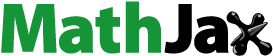
Abstract
Grass pea (Lathyrus sativus L.) is a popular legume in Ethiopia that serves as a protein source to replace chickpeas and field peas. It is a great way to address nutrition and food security in the growing population. However, one of the major issues is that it produces Oxalyl diaminopropionic acid (ODAP), which causes neurological disorders in humans and animals. The study aimed to measure the ODAP, heritability, and genetic advance of quantitative traits in northwest Ethiopian grass pea accessions. The field experiment was carried out during the 2019 rainy season, utilizing a 5x5 lattice design to assess the ODAP and non-yield quantitative traits of grass pea accessions at Ebonyi State University, Nigeria. This study showed a highly significant difference between accessions for all traits except petiole length. This finding discovered high heritability, genetic advance, genotypic and phenotypic coefficients of variation for ODAP, branch bearing length, number of secondary branches, days to flowering, plant height, and primary branch length. The average ODAP value was 0.44 percent, with values ranging from 0.17 to 0.90 percent. Only two of the 25 accessions 26627 and 238945 displayed safe ODAP values of <0.2. These findings may be important for the development of low-ODAP grass-pea cultivars.
Introduction
To feed the world’s 9.8 billion people by 2050, it is predicted that food production will increase globally by 70–100% (Tesema & Mekonen, Citation2023; Van Dijk et al., Citation2021). As a result of population growth contributing to climate change and more land is required. Thus, to drastically lower hunger, future food production needs to focus on developing new crops that can withstand a variety of extreme climatic conditions (Edwards et al., Citation2023; Van Dijk et al., Citation2021). Hence, the most important grain legume that is nutritionally secure and may be used for a variety of purposes is the grass pea (Lathyrus sativus L.). During times of famine for the impoverished in developing nations, it generates a sizable yield when other crops in the drought-prone area fail to produce (Mekonen et al., Citation2022; Jeberson et al., Citation2018). It is elasticity to both abiotic and biotic stress tempers, a potential crop for assuring nutrition and food security in an evolving climate (Edwards et al., Citation2023). Compared to cereals, it needs less labor and input and grows well in residual moisture (Banerjee et al., Citation2022; Kassie et al., Citation2009; Kumar et al., Citation2021; Sen Gupta et al., Citation2021). In Ethiopia, it is used as a rotation crop to increase soil fertility and reduce fertilizer costs. Thus, grass peas have the potential to aid in global food security (Mekonen et al., Citation2022; Teferra, Citation2021).
Overutilization of grass pea seeds greater than 40% of the daily diet for more than three months, has been reported to cause a permanent neurological disorder known as ‘lathyrism’ or ‘neurolathyrism (Edwards et al., Citation2023; Mekonen et al., Citation2022). The disease is expressed as crippling of the leg muscles due to a non-protein amino acid, oxalyl diamino propanoic acid (ODAP) (Arslan et al., Citation2020; Banerjee et al., Citation2022; Kumar et al., Citation2011; Citation2021; Sen Gupta et al., Citation2021). Because of the multifaceted aetiology of neurolathyrism, no consistent safe level of ODAP, as well as consumption percentage, has been determined so far (Edwards et al., Citation2023; Wiraguna et al., Citation2020). ODAP accumulates in all parts of the plant at all stages of development (Arslan et al., Citation2020). However, the highest accumulation has been reported in young seedlings and seeds (Girma & Korbu, Citation2012). The ODAP content in the seeds ranges from 0.02 to 2.59% (Hillocks & Maruthi, Citation2012). This is a discouraging factor in the production and utilization of the crop. In Ethiopia, only one variety of grass pea with reduced ODAP has been developed through natural germplasm and conventional breeding (Fikre, Citation2008; Tadesse & Bekele, Citation2003a,Citationb). However, to eliminate the menace of neurolathyrism and abundantly exploit the prospective of grass peas, improve varieties with very low or zero ODAP irrespective of whether extremes persist as a crucial trait (Edwards et al., Citation2023).
Understanding and analyzing genetic variability parameters, particularly heritability and genetic advance, are essential steps in a breeding program. That underpins the expression of the characters and genetic gain for identifying the intensity of selection, subsequently supporting the selection potential of breeding lines (Ahmadi et al., Citation2015; Mahapatra et al., Citation2020). However, very limited research has been conducted on grass peas because the crop is marginalized and underutilized as a result of the ODAP content in its seed and foliage. Nowadays, scientists are looking for climate-resilient or climate-smart food crops to feed the dense population of the globe in the face of adverse climatic conditions. In this context, understanding variations and categorizing accessions using morpho-agronomic traits and ODAP content would be of remarkable breeding value to utilize genetic resources and distinguish low ODAP accessions (Arslan et al., Citation2020; Mahapatra et al., Citation2020). From this point of view, this study aims to discern the levels of ODAP as well as the heritability, and genetic advance of quantitative characters of grass pea accessions originating from northwest Ethiopia that were not analyzed before and could be helpful for future breeding programs and germplasm conservation.
Materials and methods
Plant materials and experimental design
This study selected 25 grass pea accessions from the main growing areas of northwestern Ethiopian districts and zones with full passport data obtained from the Ethiopian Biodiversity Institute (EBI) (). The field experiment was conducted at Ebonyi State University, College of Agricultural Science Campus (EBSU, CAS), Department of Crop Production and Landscape Management teaching and experimental field, Abakaliki, Nigeria. It is situated in the tropical rainforest zone at latitude 60 4’ N and longitude 80 65’ E at 55.6 meters above sea level. Monthly rainfall varies from 151.6 mm to 220.1 mm, has an average of 194 mm, and has an average monthly relative humidity of 86%. The minimum, maximum, and average temperatures are 230C,310C, and 27 °C degrees Celsius, respectively. The field experiment employed a 5 × 5 simple lattice design under rainfed conditions in 2019. The plots are divided into three rows of two meters each, 30 cm between rows, and 20 cm between plants (Abate et al., Citation2018; Yates, Citation1936). Hand seeding was performed on a flat bed.
Table 1. The grass pea accessions used in the study with zonal, district, latitudinal, longitudinal, and altitudinal records.
Grass pea seed ODAP analysis
ODAP content was extracted from 25 accessions grown in a field experiment with two replicates and analyzed using a UV-spectrophotometer in triplicate on 50 samples. Rao et al. (Citation1964) ortho-phthalaldehyde (OPT) method, modified by Briggs et al. (Citation1983), was used to determine the concentration of ODAP in dry seeds. The procedure involved dissolving 0.25 g of ground seeds in 5 mL of 60% ethanol, shaking for 45 min, centrifuging at 4000 rpm for 15 min, and hydrolyzing with 4 mL of 3 N aqueous potassium hydroxide solution (3 N KOH) by boiling at 95 °C in a water bath for 30 min. Unhydrolyzed samples were also prepared along with blank controls.
A mixture of 0.3 mL of the unhydrolyzed sample extract, 0.7 mL, dH2O, and OPT reagent (2 mL of OPT reagent) was prepared (OPT blank).
A 0.3 mL sample extract that had not been hydrolyzed was mixed with 0.70 mL dH2O and potassium borate buffer (2 mL).
A test sample was made up of 0.3 mL of hydrolyzed sample extract, 0.7 mL, of dH2O, and the OPT reagent (2 mL of OPT reagent).
The sample extract was hydrolyzed in 2 ml of 0.5 M potassium borate buffer, and 0.7 ml of dH2O was incubated for two hours at 40 °C. The solution then turned brown-yellow, and its absorbance was measured at λmax = 425 nm using a spectrophotometer. A standard curve was produced and used to calculate the ODAP concentration using the equation proposed by Briggs et al. (Citation1983).
The most important idea is that the Y-intercept and slope of an experiment are the average of the triplicate absorbance readings from each assay to extrapolate the ODAP regression line equation and the standard ODAP absorbance reading (Table S1 and Figure S1). where a and b represent the unhydrolyzed sample extracts with OPT and buffer reagent, respectively, and c and d represent the hydrolyzed sample extracts with OPT and buffer, respectively.
Preparation of orthophtalaldehyde (OPT)
The ortho-paraldehyde (OPT) reagent was made by combining 500 mg of ortho-paraldehyde (OPT) with 5 mL of 95% ethanol, 1 mL of mercaptoethanol, and 494 mL of 0.5 M potassium borate (BK3O3) buffer [power of hydrogen (pH)] 9.99 (Briggs et al., Citation1983).
Preparation of standard β-ODAP solution
A standard calibration curve was produced by dissolving 50 mg of N-oxalyl-L-di-amino propionate (β-ODAP) in 4 mL of 3 N HCl solution and 500 mL of dH2O in 10 different sets of concentrations in triplicate. Distilled water was used to avoid background absorbance noise in the standard solutions (Table S1).
Data collection for quantitative traits
Ten plants from each accession were used to collect data on 13 quantitative traits on a plant-by-plant basis using IPGRI’s (Citation2000) Lathyrus sativus descriptors. The quantitative traits included plant height (PH), primary branch length (PBL), length of branch bearing (LBB), number of nodes in the primary branch (NNPB), primary branch internode length PBINL (cm), number of secondary branches (NSB), number of branches per plant (NBPP), number of primary branches (NPB), petiole length (PtL), leaflet length (LL), leaf width (LW), days to 50% emergence (DE), and days to 50% flowering. All data were collected at a 50% inflorescence date for the grass pea genetic variability study.
Statistical analysis
For 13 quantitative traits and one quality trait, a simple lattice design hit RCBD, and ANOVA was performed using the SAS software (Sas, Citation2002). Genotypic and phenotypic variations in all traits were calculated using the Omidi et al. (Citation2009) algorithm. Broad-sense heritability and expected genetic advances were calculated using the methods described by Allard (Citation1960) and Johnson et al. (Citation1955).
Results and discussions
Analysis of variance (ANOVA) for ODAP and quantitative traits
ANOVA revealed highly significant differences (P < 0.001) among accessions in ODAP content and quantitative traits, including days to flowering (DF), number of secondary branches (NSB), number of branches per plant (NBPP), plant height (PH), primary branch length (PBL), length of branch bearing (LBB), number of nodes on the primary branch (NNPB), primary branch internode length (PBINL), and leaf width (LW). There was a significant variation (P < 0.01) in the number of primary branches (NPB) and leaflet length (LL) across the examined accessions. These indicate the existence of genetic diversity among grasspea accessions, which could be useful for future breeding programs. Whereas days to fifty percent emergency (DE) showed a significant difference at (p < 0.05). While petiole length (PtL) showed no significant difference among grass pea accessions (). The presence of high genetic diversity for plant height (PH), number of primary branches (NPB), days to flowering (DF), number of secondary branches (NSB), primary branch length (PBL), and ODAP content have been reported by several authors (Abate et al., Citation2018; Das, Citation2015; Kumari, Citation2016).
Table 2. Analysis of variance (mean square) of 13 quantitative and one quality trait in 25 grass pea accessions grown at EBSU, CAS campus (2019).
Variability in ODAP content of the seed
The ODAP content ranged from 0.17 to 0.90 percent among 25 grass pea accessions, with an average of 0.44 percent (). Scholars rated the ODAP’s toxicity level as <0.2 low safe range for consumption, 0.2-0.30 medium level, 0.3-0.5 high toxic, and >0.5 extremely highly toxic (Chowdhury et al., Citation2005; Dahiya, Citation1976; Hanbury et al., Citation2000). Accordingly, the majority of the evaluated accessions (80%) had a recorded high level of ODAP (>0.30%). Of this, 48% of the accessions had high ODAP contents ranging from 0.3 to 0.5%, and 32% of the accessions had a very high β-ODAP content greater than 0.5%. Whereas, 12% of the accessions Acc 238928 (0.25%), Acc 226017 (0.26%), and Acc 238947 (0.29%) were identified as having a medium level of ODAP content ranging from 0.2 to 0.30%. Furthermore, 8% of the accessions having an ODAP level in the low range and safe for human consumption (< 0.2%) were identified in Acc 26627 (0.185%) and Acc 238945 (0.17%). They were collected from the East Gojjam zone at Baso Liben district and the West Gojjam zone at Achefer district, respectively. This suggests low and medium-level accessions could be vital for screening and creating a low ODAP breeding population for the future line of breeding activities to improve these crop interests.
Figure 1. The mean seed ODAP contents of 25 grass pea accessions were evaluated at EBSU, Abakaliki, Nigeria, in 2019.
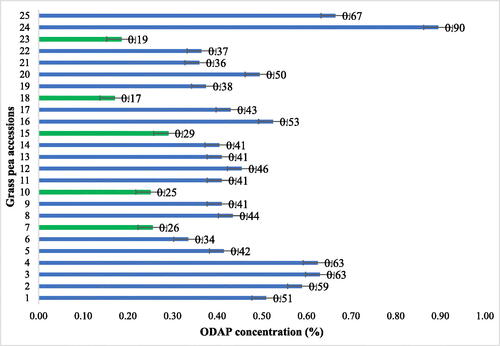
The mean of the present study fell within the range of several authors (Tekle-Haimanot et al., Citation1993) ranging from 0.367 to 1.042%, (Emmrich, Citation2017) ranging from 0.04 to 0.607%; (Hanbury et al., Citation2000), reviewed from different authors and countries such as Syria (0.07 to 0.75), Bangladesh (0.45 to 1.4), India (0.28 to 1.5), and China (0.08 to 0.99). Moreover, Tadesse & Bekele, Citation2003b, β-ODAP content varied from 0.146 to 0.916%; and they also found the lowest safe range β-ODAP content as a single plant basis was in Acc 46027 (0.149) from the Shewa region, and they also found a low safe range value from three entries collected from Wollo, Gondar, and Tigray from a single plant.
Estimation of phenotypic and genotypic coefficient of variation
The genotypic and phenotypic coefficients of variation (GCV and PCV) of traits ranged from 4.88-41.14% (). According to Deshmukh et al. (Citation1986), the classification of GCV and PCV is rated as low (< 10), moderate (10 to 20), and high (>20). In the present study, the highest GCV and PCV were for NSB (40.93%; 41.14%), NBPP (34.28%; 34.44%), ODAP content of seed (32.82%; 32.94%), and LBB (23.47%; 24.56%). A moderate level of PCV and GCV for DF, NPB, PH, and PBL. Low GCV and PCV values were recorded for PtL, LL, PBINL, LW, and NNPB. Abate et al. (Citation2018), Kumari (Citation2016), and Das (Citation2015) reported similar results for the traits NSB, NBPP, and ODAP content. The results for NPB and DF varied in different reports, which might be due to the diverse set of genotypes studied under various environmental conditions (Abate et al., Citation2018). Characters with high and moderate values of PCV and correspondingly higher values of GCV imply more genetic contribution and the effectiveness of simple selection practices for further improvement. However, high values of genotypic and phenotypic coefficients of variation alone do not imply the effectiveness of selection when the amount of genetic gain expected from selection and broad-sense heritability values are low (Das, Citation2015).
Table 3. Estimates of genetic parameters for 13 traits and ODAP among 25 accessions of grass pea evaluated at EBSU, CAS campus (2019).
Heritability in the broad sense (h2%)
The results showed that all tested traits had a high heritability, ranging from 66.6 for seed emergence to 99.29% for ODAP content (). This is in the range of Johnson et al. (Citation1955) categorization, which is low (30%), moderate (30 to 60%), or high (>60%). Accordingly, high heritability was found in all traits: ODAP content (99.29%), NBPP (99.09%), NSB (98.96%), DF (97.68%), LBB (91.37%), LW (91.05%), PH (89.54%), PBL (89.42%), PBINL (88.46%), NNPB (87.21%), LL (76.0%), NPB (72.80%), and DE (66.0%). Related results have been noted by Abate et al. (Citation2018), Das (Citation2015) and Tadesse and Bekele (Citation2003a) who found high heritability for DF, PH, PBL, PBINL, NNPB, and ODAP content.
Genetic advance as a percentage of means (GAM)
Genetic advance as a percent mean (GAM) varied from LL 8.74% to NSB 83.71% (). Johnson et al. (Citation1955) categorized values as low (10), moderate (10-20), or high (>20). Based on that, this finding showed high GAM for the traits NSB (83.71%), NBPP (70.16%), ODAP (67.23%), LBB (46.13%), NPB (30.32), DF (26.49%), PBL (21.55%), and PH (21.15%). This finding suggests that additive gene action is a significant contributor to these features. A moderate GAM was found in NNPB (18.89%), LW (17.01%), PBINL (14.13%), and DE (13.02%). Whereas a low GAM was observed for the trait LL (8.74%). Similarly, Abate et al. (Citation2018) and Kumari (Citation2016) reported the highest GAM values for NSB, NBPP, NPB, DF, PBL, PH, and ODAP content. A previous study has supported our finding that the trait LL is not suitable for selection (Abate et al., Citation2018; Tadesse & Bekele, Citation2003b).
This finding revealed that high heritability and genetic advance as a percent of the mean have been observed for the NSB, NBPP, LBB, ODAP content, NPB, DF, PH, and PBL. This result suggests a larger contribution of additive gene action to the exhibition of these attributes. Thus, improvement could be achieved through a selection of these traits. A similar result was reported by Abate et al. (Citation2018), Das (Citation2015) and Tadesse and Bekele (Citation2003b) who obtained high heritability coupled with genetic advancement for DF, ODAP content, PH, and NPB. This study also showed high heritability with a low genetic advance for leaflet length. This indicates that the prevalence of non-additive gene effect on the inheritance of leaflet length selection based on this trait may not be achieved for the improvement of this crop similar explanation for the trait having a non-additive gene effect had been noted by Abate et al. (Citation2018) and Phundan & Narayanan (Citation1993).
Conclusion
Grass pea has been given little research focus at the global level in general and in Ethiopia in particular. The present study clearly showed the existence of good levels of genetic diversity and variability for non-yield quantitative traits and seed ODAP content. The existence of accessions with ODAP content lower than the safe level for human consumption, namely Acc 238945 (0.17%) and Acc 26627 (0.185%). This suggests possibilities for developing better genotypes through breeding using genetic materials from local sources. This could be substantiated by the high estimates of heritability and expected genetic advance from selection observed in the present study.
Authors’ contributions
All authors contributed to the study conception and design and provided the last consent for publication. MDA designed the experiments, executed the experiment, analyzed the data, and drafted the manuscript; AA was the main mentor and revised the manuscript; OH and AC were co-supervisors and revised the manuscript; ATD, ZA, and TAA revised the manuscript.
Supplemental Material
Download MS Word (44.2 KB)Acknowledgments
Thanks a lot, to the Department of Biotechnology, Faculty of Bioscience at Ebonyi State University (EBSU), Abakaliki, Nigeria, and the GENEs Intra-Africa academic mobility project for hosting and funding this research. I’m grateful to the Ethiopian Biodiversity Institute (EBI) for giving us the grass pea accessions.
Disclosure statement
The authors state there are no issues with money or competing interests regarding the work.
Data availability statement
The data backing up this finding can be obtained through the corresponding author, who will provide it upon reasonable request.
Additional information
Funding
Notes on contributors
Desalegn Abebe Mekonen
Desalegn Abebe Mekonen M.Sc. in Agricultural Biotechnology, leads the plant tissue culture and biotechnology research case team and works as an associate researcher at Amhara Agricultural Research Institute/ARARI in Bahir Dar, Ethiopia. He studied biotechnology as well as agricultural biotechnology. He currently works at the Amhara Agricultural Research Institute. Furthermore, he has written over two articles in reputed journals. His research interests include genomics, metabolomics, proteomics, transcriptomics, gene editing, plant-microbe interaction, crop genetic diversity, plant tissue culture, plant breeding, and molecular breeding.
References
- Abate, A., Mekbib, F., Fikre, A., & Ahmed, S. (2018). Genetic variability and heritability in Ethiopian Grass pea (Lathyrus sativus L.) accessions. Ethiopian Journal of Crop Science, 6, 1–8.
- Ahmadi, J., Vaezi, B., & Pour-Aboughadareh, A. (2015). Assessment of heritability and relationships among agronomic characters in grass pea (Lathyrus sativus L.) under rainfed conditions. Biharean Biologist, 9, 29–34.
- Allard, R. (1960). Principles of plant breeding (pp. 458). John Wiley and Sons Inc.
- Arslan, M., Basak, M., Aksu, E., Uzun, B., & Yol, E. (2020). Genotyping of Low β-ODAP Grass Pea (Lathyrus sativus L.) Germplasm with EST-SSR Markers. Brazilian Archives of Biology and Technology, 63, 1–13. https://doi.org/10.1590/1678-4324-2020190150
- Banerjee, J., Das, A., Parihar, A. K., Sharma, R., Pramanik, K., & Barpete, S. (2022). Genomic designing towards development of abiotic stress tolerant grass pea for food and nutritional security. In: Kole, C. (Eds.), Genomic designing for abiotic stress resistant pulse crops (pp. 345–381). Springer. https://doi.org/10.1007/978-3-030-91039-6_9
- Briggs, C., Parreno, N., & Campbell, C. (1983). Phytochemical assessment of Lathyrus species for the neurotoxic agent, β-N-oxalyl-L-α-β-diamino propionic acid. Planta Medica, 47(3), 188–190. https://doi.org/10.1055/s-2007-969982
- Chowdhury, S. D., Sultana, Z., Ahammed, M., Chowdhury, B. L., Das, S. C., & Roy, B. C. (2005). The nutritional value of khesari (Lathyrus sativus) for growing and laying pullets. The Journal of Poultry Science, 42(4), 308–320. https://doi.org/10.2141/jpsa.42.308
- Dahiya, B. (1976). Seed morphology as an indicator of low neurotoxin in Lathyrus sativus L. Qualitas Plantarum Plant Foods for Human Nutrition, 25(3-4), 391–394. https://doi.org/10.1007/BF02590314
- Das, P. (2015). Genetic analysis and molecular characterization in grass pea (Lathyrus sativus L [Doctoral dissertation]. IGKV.
- Deshmukh, S., Basu, M., & Reddy, P. (1986). Genetic variability, character association and path coefficients of quantitative traits in Virginia bunch varieties of groundnut. Indian Journal of Agricultural Sciences, 56, 812–816.
- Edwards, A., Njaci, I., Sarkar, A., Jiang, Z., Kaithakottil, G. G., Moore, C., Cheema, J., Stevenson, C. E. M., Rejzek, M., Novák, P., Vigouroux, M., Vickers, M., Wouters, R. H. M., Paajanen, P., Steuernagel, B., Moore, J. D., Higgins, J., Swarbreck, D., Martens, S., … Emmrich, P. M. F. (2023). Genomics and biochemical analyses reveal a metabolon key to β-L-ODAP biosynthesis in Lathyrus sativus. Nature Communications, 14(1), 876. https://doi.org/10.1038/s41467-023-36503-2
- Emmrich, P. (2017). Genetic improvement of grass pea (Lathyrus sativus) for low β-L-ODAP content [Doctoral dissertation]. University of East Anglia.
- Fikre, A. (2008). Improving the nutritional quality of Lathyrus sativus L.(grass pea) for safer consumption [Doctoral dissertation]. Ghent University.
- Girma, D., & Korbu, L. (2012). Genetic improvement of grass pea (Lathyrus sativus) in Ethiopia: an unfulfilled promise. Plant Breeding, 131(2), 231–236. https://doi.org/10.1111/j.1439-0523.2011.01935.x
- Hanbury, C., White, C., Mullan, B., & Siddique, K. (2000). A review of the potential of Lathyrus sativus L. and L. cicera L. grain for use as animal feed. Animal Feed Science and Technology, 87(1-2), 1–27. https://doi.org/10.1016/S0377-8401(00)00186-3
- Hillocks, R., & Maruthi, M. (2012). Grass pea (Lathyrus sativus): Is there a case for further crop improvement? Euphytica, 186(3), 647–654. https://doi.org/10.1007/s10681-012-0702-4
- IPGRI. (2000). Descriptors for Lathyrus spp. International Plant Genetic Resources Institute.
- Jeberson, M. S., Gonmei, R., Manish Kumar, S. K., Singh, N. B., & Sharma, P. R. (2018). Genetic variability, heritability, correlation coefficient, and path analysis in Lathyrus for yield and its related contributions under NEH conditions. Journal of Pharmacognosy and Phytochemistry, 7, 1806–1809.
- Johnson, H. W., Robinson, H. F., & Comstock, R. E. (1955). Estimates of genetic and environmental variability in soybeans. Agronomy Journal, 47(7), 314–318. https://doi.org/10.2134/agronj1955.00021962004700070009x
- Kassie, M., Shiferaw, B., Asfaw, S., Abate, T., Muricho, G., Ferede, S., … Assefa, K. (2009). Current situation and future outlooks of the chickpea sub-sector in Ethiopia. ICRISAT and EIAR. http://www. icrisat
- Kumar, S., Bejiga, G., Ahmed, S., Nakkoul, H., & Sarker, A. (2011). Genetic improvement of grass pea for low neurotoxin (β-ODAP) content. Food and Chemical Toxicology: An International Journal Published for the British Industrial Biological Research Association, 49(3), 589–600. https://doi.org/10.1016/j.fct.2010.06.051
- Kumar, S., Gupta, P., Barpete, S., Choukri, H., Maalouf, F., Sarkar, A., Pratap, A., & Gupta, S. (2021). Grass pea. In The beans and the peas. From orphan to mainstream crops (pp. 273–287). Woodhead Publishing, (Elsevier). https://doi.org/10.1016/B978-0-12-821450-3.00005-6
- Kumari, M. (2016). Genetic and molecular diversity analysis for high yield and drought tolerance in grass pea (Lathyrus sativus L) [Doctoral dissertation]. Indira Gandhi Krishi Vishwavidyalaya.
- Mahapatra, N. S., DAS, A., Bhattacharyya, P. K., Bhattacharya, S., Pal, S., & Barpete, S. (2020). Studies on genetic variability, divergence, and association of characters in grass pea. Journal of Crop and Weed, 16(1), 155–161. https://doi.org/10.22271/09746315.2020.v16.i1.1287
- Mekonen, D. A., Abraham, A., Oselebe, H., Afiukwa, C., Ilesanmi, O., & Abebe, T. D. (2022). Genetic diversity and population structure analysis of Grass pea (Lathyrus sativus L.) accession collected from North-Western Ethiopia using SSR markers. Genetic Resources and Crop Evolution, 69(3), 1247–1260. https://doi.org/10.1007/s10722-021-01302-5
- Omidi, A. H., Khazaei, H., & Hongbo, S. (2009). Variation for some important agronomic traits in 100 spring safflower (Carthamus tinctorius L.) genotypes. American-Eurasian Journal of Agricultural & Environmental Sciences, 5, 791–795.
- Phundan, S., & Narayanan, S. S. (1993). Biometrical techniques in plant breeding. Kalyani Publishers.
- Rao, S., Adiga, P., & Sarma, P. (1964). The isolation and characterization of β-N-oxalyl-L-α, β-diaminopropionic acid: a neurotoxin from the seeds of Lathyrus sativus. Biochemistry, 3(3), 432–436. https://doi.org/10.1021/bi00891a022
- Sas, I. (2002). SAS software (vol. 9). SAS Institute Inc.
- Sen Gupta, D., Barpete, S., Kumar, J., & Kumar, S. (2021). Breeding for better grain quality in Lathyrus. In Gupta, D. S., Gupta, S., Kumar, J. (Eds.), Breeding for enhanced nutrition and bio-active compounds in food legumes (pp. 131–156). Springer. https://doi.org/10.1007/978-3-030-59215-8_6
- Tadesse, W., & Bekele, E. (2003a). Phenotypic diversity of Ethiopian grass pea (Lathyrus sativus L.) in relation to geographical regions and altitudinal range. Genetic Resources and Crop Evolution, 50(5), 497–505. https://doi.org/10.1023/A:1023923813312
- Tadesse, W., & Bekele, E. (2003b). Variation and association of morphological and biochemical characters in grass pea (Lathyrus sativus L. Euphytica, 130(3), 315–324. https://doi.org/10.1023/A:1023087903679
- Teferra, T. F. (2021). Advanced and feasible pulses processing technologies for Ethiopia to achieve better economic and nutritional goals: A review. Heliyon, 7(7), e07459. https://doi.org/10.1016/j.heliyon.2021.e07459
- Tekle-Haimanot, R., Abegaz, B. M., Wuhib, E., Kassina, A., Kidane, Y., Kebede, N., Alemu, T., & Spencer, P. S. (1993). Pattern of Lathyrus sativus (grass pea) consumption and beta-N-oxalyl-α-β-diamino propionic acid (β-ODAP) content of food samples in the Lathyrism endemic region of northwest Ethiopia. Nutrition Research, 13(10), 1113–1126. https://doi.org/10.1016/S0271-5317(05)80736-5
- Tesema, A. A., & Mekonen, D. A. (2023). Responses of Niger [Guizotia abyssinica (Lf) Cass.] to cadmium and nickel stress. Vegetos, 1–11. https://doi.org/10.1007/s42535-023-00591-8
- Van Dijk, M., Morley, T., Rau, M. L., & Saghai, Y. (2021). A meta-analysis of projected global food demand and population at risk of hunger for the period 2010–2050. Nature Food, 2(7), 494–501. https://doi.org/10.1038/s43016-021-00322-9
- Wiraguna, E., Malik, A. I., Colmer, T. D., & Erskine, W. (2020). Waterlogging tolerance of grass pea (Lathyrus sativus L.) at germination related to country of origin. Experimental Agriculture, 56(6), 837–850. https://doi.org/10.1017/S0014479720000356
- Yates, F. (1936). Incomplete Latin squares. The Journal of Agricultural Science, 26(2), 301–315. https://doi.org/10.1017/S0021859600022000