doi.org/10.1007/s12393-015-9128-9
- Ortiz-Avila, O., Esquivel-Martínez, M., Olmos-Orizaba, B. E., Saavedra-Molina, A., Rodriguez-Orozco, A. R., & Cortés-Rojo, C. (2015). Avocado oil improves mitochondrial function and decreases oxidative stress in brain of diabetic rats. Journal of Diabetes Research, 2015, 1–9. https://doi.org/10.1155/2015/485759
- Osorio, C., Forero, D. P., & Carriazo, J. G. (2011). Characterisation and performance assessment of guava (Psidium guajava L.) microencapsulates obtained by spray-drying. Food Research International, 44(5), 1174–1181. https://doi.org/10.1016/j.foodres.2010.09.007
- Pala, M., Mahmutoǧlu, T., & Saygi, B. (1996). Effects of pretreatments on the quality of open-air and solar dried apricots. Food / Nahrung, 40(3), 137–141. https://doi.org/10.1002/food.19960400308
- Rafidah, H., Ando, Y., Amin, I., Shirai, Y., & Ali, H. M. (2014). Enhanced polyphenol content and antioxidant capacity in the edible portion of avocado dried with superheated-steam. International Journal of Advanced Research, 8, 241–248.
- Riadh, M. H., Ahmad, S. A. B., Marhaban, M. H., & Soh, A. C. (2015). Infrared heating in food drying: An overview. Drying Technology, 33(3), 322–335. https://doi.org/10.1080/07373937.2014.951124
- Sadin, R., Chegini, G.-R., & Sadin, H. (2014). The effect of temperature and slice thickness on drying kinetics tomato in the infrared dryer. Heat and Mass Transfer, 50(4), 501–507. https://doi.org/10.1007/s00231-013-1255-3
- Sakai, N., & Hanzawa, T. (1994). Applications and advances in far-infrared heating in Japan. Trends in Food Science and Technology, 5(11), 357–362. https://doi.org/10.1016/0924-2244(94)90213-5
- Singh Gujral, H., & Singh Brar, S. (2003). Effect of hydrocolloids on the dehydration kinetics, color, and texture of mango leather. International Journal of Food Properties, 6(2), 269–279. https://doi.org/10.1081/JFP-120017846
- Souza, D. S., Marques, L. G., Gomes, E. d B., & Narain, N. (2015). Lyophilization of avocado (Persea americana Mill.): effect of freezing and lyophilization pressure on antioxidant activity, texture, and browning of pulp. Drying Technology, 33(2), 194–204. https://doi.org/10.1080/07373937.2014.943766
- Temu, A. T. (2013). Effect of temperature and slice size on avocado pulp drying rate and oil yield. Tanzania Journal of Engineering and Technology, 34(2), 116–124. https://doi.org/10.52339/tjet.v34i2.464
- Tzempelikos, D. A., Vouros, A. P., Bardakas, A. V., Filios, A. E., & Margaris, D. P. (2015). Experimental study on convective drying of quince slices and evaluation of thin-layer drying models. Engineering in Agriculture, Environment and Food, 8(3), 169–177. https://doi.org/10.1016/j.eaef.2014.12.002
- Uribe, E., Vega-Gálvez, A., Di Scala, K., Oyanadel, R., Saavedra Torrico, J., & Miranda, M. (2011). Characteristics of convective drying of pepino fruit (Solanum muricatum Ait.): Application of weibull distribution. Food and Bioprocess Technology, 4(8), 1349–1356. https://doi.org/10.1007/s11947-009-0230-y
- Weil, M., Shum Cheong Sing, A., Méot, J. M., Boulanger, R., & Bohuon, P. (2017). Impact of blanching, sweating and drying operations on pungency, aroma and color of Piper borbonense. Food Chemistry, 219, 274–281. https://doi.org/10.1016/j.foodchem.2016.09.144
- Wen, Y.-X., Chen, L.-Y., Li, B.-S., Ruan, Z., & Pan, Q. (2020). Effect of infrared radiation-hot air (IR-HA) drying on kinetics and quality changes of star anise (Illicium verum). Drying Technology, 39(1), 90–103. https://doi.org/10.1080/07373937.2019.1696816
- Wu, B., Ma, H., Qu, W., Wang, B., Zhang, X., Wang, P., Wang, J., Atungulu, G. G., & Pan, Z. (2014). Catalytic infrared and hot air dehydration of carrot slices. Journal of Food Process Engineering, 37(2), 111–121. https://doi.org/10.1111/jfpe.12066
- Wu, X., Zhang, M., & Li, Z. (2019). Influence of infrared drying on the drying kinetics, bioactive compounds and flavor of Cordyceps militaris. LWT, 111, 790–798. https://doi.org/10.1016/j.lwt.2019.05.108