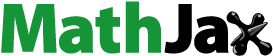
ABSTRACT
In this study, we investigated the effects of an ethanolic extract of Mangifera indica L. kernel on the viability and proliferation of human lung cancer cells. We utilized MTT and BrdU cell proliferation assays, morphological assessments, cell cycle analyses, and apoptosis assays to investigate the extract’s effects on lung cancer (A549 and NCI-H292) and normal lung (MRC-5) cells. The extract demonstrated a toxicity toward cancer cells compared to normal cells with dose-dependent anti-proliferative effect on lung cancer cells. The extract also caused differential effects on the cell cycle, inducing G0/G1 arrest and increasing the Sub-G1 population in both lung cancer and normal lung cells. Notably, the extract induced loss of membrane integrity, shrinkage, membrane blebbing, and apoptosis in lung cancer cells, while normal cells exhibited only early apoptosis. Furthermore, the extract exhibited higher toxicity towards NCI-H292 cells, followed by A549 and normal MRC-5 cells in decreasing order of potency. Our results suggest that the ethanolic extract of M. indica L. kernel has significant potential as a novel therapeutic agent for treating lung cancer cells, given its ability to induce apoptosis in cancer cell lines while causing minimal harm to normal cells.
1. Introduction
The global burden of cancer remains a significant health concern, as it is the second leading cause of mortality worldwide.Citation1–3 In 2020, approximately 19.3 million new cancer cases and 10.0 million cancer-related deaths were reported, representing a 22% rise in cancer diagnoses since 1990. Lung, breast, colon, and stomach cancers were identified as the most common types. Lung cancer is responsible for the greatest number of cancer fatalities (18.0%), followed by colorectal (9.4%), liver (8.3%), stomach (7.7%), and female breast (6.9%) cancers.Citation4 For men, lung cancer is the primary cause of cancer deaths, followed by prostate, colorectal, and liver cancers.Citation2,Citation4 Current chemotherapeutic drugs face limitations due to their low tolerability and severe side effects, including myelosuppression, heart damage, and kidney toxicity.Citation5–8 Moreover, cancer cells’ development of drug resistance further hinders treatment success.Citation9,Citation10 As a result, there is a pressing need for alternative therapeutic options, such as targeted treatments, immune-based therapies, and the use of natural compounds with fewer side effects and improved effectiveness.Citation11,Citation12 Researchers have recently turned their attention to plant-derived compounds, exploring their potential anticancer properties to discover new agents with greater specificity, reduced toxicity, and a lower risk of resistance development.Citation13–15 The investigation of bioactive compounds in plants, including flavonoids, tannins, and phenols, has uncovered promising leads for the development of innovative anticancer drugs. These natural compounds demonstrate potential in affecting various cancer-related pathways, such as regulating the cell cycle, inducing apoptosis, inhibiting angiogenesis, and preventing metastasis.Citation16–18 The increasing prevalence of cancer worldwide necessitates the continuous pursuit of inventive and efficacious therapeutic approaches. By examining the action mechanisms of plant-derived compounds and assessing their safety and effectiveness in preclinical and clinical trials, researchers can contribute to the advancement of novel treatment options for cancer patients.
A wealth of medicinal plants are recognized for their rich phytochemical content, which possesses antitumor properties.Citation19–21 These plant-derived antitumor agents encompass a variety of compound classes, including vinca alkaloids,Citation22,Citation23 epipodophyllotoxins,Citation23,Citation24 taxanes,Citation23,Citation25 and camptothecins.Citation26 Notably, taxanes and camptothecins constitute approximately 33% of all commercially available anticancer drugs.Citation27,Citation28
Species within the Mangifera genus (belonging to the Anacardiaceae family) have a long-standing traditional use in treating a range of respiratory tract ailments, such as influenza,Citation29 asthma,Citation30 cough,Citation31 bronchitisCitation32 and other bronchial conditions.Citation33 Additionally, extracts from certain Mangifera species exhibit anti-inflammatoryCitation34–37 and antimicrobial properties.Citation38,Citation39 In particular, products derived from Mangifera indica have demonstrated antimicrobial activity against Staphylococcus aureus, Bacillus subtilis, Escherichia coli, and Pseudomonas aeruginosa.Citation40 Mangifera indica is native to tropical regions and is widely found in countries such as the Philippines, Bangladesh, India, Pakistan, Africa, and Brazil.Citation41 The plant produces mangoes, which serve as the national fruit of India, Pakistan, and the Philippines.Citation42 A significant advantage of Mangifera indica-derived products is their lack of side effects upon consumption,Citation43,Citation44 rendering them safe for use as therapeutic agents in treating various diseases. The therapeutic potential of Mangifera indica can be attributed to its rich phytochemical composition, including bioactive compounds such as flavonoids, tannins, and phenols, which are known to exert various pharmacological effects. Studies have revealed that these compounds possess antioxidative, anti-inflammatory, and immunomodulatory properties, which contribute to their efficacy in treating respiratory and inflammatory conditions. Moreover, their antimicrobial action may be linked to their ability to disrupt bacterial cell walls, inhibit essential enzymes, and interfere with quorum sensing, a mechanism that regulates bacterial virulence and biofilm formation.
In our prior research, we demonstrated that the primary chemical constituents of the Mangifera plant are flavonoids, tannins, and phenols.Citation45 These bioactive compounds encompass a diverse range of molecules, including epigallocatechin, chlorogenic acid, apigenin, epicatechin, rutin, p-coumaric acid, myricetin, mangiferin, 4-hydroxybenzoic acid, gallic acid, quercetin, and syringic acid.Citation45
In a series of prior investigations,Citation40,Citation45–48 we demonstrated that the ethanolic extract derived from the kernel of Mangifera indica possesses bioactive components with cytotoxic properties against breast cancer cell lines. Building on these findings and to further explore the anticancer potential of Mangifera indica, we conducted the present study to examine the impact of the plant’s kernel extract on lung cancer cell lines. This research aims to assess the extract’s efficacy in inhibiting the growth, proliferation, and survival of lung cancer cells, as well as elucidate the underlying molecular mechanisms involved in cancer progression. Ultimately, these findings could contribute to the development of novel, plant-based lung cancer therapeutic.
2. Materials and methods
2.1. Chemicals and reagents
Various chemicals and reagents were sourced from different suppliers for this study. Invitrogen (Carlsbad, CA, USA) provided the trypsin/ethylenediaminetetraacetic acid (EDTA) solution. Sigma Chemical Company (Perth, Australia) supplied dimethyl sulfoxide (DMSO), phosphate-buffered saline (PBS), 3-(4,5-dimethylthiazol-2-yl)-2,5-diphenyltetrazolium bromide (MTT), Dulbecco’s modified Eagle’s medium (DMEM), and trypan blue dye. Additionally, the diphenylamine (DPA) reagent was prepared using 100 mL glacial acetic acid, 1.5 g diphenylamine, 1.5 mL concentrated sulfuric acid, and 0.5 mL of a 16 mg/mL acetaldehyde stock solution, also obtained from Sigma Chemical Company.
2.2. Ethanolic extraction of Mangifera indica kernel
Mangifera indica waterlily mango fruits were obtained from a local market in Kuala Lumpur, Malaysia, and identified by Dr. Shamsul Khamis, a resident botanist at the Institute of Bioscience, Universiti Putra Malaysia. A voucher specimen (SK2448/14) was deposited at the Institute. The kernels were manually processed by soaking them in water to remove any residual flesh, followed by drying in an oven at 45°C for two days. Once dried, the kernels were ground using a Waring blender 7011HS (Osaka Chemical Co. Ltd., Kita-Ku, Osaka, Japan) and stored at 4°C until further use. For the extraction process, 95% ethanol was added to the ground kernel at a 10:1 (v/w) ratio, and the mixture was shaken at 200 rpm in an incubator shaker (INNOVA 4000, New Jersey, USA) at 37°C for 24 hours. After incubation, insoluble materials were filtered out, and the suspension was centrifuged at 4000 rpm (Benchtop Centrifuge Z200A, Labnet International, Inc., Woodbridge, NJ, USA) for 10 minutes. The resulting supernatant was dried using a 1 L Rotary Evaporator N1001S-WD (Tokyo Rikakikai Co., Ltd., Koishikawa Bunkyo-Ku, Tokyo, Japan). The dried extract was then dissolved in DMSO and stored at −20°C until further analysis.Citation48
2.3. Cell culture
Virus-negative human mucoepidermoid bronchiolar carcinoma (NCI-H292) cells, human adenocarcinoma alveolar basal epithelial (A549) cells, and normal human lung (MRC-5) cells were procured from the American Type Culture Collection (ATCC; Rockville, MD, USA). The cells were cultured in Dulbecco’s Modified Eagle Medium (DMEM) (Sigma Aldrich, USA), supplemented with 10% fetal bovine serum (FBS) and 1% penicillin (100 U/mL) (Isocillin, Aventis, Germany). The cell cultures were maintained in a controlled environment at 37°C with 5% CO2 in an incubator.
2.4. MTT assay for cell viability
In this study, the 3-(4,5-dimethylthiazol-2-yl)-2,5-diphenyltetrazolium bromide (MTT) assay was performed to assess cell viability. Initially, 200 µL of cancer cell suspension (5 × 103 cells/mL) and normal cell suspension (5 × 105 cells/mL) were added to the appropriate wells of a 96-well cell culture plate. After aspirating the medium from the wells, the cells were treated with 200 μL of Mangifera indica kernel ethanolic extract (concentrations ranging from 1.56 to 100 μg/mL) or paclitaxel (0.156 to 10.0 μg/mL) in the medium. One row of wells in the plate was designated as the non-treatment control group. The plates were incubated at 37°C under 5% CO2 for 24 hours. Following incubation, the medium was aspirated, and the cells were washed three times with PBS buffer to remove test compounds. Fresh medium was then added to the wells. Subsequently, 200 μL of 5 mg/mL MTT solution was added to each well, and the plate was incubated for an additional 4 to 6 hours at 37°C under 5% CO2. After the incubation period, the MTT-containing medium was carefully removed and replaced with 200 μL of DMSO per well to dissolve the formazan crystals. The absorbance was measured at 570 nm using an automated spectrophotometric EL 340 multiplate reader (Bio-Tek Instruments Inc., USA). Cell viability was determined by employing the following formula:
Where OD is the optical density
The dose-response curves were utilized to establish the concentration of test compounds required to inhibit 50% of cell growth (IC50) for each cell line under investigation.
2.5. BrdU cell proliferation assay to assess anti-proliferative effects
To evaluate the anti-proliferative effects of the extract on the cells, the Bromodeoxyuridine (BrdU) incorporation assay was conducted using the colorimetric Cell Proliferation ELISA Kit (Roche, Germany). The cells were seeded at a density of 1 × 104 cancer cells and 1 × 105 normal cells per 200 µL in the respective wells of 96-well plates and maintained under optimal conditions.Citation17 After a 24-hour incubation, the medium was aspirated and replaced with 200 µL of either extract (concentrations ranging from 1.56 to 100 μg/mL) or paclitaxel (0.156 to 10.0 μg/mL) in the medium. The cells were then treated for 24, 48, or 72 hours. Subsequently, 10 µL of BrdU solution was added to each well to achieve a final concentration of 10 μM, and the plate was incubated for an additional 2 hours Following the manufacturer protocol. Cells incorporate BrdU into their DNA during the S-phase of the cell cycle when DNA synthesis occurs thus its used to estimate the proliferation of cells. After incubation, the BrdU-containing medium was removed, and the cells were fixed at room temperature for 30 minutes using cold 70% ethanol afterword, 25 μL of 1 M H2SO4 solution was added to each well to denature the DNA then it was incubated with peroxidase-conjugated anti-BrdU antibody for 90 minutes. The peroxidase conjugate was then coupled with a substrate and the resulting reaction product was measured for its light absorbance read at 450 nm (reference: 690 nm) using an ELISA reader (Bio-Tek Instruments Inc., USA). To account for any variations, the results were normalized by the number of viable cells present.
2.6. Cell cycle analysis using flow cytometry
The cells were treated with the ethanolic M. indica kernel extract at its IC50 concentration for 24 hours. Subsequently, the cells were washed twice with cold PBS, resuspended in 200 µL PBS, and fixed in 2 mL of ice-cold 70% ethanol for 2 hours at 4°C. The fixed cells were stored at 4°C until further analysis. Before the analysis, the cells were centrifuged to collect the pellets, which were resuspended in PBS. The cells were then stained with 50 µg/mL propidium iodide (PI) solution containing 0.1 mg/mL RNase A in PBS and incubated at 37°C for 15 minutes. The cells were mechanically resuspended using a 25-gauge syringe needle and subjected to cell cycle analysis using flow cytometry (FACS, Beckman Coulter). For the cell cycle distribution, at least 10,000 cells were analyzed. The proportions of cells in the G1/Go, S, and G2/M phases were determined from the DNA histograms. Cells with a sub-diploid amount of DNA in the sub-G1 peak indicated that they had undergone apoptosis.
2.7. Annexin V/Propidium iodide apoptosis assay using flow cytometry
To determine apoptosis in treated cells, an annexin V-FITC binding assay (BD Biosciences Pharmingen, San Diego, CA) was performed following the manufacturer’s guidelines. Treated cells were washed with PBS buffer and then resuspended in the staining solution for 10 minutes before flow cytometry analysis. In brief, 2 × 10^6 cells in a 200 µL suspension were plated in a 6-well plate containing DMEM with 10% FCS. The cells were treated with the extract at IC50 concentrations for 24 hours. Afterward, the cells were harvested, washed, and resuspended in 5 µL of Annexin V solution, followed by incubation in the dark for 10 minutes. Then, 10 µL of propidium iodide (PI) solution was added to the cell suspension before analysis using the Coulter Epics Altra flow cytometer (Beckman Coulter, USA). The acquired data, expressed as a percentage of cells, were analyzed using the BD Accuri™ Flow cytometer. The lower left quadrant (Q3: Annexin-V and PI negative) represented viable cells, the lower right quadrant (Q4: Annexin-V positive) represented early apoptotic cells, the upper right quadrant (Q2: Annexin-V and PI-positive) represented late apoptotic cells, and the upper left quadrant (Q1: PI-positive) represented necrotic cells.
2.8. Morphological assessment of cells through inverted light microscopy
A 200 µL suspension of either 1 × 10^4 NCI-H292, MRC-5, or A549 cells was seeded into the respective wells of a 6-well plate and treated with the ethanolic M. indica kernel extract at their respective IC50 concentrations. The cell morphology, including changes in cell shape, size, and integrity, was assessed using an inverted light microscope, which allowed for the examination of living cells in their natural environment. This analysis helped to identify any alterations in cell structure or behavior, such as cell shrinkage, membrane blebbing, or the formation of apoptotic bodies, which could be indicative of cell death or apoptosis triggered by the M. indica kernel extract treatment. Additionally, any differences in cell adhesion, spreading, and density between treated and untreated cells were carefully observed, as these factors may also provide insights into the anti-cancer potential of the extract.
2.9. Statistical analysis
All experiments were carried out in triplicate, and the data were expressed as mean ± standard deviation. Statistical analyses were conducted using Minitab statistical software (Minitab Inc., State College, PA). To determine the differences among the means of the treatment groups, a one-way analysis of variance (ANOVA) was performed, followed by Dunnett’s multiple comparison tests for post-hoc analysis. A p-value of less than 0.05 was considered statistically significant, indicating that the differences between the treatment groups were unlikely to have occurred by chance alone. This rigorous statistical approach ensured the reliability and validity of the study findings, providing a robust foundation for understanding the potential anti-cancer effects of M. indica kernel extract.
3. Results
3.1. Cytotoxicity
The cytotoxicity of ethanolic M. indica kernel extract against human lung cancer cell lines (A549 and NCI-H292) and normal lung cells (MRC-5) was assessed using the MTT assay. The estimated IC50 values after 24 hours were 9.55 μg/mL for A549, 12.32 μg/mL for NCI-H292, and 63.86 μg/mL for MRC-5. The M. indica kernel extract showed dose-dependent cytotoxicity against A549 and NCI-H292 cells (). The toxic effect was significant (p < .05) at concentrations as low as 3.125 µg/mL for NCI-H292 and 6.26 µg/mL for A549 cells. While the extract also caused a decrease in viability of MRC-5 cells, this effect was observed only at high extract concentrations of 50.0 and 100 µg/mL. Interestingly, the antiproliferative effect of the M. indica kernel extract on lung cancer and normal lung cells was found to be less potent than that of the anticancer drug, paclitaxel. The IC50 values of paclitaxel against A549, NCI-H292, and MRC-5 cells were found to be in the range of 0.89 to 0.15 μg/mL and were significantly (p < .05) lower than the IC50 values of the M. indica kernel extract. Despite this, the M. indica kernel extract exhibited selective toxicity against lung cancer cells as compared to normal lung cells (). These findings highlight the potential of the M. indica kernel extract as a promising candidate for further investigation in lung cancer therapy.
Figure 1. Viability of lung cancer, A549 and NCI-H292, and normal lung, MRC-5, cells treated with the ethanolic Mangifera indica L. kernel extract after 24 h, determined by the MTT assay. A549 = human adenocarcinoma alveolar basal epithelial cell; NCI-H292 = human muco-epidermoid bronchiolar carcinoma cell; MRC-5 = normal human lung cell. Values are mean ± standard deviation (n = 3 wells/treatment). p < .05 determined the significant differences among means.
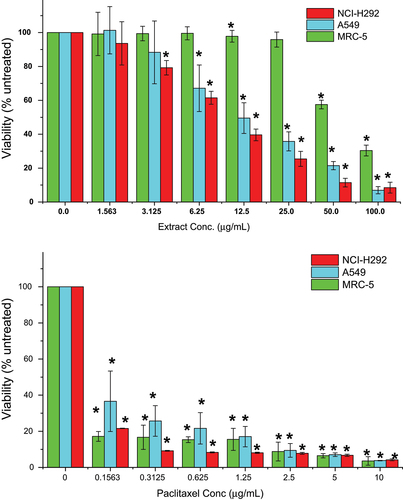
Table 1. IC50* values of mangifera indica L. kernel ethanolic extract and paclitaxel on NCI-H292, A549 and MRC-5 cells after 24 hours treatment.
3.2. Anti-proliferative effect
To assess the anti-proliferative effect of the M. indica kernel extract, BrdU incorporation assay was performed. The results showed that the lung cancer cells treated with the extract had low levels of BrdU incorporation, indicating poor proliferation of the cells. The effect of the extract on cancer cell proliferation was found to be more dependent on the concentration of the extract rather than the time of exposure ().
Figure 2. Proliferation of lung cancer, A549 and NCI-H292, and normal lung, MRC-5, cells treated with the ethanolic Mangifera indica L. kernel extract for 24, 48, or 72 h, determined by the BrdUassay.A549 = human adenocarcinoma alveolar basal epithelial cell; NCI-H292 = human muco-epidermoid bronchiolar carcinoma cell; MRC-5 = normal human lung cell. Values are mean ± standard deviation (n = 3 wells/treatment). p < .05 determined the significant differences among means.
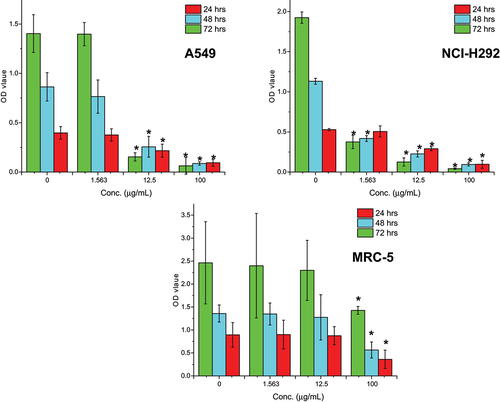
After 72 hours of exposure at a concentration of 12.5 μg/mL, the extract significantly (p < .05) decreased the proliferation of NCI-H292 and A549 cells by 93.5% and 89.0%, respectively, as compared to the untreated cells. On the other hand, the extract did not significantly (p > .05) inhibit MRC-5 cell proliferation except at very high concentrations of 100 μg/mL.
Overall, the M. indica kernel extract demonstrated a dose-dependent anti-proliferative effect on lung cancer cells, highlighting its potential as a promising candidate for further investigation in lung cancer therapy.
3.3. Morphological abnormality
The morphology of the nontreated lung cancer (NCI-H292 and A549) and normal lung (MRC-5) cells showed typical monolayer growth with cells adhering to the surface of the culture plate. The A549 cells exhibited a slightly polyhedral shape, typical of epithelial cells, while the MRC-5 cells were elongated and spindle-shaped. The NCI-H292 cells displayed a polyhedral structure, and all cells were aggregated, indicating normal growth ().
Figure 3. Morphology of lung cancer, A549 and NCI-H292, and normal lung, MRC-5, cells treated with the ethanolic Mangifera indica L. kernel extract at the IC50 concentration of 12.33, 9.55, and 63.86 μg/mL, respectively, for 24 h. A549 = human adenocarcinoma alveolar basal epithelial cell; NCI-H292 = human muco-epidermoid bronchiolar carcinoma cell; MRC-5 = normal human lung cell. Magnification: 200×.
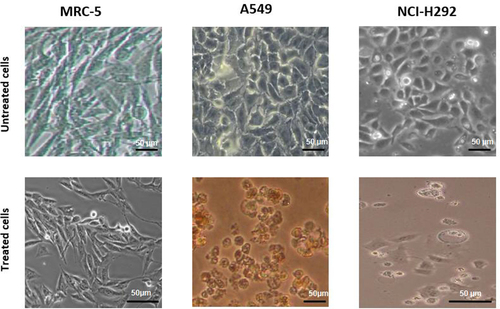
Treatment with the M. indica kernel extract at the respective IC50 concentration significantly altered the morphology of both cancerous and normal lung cells, as evidenced by a change in cell shape from elongated to rounded. The cytotoxic effect of the extract manifested in decreased growth of the normal lung MRC-5 cells and loss of membrane integrity, shrinkage, membrane blebbing, and apoptosis of the lung cancer cells.
The observed morphological abnormalities of the cancer cells upon treatment with the M. indica kernel extract are indicative of the disruption of key cellular processes, such as mitosis, DNA synthesis, and cell division, resulting in impaired growth and ultimately leading to cell death. The results of this study suggest that the M. indica kernel extract possesses potent anti-cancer activity and has the potential to be developed into an effective lung cancer therapeutic agent.
3.4. 4 cell cycle and sub-G1phase
Treatment with the M. indica kernel extract at its IC50 concentration for 24 hours caused significant alterations in the cell cycle distribution of the lung cancer and normal lung cells. As shown in (), the normal lung MRC-5 cells showed a significant (p < .05) increase in the percentage of cells in the G0/G1 phase after treatment, indicating cell cycle arrest at this phase. In contrast, the cancerous A549 and NCI-H292 cells had a lower population in the G0/G1 phase after treatment, suggesting a decrease in cell cycle arrest at this phase compared to the nontreated cells. Interestingly, the extract-treated MRC-5, A549, and NCI-H292 cells showed a significant increase (p < .05) in the percentage of cells in the sub-G1 phase, which represents apoptotic cells, compared to the nontreated cells. This increase was approximately 5.47X, 6.74X, and 14.19X for the MRC-5, A549, and NCI-H292 cells, respectively.
Figure 4. Effects of ethanolic Mangifera indica L. kernel extract at IC50 concentrations on cell cycle distribution of NCI-H292, A549 and MRC-5 cells and analyzed by flow cytometry. G0/G1, G2/M and S DNA contents indicate the cell phase, while and sub-G1 refers to the proportion of apoptotic cells. Values are means ± standard deviations (n = 3 well/treatment). *P<.05 compared to non-treated cells.
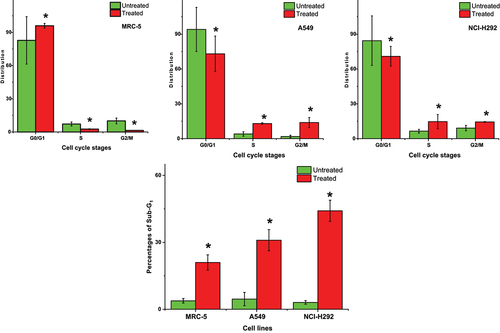
Furthermore, the MRC-5 cell population in the S and G2/M phases decreased significantly (p < .05) with extract treatment compared to the nontreated cells, indicating that the extract caused cell cycle arrest at these phases (). In contrast, the A549 and NCI-H292 cell populations in these cell cycle phases increased significantly (p < .05) after treatment compared to the nontreated cells, suggesting that the extract induced cell cycle progression at these phases. Specifically, the extract-treated A549 and NCI-H292 cell populations were approximately 2.2X and 1.3X more in the S phase compared to the untreated cells. Also, there was 6.35X and 0.58X increase in the G2/M phase compared to the nontreated cells, respectively. These findings suggest that the M. indica kernel extract may induce cell cycle arrest and apoptosis in the normal and lung cancer cells, and that its effect is concentration dependent.
3.5. Apoptosis
In the study, the ethanolic M. indica kernel extract was found to induce apoptosis in the lung cancer cells. The extract was able to induce early apoptosis in both A549 and MRC-5 cells, but it was observed that the extract caused more A549 cells to undergo early apoptosis compared to MRC-5 cells (). It was also noted that a significant proportion of NCI-H292 cells entered the late apoptotic and necrotic states after only 24 hours of treatment with the extract at a comparatively low concentration. These findings suggest that the NCI-H292 cells were most susceptible to the toxic effect of the extract. Interestingly, very few NCI-H292 cells were observed to undergo early apoptosis during the same period (). It was also noted that most of the nontreated cells remained viable after 24 hours, further emphasizing the potency of the extract in inducing apoptosis in lung cancer cells. Overall, the results suggest that the M. indica kernel extract has the potential to induce apoptosis in lung cancer cells, which may be beneficial in the development of new anticancer agents.
Figure 5. Scatter graph depicting annexin V (an) and propidium iodide (PI) double-staining assay of NCI-H292, A549 and MRC-5 cells treated with Mangifera indica L. kernel ethanolic extract at IC50 concentrations for 24 h. The Y-axis represents the PI-labeled population, whereas the X-axis represents the FITC-labeled annexin V positive cells. The lower left portion of the fluorocytogram (an ̶, PI ̶) shows viable cells, whereas the lower right portion of the fluorocytogram (An+, PI ̶) shows early apoptotic cells. The upper right portion of the fluorocytogram (An+, PI+) shows late apoptotic cells, whereas the upper left portion of the fluorocytogram (an ̶, PI+) shows necrotic cells.
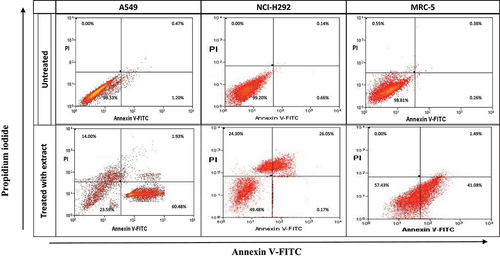
4. Discussion
Cancer therapeutics are developed to regulate uncontrolled cell proliferation and abnormal mass formation that interferes with the body’s vital functions during the development of tumors.Citation7,Citation49,Citation50 However, the most common cancer therapeutic strategy, the use of chemicals that induce apoptosis of cancer cells, has low specificity toward cancer cells and can be toxic to normal host cells.Citation17,Citation51
In our study, we investigated the potential of ethanolic M. indica L. kernel extract as an anti-lung cancer compound. To evaluate its efficacy, we used two lung cancer cell lines, A549 and NCI-H292. We found that the extract was highly toxic to NCI-H292 cells, and to a slightly lesser extent to A549 cells. The toxic effect of the extract on the cancer cells became apparent at a concentration of 6.26 μg/mL. This is a significant finding, as traditional chemotherapy agents such as paclitaxel are often toxic to both cancer and normal cell lines, even at low concentrations.
Furthermore, the anti-cancer effect of the M. indica kernel extract appeared to be time-dependent. At a low concentration of 1.563 μg/mL, the extract did not influence the rate of proliferation of A549 cells but inhibited the proliferation of NCI-H292 cells. However, at concentrations of 12.5 and 100 μg/mL, the extract significantly decreased (p < .05) the proliferation of both lung cancer cells, and the effect was more evident with an increase of exposure time.
We also evaluated the effect of the M. indica kernel extract on normal lung cells, MRC-5. We found that the extract only showed decreased proliferation after treatment with 100 μg/mL. This suggests that the extract may be selectively toxic to cancer cells and not normal cells, which is an important characteristic of an effective anti-cancer agent.
Our findings are consistent with a previous study, where we demonstrated that the M. indica kernel extract selectively inhibits the growth of human breast cancer cell lines, MCF-7 and MDA-MB-231, without significantly affecting the viability of normal breast cells, MCF-10a. Taken together, these findings suggest that the M. indica kernel extract may be a promising candidate for the development of a novel anti-cancer therapy. Further research is needed to determine the mechanisms underlying its anti-cancer effects and to optimize its therapeutic potential.Citation46
The ethanolic extract of M. indica kernel has been found to contain several phytochemicals, including fumaric acid, apigenin 7-glucoside, butylated hydroxytoluene, and phenols, which exhibit both antioxidantCitation45 and anticancer properties.Citation46 Certain phytochemicals such as quercetin and myricetin have been shown to induce apoptosis by activating caspase-3 and −9 activities.Citation46 In addition, the extract has been demonstrated to upregulate the expression of p53, Bax, and Bcl-2 proteins in estrogen receptor-positive human breast cancer MCF-7 cells,Citation47 suggesting that the extract may also target the same pathway in lung cancer cells. Interestingly, despite the presence of antioxidant phytochemicals, the M. indica kernel extract has been found to exert its anti-proliferative effects on breast cancer cells through the activation of lipid peroxidation and the generation of reactive oxygen species.Citation48 Therefore, the extract’s mechanism of action in lung cancer cells may be multifaceted and involve various signaling pathways.
Our study revealed that the M. indica kernel extract induced apoptosis in both the A549 and NCI-H292 lung cancer cells, as evidenced by changes in cell morphology. Apoptotic cells exhibit characteristic features such as cell shrinkage, chromatin condensation, and formation of apoptotic bodies.Citation44 These changes were similar to those observed in breast cancer cells treated with the extract.Citation47,Citation48 The reduction in cell number of treated cells further confirmed the toxic and anti-proliferative effects of the extract.
Apoptosis plays a crucial role in maintaining tissue homeostasis and preventing the development of cancer.Citation52 In contrast, necrosis is a form of cell death that occurs as a result of injury or disease, and is not under genetic control.Citation53 However, necroptosis is a programmed form of necrosis that is genetically regulated. Routine flow cytometry analysis cannot differentiate between necrosis and necroptosis, making apoptosis the logical target of anticancer drugs.Citation54 The ability of the M. indica kernel extract to induce apoptosis in lung cancer cells, though it’s very promising but it demands further research to study its potential as an anti-lung cancer agent. Our previous studies have shown that the extract contains phytochemicals such as quercetin and myricetin that have been shown to stimulate caspase-3 and −9 activities, leading to apoptosis in cancer cells.Citation41 Additionally, the extract was found to upregulate p53, Bax, and Bcl-2 proteins in breast cancer cells.Citation42 It is likely that similar mechanisms are responsible for the demonstrated effect of the extract.
Interestingly, despite containing phytochemicals with antioxidant properties, the extract was found to exert its anti-proliferative effects on breast cancer cells by activating lipid peroxidation and generating reactive oxygen species (ROS).Citation43 ROS are known to induce apoptosis in cancer cells,Citation55 and it is possible that the extract’s anticancer activity is also mediated through this mechanism in lung cancer cells.
Using cell cycle analysis, we observed that the M. indica kernel extract induced varying degrees of apoptosis in lung cancer cells. Specifically, after 24 hours of treatment, the extract caused a proportion of A549 cells to enter the early apoptosis stage, with some in the necrotic stage and very few in the late apoptotic stage. Similarly, in NCI-H292 cells, the extract caused the death of approximately 50% of the cells after the same treatment period, with cells almost equally distributed between the late apoptotic and necrotic stages. Few cells in this group were in the early apoptotic stage. The extract also caused some normal MRC-5 cells to enter the early apoptotic stage.
Our results indicate that the M. indica kernel extract is a more potent antiproliferative agent for NCI-H292 cells compared to A549 cells. However, it is important to note that the extract is not cancer-specific, as it also caused the death of some normal lung cells, albeit at a lower potency.
It is worth mentioning that the differences observed in the degree of apoptosis induction could be attributed to the varying genetic and molecular characteristics of the different cell lines used in this study. Further studies are necessary to determine the mechanisms underlying the differential effects of the extract on the different cell lines.
The cell cycle is a tightly regulated sequence of events that governs cell growth and replication. It is controlled by a network of regulatory proteins, including cyclins, cyclin-dependent kinases (CDKs), and CDK inhibitors such as p53, p21, and p27.Citation17,Citation56 In this study, the M. indica kernel extract was found to induce cell cycle arrest at the G2/M stages and apoptosis in both lung cancer and normal cells at their respective IC50 concentrations. However, the extract was most effective at inducing apoptosis in NCI-H292 cells, followed by A549 cells and normal MRC-5 cells, in order of decreasing potency.
A previous study using pure mangiferin, one of the active ingredients in M. indica, showed that it induced G1/M cell cycle arrest while also causing apoptosis in A549 cells.Citation54 However, the concentration of mangiferin in the M. indica kernel extract used in this study may be too low to induce G1/M cell cycle arrest but sufficient to induce apoptosis. Additionally, other chemical components of the extract, such as phenolic acids, flavonoids, and tannins, may also contribute to its anticancer effects.Citation57–60 The exact mechanisms by which each component of the extract induces anticancer effects remain unclear and require further investigation.
Overall, this study highlights the potential of the M. indica kernel extract as an anticancer agent through its ability to induce cell cycle arrest and apoptosis in lung cancer cells. However, the extract’s nonspecific cytotoxicity against normal lung cells warrants further investigation to ensure its safe use as a therapeutic agent.
5. Conclusion
The findings of this study demonstrated that the ethanolic M. indica kernel extract has selective toxicity toward lung cancer cells, with NCI-H292 cells being the most susceptible and A549 cells being less so. On the other hand, the normal lung cells, MRC-5, were found to be the least affected by the extract. The morphological changes observed in the treated lung cancer cells, such as membrane blebbing, shrinkage, and loss of membrane integrity, are characteristic of cells undergoing apoptosis. Furthermore, the extract induced G0/G1 phase cell cycle arrest and increased the apoptotic Sub-G1 population in all treated cells. These results suggest that the extract may have a potential therapeutic value as an antiproliferative agent for lung cancers. However, this claim demands further investigation to prove its efficacy in clinical setting.
It is important to note that the mechanism underlying the antiproliferative effects of the extract is not fully understood, and it is likely that various active components of the extract contribute to its efficacy. Previous studies have suggested that mangiferin, a component of the M. indica plant parts, can induce cell cycle arrest and apoptosis in lung cancer cells. However, the concentration of mangiferin in the M. indica kernel extract used in this study was relatively low, indicating that other chemical components of the extract may also be responsible for its antiproliferative effects.
The results of this study suggest the potential of M. indica kernel extract as a candidate for further exploration in treating lung cancers. However, it’s important to note that more research is required to fully understand the mechanisms of action, as well as to assess the safety and effectiveness of the extract in both preclinical and clinical settings.
Compliance with ethical guidelines involving plant-derived products
In this manuscript, we present our experimental research and field studies on Mangifera indica L. Kernel Ethanol Extract, a plant-based resource with potential applications in various fields. We strictly adhered to all relevant institutional, national, and international guidelines and legislation pertaining to the handling, collection, and utilization of plant material. Our study ensures the ethical and responsible use of plants, both cultivated and wild, while upholding the highest scientific standards for the benefit of our ecosystem and the advancement of knowledge.
supplementary.docx
Download MS Word (153.9 KB)Disclosure statement
The authors have no relevant affiliations or financial involvement with any organization or entity with a financial interest in or financial conflict with the subject matter or materials discussed in the manuscript. This includes employment, consultancies, honoraria, stock ownership or options, expert testimony, grants or patents received or pending, or royalties.
Data availability statement
The data that support the findings of this study are available from the corresponding author upon reasonable request. Restrictions apply to the availability of these data, which were used under license for the current study and so are not publicly available. However, data are available from the authors upon reasonable request and with permission of the data owner.
Supplementary data
Supplemental data for this article can be accessed online at https://doi.org/10.1080/23723556.2023.2299046
Additional information
Funding
References
- Al-Fahdawi MQ, Al-Doghachi FA, Abdullah QK, Hammad RT, Rasedee A, Ibrahim WN, Alshwyeh HA, Alosaimi AA, Aldosary SK, Eid EEM, et al. Oxidative stress cytotoxicity induced by platinum-doped magnesia nanoparticles in cancer cells. Biomed Pharmacother. 2021;138:111483. doi:10.1016/j.biopha.2021.111483.
- Al-Fahdawi MQ, Rasedee A, Al-Doghachi FA, Rosli R, Taufiq-Yap YH, Al-Qubaisi MS. Anticancer palladium-doped magnesia nanoparticles: synthesis, characterization, and in vitro study. Nanomedicine. 2020;15(6):547–11. doi:10.2217/nnm-2019-0178.
- Ibrahim WN, Doolaanea AA, MSB BAR. Effect of shRNA mediated silencing of YB-1 protein on the expression of matrix collagenases in malignant melanoma cell in vitro. Cells. 2018;7(1):7. doi:10.3390/cells7010007.
- Bray F, Ferlay J, Soerjomataram I, Siegel RL, Torre LA, Jemal A. Global cancer statistics 2018: GLOBOCAN estimates of incidence and mortality worldwide for 36 cancers in 185 countries. CA Cancer J Clin. 2018;68(6):394–424. doi:10.3322/caac.21492.
- Labbozzetta M, Notarbartolo M, Poma P. Can NF-κB be considered a valid drug target in neoplastic diseases? Our point of view. Int J Mol Sci. 2020;21(9):3070. doi:10.3390/ijms21093070.
- Al-Qubaisi MS, Rasedee A, Flaifel MH, Ahmad SH, Hussein-Al-Ali S, Hussein MZ, Eltayeb EM, Eid EEM, Arbab IA, Bandar Al-Asbahi A, et al. Induction of apoptosis in cancer cells by NiZn ferrite nanoparticles through mitochondrial cytochrome C release. Int J Nanomedicine. 2013;8:4115. doi:10.2147/IJN.S50061.
- Al-Qubaisi MS, Rasedee A, Flaifel MH, Ahmad SH, Hussein-Al-Ali S, Hussein MZ, Hussein MZ, Eltayeb EM, Eid EEM, et al. Cytotoxicity of nickel zinc ferrite nanoparticles on cancer cells of epithelial origin. Int J Nanomedicine. 2013;8:2497. doi:10.2147/IJN.S42367.
- D’Arcy MS. Cell death: a review of the major forms of apoptosis, necrosis and autophagy. Cell Biol Int. 2019;43(6):582–92. doi:10.1002/cbin.11137.
- Nurgali K, Jagoe RT, Abalo R. Editorial: adverse effects of cancer chemotherapy: anything new to improve tolerance and reduce sequelae? Front Pharmacol. 2018;9:245. doi:10.3389/fphar.2018.00245.
- Demaria M, O’Leary MN, Chang J, Shao L, Liu S, Alimirah F, Koenig K, Le C, Mitin N, Deal AM, et al. Cellular senescence promotes adverse effects of chemotherapy and cancer relapse. Cancer Discov. 2017;7(2):165–76. doi:10.1158/2159-8290.CD-16-0241.
- Said SS, Ibrahim WN. Cancer resistance to immunotherapy: comprehensive insights with future perspectives. Pharmaceutics. 2023;15(4):1143. doi:10.3390/pharmaceutics15041143.
- Almajali B, Johan MF, Al-Wajeeh AS, Wan Taib WR, Ismail I, Alhawamdeh M, Al-Tawarah NM, Ibrahim WN, Al-Rawashde FA, Al-Jamal HAN, et al. Gene expression profiling and protein analysis reveal suppression of the C-Myc oncogene and inhibition JAK/STAT and PI3K/AKT/mTOR Signaling by thymoquinone in acute myeloid leukemia cells. Pharm (Basel). 2022;15(3):307. doi:10.3390/ph15030307.
- Organization WHO. WHO traditional medicine strategy: 2014-2023. Hong Kong SAR, China: World Health Organization; 2013.
- Almajali B, Al-Jamal HAN, Wan Taib WR, Ismail I, Johan MF, Doolaanea AA, Ibrahim W, Tajudin SA. Thymoquinone suppresses cell proliferation and enhances apoptosis of HL60 leukemia cells through re-expression of JAK/STAT negative regulators. Asian Pac J Cancer Prev. 2021;22(3):879–885. doi:10.31557/APJCP.2021.22.3.879.
- Al-Qubaisi MS, Al-Abboodi AS, Alhassan FH, Hussein-Al-Ali S, Flaifel MH, Eid EEM, Alshwyeh HA, Hussein MZ, Alnasser SM, Saeed MI, et al. Preparation, characterization, in vitro drug release and anti-inflammatory of thymoquinone-loaded chitosan nanocomposite. Saudi Pharm J. 2022;30(4):347–358. doi:10.1016/j.jsps.2022.02.002.
- Wattanathamsan O, Hayakawa Y, Pongrakhananon V. Molecular mechanisms of natural compounds in cell death induction and sensitization to chemotherapeutic drugs in lung cancer. Phytother Res. 2019;33(10):2531–47. doi:10.1002/ptr.6422.
- Al-Qubaisi M, Rozita R, Yeap S-K, Omar A-R, Ali A-M, Alitheen NB. Selective cytotoxicity of goniothalamin against hepatoblastoma HepG2 cells. Molecules. 2011;16(4):2944–59. doi:10.3390/molecules16042944.
- Al-Qubaisi M, Rosli R, Subramani T, Omar AR, Yeap SK, Ali AM, Alitheen NB. Goniothalamin selectively induces apoptosis on human hepatoblastoma cells through caspase-3 activation. Nat Prod Res. 2013;27(23):2216–2218. doi:10.1080/14786419.2013.800979.
- Tariq A, Mussarat S, Adnan M. Review on ethnomedicinal, phytochemical and pharmacological evidence of Himalayan anticancer plants. J Ethnopharmacol. 2015;164:96–119. doi:10.1016/j.jep.2015.02.003.
- Chandra S, Rawat D. Medicinal plants of the family Caryophyllaceae: a review of ethno-medicinal uses and pharmacological properties. Integr Med Res. 2015;4(3):123–31. doi:10.1016/j.imr.2015.06.004.
- Hussain T, Bajpai S, Saeed M, Moin A, Alafnan A, Khan M, Kamal MA, Ganash M, Ashraf GM. Potentiating effect of ethnomedicinal plants against proliferation on different cancer cell lines. Curr Drug Metab. 2018;19(7):584–595. doi:10.2174/1389200219666180305144841.
- Mbele M, Hull R, Dlamini Z. African medicinal plants and their derivatives: current efforts towards potential anti-cancer drugs. Exp Mol Pathol. 2017;103(2):121–34. doi:10.1016/j.yexmp.2017.08.002.
- Kuruppu AI, Paranagama P, Goonasekara CL. Medicinal plants commonly used against cancer in traditional medicine formulae in Sri Lanka. Saudi Pharm J. 2019;27(4):565–73. doi:10.1016/j.jsps.2019.02.004.
- Chakraborty P. Herbal genomics as tools for dissecting new metabolic pathways of unexplored medicinal plants and drug discovery. Biochimie open. 2018;6:9–16. doi:10.1016/j.biopen.2017.12.003.
- Shah U, Shah R, Acharya S, Acharya N. Novel anticancer agents from plant sources. Chin J Nat Med. 2013;11(1):16–23. doi:10.1016/S1875-5364(13)60002-3.
- Shi M, Gong H, Cui L, Wang Q, Wang C, Wang Y, Kai G. Targeted metabolic engineering of committed steps improves anti-cancer drug camptothecin production in ophiorrhiza pumila hairy roots. Ind Crops Prod. 2020;148:112277. doi:10.1016/j.indcrop.2020.112277.
- Lichota A, Gwozdzinski K. Anticancer activity of natural compounds from plant and marine environment. Int J Mol Sci. 2018;19(11):3533. doi:10.3390/ijms19113533.
- Oberlies NH, Kroll DJ. Camptothecin and taxol: historic achievements in natural products research. J Nat Prod. 2004;67(2):129–35. doi:10.1021/np030498t.
- Amin AA, HSH R, Dulaimi AL, AA M, Rawi AL. Antiviral Activity of Mangifera Extract on Influenza Virus Cultivated in Different Cell Cultures. J Pure Appl Microbiol. 2019;13(1):455–458. doi:10.22207/JPAM.13.1.50.
- Rivera DG, Hernández I, Merino N, Luque Y, Álvarez A, Martín Y, Amador A, Nuevas L, Delgado R. Mangifera indica L. extract (Vimang) and mangiferin reduce the airway inflammation and Th2 cytokines in murine model of allergic asthma. J Pharm Pharmacol. 2011;63(10):1336–1345. doi:10.1111/j.2042-7158.2011.01328.x.
- Parvez GM. Pharmacological activities of mango (Mangifera indica): a review. J Pharmacogn Phytochem. 2016;5:1.
- Nikhal S, Dambe P, Ghongade D, Goupale D. Hydroalcoholic extraction of Mangifera indica (leaves) by Soxhletion. Int J Pharm Sci. 2010;2:30–32.
- Omar Bakr R, Wasfi R, Swilam N, Ezz Sallam I. Characterization of the bioactive constituents of nymphaea alba rhizomes and evaluation of anti-biofilm as well as antioxidant and cytotoxic properties. J Med Plant Res. 2016.
- Garrido G, González D, Lemus Y, Garcıa D, Lodeiro L, Quintero G, Delporte C, Núñez-Sellés AJ, Delgado R. In vivo and in vitro anti-inflammatory activity of Mangifera indica L. extract (VIMANG®). Pharmacol Res. 2004;50(2):143–149. doi:10.1016/j.phrs.2003.12.003.
- Márquez L, Pérez-Nievas BG, Gárate I, García-Bueno B, Madrigal JL, Menchén L, Garrido G, Leza JC. Anti-inflammatory effects of Mangifera indica L. extract in a model of colitis. World J Gastroenterol: wJG. 2010;16(39):4922. doi:10.3748/wjg.v16.i39.4922.
- Mohan C, Deepak M, Viswanatha G, Savinay G, Hanumantharaju V, Rajendra C, Halemani PD. Anti-oxidant and anti-inflammatory activity of leaf extracts and fractions of Mangifera indica. Asian Pac J Trop Med. 2013;6(4):311–314. doi:10.1016/S1995-7645(13)60062-0.
- Kim H, Banerjee N, Ivanov I, Pfent CM, Prudhomme KR, Bisson WH, Dashwood RH, Talcott ST, Mertens‐Talcott SU. Comparison of anti-inflammatory mechanisms of mango (Mangifera Indica L.) and pomegranate (Punica Granatum L.) in a preclinical model of colitis. Molecular Nutrition Food Res. 2016;60(9):1912–1923. doi:10.1002/mnfr.201501008.
- Engels C, Schieber A, Gänzle MG. Inhibitory spectra and modes of antimicrobial action of gallotannins from mango kernels (Mangifera indica L.). Appl Environ Microb. 2011;77(7):2215–23. doi:10.1128/AEM.02521-10.
- Anand G, Ravinanthan M, Basaviah R, Shetty AV. In vitro antimicrobial and cytotoxic effects of Anacardium occidentale and Mangifera indica in oral care. J Pharm Bioallied Sci. 2015;7(1):69. doi:10.4103/0975-7406.148780.
- Abdullah A-S, Mirghani MES, Jamal P. Antibacterial activity of Malaysian mango kernel. Afr J Biotechnol. 2011;10(81):18739–18748. doi:10.5897/AJB11.2746.
- Singh Nagendra K, Sharma Tilak R. crop of the Day: mango, Mangifera indica, the evolution of crop plants. Indian J Hist Sci. 2016;51.2.2:355–368.
- Lauricella M, Emanuele S, Calvaruso G, Giuliano M, D’Anneo A. Multifaceted health benefits of Mangifera indica L.(Mango): the inestimable value of orchards recently planted in Sicilian rural areas. Nutrients. 2017;9(5):525. doi:10.3390/nu9050525.
- López-Ríos L, Wiebe JC, Vega-Morales T, Gericke N. Central nervous system activities of extract Mangifera indica L. J Ethnopharmacol. 2020;260:112996. doi:10.1016/j.jep.2020.112996.
- Wightman EL, Jackson PA, Forster J, Khan J, Wiebe JC, Gericke N, Kennedy DO. Acute effects of a polyphenol-rich leaf extract of Mangifera indica l.(zynamite) on cognitive function in healthy adults: a double-blind, placebo-controlled crossover study. Nutrients. 2020;12(8):2194. doi:10.3390/nu12082194.
- Abdullah A-S, Mohammed AS, Abdullah R, Mirghani MES. Identification and quantification of phenolic compounds in Mangifera indica waterlily kernel and their free radical scavenging activity. J Adv Agric Technol Vol. 2015;2(1). doi: 10.12720/joaat.2.1.1-7.
- Abdullah A-S, Mohammed AS, Abdullah R, Mirghani MES, Al-Qubaisi M. Cytotoxic effects of Mangifera indica L. kernel extract on human breast cancer (MCF-7 and MDA-MB-231 cell lines) and bioactive constituents in the crude extract. BMC Complement Altern Med. 2014;14(1):1–10. doi:10.1186/1472-6882-14-199.
- Abdullah A-S, Mohammed AS, Rasedee A, Mirghani MES. Oxidative stress-mediated apoptosis induced by ethanolic mango seed extract in cultured estrogen receptor positive breast cancer MCF-7 cells. Int J Mol Sci. 2015;16(2):3528–36. doi:10.3390/ijms16023528.
- Abdullah A-S, Mohammed AS, Rasedee A, Mirghani MES, Al-Qubaisi MS. Induction of apoptosis and oxidative stress in estrogen receptor-negative breast cancer, MDA-MB231 cells, by ethanolic mango seed extract. BMC Complement Altern Med. 2015;15(1):1–7. doi:10.1186/s12906-015-0575-x.
- Said SS, Ibrahim WN. Cancer Resistance to Immunotherapy: Comprehensive Insights with Future Perspectives. Pharmaceutics. 2023;15(4):1143–1161.
- Al-Qubaisi MS, Rasedee A, Flaifel MH, Eid EE, Hussein-Al-Ali S, Alhassan FH, Salih AM, Hussein MZ, Zainal Z, Sani D, et al. Characterization of thymoquinone/hydroxypropyl-β-cyclodextrin inclusion complex: application to anti-allergy properties. Eur J Pharm Sci. 2019;133:167–82. doi:10.1016/j.ejps.2019.03.015.
- Shanmugam PST. Understanding cancer. Therapies: CRC Press; 2018.
- Lopez J, Tait S. Mitochondrial apoptosis: killing cancer using the enemy within. Br J Cancer. 2015;112(6):957–62. doi:10.1038/bjc.2015.85.
- Proskuryakov SY, Konoplyannikov AG, Gabai VL. Necrosis: a specific form of programmed cell death? Exp Cell Res. 2003;283(1):1–16. doi:10.1016/S0014-4827(02)00027-7.
- Pfeffer CM, Singh AT. Apoptosis: a target for anticancer therapy. Int J Mol Sci. 2018;19(2):448. doi:10.3390/ijms19020448.
- Al-Fahdawi MQ, Al-Doghachi FAJ, Abdullah QK, Hammad RT, Rasedee A, Ibrahim WN, Alshwyeh HA, Alosaimi AA, Aldosary SK, Eid EEM, et al. Oxidative stress cytotoxicity induced by platinum-doped magnesia nanoparticles in cancer cells. Biomed Pharmacother. 2021;138:111483. doi:10.1016/j.biopha.2021.111483.
- Bury M, Le Calvé B, Ferbeyre G, Blank V, Lessard F. New insights into CDK regulators: novel opportunities for cancer therapy. Trends Cell Biol. 2021;31(5):331–344. doi:10.1016/j.tcb.2021.01.010.
- González-Sarrías A, Li L, Seeram NP. Anticancer effects of maple syrup phenolics and extracts on proliferation, apoptosis, and cell cycle arrest of human colon cells. J Funct Foods. 2012;4(1):185–96. doi:10.1016/j.jff.2011.10.004.
- Noratto GD, Bertoldi MC, Krenek K, Talcott ST, Stringheta PC, Mertens-Talcott SU. Anticarcinogenic effects of polyphenolics from mango (Mangifera indica) varieties. J Agr Food Chem. 2010;58(7):4104–12. doi:10.1021/jf903161g.
- Bakar MFA, Mohamad M, Rahmat A, Burr SA, Fry JR. Cytotoxicity, cell cycle arrest, and apoptosis in breast cancer cell lines exposed to an extract of the seed kernel of Mangifera pajang (bambangan). Food Chem Toxicol. 2010;48(6):1688–97. doi:10.1016/j.fct.2010.03.046.
- Nemec MJ, Kim H, Marciante AB, Barnes RC, Hendrick ED, Bisson WH, Talcott ST, Mertens-Talcott SU. Polyphenolics from mango (Mangifera indica L.) suppress breast cancer ductal carcinoma in situ proliferation through activation of AMPK pathway and suppression of mTOR in athymic nude mice. J Nutr Biochem. 2017;41:12–19. doi:10.1016/j.jnutbio.2016.11.005.