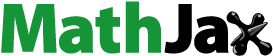
ABSTRACT
Structured light refers to the ability to tailor light in its many degrees of freedom, from the traditional control in time and space to more exotic multi-dimensional control for new forms of light, including vectorial light, toroidal excitations, spatio-temporal vortices, skyrmions, and optical mobius strips to name but a few. While the toolbox for the creation and detection of structured light has advanced tremendously, this has mostly been in the low power regime. More recently, structured light at high-power and high-intensities has emerged, fuelling new science and applications. In this progress report, we showcase the seminal work that has advanced this field, and indicate the open challenges and opportunities that remain.
1. Introduction
Shaping light dates back thousands of years [Citation1] but remained very much a niche topic because of the complexity to calculate the desired shaping solution, and the improbability to implement the solution with technology on hand. The field exploded in the 1990s when micro-electronic technology changed the game: modern lithography meant both faster computing chips and small feature sizes in fabricated devices, which could easily be transferred to the optical domain. Complex beam shaping solutions could now both be calculated numerically and the solutions fabricated using accurate lithographic technology: digital tools for optics. The resulting advances based on computer generated holograms [Citation2–5] saw enhanced optical functionality beyond just reflection and refraction and led directly to control of light in all its degrees of freedom (DoFs), for what today we refer to as structured light [Citation6]. At this point, the authors would like to point out that in the field of optical engineering, structured light refers to the process in which a known pattern is projected onto an object or scene of interest. The manner in which this pattern of light deforms when illuminating the surfaces allows for depth and surface information of the objects within the scene to be inferred (as in the case of structured light 3D scanners). However, the term ‘structured light’ in this review article refers to altering the various DoFs of an optical field, such as spatial (amplitude and phase) and polarisation profiles.
At first, the spatial DoFs were exploited, for example, amplitude, phase, and polarization, in 2D (the transverse plane) and later in 3D (all three components of the electric field), while the time and frequency domains could be shaped with the aid of simple dispersive elements for 4D control. In parallel, the shaping tools extended beyond refractive ray-tracing based elements [Citation7,Citation8] and propagation-phase control [Citation9], to include geometric phase [Citation10,Citation11] and combinations of both phases through metasurfaces [Citation12]. The aforementioned approaches are all static, which greatly limits their versatility. In the past decade or so, the field has accelerated again with the introduction of rewritable solutions in the form of liquid crystal spatial light modulators (SLMs) [Citation13,Citation14] and digital micro-mirror devices (DMDs) [Citation15,Citation16], which have been used extensively for structuring light. Compared to liquid crystal SLMs, DMDs are faster, cheaper, polarization, and wavelength insensitive, but at the expense of optical efficiency [Citation17].
A particularly topical form of structured light has been that carrying angular momentum, both as a spin component of per photon and as an orbital component of
per photon, where
is an integer. The interest stems from the mechanical equivalence of photonic angular momentum to mechanical angular momentum [Citation18], and the fact that the orbital component of photonic angular momentum can be linked directly to light’s spatial structure through an azimuthally varying phase, making orbital angular momentum (OAM) light directly associated to conventional laser modes [Citation19]. This opened the path for OAM carrying light to be easily created in optical laboratories, resulting in an explosion of activity in many diverse fields of research. The creation of both scalar OAM beams (with one polarization) and vectorial OAM beams (with orthogonal polarization components) at low power are possible with a range of tools, including spiral phase elements [Citation20,Citation21], q-plates [Citation22], simple glass cones [Citation23], stressed optics [Citation24], GRIN lenses [Citation25], as well as non-linear crystals [Citation26–28] and metasurfaces [Citation12]. It is beyond the scope of this article to cover this impressive body of work, and instead the reader is referred to many good reviews [Citation28–39] on the topic.
The design approaches to structuring light (the recipes) are very well established, and the toolkit for low power control is extensive, but the open challenge lies in how to translate this to high power and high-intensity fields, crucial if structured light is to migrate from the laboratory to real-world applications. The need for high power structured light over low powers is driven by a range of applications, such as laser machining, laser-enabled manufacturing, and optical communications, where spatial profiles enhance and improve the efficiency of such processes. In this progress report, we showcase the seminal work that has advanced this field and indicate the open challenges and opportunities that remain.
2. High-power OAM beams
In this section, we will highlight and discuss the progress made in generating high-power OAM beams. Structured light beams carrying OAM with different polarization states (scalar and vector) and topological charges (l) can be generated directly (intra) from the emission of solid-state or fiber laser cavities. Alternatively, they can be created after (extra) the output of a laser cavity using various beam-shaping or beam-manipulation techniques. Here, we will discuss techniques for both intra- and extra-cavity generation of scalar and vector OAM beams, including the generation and transformation from one mode family to another (i.e. HG
LG
). Considering the extensive scope of this topic, each of the subsections will highlight a few exemplary demonstrations of OAM beams from a continuous wave laser source having high average output powers, high topological charges, or both. Additionally, we highlight new technologies that show potential in these areas.
2.1. Intra-cavity OAM beams
2.1.1. Scalar fields
summarises the generation of scalar OAM beams by intra-cavity approaches. The critical point is that to achieve OAM emission it is necessary to suppress the competing eigenmodes. As illustrated in , one common approach is to excite the gain medium with an annular pump beam intensity profile to overlap optimally with the LG mode of the resonator cavity, thereby adjusting the cavity’s fundamental mode by controlling the gain region. Several approaches have been used to obtain annular pump beam shapes, such as capillary fibers [Citation44,Citation54], Dammann grating binary-phase elements [Citation43], and center-punched pump mirrors [Citation55] producing OAM emission at output powers in the range of a few watts. These systems are typically limited to selecting a fixed topological charge determined by the spatial characteristics of the annular pump intensity. Utilizing a spiral phase plate (SPP), which imparts an azimuthally varying phase, to create annular pump beams in optical parametric oscillators (OPOs) has proven to be an effective method for achieving dynamic and tunable OAM emission. These systems can generate OAM beams with topological charges ranging from l = 1 to 6, tunable wavelengths over the mid-IR wavelength range of 2260–3576 nm, and average output powers of up to 8 W [Citation40].
Figure 1. A collage of figures depicting various design approaches for intra-cavity scalar beam creation. These methods include annular optical pumping of solid-state crystals [Citation40–44], laser mode control through cavity design [Citation45–47], direct system-on-chip (SoC) OAM emitters [Citation48,Citation49], vertical-external-cavity surface-emitting (VECSEL) OAM lasers [Citation50,Citation51], complex laser emission using digital micro-mirror devices for optical pump profile control [Citation52], and degenerate ray-wave emission through off-axis optical pumping [Citation53].
![Figure 1. A collage of figures depicting various design approaches for intra-cavity scalar beam creation. These methods include annular optical pumping of solid-state crystals [Citation40–44], laser mode control through cavity design [Citation45–47], direct system-on-chip (SoC) OAM emitters [Citation48,Citation49], vertical-external-cavity surface-emitting (VECSEL) OAM lasers [Citation50,Citation51], complex laser emission using digital micro-mirror devices for optical pump profile control [Citation52], and degenerate ray-wave emission through off-axis optical pumping [Citation53].](/cms/asset/67318fe5-5689-4974-b119-7e7b4956d51d/tapx_a_2327453_f0001_oc.jpg)
An alternative to controlling the gain region in the resonator cavity is to manipulate the cavity losses by using circular defects etched on laser cavity mirrors to produce OAM emission capable of producing high-order topological charge output, as shown in . At the time of writing, this work has demonstrated the highest topological charge OAM emission from an intra-cavity resonator using an output coupler mirror inscribed with circular markings produced by ablating the coating layer, which produced l = 288 OAM emission [Citation47]. The topological charge number was determined using an astigmatic mode converter (AMC) to transform the LG mode into an HG mode and simply count the number of dark fringe lines [Citation56]. The authors claim that this technique can support intra-cavity OAM emission with topological charges of l > 1000.
Figure 2. (a) Experimental setup for generating an optical vortex from the laser reported in ref [Citation47]. (b) A photograph of the output coupler inscribed with round patterns, as well as a photograph and micrograph of the round patterns. (c) Beam profiles of the generated vortices for various topological charges. (d) The cylindrical lens mode converter and the accompanying HG beam profile. Adapted with permission from Ref. [Citation47].
![Figure 2. (a) Experimental setup for generating an optical vortex from the laser reported in ref [Citation47]. (b) A photograph of the output coupler inscribed with round patterns, as well as a photograph and micrograph of the round patterns. (c) Beam profiles of the generated vortices for various topological charges. (d) The cylindrical lens mode converter and the accompanying HG beam profile. Adapted with permission from Ref. [Citation47].](/cms/asset/1274e83e-c633-46e7-894a-647a3f002a1a/tapx_a_2327453_f0002_oc.jpg)
Tailoring the pump beam to have an annular spatial profile or introducing mirror defects is a fairly straightforward and convenient method for selecting circularly symmetric LG modes inside a cavity. Analogous to annular pumping for circular symmetric OAM emission, complex beam shaping devices such as DMDs can be used to shape the input pump beam to excite the gain region so that the rectangular symmetric resonator modes are favoured. This technique has demonstrated direct cavity emission of higher-order HG modes which can then be transformed, using an AMC, into a Laguerre-Gaussian mode LG
with a high topological charge number l = 90 [Citation52].
Alternatively, constructing a laser cavity to emit rectangular HG modes can be achieved through simple cavity alignment and subsequently transformed to an OAM beam using an AMC optical system placed outside the cavity. For instance, if the pump beam is displaced from the optical axis in an optical resonator cavity, known as off-axis pumping, one can easily create high-order HG mode emission without the need for spatial transformation of the pump beam. When combined with an AMC optical setup, this provides a straightforward method for generating pure OAM beams at high average powers. Similarly, applying off-axis pumping to degenerate laser cavities can produce ray-wave beams, whereby multiple higher-order HG beams are emitted from the cavity, resulting in multi-axis OAM beams when passed through an external AMC [Citation53].
Recently, an HG mode resonator cavity was constructed with an integrated Sagnac interferometric mode transforming output coupler, as shown in . This unique output coupler design produced a 95
pure LG
mode OAM output with an average power of 31.1 W, the highest intra-cavity OAM laser emission so far reported [Citation45]. This simple cavity design demonstrated an impressive level of absorbed pump-to-OAM emission optical efficiency of
, in both continuous-wave and pulsed operation.
Figure 3. (a) The Nd:YVO4 crystal vortex cavity laser setup. (b) The average output vortex laser power versus absorbed pump power in pulsed operation at 150 kHz Q-switching rate (black) and in continuous wave (CW) operation (red). Adapted with permission from ref [Citation45].
![Figure 3. (a) The Nd:YVO4 crystal vortex cavity laser setup. (b) The average output vortex laser power versus absorbed pump power in pulsed operation at 150 kHz Q-switching rate (black) and in continuous wave (CW) operation (red). Adapted with permission from ref [Citation45].](/cms/asset/1e08c5a8-b5c9-4c72-8056-9e2c35041eec/tapx_a_2327453_f0003_oc.jpg)
Thermally-induced aberrations in solid-state laser systems are a result of heat generated by inefficient energy transfer pathways from the pump absorption and laser emission energy bands in the gain medium. Typically, thermally-induced aberrations, such as spherical aberration, are mitigated by actively cooling the laser crystal; however, there are instances where thermal aberrations may be useful. For example, a novel bounce laser has been demonstrated using a simple cavity design with no additional intra-cavity optics, exploiting the usually unfavorable effects of spherical aberration to obtain an impressive 16 W average power pure emission [Citation57]. Other approaches, which also exploited spherical aberration, using lenses, have demonstrated pure
mode emission up to
with 1.87 W of output power [Citation46]. An improved version of this design allowed for pure
emission up to
, but the power was limited to <140 mW [Citation58].
Complex diffractive optical devices and elements placed directly inside the laser cavity provide a more direct approach to producing OAM beam emission. One example of this is the digital laser, which utilized a SLM as an end-mirror with an appropriate phase mask to control the fundamental resonator mode, capable of high topological charge output [Citation59]. However, due to the low damage threshold of liquid crystal cells and high diffraction losses, the digital laser is limited to producing only a few mW’s of structured light output. To achieve high-power intra-cavity OAM emission, static diffractive optical elements, such as metasurfaces [Citation60] and spiral phase mirrors [Citation61], are promising due to their high damage tolerance and conversion efficiencies.
Vertical External Cavity Surface Emitting Lasers (VECSELs) are semiconductor chips that comprise a compact multilayered system, typically consisting of a highly reflective end mirror and a gain medium. VECSEL technology has garnered significant interest from the photonics community, specifically quantum technologies, due to its small footprint, high-power capabilities, broad spectral emission range, and versatility for technology integration [Citation62]. The direct generation of structured light, using VECSEL technology, has been demonstrated using an off-axis pumped resonator to produce higher-order HG modes and converted to higher-order LG modes using an extra-cavity AMC at average powers exceeding 2 W [Citation63]. Recently, a similar system was designed with the AMC integrated into the resonator cavity, thus enabling simultaneous emission of HG and mode-transformed LG modes at low average power (20 mW) [Citation51]. To fully realize next-generation telecommunications and quantum computing devices, further miniaturization of structured light-emitting lasers with high topological charges and tunable wavelength outputs is necessary. Examples of such lasers include microchip lasers [Citation41,Citation42] and complete micron-sized laser systems [Citation48,Citation64,Citation65].
2.1.2. Vector fields
In this subsection, methods for generating inhomogeneously polarized (vector) OAM emission directly from a laser cavity will be discussed and are highlighted in . The advantages of using structured light with vector polarization states for material processing are well-known and have been studied for over two decades [Citation79]. During this time, only radially and azimuthally polarized OAM vector beams, particularly at the CO2 10.6 µm wavelength, were of interest due to the relatively simplistic and highly efficient ability to select them inside the resonator. To date, these systems still hold the title for the highest average power vector OAM beam output, achieved by retrofitting a resonant grating mirror into a commercial CO2 laser system producing a 3 kW RP OAM beam [Citation73]. Other more recent examples of vector OAM beams, at shorter Near-IR wavelengths, with an average output power of up to 2 kW have been demonstrated using other techniques, such as: triple-axicon retroreflectors with intra-cavity quarter-wave plates [Citation80], copper axicons [Citation81], and resonant grating mirrors [Citation82].
Figure 4. A collage of figures depicting design approaches for intra-cavity vectorial OAM beam creation. These systems include annular optical pumping of anisotropic solid-state crystals and single crystal fibers [Citation66–70], manipulation of the spatial, phase and polarization properties of cavity emission in solid-state [Citation71–76] and fiber lasers [Citation77,Citation78] using various control mechanisms.
![Figure 4. A collage of figures depicting design approaches for intra-cavity vectorial OAM beam creation. These systems include annular optical pumping of anisotropic solid-state crystals and single crystal fibers [Citation66–70], manipulation of the spatial, phase and polarization properties of cavity emission in solid-state [Citation71–76] and fiber lasers [Citation77,Citation78] using various control mechanisms.](/cms/asset/ae9408a7-f8ee-42e2-8fd1-2f4a12c2085a/tapx_a_2327453_f0004_oc.jpg)
As highlighted in , advancements in high-resolution lithography have made it possible to achieve up to 1 kW average power azimuthally polarized vector OAM beams of high vector purity in the 1 µm wavelength range utilizing the next generation of ultra-high damage threshold grating wave-guide end-mirrors and output couplers [Citation72,Citation83]. The advancement of grating waveguide mirror technologies offers a convenient solution for generating kW average power vector OAM emission. However, the current lithographic processes involved are not easily accessible and costly. In the following section, we explore a variety of alternative and exotic intra-cavity vector OAM generation techniques allowing not only azimuthally and radially polarized states but arbitrary vector polarization states and higher-order topological charges.
Figure 5. (a) Experimental setup whereby the HR mirror was replaced with a GWH to generate a radially polarized beam. (b) The laser performance comparing the grating output coupler generation and (c) the experimental beam profiles obtained with the grating output coupler. Adapted with permission from ref [Citation72,Citation83].
![Figure 5. (a) Experimental setup whereby the HR mirror was replaced with a GWH to generate a radially polarized beam. (b) The laser performance comparing the grating output coupler generation and (c) the experimental beam profiles obtained with the grating output coupler. Adapted with permission from ref [Citation72,Citation83].](/cms/asset/3fc454f6-068b-4da3-b25b-0ec3c0ab12a3/tapx_a_2327453_f0005_oc.jpg)
In a typical LG optical resonator, both radial and azimuthal polarization states can coexist simultaneously in the absence of polarization-selecting optics. However, when a polarization-sensitive component, such as a birefringent material, is introduced, the S and P-polarization states become decoupled. As a result, either polarization state may suffer a significant loss, while the other can continue to oscillate. This straightforward method of producing either radial or azimuthally polarized OAM beams using an intra-cavity birefringent material has been well-documented and demonstrated using a birefringent c-cut Nd: YVO4 crystal [Citation84,Citation85]. In optical resonators that incorporate a non-birefringent active gain media, a phenomenon known as thermal-lens-induced bi-focusing can occur. This effect leads to different focusing conditions for each S and P-polarization state. By adjusting the incident pump beam power and the relative position of the crystal with respect to the pump beam focus, the fundamental cavity oscillation can switch between radial and azimuthal polarization modes [Citation68,Citation86].
Under high-power pumping conditions, a pronounced bi-focusing effect arises, enabling the generation of vector polarization states with high topological charges, ranging from l = 1 to 14 [Citation70]. These mechanisms can be leveraged collectively. Recently, a stable, highly pure (95%), and high average power (20 W) emission of radially polarized LG mode at a wavelength of 2090 nm was achieved using annular pumping, thermally-induced birefringence, and bi-focusing mechanisms. This was realized using an end-pumped Ho: YAG crystal with an intra-cavity lens, placed on a translation stage, and controlled using a machine learning feedback loop [Citation67].
By incorporating a birefringent material within a laser resonator, the orthogonal polarization states become uncoupled and separated over free-space propagation. This displacement allows for the independent addressing of each polarization arm using a single phase-only SLM screen, which is divided into two halves. This technique, which shares similarities with the concept of a digital laser, enables simultaneous addressing of two beams. Experimental demonstrations have showcased the selection of orthogonally polarized HG modes on each half of the SLM screen. As the orthogonally polarized HG modes are reflected off the SLM and pass through the birefringent material upon their return, a superposition state is generated, resulting in the production of vector LG modes possessing topological charges of l = 1 and 2 [Citation71].
A more straightforward method for generating vector OAM beam emission involves the use of a vortex wave plate (VWP). The VWP is a static optical element that comprises a distribution of rotated half-wave phase retarders with an azimuthal variation. This configuration effectively converts linearly polarized Gaussian modes into OAM beams with radial or azimuthal-oriented polarization. The VWP has been shown to generate high-purity vortex OAM beams (with over 90% modal purity) and moderate slope efficiency of (11%) when placed inside a Gaussian mode resonator [Citation76]. Other instances of vector OAM emission have been showcased by employing an end-pumped Yb-doped fiber, a VWP, and a polarizing element to drive the fundamental mode of the fiber laser. These setups have exhibited remarkable performance, achieving high efficiency (66%), high modal purity (89.5%), and high average power (32 W) [Citation78]. Tunable topological charge OAM emission (l = 1 up to 3) with simultaneous vector polarization distribution control has been demonstrated, although at low output powers (2 mW) [Citation77].
A noteworthy demonstration of dynamic topological charge selection within the cavity has been presented using a J-plate metasurface, as shown in . This advancement marked the first demonstration of a ‘metasurface-assisted laser’ and produced the highest intra-cavity topological charge output for vector OAM beams [Citation75]. By rotating the metasurface within the cavity, the laser cavity showcased variable topological charge emissions, ranging from l = 10 to l = 100.
Figure 6. (a) Concept of the metasurface (J-plate) laser cavity with (b) corresponding produced states displayed on the generalised OAM sphere, with OAM orders (north pole) and
(south pole), illustrating lasing across differing OAM values as superpositions. Adapted with permission from ref [Citation75].
![Figure 6. (a) Concept of the metasurface (J-plate) laser cavity with (b) corresponding produced states displayed on the generalised OAM sphere, with OAM orders ℓ=10 (north pole) and ℓ=100 (south pole), illustrating lasing across differing OAM values as superpositions. Adapted with permission from ref [Citation75].](/cms/asset/cf124698-fb27-4c51-9571-a038f5444a6e/tapx_a_2327453_f0006_oc.jpg)
2.2. Extra-cavity OAM beams
2.2.1. Scalar fields
Generating scalar or vector OAM emission from within the laser cavity at high average output powers, especially with variable or high-topological charges, is challenging and generally requires high-precision alignment. This process demands high sensitivity and low tolerances to achieve optimal performance. An alternative approach to address this challenge is to create these OAM beams outside the laser cavity, utilizing static and/or dynamic phase and amplitude beam shaping devices in different configurations, some of which are highlighted in the summary figure given in . Extra-cavity beam selection offers advantages such as simpler implementation, higher sensitivity tolerances, greater controllability, and seamless integration with existing single-mode laser systems using a modular approach. In this section, we highlight the recent advances in the extra-cavity generation of high-power and high topological charge scalar and vector OAM fields using static and/or dynamic beam shaping elements.
Figure 7. A collage of figures depicting the various approaches for producing extra-cavity scalar OAM beams. These methods include utilizing transmissive or refractive spiral-phase elements [Citation87–92], through power scaling with an amplifier [Citation93], or by employing dynamic phase modulation techniques and modern technologies [Citation94–100].
![Figure 7. A collage of figures depicting the various approaches for producing extra-cavity scalar OAM beams. These methods include utilizing transmissive or refractive spiral-phase elements [Citation87–92], through power scaling with an amplifier [Citation93], or by employing dynamic phase modulation techniques and modern technologies [Citation94–100].](/cms/asset/e4aedb73-7cc0-4589-91d7-1443f71941d3/tapx_a_2327453_f0007_oc.jpg)
A common method for generating scalar OAM beams is to impart the azimuthally varying phase term () in the LG
function onto a Gaussian beam using a spiral phase element such as SPP. SPPs are fabricated to achieve a fixed topological charge, and the higher the desired topological charge, the more challenging the fabrication process [Citation101]. By stacking multiple SPPs with low-order (l) in series, it is possible to generate a vortex beam with a high topological charge, which accumulates a net OAM. In a demonstration, 6 SPPs (3 times l = 2 and 3 times l = 4) were stacked to create a vortex beam with a total topological charge of l = 18 [Citation90]. However, the purity of the final OAM beam is compromised due to unoptimized spatial overlap as the beam diverges. The highest reported average output power for an LG
mode with l = 3 and radial modes (p = 3) is 130 W with 97
purity, which was produced using a single phase element made of fused silica and etched with a phase pattern for a LG
mode with a blazed grating [Citation92]. The results and experimental setup of such are displayed in . Although there is no definitive value for the highest achievable power with SPP, theoretically, high powers (>100 W) are feasible since SPPs are also made of fused silica.
Figure 8. (a) The experimental setup for the mode cleaner cavity. (b) The mode purity for the beam transmitted by the mode cleaner and the fraction of the injected light power which is transmitted from the mode cleaner with intensity profiles of the 83 W LG beam transmitted by the mode cleaner. Adapted with permission from Ref. [Citation92].
![Figure 8. (a) The experimental setup for the mode cleaner cavity. (b) The mode purity for the beam transmitted by the mode cleaner and the fraction of the injected light power which is transmitted from the mode cleaner with intensity profiles of the 83 W LG 33 beam transmitted by the mode cleaner. Adapted with permission from Ref. [Citation92].](/cms/asset/26a6e9fe-9c92-4064-94a6-62c7aa139b59/tapx_a_2327453_f0008_oc.jpg)
It has been possible to create single-mode fiber exit tips with spiral-phase structures that permit direct transformation at the output using topological charges ranging from l = 1 to l = 3 [Citation89]. However, the output power of this approach is low. To improve on this technique, a nanostructured gradient index vortex mask (nVPM) was produced using 7651 nanorods made of two types of borosilicate materials with different refractivity indexes, which allowed for the effective imparting of the azimuthally varying 02π phase of topological charge l = 1 to the transmitted beam [Citation88]. Although nVPMs on fibers are encouraging for direct high-power generation, it will be difficult to achieve higher-order topological charges due to limitations in fabrication tolerances.
The spiral-phase mirror (SPM) is an analog to the SPP, which is reflective rather than transmissive but imparts the same azimuthally varying phase. The SPM holds the record for the highest topological charge OAM beam produced with using an aluminium mirror constructed with 125 segments, each imprinting a phase ramp of 160π [Citation91], the results of which are highlighted in .
Figure 9. A series of OAM modes generated with an intense laser and SPMs contained as inserts for (a) 500, (b) 1 000
and (c) 10 000
. Adapted with permission from Ref. [Citation91].
![Figure 9. A series of OAM modes generated with an intense laser and SPMs contained as inserts for (a) 500ℏ, (b) 1 000ℏ and (c) 10 000ℏ. Adapted with permission from Ref. [Citation91].](/cms/asset/6e99886a-2c1b-4ed6-baeb-dbfa79c3e15f/tapx_a_2327453_f0009_oc.jpg)
A liquid crystal cell-based reprogrammable SPP has been demonstrated as a cost-effective alternative to expensive devices like SLMs for generating high OAM beams. The SPP utilizes 24 pie-sliced segments of liquid crystal cells that allow for selective topological charges of , and 12, while still maintaining a high fill factor since there are no individual pixels. This technology has the potential to be useful in preparing high OAM beams [Citation96].
SLMs are considered the most versatile beam-shaping tool capable of performing phase-only or amplitude and phase beam shaping in real-time. Traditional SLMs have a liquid crystal display resolution of 1920 × 1080 pixels and can generate an OAM beam with a topological charge of up to l = 600 with high purity [Citation102]. Newer SLMs have a resolution of 3840 × 2160 pixels, four times greater than previous models, and can produce the highest topological charge of l = 1400 by following the same optimization process as previous models [Citation94]. Active water-cooling has substantially improved the thermal handling capabilities of SLMs from 2 W/cm2 (without water-cooling) to over 200 W/cm2 (with water-cooling) [Citation103], which is a promising development for direct generation of high-power and high OAM beams and structured light in general. During the preparation of this review, a research team at the Max Planck Institute for Gravitational Physics demonstrated the high-power selection of higher-order HG modes HG, HG
, and HG
, using a water-cooled Hamamatsu SLM. The input Gaussian mode used had a maximum continuous wave power of 85 W, corresponding to an average power density of > 600 W/cm2 at the SLM screen, which is a significant leap forward in the thermal-handling abilities of liquid crystal displays [Citation104].
Another dynamic beam-shaping system is the DMD, which is a 1024 × 768 array of micromirror pixels that can be individually turned ‘on’ and ‘off’ to direct reflected light into or away from the optical axis. On a DMD, OAM beams with topological charges as high as l = 90 can be generated through binary amplitude modulation and super-pixel resolution optimization [Citation95]. The deformable mirror has the potential to handle much higher power beams (up to a few kW) than DMDs or SLMs. Recently, a continuous surface deformable mirror demonstrated proof-of-concept dynamic generation of OAM with topological charges ranging from l = 1 to 5 at low powers [Citation100].
Coherent beam combination (CBC) has recently gained significant attention due to its potential for delivering high-power and high-purity variable OAM states on demand, aided by artificial intelligence and deep learning techniques. CBC involves the use of a seed fiber that is divided into multiple fibers with individual phase modulators, which are then amplified, collimated, and recombined. A deep learning algorithm analyzes a small portion of the output beam to perform OAM demultiplexing and makes iterative corrections to individual phase modulators in a feedback loop until the desired OAM mode is achieved with high purity [Citation99].
2.2.2. Vector fields
A summary of key findings for the generation of extra-cavity vector OAM beams that will be discussed is shown in . Various techniques exist and have been further enhanced for the creation of high-power vector OAM beams (external to the laser cavity), ranging from the use of SLMs, metasurfaces, and amplifier systems. These are discussed and highlighted below.
Figure 10. A collage of figures depicting various approaches for creating extra-cavity vectorial OAM beams. These methods include utilizing anisotropic crystal properties during solid-state and fiber amplification [Citation105–109], interferometric configurations for dynamic selection of vector polarization distributions [Citation110–114], and static phase elements for mode creation [Citation115–117].
![Figure 10. A collage of figures depicting various approaches for creating extra-cavity vectorial OAM beams. These methods include utilizing anisotropic crystal properties during solid-state and fiber amplification [Citation105–109], interferometric configurations for dynamic selection of vector polarization distributions [Citation110–114], and static phase elements for mode creation [Citation115–117].](/cms/asset/8cf3af38-125a-4cec-9e90-354e1b8c2cf0/tapx_a_2327453_f0010_oc.jpg)
Amplification of linear, radial, and azimuthal-polarized seed vector vortex laser beams via the use of a Yb: YAG single-crystal fiber (SCF) amplifier, configured in a single-pass orientation, has been shown [Citation105], highlighted in . The vector beam generation approach involves using an external polarization converter (consisting of segmented waveplates) to convert the linearly polarized seed laser to radially or azimuthally polarized beams. Here, the authors were able to demonstrate significant gain with a 17-fold and 3-fold amplification of linearly polarized modes (for seed powers of 1.1 W and 40 W, respectively). While for vector polarisation, amplified signals of 100 W at an initial pump power of 32 W has been shown. This compact SCF amplifier makes it well-suited to industrial applications. Similar approaches make use of a Yb-doped double-clad fibre as a gain medium for the amplification of radially polarized vector vortex beams [Citation106]. In this work, a radially polarized seed beam is created via a Nd:YAG laser oscillator having an output power of 1 W. The transmitted beam through the Yb-doped double-clad fibre maintains its intensity and polarization distribution, with a slight transformation of radial to azimuthal polarization, due to the cylindrical symmetry of the fibre being affected by coiling and twisting. Here, the amplified beam reaches an output power of 21 W. Other amplification approaches involve a thermally guiding fiber rod (TGFR) amplifier for the amplification of radially polarized beams [Citation108]. Here, the geometry of the TGFR ensures that thermally-induced stresses cause birefringence, allowing for purely radially polarized beams to be amplified without depolarization. This approach shows potential for scaling up to high-powers in the multi-hundred-watt regime. A more theoretical and experimental model has attempted to understand the control on the quality of high-power vector beams in amplified systems, in particular with Nd: YAG and Nd: YLF crystals in a master oscillator power amplifier (MOPA) configuration [Citation107]. It was shown that the purity of pure vector vortex beams remains invariant as the crystal orientation changes, while the purity for general vector vortex beams oscillates depending on the crystal rotation angle. Another novel amplification approaches for the creation of high-power radially polarized light uses a double-pass amplification setup in a Ho: YAG thin-slab crystal [Citation117]. The setup consists of a 13.2 W Ho: YAG rod laser, which exploits gain medium bifocusing together with a ring-shaped pump to promote preferential lasing on the radially polarized LG mode. Compensations are made for both thermally induced stress birefringence and the Gouy phase shift resulting from astigmatic focusing of the seed laser. This amplifier is capable of yielding an output power of up to 33.7 W.
Figure 11. The Yb:YAG single-crystal fiber amplifier setup (left). Intensity images of the amplified beam for radial (right, top) and azimuthal polarization (right, bottom) with accompanying intensity images for analyzer rotation angles. Adapted with permission from Ref. [Citation105].
![Figure 11. The Yb:YAG single-crystal fiber amplifier setup (left). Intensity images of the amplified beam for radial (right, top) and azimuthal polarization (right, bottom) with accompanying intensity images for analyzer rotation angles. Adapted with permission from Ref. [Citation105].](/cms/asset/d17262b0-76c1-4918-9313-01d94e0154f4/tapx_a_2327453_f0011_oc.jpg)
Some interferometric-based techniques involve the implementation of a Sagnac interferometer together with computer-generated holograms displayed on a SLM to produce annular vector vortex beams (also known as perfect vector vortex beams) possessing linear and elliptical polarizations [Citation109]. A series of cascaded q-plates in a ring Ytterbium-doped fiber laser has shown to produce arbitrary high-order Poincaré beams by adjusting the input polarization state [Citation112]. Another static approach makes use of common optical elements, such as a polarized beam splitter, right-angle prism, retroreflector, half- and quarter-wave plates, to form what the authors have labelled a vector field generator [Citation114]. It is designed in such a way to convert an input optical field carrying OAM into a vectorial field, producing various vectorial fields depending on the topological charge and the relative phase difference between the horizontal and vertical components of the input beam. A static approach for a fibre laser source involves a robust and efficient external polarization conversion method by utilizing a photonic crystal and segmented half-wave plate to perform radial polarization conversion [Citation115]. This photonic crystal is engineered to provide artificial birefringence, enabling efficient polarization conversion, and is capable of high-power handling in successfully generating over 100 W for a radially polarized beam.
Metasurfaces are becoming a popular choice for extra-cavity vector vortex beam generation due to their conversion efficiency, compactness, and ease in implementation. High-power cylindrical vector beams have been generated via a metasurface which behaves as a spatially inhomogeneous-phase delay plate with a spatially variable optical axis [Citation116]. By setting the phase retardation to π, the metasurface coverts an incident linearly polarized beam to a radially polarized cylindrical vector beam, the setup and results of which are displayed in . In this work, the researchers were able to produce a 500 W radially polarized cylindrical vector beam. Another novel, static approach for the generation of pure vortex beams with a single-step on-axis transformation uses a dielectric phase-amplitude metasurface, known as a p-plate [Citation113]. Here, the vectorial structuring of the input beam together with its projection onto an orthogonal polarization basis enables the manipulation of both the phase and amplitude of the vortex beam. It was shown that high purity can be achieved with the p-plate, where 98% purity was reached for a vortex beam with a high azimuthal index of . This makes the p-plate a promising candidate for generating high-purity, high-order vortex beams.
Figure 12. Experimental setup for the incorporation of a metasurface (MS) for the conversion from linear to radial polarization (top, left). Photo and space-varying optical axis directions of the metasurface (bottom, left). Various intensity images of the produced radially polarized cylindrical vector beams after passing through a Glan laser prism at different output powers (right). Adapted with permission from Ref. [Citation116].
![Figure 12. Experimental setup for the incorporation of a metasurface (MS) for the conversion from linear to radial polarization (top, left). Photo and space-varying optical axis directions of the metasurface (bottom, left). Various intensity images of the produced radially polarized cylindrical vector beams after passing through a Glan laser prism at different output powers (right). Adapted with permission from Ref. [Citation116].](/cms/asset/622ee439-a48f-4d4a-877f-fffbccaa022a/tapx_a_2327453_f0012_oc.jpg)
3. High-intensity OAM beams
In the previous section, scalar and vector OAM beams from an intra and extra cavity with high continuous wave power and high topological charges were presented. The advancements of structured light, specifically scalar and vector OAM beams, in pulsed operation with high peak powers are discussed in this section. Peak power (W) is proportional to the beam’s temporal pulse duration (s), which spans many orders of magnitude from nanoseconds () to femtoseconds (
), and recently to attoseconds (
), so equivalently, the peak powers of pulsed laser beams span equally as many orders of magnitude range from kilowatts (
) to terawatts (
). The logarithmic scale plot in highlights some of the most significant advances and achievements in the generation of scalar and vector OAM beams in the high peak power and ultrashort pulse duration regimes, including; solid-state resonators (green) [Citation124,Citation125], Yb-doped fiber amplifiers (light blue) [Citation122,Citation126–128], bulk solid-state MOPAs (yellow) [Citation129,Citation130], thin-disk resonators (purple) [Citation131,Citation132], single crystal fiber (SCF) amplifiers (pink) [Citation133–135], multipass thin-disk amplifiers (magenta) [Citation120,Citation123,Citation136–139], and ultrafast chirped-pulse amplified lasers (red) [Citation118,Citation119,Citation140,Citation141] are all depicted in each fiber region. The range of high-intensity OAM-producing systems is extensive, so we will only focus on a select few from some of the fibered categories mentioned.
Figure 13. A logarithmic scale plot highlighting significant advances in the generation of scalar and vector OAM beams in the high peak power and ultrashort pulse duration regimes. (a) Laser focal spots measured without and with a spiral phase plate placed in the path of a ϕ = 25 mm diameter laser beam with corresponding spectrally resolved high-order harmonic 1D angular profiles. (b) Setup of a the few-cycle vortex beam generation experiment with a 1.8 µm laser source and corresponding polarization measurements of the radially polarized beam. (c) Experimental setup of the CVB generation process with femtosecond pulses at 1030 nm, together with a S-waveplate to transform Gaussian-shaped beam into donut-shaped beam and corresponding simulated intensity profiles of CVBs. (d) Experimental setup for the generation of femtosecond optical vortex beams with megawatt powers from a fiber based mamyshev oscillator. (e) The setup for a picosecond YDF-MOPA for amplifying radially polarized beams and corresponding radially polarized beams generated by a q-plate. (f) The setup for a thin-disk multipass amplifier which delivers linearly polarized pulses subsequently converted to azimuthal polarization which is later amplified to kW-levels. Adapted with permission from refs: (a) [Citation118], (b) [Citation119], (c) [Citation120], (d) [Citation121], (e) [Citation122], (f) [Citation123].
![Figure 13. A logarithmic scale plot highlighting significant advances in the generation of scalar and vector OAM beams in the high peak power and ultrashort pulse duration regimes. (a) Laser focal spots measured without and with a spiral phase plate placed in the path of a ϕ = 25 mm diameter laser beam with corresponding spectrally resolved high-order harmonic 1D angular profiles. (b) Setup of a the few-cycle vortex beam generation experiment with a 1.8 µm laser source and corresponding polarization measurements of the radially polarized beam. (c) Experimental setup of the CVB generation process with femtosecond pulses at 1030 nm, together with a S-waveplate to transform Gaussian-shaped beam into donut-shaped beam and corresponding simulated intensity profiles of CVBs. (d) Experimental setup for the generation of femtosecond optical vortex beams with megawatt powers from a fiber based mamyshev oscillator. (e) The setup for a picosecond YDF-MOPA for amplifying radially polarized beams and corresponding radially polarized beams generated by a q-plate. (f) The setup for a thin-disk multipass amplifier which delivers linearly polarized pulses subsequently converted to azimuthal polarization which is later amplified to kW-levels. Adapted with permission from refs: (a) [Citation118], (b) [Citation119], (c) [Citation120], (d) [Citation121], (e) [Citation122], (f) [Citation123].](/cms/asset/9233f581-35d8-4c36-b4ec-ee85c690197c/tapx_a_2327453_f0013_oc.jpg)
It is well known that injecting a pulsed Gaussian beam into a carefully stress-doped fiber amplifier can produce a high-intensity vortex output, thus performing the beam shaping and amplification in a single step [Citation126]. ) depicts a more recent technique, in which a q-plate is used to convert a linearly polarized Gaussian beam into an OAM beam (l = 1) before being injected directly into a Yb-doped fiber MOPA system, resulting in high average output power (106 W), high peak power (270 kW), and high pulse energies (30.7 µJ) radially polarized picosecond pulses with high purity [Citation122]. Among the thin-disk resonators category (purple) is a Mamyshev oscillator, ), which used an intra-cavity q-plate to produce OAM () emission with pulse energies of 235 nJ and peak power of 2.2 MW, after external pulse compression to 76 fs, which to our knowledge is the highest pulse energy and peak power obtained directly from a fiber oscillator [Citation121]. In both pulsed and continuous wave operation, CBC technology, as mentioned in the previous section, is a promising technique for obtaining dynamic high-power laser modes. Recently, a 61-channel femtosecond CBC system demonstrated variable OAM (
) in the femtosecond pulse regime with peak powers reaching 35 MW [Citation142].
Power scaling of OAM beams using thin-disk multipass MOPAs has seen major improvements since it was first demonstrated, where it achieved an impressive average power of 635 W at 6.5 ps, corresponding to a peak power of over 240 MW [Citation137]. Since then, improvements were made to the MOPA design to increase the number of amplification passes from 20 to 30. Using a femtosecond (800 fs) laser source, radially polarized OAM beams were produced using a spatially varying waveplate and subsequently amplified using the improved MOPA design to a peak power of 1.2 GW [Citation123]. A few years later, the thin-disk architecture was applied in a dual MOPA configuration, where the first MOPA stage produced an amplified Gaussian pulse which was converted to an azimuthally polarized OAM beam using the segmented halfwave plate and injected into a second MOPA, with 44 passes, producing the highest average power azimuthally polarized OAM beam to date, with an average power and pulse energy of 1.7 kW and 5.8 mJ, respectively, and peak power of 699 MW [Citation138]. One year later, the same group used an amplified femtosecond (<500 fs) Gaussian pulse with peak powers of 1.9 GW and performed extra-cavity conversion to linearly polarized OAM beams using an S-waveplate and achieved peak powers of 1.7 GW (802 W average power) at a wavelength of 1030 nm and additionally performed frequency doubling to produce 837 MW (320 W average power) of 523 nm wavelength green OAM beams [Citation139]. Another approach to obtaining high peak power femtosecond Gaussian pulses is to add additional amplification steps during the broadband chirped pulse amplifier (CPA) stage before pulse compression and conversion from Gaussian to a radial or azimuthally polarized OAM beam using an S-waveplate [Citation120].
Naturally, because the peak power is inversely proportional to the temporal pulse duration, we can see that femtosecond time scales, specifically sub-100 fs pulses, currently have the highest peak powers. When compared to linearly polarized Gaussian beams, the angular momentum component of OAM beams allows for tighter focus, particularly in radially polarized states, and thus higher peak intensities, making them well suited for studying strong-field physics [Citation143] and high-harmonic generation [Citation118]; laser-driven particle acceleration [Citation141]; and relativistic physics [Citation144]. One of the world’s highest peak-power ultrafast systems, the 100 TW-class Ti: sapphire laser (UHI100) at CEA-IRAMIS is dedicated to ultra-high intensity (UHI) physics experiments. The UHI100 system was used to investigate the interaction of OAM beams with plasma mirrors in order to determine whether OAM is conserved during the generation of high-order harmonics in the reflected beam [Citation118]. To the best of our knowledge, this experiment is the highest peak-power extra-cavity generation of an OAM (l = 1) beam using an SPP with an incident Gaussian having a peak power of ±24 TW.
4. High power structured light
We have thoroughly investigated the vast landscape of intra- and extra-cavity, scalar and vector OAM beams with high power, intensity, topological charges, and tuneability. However, such beams only represent an infinitesimal slice of the possible forms of structured light. Bessel beams (BB) are another form of structured light that has received much attention in the last few decades for its intriguing properties, such as: extended depth of focus and non-diffracting nature that make it appealing to many high-power applications [Citation145], and are easily produced by passing a plane-wave through an axicon. The most common form of ‘structured light’ used in industry is the multi-mode fiber laser, which exits the fiber tip as a uniform intensity or ‘flat-top’ which is then imaged to the work surface to perform various applications. Multi-mode fiber lasers are cost-effective and robust solutions with commercial systems exceeding 100 kW of average power currently available.
Accessing more complex and arbitrarily structured light is not straightforward, and it necessitates high-resolution complex-phase modulation devices, such as SLMs or DMDs, which have thermal limitations and are unable to access large powers. CBC technology, multi-core beam shaping, and photo-thermo-refractive (PTR) holographic phase masks are novel and promising technologies that can address the dynamic selection of structured light at high average and peak powers.
Civan Advanced Technologies is a laser manufacturing company specializing in coherent beam-combining technology laser systems. Their dynamic beam laser (DBL) can produce arbitrary beam-shaping capabilities at continuous wave powers of up to 100 kW. A seed laser is split into multiple channels (up to 100), where each channel is amplified and recombined with adjacent channels before being combined in the far field using guided interference patterns controlled by the optical-phase array (OPA) shown in ). Civian has created a versatile high-power laser system that controls not only the beam shape but also the spot size, depth of field, and beam steering by combining CBC and OPA technology [Citation146].
Figure 14. (a) The layout for the formation of a single output beam from a seed laser which follows a series of split-amplify stages that are are finally combined from the civan laser system, with corresponding intensity profile.(b) nLIGHT® fibre profile and corresponding near-field spatial profiles images when light is guided through the various cores or for two different fibre designs (bottom and top rows). (c) The mode analysis system implementing multiplexed holographic phase masks and the intensity profiles of a theoretical and experimental LG diffracted by the HPM 100 W and 1000 W beams, respectively. Adapted with permission from refs: (a) [Citation146], (b) [Citation147], (c) [Citation148].
![Figure 14. (a) The layout for the formation of a single output beam from a seed laser which follows a series of split-amplify stages that are are finally combined from the civan laser system, with corresponding intensity profile.(b) nLIGHT® fibre profile and corresponding near-field spatial profiles images when light is guided through the various cores or for two different fibre designs (bottom and top rows). (c) The mode analysis system implementing multiplexed holographic phase masks and the intensity profiles of a theoretical and experimental LG 08 diffracted by the HPM 100 W and 1000 W beams, respectively. Adapted with permission from refs: (a) [Citation146], (b) [Citation147], (c) [Citation148].](/cms/asset/561834ad-11ef-411b-b52d-678406e56e7b/tapx_a_2327453_f0014_oc.jpg)
Recently, the fiber laser manufacturing company nLIGHT® has released a new all-fiber beam-shaping technology Corona™, which is capable of delivering multi-kW power structured light on demand, allowing for an ad hoc approach to optimization per application requirement using a single laser system [Citation147]. Corona™ technology utilises a fiber laser splice to a delivery fiber having multiple core zones that act as individual guiding regions to produce a myriad of beam shapes at the output, as shown in ). Their proprietary technology allows for the fiber laser output to be controllably and dynamically partitioned among the guiding core regions within less than 30 ms for rapid on-demand structured light. Currently, nLIGHT® offers a three-zone fiber beam shaping system delivering up to 5 kW and a two-zone version capable of up to 20 kW.
Holographic phase masks (HPMs), are a new technology that incorporates a flexible SLM device to encode complex phase patterns into PTR glass. The PTR glass can be multiplexed as with ordinary holograms, does not require electric power, perform nearly arbitrary beam-phase transformations, and is tunable over the visible and near IR regions [Citation148]. This system’s efficiency was demonstrated at 1070 nm wavelength using a Gaussian mode with an average output power of 100 W to 1 kW to produce LG modes, making it the first successful mode conversion utilising a holographic optical element at these power levels, shown in ). An ultrafast laser source with central wavelength, pulse duration, and spectral bandwidth of 780 nm, 70 fs, and 17.2 nm were tested on the same HPM system to create pure modes and showed negligible chromatic dispersion or loss of the spectral components. As with traditional SLMs, the HPM allows for the multiplexing of many modes to perform modal analysis of any input beam.
5. Structured light applications
Applications of high-power laser sources span a vast volume of literature, ranging from surface hardening [Citation158], microtechnology [Citation159], ultra-thin metal processing [Citation160], defence [Citation161] and surgery [Citation162], to name but a few. However, the scope here is limited to the role that both OAM and structured high-power and high-brightness laser beams play in various applications, of which a handful are summarized in and discussed below.
Figure 15. Applications of high-power structured light beams are highly diverse, with some examples shown here covering laser materials processing [Citation149,Citation150], optical communication [Citation151,Citation152] and micro-fabrication [Citation153–157].
![Figure 15. Applications of high-power structured light beams are highly diverse, with some examples shown here covering laser materials processing [Citation149,Citation150], optical communication [Citation151,Citation152] and micro-fabrication [Citation153–157].](/cms/asset/e8f2fc0d-0936-453d-b6d9-ea8d63a15acf/tapx_a_2327453_f0015_oc.jpg)
5.1. Laser material processing
The Laser Materials Processing (LMP) industry is one of the earliest adopters of beam-shaping, owing to the increased benefit in implementing application-specific spatial profiles. Numerous configurations of laser systems are used in LMP and predominately solid state lasers in the forms of fiber and diode lasers. Fiber lasers, such as MOPA laser systems offer robust, high-peak power, multi-role laser sources by flexibly varying laser parameters (pulse shape and duration) [Citation163]. The use of compact diode lasers, incorporated with micro-optics, for the creation of homogeneous light fields for LMP applications is growing in interest [Citation164], followed by more recent publications moving towards on-the-fly, real-time programmability of beam sizes and spatial profiles [Citation150].
Within these laser systems, many differing optical designs and components are implemented depending on the desired profile, such as adaptive optics to correct for aberrations introduced when focusing inside the work-piece or to tailor the intensity distribution for parallelisation in reducing processing times [Citation165]. Due to their high-conversion efficiency and simple structure, refractive and diffractive optics are a popular choice for transforming a collimated Gaussian intensity profile into a top-hat and/or donut profile at different working distances [Citation166–168]. With increased computation power, neural network training algorithms have been harnessed for the development of multiple cascaded diffractive optical elements to optimize complex structured distributions (in terms of both intensity and phase) at multiple planes ideal for LMP applications [Citation169].
With the advancements in beam-shaping techniques, vector vortex modes have risen to the foreground as they offer tightly focused spots with steep intensity slopes ideal for a variety of uses within LMP [Citation170–173]. More specific LMP sub-divisions and how OAM, vector vortex, and structured modes have enhanced these areas are discussed in more in the following sub-sections below.
5.1.1. Cutting and drilling
Continuous-wave CO2 lasers have long been used in the LMP industry for the cutting and drilling of metal parts and then subsequently with Nd:YAG lasers consisting of hundreds of Watts. Laser developers have, and continue to, focus attention on the laser parameters needed for intricate and fine cutting of various metals. A review of such, dating back to 1999, can be found in Ref [Citation174]. Here, it is noted that a Gaussian mode (TEM00) is often used for thin plate cutting (on the order of 3 mm or less), while a low-order multi-mode (TEM01) is more suited for thick plate cutting. The low-order multi-mode is focused to form a sharp-edged circular spot, offering a wider kerf (cut width) necessary for intricate thick plate cutting. Donut-shaped modes (TEM01) have also been implemented with femtosecond sources to suppress the conicity (cone-like section) due to ablation saturation by precession focused spots of intensity around its donut-like structure at high rotation rates [Citation175]. Comparisons between radially and circularly polarized beams in terms of cutting depth and speed have been studied extensively, showing a two-fold increase in efficiency [Citation176].
Beam-shaping capabilities for fiber-laser systems, in the domain of laser cutting and drilling, have also been implemented. For example, in the case of the nLight CoronaTM system, which offers rapid tunability of beam shapes, including flat-top and donut beams, where the high edge quality of the beams reduces the ‘roughness’ of cuts and subsequently the need for costly and time-consuming post-processing steps. Picosecond pulsed, radial, and azimuthally polarized laser beams have been used in the microdrilling of austenitic steel. Results of the transverse sections and the inlets and outlets of the microholes in 1 mm CrNi steel, when drilled with radially (left) and azimuthally (right) polarized radiation, are depicted in [Citation156]. In the case of radial polarization, radial widening, and barrelling of the capillaries were observed, while slender capillaries with straight walls were obtained with azimuthal polarization. An enhanced absorption in the upper part of the capillary for radial polarization is evident, whereas azimuthal polarization allows for a more efficient energy transport into the tip of the hole, resulting in narrower and deeper holes [Citation156].
Figure 16. SEM images of transverse sections, inlets and outlets of microholes in 1 mm CrNi steel drilled with radially (left) and azimuthally (right) polarized radiation. Adapted with permission from Ref. [Citation156].
![Figure 16. SEM images of transverse sections, inlets and outlets of microholes in 1 mm CrNi steel drilled with radially (left) and azimuthally (right) polarized radiation. Adapted with permission from Ref. [Citation156].](/cms/asset/ac8c58a7-131e-4c26-b6e4-c853758a5b76/tapx_a_2327453_f0016_oc.jpg)
5.1.2. Ablation and welding
In the case of ablation, beams carrying OAM have shown to produce smoother processed surfaces as opposed to modified Gaussian beams containing a central hole [Citation177]. This is due to the OAM transfer (via laser-induced plasma) causing the molten material to ‘spiral,’ resulting in smoother edges and deeper processing depths. Comparative results between ablation from an optical vortex (possessing OAM) and a non-vortex annular beam are contained in Ref [Citation177]. Recently, q-plates have been used extensively to produce both pure OAM beams and vector – vortex beams for use in laser ablation of metals and semiconductors. Already with non-OAM carrying light, ripples are observed in the ablated material depending on the fluence and number of laser shots. In the case of vector-vortex beams, ablation patterns display orthogonal ripples on the outer and inner parts of the ring, where the laser fluence is low. While ‘grooves’ (larger, deeper structures), aligned parallel to the local beam polarization appear in the annular, high-fluence region [Citation155]. Dynamic surface processing with radial and azimuthal polarization beams has shown to produce large areas of complex biomimetic structures, as displayed in [Citation154]. contains SEM images of Ni surfaces when irradiated with a femtosecond source possessing azimuthal, radial, or linear polarization. These bio-inspired, multi-directional, periodic structures (mimicking the morphology of a shark’s skin) for super-hydrophobic micro/nano structures will have a plethora of useful applications. While non-OAM carrying beams, such as rectangular beams (having uniform intensity profiles) produced by a diffractive optical kinoform have shown to improve the hardening of AISI 1050 steel. This is due to the steep intensity profile holding the heated area in the austenitization temperature range longer producing a more uniform martensitic transformation [Citation178]. Other examples have shown hybrid SLM holograms consisting of binary gratings and geometric masks to create arbitrary intensity profiles (such as square, ring, triangle, pentagon, and hexagon) for laser ablation on polished stainless steel [Citation179].
Figure 17. SEM images of the structures formed on Ni surfaces upon irradiation with azimuthal (left) and radial (right) polarized vector vortex beams. Adapted with permission from Ref. [Citation154].
![Figure 17. SEM images of the structures formed on Ni surfaces upon irradiation with azimuthal (left) and radial (right) polarized vector vortex beams. Adapted with permission from Ref. [Citation154].](/cms/asset/494eb190-2f8b-4884-a30b-c63ad256cbda/tapx_a_2327453_f0017_oc.jpg)
5.1.3. Micro- and nano-fabrication
Since vectorial beams possess a non-vanishing longitudinal electric field component in tightly focusing systems, they produce an extremely sharp focus (well below the diffraction limit), making them highly useful in realising micro- and nano-fabrication. For example, azimuthally polarized light has produced smaller diameter boreholes, sharper tips, faster drilling rates, and higher aspect ratios (as shown in [Citation156]). In comparative studies between linearly and radially polarized beams, it was shown that a radially polarized beam increases machining efficiency by 40–100 and reduces the focused spot size by more than 30
[Citation180]. Radially polarized beams have been implemented to enhance the feature size, efficiency, cross-sectional profile, and roughness of microvias, which are the interconnects between substrates, particularly used in printed circuit boards [Citation181]. Closed-loop adaptive beam shaping, via the use of a DMD, has been shown (in real-time) to compensate for the local surface gradient of a machined sample when micro-patterning over curved surfaces [Citation182]. It has also been shown that optical vortices with an annular spatial form and carrying OAM enable one to ‘twist’ melted metal, silicon, and photo-isomerized polymers to create either achiral or chiral micro-structures [Citation153]. Such structured material (in the form of microneedles, chiral nanostructures, and chiral surface reliefs) will advance biomedical micro- and electro-mechanical systems, detectors for chiral chemical composites and plasmonic metasurfaces for chiral chemical reactions. Other structured beams, such as non-diffracting Bessel and annular beams have advantages in improving micro-machining and deep-hole drilling due to their long depth of field, as well as reducing the feature size in multiphoton polymerization [Citation183].
5.1.4. Ultra-fast materials processing
Being able to combine both the temporal and spatial aspects of optical fields, in the form of spatial-structured, ultra-short pulses, allows for the processing of new materials. Here, digital-holographic beam shaping techniques are used to develop optimal beam shapes for various ultra-fast laser applications. Since Bessel-like beams can be tailored in all three spatial dimensions, high-quality, ultra-fast materials processing can be achieved [Citation157,Citation184,Citation185]. A range of intensity profiles and their longitudinal propagation profiles are shown in the bottom row of . These non-diffracting Bessel-like beams have been applied to achieve a preferred crack direction in glass and transparent materials [Citation186], as well as aberration-corrected Bessel-like beams for the implementation of laser cutting bevelled edges in glass [Citation187]. By adapting the transverse and longitudinal focus properties, single pass cleaving of glass with thicknesses of up to 10 mm have been achieved (illustrated in ). shows SEM images of cleaved glass edges demonstrating the precise and defined edge quality for all inclined edges on a glass sample [Citation157].
Figure 18. (a) SEM image of a cleaved edge of a (b) Glass. Detail of the cleaved surface and its corresponding (c) Surface-roughness measurement with (d) Corresponding transverse intensity profile. Adapted with permission from Ref. [Citation157].
![Figure 18. (a) SEM image of a cleaved edge of a (b) Glass. Detail of the cleaved surface and its corresponding (c) Surface-roughness measurement with (d) Corresponding transverse intensity profile. Adapted with permission from Ref. [Citation157].](/cms/asset/5567e966-c013-4610-b593-0e2bdd7566fc/tapx_a_2327453_f0018_oc.jpg)
5.2. Communications
Space division multiplexing (SDM) is emerging as a promising solution to increase the bandwidth of communication systems, be it for fiber [Citation188,Citation189], free-space (in the case of ground to UAVs [Citation190]), wireless, underwater [Citation191], and terrestrial [Citation192] communication. High-power OAM modes and their superpositions have been applied in testing the effect of atmospheric turbulence over a 143-km free-space link, between two islands, with a wavelength of 532 nm. Without performing any active compensation for the effects of turbulence, the orientations of the first three higher-order mode superpositions are distinct from one another with a probability of 82% [Citation151]. OAM multiplexing (a form of SDM) with a high-power ytterbium-doped fiber amplifier (YDFA) has been demonstrated for high-speed, high-capacity underwater optical communication [Citation152], the concept of which is shown in . In this work, the setup was executed by transmitting four multiplexed green OAM beams through 1.2 m of water with up to 40 Gbit/s. The effects of numerous underwater conditions (such as scattering, current and thermal gradients) on beam quality and system performance were studied, where it was shown that thermal gradients significantly degrade the beam-quality resulting in modal distortion and beam wander [Citation152]. Metalenses are also being implemented in underwater environments in order to improve binocular vision systems [Citation193], as well as in sixth-generation (6 G) communication technology [Citation194].
Figure 19. Application scenario for high-speed and high-capacity underwater optical communication with high-power OAM-based SDM. Adapted with permission from Ref. [Citation152].
![Figure 19. Application scenario for high-speed and high-capacity underwater optical communication with high-power OAM-based SDM. Adapted with permission from Ref. [Citation152].](/cms/asset/e2dc0c6c-5657-4920-85f5-3e03dc501238/tapx_a_2327453_f0019_oc.jpg)
5.3. Amplification improvement
Structured beams can also be implemented as pump beams where their spatial profile can enhance the pump/ seed mode intensity overlap for improvement of the amplification process. Some cases have involved a SLM together with a multi-terawatt optical parametric chirped-pulse amplification (OPCPA) system to improve the beam uniformity in the next-stage amplifier [Citation195]. A uniform profile of the amplified beam through the rod amplifier offers a safer energy ramp in the subsequent amplification and improved protection of the pulse compressor gratings [Citation195]. While seed beams structured as concentric vortex beams have shown to obtain a high energy extraction ratio from an amplification system [Citation196,Citation197]. Power-scaling of higher-order Laguerre-Gaussian modes using an end-pumped rod MOPA, selected using an SLM, has been demonstrated recently. The study showed that beam quality preservation and power scaling are simultaneously achievable when the seed-to-pump beam sizes are carefully controlled [Citation198], validated using a novel analytical 3D modeling approach [Citation199]. Interestingly, when a similar amplification approach is applied to pure OAM beams with topological charges , variations of the pump-to-seed beam can induce a ‘vortex splitting’ phenomenon [Citation200].
5.4. New emerging areas
The structuring of optical fields is fast paving the way for new application spaces, such as the acceleration of electrons. Although electron acceleration was proposed years ago [Citation201–203], its realization has only now seemed feasible due to structuring the polarization of optical fields [Citation204]. In this work, it is proposed and simulations are performed to demonstrate the focusability of a 90 GW radially-polarized pulse to a focal-spot size as small as , which is well-suited for particle acceleration. Here, a micro-channel provides the waveguide for the laser pulse, as well as the source for the electrons to accelerate to relativistic energies. Light-sheet fluorescence microscopy (LSFM) is also benefiting from the development of large depth-of-focus light sheets via genetic algorithm-based metalens design [Citation205], as well as motion-resolved, reference-free holographic imaging [Citation206].
6. Conclusion
This review has revealed that the toolkit both intra-cavity and external to lasers, for low power structured light creation and control is very well developed, and already deployed in many application areas, from microscopy to communication. The bulk of the advances have used OAM as a topical example, with only a few venturing beyond this form of structured light. The open challenge is to move beyond OAM and towards higher-power. There is promising progress in regards to the latter, with solutions reaching 1000s of Watts in average power and GigaWatts of peak power, with some solutions commercially available. Mode types under control vary from flat-top beams to non-diffracting beams, from scalar to vectorial, but with full control of all degrees of freedom from a single device still not possible beyond only a few Watts, e.g. using SLMs and DMDs. Due to the finite absorption limitation, the majority of the optics used in high-power kilowatt laser systems are large and bulky hindering attempts for miniature-sized components. A possibility could be to reassess well-established adaptive optics solutions, such as deformable mirrors, which can tolerate and efficiently reflect powers on the order of kilowatts. However, their response rate and stroke to achieve fast, high-purity spatial mode creation, would need to be improved. The enhancement provided by structured light in many applications will surely see the development of more robust and versatile solutions for the on-demand creation of desired complex light fields with power scaling, and perhaps in this regard a nascent field to explore is the amplification of structured modes inside and outside of fiber. The exciting promise is light tailored for any purpose.
Disclosure statement
No potential conflict of interest was reported by the author(s).
Additional information
Funding
References
- Dickey FM. Laser beam shaping. Opt Photonics News. 2003;14:30–35. doi: 10.1364/OPN.14.4.000030
- Caley AJ, Thomson MJ, Liu J, et al. Diffractive optical elements for high gain lasers with arbitrary output beam profiles. Opt Express. 2007;15:10699–10704. doi: 10.1364/OE.15.010699
- Soifer VA Methods for computer design of diffractive optical elements. Hoboken, NJ: John Wiley & Sons, Inc.; 2001.
- Turunen J, Wyrowski F, editors. Diffractive optics for industrial and commercial applications. Diffractive optics for industrial and commercial applications. Weinheim, Germany: Wiley-VCH; March1998. p. 440.
- Ma H, Liu Z, Zhou P, et al. Generation of flat-top beam with phase-only liquid crystal spatial light modulators. J Opt. 2010;12:045704. doi: 10.1088/2040-8978/12/4/045704
- Forbes A, de Oliveira M, Dennis MR. Structured light. Nat Photonics. 2021;15:253–262. doi: 10.1038/s41566-021-00780-4
- Hoffnagle JA, Jefferson CM. Design and performance of a refractive optical system that converts a gaussian to a flattop beam. Appl Optics. 2000;39:5488–5499. doi: 10.1364/AO.39.005488
- Thompson KP, Rolland JP. Freeform optical surfaces: a revolution in imaging optical design. Opt Photonics News. 2012;23:30–35. doi: 10.1364/OPN.23.6.000030
- Forbes A, Dudley A, McLaren M. Creation and detection of optical modes with spatial light modulators. Adv Opt Photonics. 2016;8:200–227. doi: 10.1364/AOP.8.000200
- Rubano A, Cardano F, Piccirillo B, et al. Q-plate technology: a progress review. JOSA B 2019;36:D70–D87.10.1364/JOSAB.36.000D70
- Escuti MJ, Kim J, Kudenov MW. Controlling light with geometric-phase holograms. Opt Photonics News. 2016;27:22–29. doi: 10.1364/OPN.27.2.000022
- Devlin RC, Ambrosio A, Rubin NA, et al. Arbitrary spin-to–orbital angular momentum conversion of light. Science. 2017;358(6365):896–901. doi: 10.1126/science.aao5392
- Rosales-Guzmán C, Forbes A How to shape light with spatial light modulators. Bellingham, WA: SPIE Press; 2017.
- Lazarev G, Chen PJ, Strauss J, et al. Beyond the display: phase-only liquid crystal on silicon devices and their applications in photonics [invited]. Opt Express. 2019;27:16206–16249. doi: 10.1364/OE.27.016206
- Scholes S, Kara R, Pinnell J, et al. Structured light with digital micromirror devices: a guide to best practice. Opt Eng. 2019;59:041202. doi: 10.1117/1.OE.59.4.041202
- Ren YX, Lu RD, Gong L Tailoring light with a digital micromirror device. Ann Phys 2015;527:447–470. 10.1002/andp.201500111
- Turtaev S, Leite IT, Mitchell KJ, et al. Comparison of nematic liquid-crystal and dmd based spatial light modulation in complex photonics. Opt Express. 2017;25:29874–29884. doi: 10.1364/OE.25.029874
- Beth RA. Mechanical detection and measurement of the angular momentum of light. Phys Rev. 1936;50:115–125. doi: 10.1103/PhysRev.50.115
- Allen L, Beijersbergen MW, Spreeuw R, et al. Orbital angular momentum of light and the transformation of Laguerre-gaussian laser modes. Phys Rev A. 1992;45:8185. doi: 10.1103/PhysRevA.45.8185
- Heckenberg N, McDuff R, Smith C, et al. Generation of optical phase singularities by computer-generated holograms. Optics Lett. 1992;17:221–223. doi: 10.1364/OL.17.000221
- Pinnell J, Rodríguez-Fajardo V, Forbes A Probing the limits of orbital angular momentum generation and detection with spatial light modulators. J Opt 2020;23:015602. 10.1088/2040-8986/abcd02
- Marrucci L, Manzo C, Paparo D Optical Spin-to-Orbital Angular Momentum Conversion in Inhomogeneous Anisotropic Media. Phys Rev Lett 2006;96:163905. 10.1103/PhysRevLett.96.163905
- Radwell N, Hawley R, Götte J, et al. Achromatic vector vortex beams from a glass cone. Nat Commun. 2016;7:1–6. doi: 10.1038/ncomms10564
- Beckley AM, Brown TG, Alonso MA. Full poincaré beams. Opt Express. 2010;18:10777–10785. doi: 10.1364/OE.18.010777
- He C, Chang J, Hu Q, et al. Complex vectorial optics through gradient index lens cascades. Nat Commun. 2019;10:1–8. doi: 10.1038/s41467-019-12286-3
- Wu HJ, Yang HR, Rosales-Guzmán C, et al. Vectorial nonlinear optics: type-ii second-harmonic generation driven by spin-orbit-coupled fields. Phys Rev A. 2019;100:053840. doi: 10.1103/PhysRevA.100.053840
- Tang Y, Li K, Zhang X, et al. Harmonic spin–orbit angular momentum cascade in nonlinear optical crystals. Nat Photonics. 2020;14:658–662. doi: 10.1038/s41566-020-0691-0
- Buono WT, Forbes A Nonlinear optics with structured light. Opto-Electronic Advances 2022;5:210174–1. 10.29026/oea.2022.210174
- Padgett MJ. Orbital angular momentum 25 years on [invited]. Opt Express. 2017;25:11265–11274. doi: 10.1364/OE.25.011265
- Ndagano B, Nape I, Cox MA, et al. Creation and detection of vector vortex modes for classical and quantum communication. J Lightwave Technol. 2018;36:292–301. doi: 10.1109/JLT.2017.2766760
- Shen Y, Wang X, Xie Z, et al. Optical vortices 30 years on: Oam manipulation from topological charge to multiple singularities. Light Sci Appl. 2019;8:1–29. doi: 10.1038/s41377-019-0194-2
- Forbes A, Nape I. Quantum mechanics with patterns of light: progress in high dimensional and multidimensional entanglement with structured light. AVS Quantum Science. 2019;1:011701. doi: 10.1116/1.5112027
- Willner AE, Pang K, Song H, et al. Orbital angular momentum of light for communications. Appl Phys Rev. 2021;8:041312. doi: 10.1063/5.0054885
- Rosales-Guzmán C, Ndagano B, Forbes A. A review of complex vector light fields and their applications. J Opt. 2018;20:123001. doi: 10.1088/2040-8986/aaeb7d
- He C, Shen Y, Forbes A. Towards higher-dimensional structured light. Light Sci Appl. 2022;11:205. doi: 10.1038/s41377-022-00897-3
- Erhard M, Fickler R, Krenn M, et al. Twisted photons: new quantum perspectives in high dimensions. Light Sci Appl 2018;7:17146–17146. 10.1038/lsa.2017.146
- Yang H, Xie Z, He H, et al. A perspective on twisted light from on-chip devices. APL Photonics. 2021;6:110901. doi: 10.1063/5.0060736
- Forbes A, Ramachandran S, Zhan Q. Photonic angular momentum: progress and perspectives. Nanophotonics. 2022;11:625–631. doi: 10.1515/nanoph-2022-0035
- Forbes A. Structured light from lasers. Laser Photonics Rev. 2019;13:1900140. doi: 10.1002/lpor.201900140
- Aadhi A, Sharma V, Samanta GK. High-power, continuous-wave, tunable mid-IR, higher-order vortex beam optical parametric oscillator. Optics Lett. 2018 may;43:2312. Available from: https://opg.optica.org/abstract.cfm?URI=ol-43-10-2312
- Miao Y, Zhang L, Dong J. Broadband petal-like Raman Laser. Ann Phys. 2022 Apr;534:2100476. Available from: https://onlinelibrary.wiley.com/doi/10.1002/andp.202100476
- Chen D, Miao Y, Wang H, et al. Vortex arrays directly generated from an efficient diode-pumped microchip laser. JPhys Photonics. 2020 Jul;2:035002. Available from: https://iopscience.iop.org/article/10.1088/2515-7647/ab8a5b
- Li J, Yao Y, Yu J, et al. Efficient vortex laser with annular pumping formed by circle Dammann Grating. IEEE Photonics Technol Lett. 2016 Feb;28:473–476. Available from: http://ieeexplore.ieee.org/document/7327160/
- Kim TH, Park JS, Kim JW, et al. Direct generation of a Laguerre-Gaussian-Mode Optical Vortex Beam in a single-frequency Nd: YVO4 unidirectional ring laser. J Korean Phys Soc. 2019 Oct;75:557–560. Available from: http://doi.org/10.3938/jkps.75.557
- Geberbauer JWT, Kerridge-Johns WR, Damzen MJ 30 W vortex LG 01 or HG 10 laser using a mode transforming output coupler. Opt Express 2021;29:29082.
- Wang M, Ma Y, Sheng Q, et al. Laguerre-gaussian beam generation via enhanced intracavity spherical aberration. Opt Express. 2021 Aug;29:27783. Available from: https://opg.optica.org/abstract.cfm?URI=oe-29-17-27783
- Qiao Z, Xie G, Wu Y, et al. Generating high-charge optical vortices directly from laser up to 288th order. Laser Photonics Rev. 2018 Aug;12:1800019. Available from: https://onlinelibrary.wiley.com/doi/10.1002/lpor.201800019
- Zhang J, Sun C, Xiong B, et al. An InP-based vortex beam emitter with monolithically integrated laser. Nat Commun. 2018 Jul;9:2652. Available from: https://www.nature.com/articles/s41467-018-05170-z
- Hayenga WE, Parto M, Ren J, et al. Direct generation of tunable orbital angular momentum beams in microring lasers with broadband exceptional points. ACS Photonics. 2019 Aug;6:1895–1901. Available from: https://pubs.acs.org/doi/10.1021/acsphotonics.9b00779
- Seghilani MS, Myara M, Sellahi M, et al. Vortex laser based on III-V semiconductor metasurface: direct generation of coherent Laguerre-gauss modes carrying controlled orbital angular momentum. Sci Rep. 2016 Dec;6:38156. Available from: https://www.nature.com/articles/srep38156
- Gottesman NS, Lukowski ML, Meyer JT, et al. Intra-cavity astigmatic mode converting VECSEL. IEEE Photonics J. 2022 Aug;14:1–6. Available from: https://ieeexplore.ieee.org/document/9808337/
- Schepers F, Bexter T, Hellwig T, et al. Selective hermite–Gaussian mode excitation in a laser cavity by external pump beam shaping. Appl Phys B. 2019 May;125:75. Available from: http://doi.org/10.1007/s00340-019-7186-z
- Tuan PH, Hsieh YH, Lai YH, et al. Characterization and generation of high-power multi-axis vortex beams by using off-axis pumped degenerate cavities with external astigmatic mode converter. Opt Express. 2018;26:20481. doi: 10.1364/OE.26.020481
- Kim DJ, Kim JW, Clarkson WA. Q-switched nd: YAG optical vortex lasers. Opt Express. 2013;21:29449. doi: 10.1364/OE.21.029449
- Yang Y, Li Y, Wang C, et al. Research on 946 nm LG 01 mode laser considering thermal effect. Laser Physics. 2022 Sep;32:095801. Available from: https://iopscience.iop.org/article/10.1088/1555-6611/ac7ec6
- Beijersbergen MW, Allen L, van der Veen HE, et al. Astigmatic laser mode converters and transfer of orbital angular momentum. Optical Angular Momentum 2016;96:135–144.
- Chard SP, Shardlow PC, Damzen MJ. High-power non-astigmatic TEM00 and vortex mode generation in a compact bounce laser design. Appl Phys B. 2009;97:275–280. doi: 10.1007/s00340-009-3642-5
- Sheng Q, Wang A, Ma Y, et al. Intracavity spherical aberration for selective generation of single-transverse-mode Laguerre-Gaussian output with order up to 95. PhotoniX. 2022 Dec;3:4. Available from: https://photonix.springeropen.com/articles/10.1186/s43074-022-00050-8
- Ngcobo S, Litvin I, Burger L, et al. A digital laser for on-demand laser modes. Nat Commun. 2013;4:1–6. Available from: http://doi.org/10.1038/ncomms3289
- Wang C, Gui L, Qiu K, et al. A metasurface-assisted fiber laser enables generation of high-power and high-purity structured beams. 2020 Asia Communications and Photonics Conference (ACP) and International Conference on Information Photonics and Optical Communications (IPOC); Beijing University; 2020. p. 1–3.
- Zhang L, Geng T, Gao X, et al. Formation of high-quality vortex laser beams with different orbital angular momenta in the laser resonator. J Opt Soc Am A. 2018 Sep;35:1599. Available from: https://opg.optica.org/abstract.cfm?URI=josaa-35-9-1599
- Mitten D, Hart M, Warner SH, et al. High output power, single mode, and tem00 operation of a multiple gain chip vecsel using a twisted-mode configuration. Opt Express. 2023 Apr;31:12680–12685. Available from: https://opg.optica.org/oe/abstract.cfm?URI=oe-31-8-12680
- Lukowski ML, Meyer JT, Hessenius C, et al. High-Power Higher Order Hermite-Gaussian and Laguerre-Gaussian Beams from vertical external cavity surface emitting lasers. IEEE J Sel Top Quantum Electron. 2019 Nov;25: 1–6. https://ieeexplore.ieee.org/document/8675371/
- Hayenga WE, Ren J, Parto M, et al. Direct generation of tunable orbital angular momentum beams in microring lasers with broadband exceptional points. ACS Photonics. 2019;6:1895–1901. arXiv preprint arXiv:190310108. doi: 10.1021/acsphotonics.9b00779
- Zhang Z, Qiao X, Midya B, et al. Tunable topological charge vortex microlaser. Science. 2020 May;368:760–763. Available from: https://www.science.org/doi/10.1126/science.aba8996
- Wei MD, Lai YS, Chang KC. Generation of a radially polarized laser beam in a single microchip Nd: YVO4 laser. Optics Lett. 2013 Jul;38:2443. Available from: https://opg.optica.org/abstract.cfm?URI=ol-38-14-2443
- Jefferson-Brain TL, Barber MJ, Coupe AD, et al. Stabilisation of transverse mode purity in a radially polarised Ho: YAG laser using machine learning. Appl Phys B. 2022 Jun;128:110. Available from: https://doi.org/10.1007/s00340-022-07816-9
- He HS, Chen Z, Dong J. Direct generation of vector vortex beams with switchable radial and azimuthal polarizations in a monolithic Nd: YAG microchip laser. Appl Phys Express. 2017 May;10:052701. Available from: https://iopscience.iop.org/article/10.7567/APEX.10.052701
- Chen D, Cai S, Dong J. Highly efficient Yb: YAG microchip laser for direct generation of radially polarized vector vortices. Eng Res Exp. 2020;2:045035. doi: 10.1088/2631-8695/abd2d6
- Chen D, Miao Y, Fu H, et al. High-order cylindrical vector beams with tunable topological charge up to 14 directly generated from a microchip laser with high beam quality and high efficiency. APL Photonics. 2019 Oct;4:106106. Available from: http://aip.scitation.org/doi/10.1063/1.5119789
- Chen X, Liu S, Lin Z, et al. Dual-cavity digital laser for intra-cavity mode shaping and polarization control. Opt Express. 2018 Jul;26:18182. Available from: https://opg.optica.org/abstract.cfm?URI=oe-26-14-18182
- Dietrich T, Rumpel M, Beirow F, et al. Thin-disk oscillator delivering radially polarized beams with up to 980 W of CW output power. Optics Lett. 2018;43:1371. doi: 10.1364/OL.43.001371
- Ahmed MA, Voss A, Vogel MM, et al. Multilayer polarizing grating mirror used for the generation of radial polarization in Yb: YAG thin-disk lasers. Optics Lett. 2007;32:3272. doi: 10.1364/OL.32.003272
- Sharma V, Kumar SC, Aadhi A, et al. Tunable vector-vortex beam optical parametric oscillator. Sci Rep. 2019;9:1–7. doi: 10.1038/s41598-019-46016-y
- Sroor H, Huang YW, Sephton B, et al. High-purity orbital angular momentum states from a visible metasurface laser. Nat Photonics. 2020 Aug;14:498–503. Available from: http://www.nature.com/articles/s41566-020-0623-z
- Wei D, Cheng Y, Ni R, et al. Generating controllable Laguerre-gaussian laser modes through intracavity spin-orbital angular momentum conversion of light. Phys Rev Appl. 2019 Jan;11:014038. Available from: https://link.aps.org/doi/10.1103/PhysRevApplied.11.014038
- Zhao Y, Fan J, Shi H, et al. Intracavity cylindrical vector beam generation from all-PM Er-doped mode-locked fiber laser. Opt Express. 2019 Mar;27:8808. Available from: https://opg.optica.org/abstract.cfm?URI=oe-27-6-8808
- Lin D, Daniel JMO, Gecevičius M, et al. Cladding-pumped ytterbium-doped fiber laser with radially polarized output. Optics Lett. 2014;39:5359. doi: 10.1364/OL.39.005359
- Niziev VG, Nesterov AV Influence of beam polarization on laser cutting efficiency. J Phys D Appl Phys 1999;32:1455–1461. 10.1088/0022-3727/32/13/304
- Li B, Hu Y, Hu Y, et al. 15 kW radially polarized beam irradiated from a FAF CO2 laser based on an intracavity triple-axicon retroreflector and quarter wave phase retarders. Appl Optics. 2017 Apr;56:3383. Available from: https://opg.optica.org/abstract.cfm?URI=ao-56-12-3383
- Endo M. Azimuthally polarized 1 kW CO_2 laser with a triple-axicon retroreflector optical resonator. Optics Lett. 2008;33:1771. doi: 10.1364/OL.33.001771
- Niz’ev VG, Yakunin VP, Turkin NG. Generation of polarisation-nonuniform modes in a high-power CO 2 laser. Quantum Electronics. 2009 Jun;39:505–514. Available from: https://iopscience.iop.org/article/10.1070/QE2009v039n06ABEH013962
- Abdou Ahmed M, Beirow F, Loescher A, et al. High-power thin-disk lasers emitting beams with axially-symmetric polarizations. Nanophotonics. 2022;11:835–846. doi: 10.1515/nanoph-2021-0606
- Yonezawa K, Kozawa Y, Sato S. Generation of a radially polarized laser beam by use of the birefringence of a c-cut Nd: YVO4 crystal. Optics Lett. 2006;31:2151. doi: 10.1364/OL.31.002151
- Thirugnanasambandam MP, Senatsky Y, Ueda K. Generation of radially and azimuthally polarized beams in Yb: YAG laser with intra-cavity lens and birefringent crystal. Opt Express. 2011;19:1905. doi: 10.1364/OE.19.001905
- Chen D, Wang X, He H, et al. Vector vortices with tunable polarization states directly generated in a microchip laser. Appl Phys Express. 2019 May;12:052012. Available from: https://iopscience.iop.org/article/10.7567/1882-0786/ab1558
- Cao F, Zhao Y, Yao C, et al. All diffractive optical element setup for creating and characterizing optical vortices with high topological charges: analytical models and numerical results. Opt Commun. 2021 Sep;495:127119. Available from: https://linkinghub.elsevier.com/retrieve/pii/S0030401821003680
- Nguyen HT, Switkowski K, Filipkowski A, et al. All-fiber nanostructured gradient optics vortex beam converter. Opt Lasers Eng. 2022 Mar;150:106841. Available from: https://linkinghub.elsevier.com/retrieve/pii/S0143816621003110
- Weber K, Hütt F, Thiele S, et al. Single mode fiber based delivery of OAM light by 3D direct laser writing. Opt Express. 2017 Aug;25:19672. Available from: https://opg.optica.org/abstract.cfm?URI=oe-25-17-19672
- Zhou Z, Li P, Ma J, et al. Generation and detection of optical vortices with multiple cascaded spiral phase plates. Photonics. 2022 May;9:354. Available from: https://www.mdpi.com/2304-6732/9/5/354
- Fickler R, Campbell G, Buchler B, et al. Quantum entanglement of angular momentum states with quantum numbers up to 10,010. Proc Natl Acad Sci USA. 2016;113:13642–13647. doi: 10.1073/pnas.1616889113
- Carbone L, Bogan C, Fulda P, et al. Generation of high-purity higher-order Laguerre-gauss beams at high laser power. Phys Rev Lett 2013;110:1–5. 10.1103/PhysRevLett.110.251101
- Kim DJ, Kim JW, Clarkson WA. High-power master-oscillator power-amplifier with optical vortex output. Appl Phys B. 2014;117:459–464. doi: 10.1007/s00340-014-5855-5
- Li R, Ren Y, Liu T, et al. Generating large topological charge Laguerre–gaussian beam based on 4K phase-only spatial light modulator. Chin Opt Lett. 2022;20:120501. doi: 10.3788/COL202220.120501
- Chen Y, Fang ZX, Ren YX, et al. Generation and characterization of a perfect vortex beam with a large topological charge through a digital micromirror device. Appl Optics. 2015 Sep;54:8030. Available from: https://opg.optica.org/abstract.cfm?URI=ao-54-27-8030
- Caño-García M, Quintana X, Otón JM, et al. Dynamic multilevel spiral phase plate generator. Sci Rep. 2018 Oct;8:15804. Available from: https://www.nature.com/articles/s41598-018-34041-2
- Li S, Mo Q, Hu X, et al. Controllable all-fiber orbital angular momentum mode converter. Optics Lett. 2015;40:4376. doi: 10.1364/OL.40.004376
- Shen Y, Meng Y, Fu X, et al. Wavelength-tunable hermite–gaussian modes and an orbital-angular-momentum-tunable vortex beam in a dual-off-axis pumped yb: calgo laser. Optics Lett. 2018;43:291–294. doi: 10.1364/OL.43.000291
- Hou T, An Y, Chang Q, et al. Deep learning of coherent laser arrays in angular domain for orbital angular momentum beams customization. IEEE J Sel Top Quantum Electron. 2022 Jul;28:1–10. Available from: https://ieeexplore.ieee.org/document/9721075/
- Xiong G, Tang A, Lan B, et al. Vortex field manipulation based on deformation mirror with continuous surface. Guangdian Gongcheng/opto-Electron Eng. 2022;49(11):1–13.
- Massari M, Ruffato G, Gintoli M, et al. Fabrication and characterization of high-quality spiral phase plates for optical applications. Appl Optics. 2015;54:4077. doi: 10.1364/AO.54.004077
- Pinnell J, Rodríguez-Fajardo V, Forbes A. Probing the limits of orbital angular momentum generation and detection with spatial light modulators. J Optics (United Kingdom). 2021 Jan;23:015602. Available from: https://iopscience.iop.org/article/10.1088/2040-8986/abcd02
- HOLOEYE Photonics AG. Thermal management systems for HOLOEYE 2023. https://holoeye.com/slm-thermal-management-systems/
- Behren B, Heinze J, Bode N, et al. High-power laser beam in higher-order hermite–gaussian modes. Appl Phys Lett 2023 05;122. 191105; Available from: http://doi.org/10.1063/5.0137085.
- Piehler S, Délen X, Rumpel M, et al. Amplification of cylindrically polarized laser beams in single crystal fiber amplifiers. Opt Express. 2013;21:11376. doi: 10.1364/OE.21.011376
- Kanazawa S, Kozawa Y, Sato S. High-power and highly efficient amplification of a radially polarized beam using an yb-doped double-clad fiber. Optics Lett. 2014;39:2857. doi: 10.1364/OL.39.002857
- Sroor H, Litvin I, Naidoo D, et al. Amplification of higher order poincaré sphere beams through nd: ylf and nd: yag crystals. Appl Phys B. 2019;125:49. doi: 10.1007/s00340-019-7158-3
- Jefferson-Brain TL, Smith CR, Burns MD, et al. Amplification of a radially polarized beam in a thermally guiding ytterbium-doped fiber rod. Appl Phys B. 2019 Sep;125:167. Available from: http://doi.org/10.1007/s00340-019-7276-y
- Li P, Zhang Y, Liu S, et al. Generation of perfect vectorial vortex beams. Optics Lett. 2016;41:2205. doi: 10.1364/OL.41.002205
- Feng L, Li Y, Wu S, et al. All-fiber generation of arbitrary cylindrical vector beams on the first-order poincaré sphere. Photonics Res. 2020 Aug;8:1268. Available from: https://opg.optica.org/abstract.cfm?URI=prj-8-8-1268
- Fickler R, Lapkiewicz R, Plick WN, et al. Quantum entanglement of high angular momenta. Science. 2012;338:640–643. 10.1126/science.1227193
- Ma Z, Zhao W, Zhao J, et al. Generation of arbitrary higher-order poincaré sphere beam from a ring fiber laser with cascaded Q-plates. Opt Laser Technol. 2022 Dec;156:108552. Available from: https://linkinghub.elsevier.com/retrieve/pii/S0030399222007022
- de Oliveira M, Piccardo M, Eslami S, et al. Radially and azimuthally pure vortex beams from phase-amplitude metasurfaces. ACS Photonics. 2023;10:290–297. doi: 10.1021/acsphotonics.2c01697
- Liu R, Kong LJ, Qi WR, et al. Compact, robust, and high-efficiency generator of vector optical fields. Optics Lett. 2019 May;44:2382. Available from: https://opg.optica.org/abstract.cfm?URI=ol-44-9-2382
- Phua PB, Lai WJ, Lim YL, et al. High power radial polarization conversion using photonic crystal segmented half-wave-plate. Optics InfoBase Conference Papers; Rochester, New York; 2008.
- Xie L, Tao R, Guo C, et al. High-power cylindrical vector beams generated from an all-fiber linearly polarized laser by metasurface extracavity conversion. Appl Optics. 2021 Aug;60:7346. Available from: https://opg.optica.org/abstract.cfm?URI=ao-60-24-7346
- Barber MJ, Shardlow PC, Smith CR, et al. Radially polarized 33 W emission from a double-pass Ho: YAG thin-slab amplifier. J Opt Soc Am B. 2022 Sep;39:2395. Available from: https://opg.optica.org/abstract.cfm?URI=josab-39-9-2395
- Denoeud A, Chopineau L, Leblanc A, et al. Interaction of ultraintense laser vortices with plasma mirrors. Phys Rev Lett. 2017 Jan;118:033902. Available from: https://link.aps.org/doi/10.1103/PhysRevLett.118.033902
- Kong F, Larocque H, Karimi E, et al. Generating few-cycle radially polarized pulses: erratum. Optica. 2019;6:531. doi: 10.1364/OPTICA.6.000531
- Chen H, Yin J, Zhang M, et al. High-power, femtosecond vortex beams generation in the visible and near-infrared region. J Lumin. 2022 Aug;248:118949. Available from: https://linkinghub.elsevier.com/retrieve/pii/S0022231322002241
- Lin D, Feng Y, Ren Z, et al. The generation of femtosecond optical vortex beams with megawatt powers directly from a fiber based mamyshev oscillator. Nanophotonics. 2022 Feb;11:847–854. Available from: https://www.degruyter.com/document/doi/10.1515/nanoph-2021-0537/html
- Lin D, Baktash N, Alam S, et al. 106 W, picosecond yb-doped fiber MOPA system with a radially polarized output beam. Optics Lett. 2018;43:4957. doi: 10.1364/OL.43.004957
- Negel JP, Loescher A, Dannecker B, et al. Thin-disk multipass amplifier for fs pulses delivering 400 W of average and 2.0 GW of peak power for linear polarization as well as 235 W and 1.2 GW for radial polarization. Appl Phys B. 2017 May;123:156. Available from: http://doi.org/10.1007/s00340-017-6739-2
- Liu Q, Zhao Y, Zhou W, et al. Vortex operation in Er: LuYAG crystal laser at 1.6 µm. Opt Mater. 2017;71:31–34. Available from: http://doi.org/10.1016/j.optmat.2016.06.034
- Xia KG, Ueda KI, Li JL. Radially polarized, actively Q-switched, and end-pumped nd: YAG laser. Appl Phys B. 2012;107:47–51. doi: 10.1007/s00340-012-4886-z
- Koyama M, Hirose T, Okida M, et al. Nanosecond vortex laser pulses with millijoule pulse energies from a yb-doped double-clad fiber power amplifier. Opt Express. 2011;19:14420. doi: 10.1364/OE.19.014420
- Tanaka Y, Furuki K, Maeda Y, et al. High-power pico-second vortex laser based on a large-mode area fiber amplifier. Optics Express. 2009;17(16):1338–1342. doi:10.1364/OE.17.014362.
- Koyama M, Hirose T, Okida M, et al. Power scaling of a picosecond vortex laser based on a stressed Yb-doped fiber amplifier. Opt Express. 2011;19:994. doi: 10.1364/OE.19.000994
- Chen X, Chang C, Lin Z, et al. High-energy nanosecond optical vortex output from Nd: YAG amplifiers. IEEE Photonics Technol Lett. 2016 Jun;28:1271–1274. Available from: http://ieeexplore.ieee.org/document/7426776/
- Xu H, Song Z, Huang X, et al. High-gain nanosecond vortex laser. J Russian Laser Res 2018;39:275–279. 10.1007/s10946-018-9718-0
- Beirow F, Eckerle M, Dannecker B, et al. Radially polarized passively mode-locked thin-disk laser oscillator emitting sub-picosecond pulses with an average output power exceeding the 100 W level. Opt Express. 2018 Feb;26:4401. Available from: https://opg.optica.org/abstract.cfm?URI=oe-26-4-4401
- Eckerle M, Dietrich T, Schaal F, et al. Novel thin-disk oscillator concept for the generation of radially polarized femtosecond laser pulses. Optics Lett. 2016;41:1680. doi: 10.1364/OL.41.001680
- Eckerle M, Beirow F, Dietrich T, et al. High-power single-stage single-crystal Yb: YAG fiber amplifier for radially polarized ultrashort laser pulses. Appl Phys B. 2017;123:1–6. doi: 10.1007/s00340-017-6720-0
- Lesparre F, Gomes JT, Délen X, et al. High-power Yb: YAG single-crystal fiber amplifiers for femtosecond lasers in cylindrical polarization. Optics Lett. 2015;40:2517. doi: 10.1364/OL.40.002517
- Beirow F, Eckerle M, Graf T, et al. Amplification of radially polarized ultra-short pulsed radiation to average output powers exceeding 250 W in a compact single-stage Yb: YAG single-crystal fiber amplifier. Appl Phys B. 2020;126. doi: 10.1007/s00340-020-07502-8
- Loescher A, Oldorf P, Peters R, et al. Efficient and high-throughput ablation of platinum using high-repetition rate radially and azimuthally polarized sub-picosecond laser pulses. Opt Express. 2021;29:19551. doi: 10.1364/OE.415855
- Loescher A, Negel JP, Graf T, et al. Radially polarized emission with 635 W of average power and 21 mJ of pulse energy generated by an ultrafast thin-disk multipass amplifier. Optics Lett. 2015 Dec;40:5758. Available from: https://opg.optica.org/abstract.cfm?URI=ol-40-24-5758
- Loescher A, Röcker C, Graf T, et al. Azimuthally polarized picosecond vector beam with 1.7 kW of average output power. Optics Lett. 2021 Jul;46:3492. Available from: https://opg.optica.org/abstract.cfm?URI=ol-46-14-3492
- Loescher A, Rocker C, Bienert F, et al. Frequency-doubled high-power optical vortex beam with sub 500 fs pulse duration. J Lightwave Technol. 2022;41:2174–2178. Available from: https://ieeexplore.ieee.org/document/9968024/
- Carbajo S, Granados E, Schimpf D, et al. Efficient generation of ultra-intense few-cycle radially polarized laser pulses. Optics Lett. 2014;39:2487. doi: 10.1364/OL.39.002487
- Carbajo S, Nanni EA, Wong LJ, et al. Direct longitudinal laser acceleration of electrons in free space. Phys Rev Accelerators Beams. 2016;19. doi: 10.1103/PhysRevAccelBeams.19.021303
- Veinhard M, Bellanger S, Daniault L, et al. Orbital angular momentum beams generation from 61 channels coherent beam combining femtosecond digital laser. Optics Lett. 2021 Jan;46:25. Available from: https://opg.optica.org/abstract.cfm?URI=ol-46-1-25
- Fang Y, Guo Z, Ge P, et al. Probing the orbital angular momentum of intense vortex pulses with strong-field ionization. Light Sci Appl. 2022;11. doi: 10.1038/s41377-022-00726-7
- Chen ZY, Hu R, Zhang S, et al. Relativistic high-order harmonic generation of spatiotemporal optical vortices. Phys Rev A. 2022;106. doi: 10.1103/PhysRevA.106.013516
- Khonina SN, Kazanskiy NL, Karpeev SV, et al. Bessel Beam: Significance and Applications—A Progressive Review. Micromach. 2020 Nov;11:997. Available from: https://www.mdpi.com/2072-666X/11/11/997
- Nissenbaum A, Armon N, Shekel E Dynamic beam lasers based on coherent beam combining. In: Jáuregui-Misas C Supradeepa V, editors Fiber lasers XIX: technology and systems; mar. SPIE; 2022. p. 16. Available from: https://www.spiedigitallibrary.org/conference-proceedings-of-spie/11981/2608218/Dynamic-beam-lasers-based-on-coherent-beam-combining/10.1117/12.2608218.full.
- Kliner DA, Farrow RL, Lugo J, et al. Advanced metal processing enabled by fiber lasers with tunable beam properties. In: Jáuregui-Misas C, and Supradeepa V, editors Fiber lasers XIX: technology and systems; Vol. 11981; mar. Bellingham, WA: SPIE; 2022. p. 18.
- Mohammadian N, Mhibik O, Segall M, et al. Versatile approach to laser beam shaping and analyzing by holographic phase masks. J Optics (United Kingdom). 2021 Nov;23:115609. Available from: https://iopscience.iop.org/article/10.1088/2040-8986/ac2787
- Civian. Civan’s dynamic beam laser - integration Guide 2023. https://www.youtube.com/watch?v=VfJ_2TmgwTI&ab_channel=CivanLasers
- Kliner DA, Farrow R, Lugo J, et al. Advanced metal processing enabled by fiber lasers with tunable beam properties. In Fiber lasers XIX: technology and systems. Vol. 11981. Bellingham, WA: SPIE; 2022. pp. 72–85.
- Krenn M, Handsteiner J, Fink M, et al. Twisted light transmission over 143 km. Proc Nat Acad Sci. 2016;113:13648–13653. doi: 10.1073/pnas.1612023113
- Ren Y, Li L, Wang Z, et al. Orbital angular momentum-based space division multiplexing for high-capacity underwater optical communications. Sci Rep. 2016;6:1–10. doi: 10.1038/srep33306
- Omatsu T, Miyamoto K, Morita R Optical vortices illumination enables the creation of chiral nanostructures. Vortex Dynamics and Optical Vortices. 2017;119. doi:10.5772/67073.
- Skoulas E, Manousaki A, Fotakis C, et al. Biomimetic surface structuring using cylindrical vector femtosecond laser beams. Sci Rep. 2017;7:1–11. doi: 10.1038/srep45114
- Jj Nivas J, He S, Rubano A, et al. Direct femtosecond laser surface structuring with optical vortex beams generated by a q-plate. Sci Rep. 2015;5:17929. doi: 10.1038/srep17929
- Kraus M, Ahmed MA, Michalowski A, et al. Microdrilling in steel using ultrashort pulsed laser beams with radial and azimuthal polarization. Opt Express. 2010;18:22305–22313. doi: 10.1364/OE.18.022305
- Flamm D, Grossmann DG, Jenne M, et al. Beam shaping for ultrafast materials processing. In Laser resonators, microresonators, and beam control XXI. Vol. 10904. Bellingham, WA: SPIE; 2019. pp. 130–146.
- Kennedy E, Byrne G, Collins D. A review of the use of high power diode lasers in surface hardening. J Mater Process Technol. 2004;155:1855–1860. doi: 10.1016/j.jmatprotec.2004.04.276
- Gillner A, Holtkamp J, Hartmann C, et al. Laser applications in microtechnology. J Mater Process Technol 2005;167:494–498. 10.1016/j.jmatprotec.2005.05.049
- Banat D, Ganguly S, Meco S, et al. Application of high power pulsed nanosecond fibre lasers in processing ultra-thin aluminium foils. Opt Lasers Eng. 2020;129:106075. doi: 10.1016/j.optlaseng.2020.106075
- Kalisky Y, Kalisky O The status of high-power lasers and their applications in the battlefield. Opt Eng 2010;49:091003–091003. 10.1117/1.3484954
- Azadgoli B, Baker RY. Laser applications in surgery. Ann translat Med. 2016;4:452–452. doi: 10.21037/atm.2016.11.51
- Desbiens L, Deladurantaye P, Cournoyer A, et al. Flexible and programmable pulse shaping mopa fiber laser platform, performances and applications. Rev Laser Eng 2013;41. 691 10.2184/lsj.41.9_691
- Kubacki F, Weitze H, Bruns P, et al. Successful diode laser material processing using application specific micro-optical beam shaping. In Semiconductor lasers and applications III. Vol. 6824. Bellingham, WA: SPIE; 2008. pp. 29–38.
- Salter PS, Booth MJ. Adaptive optics in laser processing. Light Sci Appl. 2019;8:110. doi: 10.1038/s41377-019-0215-1
- Möhl A, Kaldun S, Kunz C, et al. Tailored focal beam shaping and its application in laser material processing. J Laser Appl. 2019;31:042019. doi: 10.2351/1.5123051
- Laskin A, Laskin V Refractive beam shapers for material processing with high power single mode and multimode lasers. In: Laser resonators, microresonators, and beam control XV; Vol. 8600; Bellingham, WA: SPIE; 2013. pp. 140–150.
- Račiukaitis G, Stankevičius E, Gečys P, et al. Laser processing by using diffractive optical laser beam shaping technique. J Laser Micro/Nanoengineer 2011;6. 37–43 10.2961/jlmn.2011.01.0009
- Buske P, Völl A, Eisebitt M, et al. Advanced beam shaping for laser materials processing based on diffractive neural networks. Opt Express. 2022;30:22798–22816. doi: 10.1364/OE.459460
- Meier M, Romano V, Feurer T. Material processing with pulsed radially and azimuthally polarized laser radiation. Appl Phys A. 2007;86:329–334. doi: 10.1007/s00339-006-3784-9
- Weber R, Michalowski A, Abdou-Ahmed M, et al. Effects of radial and tangential polarization in laser material processing. Phys Procedia. 2011;12:21–30. doi: 10.1016/j.phpro.2011.03.004
- Tang Y, Li J, Zhou T, et al. Dynamic beam shaping with polarization control at the image plane for material processing. Procedia CIRP. 2018;74:581–584. doi: 10.1016/j.procir.2018.08.083
- Grabusovas A, Vosylius V, Gertus T, et al. Vector mathieu beam profile engineering for laser material processing applications. Procedia CIRP. 2018;74:598–601. doi: 10.1016/j.procir.2018.08.079
- Tabata N, Yagi S, Hishii M. Present and future of lasers for fine cutting of metal plate. J Mater Process Technol. 1996;62:309–314. doi: 10.1016/S0924-0136(96)02426-0
- Martin PE, Estival S, Dijoux M, et al. High-power femtosecond laser cutting and drilling combining beam-shaping and beam-splitting. In Laser beam shaping XVIII. Vol. 10744. Bellingham, WA: SPIE; 2018. pp. 163–173.
- Niziev V, Nesterov A. Influence of beam polarization on laser cutting efficiency. J Phys D Appl Phys. 1999;32:1455. doi: 10.1088/0022-3727/32/13/304
- Hamazaki J, Morita R, Chujo K, et al. Optical-vortex laser ablation. Opt Express. 2010;18:2144–2151. doi: 10.1364/OE.18.002144
- Leung MK, Man HC, Yu J Theoretical and experimental studies on laser transformation hardening of steel by customized beam. Int J Heat & Mass Trans 2007;50:4600–4606.10.1016/j.ijheatmasstransfer.2007.03.022
- Liu D, Wang Y, Zhai Z, et al. Dynamic laser beam shaping for material processing using hybrid holograms. Opt Laser Technol. 2018;102:68–73. doi: 10.1016/j.optlastec.2017.12.022
- Venkatakrishnan K, Tan B Generation of radially polarized beam for laser micromachining. J Laser Micro Nanoengin 2012;7:274. 10.2961/jlmn.2012.03.0008
- Venkatakrishnan K, Tan B. Interconnect microvia drilling with a radially polarized laser beam. J Micromech Microeng. 2006;16:2603. doi: 10.1088/0960-1317/16/12/013
- Heath DJ, Mackay BS, Grant-Jacob JA, et al. Closed-loop corrective beam shaping for laser processing of curved surfaces. J Micromech Microeng. 2018;28:127001. doi: 10.1088/1361-6439/aae1d5
- Duocastella M, Arnold CB. Bessel and annular beams for materials processing. Laser Photonics Rev. 2012;6:607–621. doi: 10.1002/lpor.201100031
- Flamm D, Chen K, Jenne M, et al. Generalized non-diffracting beams for ultrafast materials processing. In Frontiers in ultrafast optics: biomedical, scientific, and industrial applications XX. Vol. 11270. Bellingham, WA: SPIE; 2020. pp. 68–75.
- Flamm D, Grossmann DG, Sailer M, et al. Structured light for ultrafast laser micro-and nanoprocessing. Opt Eng 2021;60:025105–025105. 10.1117/1.OE.60.2.025105
- Jenne M, Flamm D, Chen K, et al. Facilitated glass separation by asymmetric bessel-like beams. Opt Express. 2020;28:6552–6564. doi: 10.1364/OE.387545
- Jenne M, Flamm D, Ouaj T, et al. High-quality tailored-edge cleaving using aberration-corrected bessel-like beams. Optics Lett. 2018;43:3164–3167. doi: 10.1364/OL.43.003164
- Richardson D New optical fibres for high-capacity optical communications. Philos Trans R Soc A Math Phys Eng Sci. 2016;374:20140441.10.1098/rsta.2014.0441
- Wang Y, Lu Y, Bao C, et al. Hollow ring-core photonic crystal fiber with 500 oam modes over 360-nm communications bandwidth. IEEE Access 2021;9:66999–67005.
- Li L, Zhang R, Zhao Z, et al. High-capacity free-space optical communications between a ground transmitter and a ground receiver via a uav using multiplexing of multiple orbital-angular-momentum beams. Sci Rep. 2017;7:1–12. doi: 10.1038/s41598-017-17580-y
- Zhu S, Chen X, Liu X, et al. Recent progress in and perspectives of underwater wireless optical communication. Prog Quantum Electron. 2020;73:100274. doi: 10.1016/j.pquantelec.2020.100274
- Kaushal H, Kaddoum G Optical communication in space: Challenges and mitigation techniques. IEEE Commun Surv Tutorials 2016;19:57–96. 10.1109/COMST.2016.2603518
- Liu X, Chen MK, Chu CH, et al. Underwater binocular meta-lens. ACS Photonics. 2023;10:2382–2389. doi: 10.1021/acsphotonics.2c01667
- Zhang JC, Wu GB, Chen MK, et al. A 6g meta-device for 3d varifocal. Sci Adv 2023;9:eadf8478. doi: 10.1126/sciadv.adf8478
- Bahk SW, Begishev I, Zuegel J. Precompensation of gain nonuniformity in a nd: glass amplifier using a programmable beam-shaping system. Opt Commun. 2014;333:45–52. doi: 10.1016/j.optcom.2014.07.054
- Li Y, Li W, Zhang Z, et al. Concentric vortex beam amplification: experiment and simulation. Opt Express. 2016;24:1658–1667. doi: 10.1364/OE.24.001658
- Li Y, Zhang Z, Miller JK, et al. Ho: yag rod amplifier for vortex beams. 2015 IEEE Photonics Conference (IPC); Virginia, USA; IEEE; 2015. p. 321–322.
- Harrison J, Forbes A, Naidoo D. Amplification of higher-order Laguerre-gaussian modes using a dual-pass mopa system. Opt Express. 2023 May;31:17408–17423. Available from: https://opg.optica.org/oe/abstract.cfm?URI=oe-31-11-17408
- Harrison J, Forbes A, Naidoo D. Improving performance prediction of diode end-pumped solid-state nd: yag rod amplifiers by incorporating pump mode evolution. Opt Express. 2023 Jul;31:24516–24536. Available from: https://opg.optica.org/oe/abstract.cfm?URI=oe-31-15-24516
- Harrison J, Buono WT, Forbes A, et al. Aberration-induced vortex splitting in amplified orbital angular momentum beams. Opt Express. 2023 May;31:17593–17608. Available from: https://opg.optica.org/oe/abstract.cfm?URI=oe-31-11-17593
- Durfee Iii C, Milchberg H Light pipe for high intensity laser pulses. Phys Rev Lett 1993;71:2409. 10.1103/PhysRevLett.71.2409
- Butler A, Spence D, Hooker SM Guiding of high-intensity laser pulses with a hydrogen-filled capillary discharge waveguide. Phys Rev Lett 2002;89:185003. 10.1103/PhysRevLett.89.185003
- Rowlands-Rees T, Kamperidis C, Kneip S, et al. Laser-driven acceleration of electrons in a partially ionized plasma channel. Phys Rev Lett 2008;100:105005. 10.1103/PhysRevLett.100.105005
- Wen M, Salamin YI, Keitel CH. Electron acceleration by a radially-polarized laser pulse in a plasma micro-channel. Opt Express. 2019;27:557–566. doi: 10.1364/OE.27.000557
- Fan Y, Chen MK, Qiu M, et al. Experimental demonstration of genetic algorithm based metalens design for generating side-lobe-suppressed, large depth-of-focus light sheet. Laser Photonics Rev. 2022;16:2100425. doi: 10.1002/lpor.202100425
- Gao Y, Cao L. Motion-resolved, reference-free holographic imaging via spatiotemporally regularized inversion. Optica. 2024;11:32–41. doi: 10.1364/OPTICA.506572