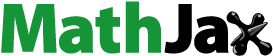
ABSTRACT
Cross-laminated timber (CLT) is a sustainable engineered wood product which is utilised in modern multi-storey timber buildings. The fire behaviour of timber structures is often a concern due to their combustible nature. In this paper, experimental fire testing of CLT panels made of Irish spruce was performed. This series of tests consisted of four vertically loaded CLT wall panels which were tested under Standard fire curves in the Structural Laboratory of Munster Technological University, Cork (MTU). To improve the fire performance of CLT panels, different types of protective claddings were used. The effectiveness of each system of protection has been stated particularly in terms of the delay in the start of charring of the CLT panels. The location of joints in the protective cladding was also analysed and was found to be a key factor in the fall-off time of the protective claddings. The results show that protective claddings made with Fireline gypsum plasterboard and a combination of plywood and Fireline gypsum plasterboard delayed the charring of CLT panels by as much as 30 and 44 min respectively. This paper analyses the detailed results of experimental fire testing and measures the charring rate and temperature distribution across the panels.
Introduction
Cross-laminated timber (CLT) is a multi-layer engineered timber product that has recently gained popularity as a structural member including for load-bearing floor and wall panels. CLT consists of an odd number of layers with a minimum of 3 layers that are bonded together using adhesives. In cross-laminated timber, the adjacent layers are oriented orthogonally, which provides structural stability in both directions, as shown in . CLT was developed with the idea to decrease the effect of the anisotropic behaviour of timber as well as reduce the influence of knots compared to solid timber (Mestek & Kreuzinger, Citation2008).
Figure 1. A three-layer CLT panel (Sandoli et al., Citation2021).

Thermal degradation occurs in timber when exposed to higher temperatures and thus evolving pyrolysis gases and chars (Bartlett et al., Citation2015). The formation of char is usually considered to happen when the temperature of timber reaches 300°C, with pyrolysis starting at around 200°C (Buchanan & Abu, Citation2017). The rate of pyrolysis reactions controls the rate of formation of char and the different factors which affect the char formation include density (Friquin, Citation2011; Yang et al., Citation2009), grain direction (Friquin, Citation2011), moisture content (Lau et al., Citation1999), and species (Lau et al., Citation1999) etc.
Due to the combustible nature of timber, the study of the fire performance of CLT at elevated temperatures is required to design safe, cost-effective, and resilient timber buildings. EN 1995-1-2:2004 (European Committee for Standardisation [CEN], Citation2004) specifically focuses on the design of timber structures in fire and provides a one-dimensional design char rate of 0.65 mm/min for softwood with a density of more than 290 kg/m3. However, the behaviour of CLT under fire can be different from solid timber and has not been provided for in the current version of the Eurocodes. Both small and large-scale experimental tests (Bartlett et al., Citation2015; Dârmon & Lalu, Citation2019; Frangi et al., Citation2008, Citation2009; Miyamoto et al., Citation2021; Schmid et al., Citation2010) were performed in recent years to study the behaviour of CLT specimens in a fire. A short description and the key conclusions of these fire tests on CLT panels are provided here from research by Frangi et al. (Citation2008). Who conducted fire tests on CLT panels to understand the behaviour and comparisons were made with solid timber panels (Frangi et al., Citation2008). The charring rate of homogenous timber panels was found to be less than the 3-layered CLT timber panels. Moreover, thin-layered CLT panels showed a higher charring rate compared to thicker-layered CLT panels, which confirms that the thickness and number of layers affect the fire behaviour and the char rate. In some cases, excessive charring can result in layers of CLT falling off which may have adverse effects. This is typically referred to as “fall off” and can contribute to the fire load and also expose new timber to a fire. In Frangi et al. (Citation2008), no fall-off of charred layers was observed in the fire tests on CLT wall panels and this showed good agreement with the one-dimensional charring rate for solid timber panels as well as showing better fire behaviour than floor panels.
Many authors have examined the behaviour of different adhesives under fire loading (Frangi et al., Citation2009; Miyamoto et al., Citation2021; Muszyński et al., Citation2019; Wiesner et al., Citation2022). Experimental testing was conducted by Frangi et al. (Citation2009). To understand the performance of adhesive as well as the effect of thickness and number of layers on the fire resistance under the standard fire curve. CLT panels were made using melamine-urea-formaldehyde (MUF), and different types of polyurethane adhesive (PUR) with different layer sizes and having an overall thickness of 60 mm. It was found that the adhesive used in the glue line between the panels affects the fire performance of CLT panels. CLT panels made with MUF adhesives show a uniform charring rate which was less than the one-dimensional charring rate as defined in EN1995-1-2 for solid timber. All PUR-glued CLT panels showed a char rate of 0.85 mm/min and 1 mm/min for 3 and 5-layered CLT panels respectively, which showed that the thickness and number of layers influence the charring rate of the panel (Frangi et al., Citation2009). Wiesner et al. (Citation2022). Studied the thermomechanical behaviour of CLT wall panels and concluded that global instability can be the main failure mode in a fire.
In addition to the above, the literature also shows experimental studies on CLT panels with a focus made on the charring rate (Kippel et al., Citation2014; Wiesner et al., Citation2017), load-bearing capacity under fire (Wiesner et al., Citation2019; Östman et al., Citation2010) and fire resistance (Kippel et al., Citation2014; Suzuki et al., Citation2016). The charring rate of CLT samples was investigated under different heating conditions and it was found that the charring rates are dependent on the test set-up, rate of heating, orientation, and size of the sample (Bartlett et al., Citation2015). Furthermore, it was found that the charring rate fell to a lower, quasi-steady value after it reached a peak value at the beginning which occurred in the first half-hour of the test (Bartlett et al., Citation2015).
A new charring model was developed specifically for CLT by Klippel and Schmid (Suzuki et al., Citation2016) based on numerous fire tests and compared to the European model in EN 1995-1-2. The results showed that when measuring the charring depth, the developed model which incorporates the structure of CLT gives conservative results.
A series of tests were performed by Fragiacomo et al. (Citation2012). On CLT floor panels exposed to fire. The 5-layer loaded CLT floor panels were tested under standard fire conditions to study their fire behaviour. The authors performed tests on both initially protected and unprotected panels. In addition, finite element (FE) modelling was performed to measure the numerical analysis and a comparison with the experimental results was made. It was observed that the charring rate for the panels directly exposed to fire showed a similar value to the one-dimensional char rate of 1995-1-2:2004 (CEN, Citation2004). Furthermore, both experimental and numerical models show similar results with reference to the resistance in fire and temperature distribution when modelled using Eurocode 5 for the temperature-dependent density, conductivity, and specific heat of the CLT panels.
Studies (Harte et al., Citation2014; O’Ceallaigh et al., Citation2018) have been performed recently on Irish Sitka spruce to determine its potential use in construction applications including CLT. The research performed shows that the CLT products made with Irish Sitka spruce C16 timber gave a higher bending strength than expected for CLT panels with the same grade, and hence suitable for structural use (O’Ceallaigh et al., Citation2018).
While it is clear there has been significant research in this field in recent years, there is limited information available on the fire analysis of CLT panels manufactured from C16 grade material. The main aim of this research is to perform fire testing on Irish-grown C16 grade Sitka Spruce CLT wall panels. This research also focuses on the use of different types of cladding to protect the CLT panels from direct exposure to fire. The performance of different types of cladding is measured based on the time delay of charring of the CLT panel for each type of protection. The research also focuses on the significance of joints in protective cladding, as they can be a significant factor in the fall-off of the cladding during a fire.
Test methodology
Materials
Boards of C16 Irish Sitka spruce with individual plank sizes of 150 mm wide and 40 mm thick were used to produce wall panels that were 1200 mm long and 900 mm high with an overall thickness of 120 mm. The boards used in the preparation of CLT wall panels were first stored in a conditioning room at room temperature with a relative humidity of 65 ± 5% for 8 weeks before the preparation of the specimens. One-component PUR adhesive (PURBOND HB S309) was used with a spread rate of 160 g/m2 in the preparation of the CLT panels. The panels were pressed with a pressure of 600 kN/m2 using steel plates for 2 hours as per EN 16351 (European Committee for Standardisation [CEN], Citation2015). Before experimental testing, the manufactured CLT panels were placed in a conditioning room for at least 4 weeks.
Test specimens
Overall, four three-layered CLT wall panels made of Irish Spruce boards were tested, each having a moisture content of 12–13% measured before the test using a pin-type moisture meter. The details of the tested wall panels are provided in .
Table 1. Tested CLT wall panels.
One CLT wall panel was directly exposed to fire on one side while the rest of the three panels were protected on the fire-exposed side with claddings having different joint distributions. In Specimen W-15FP the exposed side of the CLT panel was protected with a 15 mm Fireline gypsum plasterboard that had no joint. Similarly, specimen W-15FPJ was protected with a 15 mm Fireline gypsum plasterboard but with joints along the perimeter of the panel that is exposed to fire as well as a vertical joint at the centre of the plasterboard panel. In specimen W-12.5FP25PW, the CLT panel was protected with a 12.5 mm Fireline plasterboard which was then protected with 25 mm of plywood. Joints were provided in both the gypsum plasterboard as well as in the plywood. Vertical and horizontal joints were placed at the centre of the gypsum plasterboard and plywood respectively. Joints were also placed at 50 mm and 30 mm inside the furnace perimeter in the gypsum plasterboard and plywood respectively as shown in . The main idea of these joints was to mimic the protective claddings falling off during a fire. These different types of CLT panels are illustrated in . In all the test panels, the surface that is directly exposed to fire is 1000 mm wide x 600 mm high as represented in .
Figure 2. Location of joints in the protective claddings of W-12.5FP25PW panel, (a) Joints location in Fireline gypsum plasterboard, placed between CLT panel and plywood, (b) Location of joints in the outer plywood layer.

Figure 3. (a) Unprotected CLT panel (W-1), (b) CLT panel protected with 15 mm Fireline gypsum plasterboard without joints (W-15FP).

Test setup
Each wall panel was placed up against the front of the fire testing furnace, with the furnace door swung to one side. The CLT test panel was held up against the furnace with a steel frame on the unexposed side of the panel as shown in . Timber packing pieces were placed between the frame and the CLT panel at the top and the bottom of the panel. The load cell on the top of the panel recorded the applied constant vertical load of 85 kN throughout the test. The load was applied using a steel spreader beam along the top of the panel. A deflection gauge at the mid-span of the panel was placed to measure the horizontal displacement throughout the testing. The furnace was also equipped with a propane gas burner which was manually controlled to ensure the ISO 834 standard fire curve (Citation1999) was followed for heating conditions in the furnace. In all of these panels, fasteners with a length of 60 mm were used for the panel protected with one layer of cladding. In the panel, which was protected with two layers of protective claddings, the length of the fasteners used were 60 mm and 70 mm for the inner and outer claddings respectively. In all of these tests, the fasteners were embedded in the CLT wall panel by approximately 45 mm. The spacings between the fasteners were kept at 180 mm for the fasteners on the perimeter of the cladding, while the internal spacings were kept at 250 mm.
Location of thermocouples
A plate thermometer was used to record the temperature in the furnace during the test. Type K thermocouples were inserted at different depths to record the internal temperature distribution and the progression of the char line during fire tests. Thermocouples were placed between each layer of protective claddings and the CLT panel. Thermocouples between the protective claddings and the CLT panel were inserted from the side to remain in the same isotherm. Within the CLT panel itself, the thermocouples were inserted from the unexposed side of the panels at different depths in drilled holes, each 2 mm in diameter. Three sets of K-type thermocouples (TC) were placed at similar depths but at different locations to ascertain if significant variation in temperature occurred depending on the TC locations throughout the test. The details of the thermocouple tip locations and their depths from the exposed surface are shown in .
Table 2. Positioning of thermocouples.
Experimental results and discussions
The experimental results are explained in the following sections in terms of the charring rates of different panels, fire resistance, the effect of protective cladding on charring as well as the effect of joint location in protective cladding on fire performance.
Charring rate
The charring rate is the charred depth divided by the time in which the temperature at a certain depth reaches 300°C. The charring rate and charred depth were measured in two ways. The first method was to remove the panel from the furnace after the test has finished, cool down the specimen with water and then measure the charred depth. Using this method, the overall charring rate is calculated after the tests were finished based on the measured charred depth and the total fire time. The second technique was the installation of thermocouples at the mid-depth of each layer and in the joints between layers. The charring of the panel at a specific depth was considered to have commenced when the temperature of the thermocouples reached 300°C. The temperature recorded by TCs installed at various depths of the CLT panels was used to calculate the charred depth over time which in turn gives the charring rate. The charring rates measured by the Set-1 TC were used for the comparison with the actual charring rates as the maximum char depth was measured at the location close to Set-1 TCs.
Unprotected CLT panel (W-1)
The furnace temperatures recorded by the plate thermometer and the temperatures measured by different K-thermocouples installed at various depths are shown in . As can be seen in this , the different sets of K-thermocouples installed at the same depth but at different locations in the CLT panels gives similar temperature distribution throughout the test. Also, the furnace temperature closely followed the Standard ISO 834 fire curve throughout the test.
The charring rates of the W-1 panel at different depths are shown in . The charring rate of the unprotected wall panel was in the range of 0.63–0.66 mm/min which is very close to the one-dimensional charring rate tabulated in Eurocode 5 (Bartlett et al., Citation2015). It can be observed that in an unprotected CLT wall panel (W-1), the charring rate for the first 20 mm thickness of the CLT panel from the exposed face to fire was 0.63 mm/min. However, the charring rate for the next 20 mm of the CLT panel (until reaching the interface between layers 1 & 2) was reduced to 0.48 mm/min. The decrease in the charring rate could be due to the protective char layer preventing the heating up of the panel directly beneath it. In layer-2 of the W-1 panel, the charring rate increased significantly to 0.93 mm/min, due to the fact that some of the char of layer-1 has fallen-off when the interface temperature between the two layers reached the char temperature (300°C). Furthermore, the gaps between the individual lamellae increase causing the heat to move through the opening of the lamellae of the panel. There was no delamination or fall-off of the entire layer of the CLT panel during the test as shown in . The charred depth of 91 mm was measured after the fire test time of 137.5 mins which gives an overall charring rate of 0.66 mm/min.
Protected CLT panels
The application of protective cladding delayed the charring of the CLT panels and reduced the overall charring rate. CLT panels which were protected by different claddings, the charring rate of the panels were calculated when the temperature at the face of the CLT panel behind the cladding reached 300°C.
W-15FP
The furnace temperatures and the temperatures measured at various depths within the CLT panel, protected with a 15 mm Fireline gypsum plasterboard with no joints in it, are shown in . The different sets of K-thermocouples installed at the same depth but at different locations in the CLT panels give similar temperature distribution throughout the test. The furnace temperature closely followed the Standard ISO 834 fire curve throughout the test.
The charring rate calculated from TCs data and from the residual uncharred cross-section of the CLT panels is illustrated in . In W-15FP, the charring rate for the first 20 mm of the CLT panel was low at 0.33 mm/min as shown in . The charring rate remained constant throughout the depth of layer 1 (40 mm depth) at 0.33 mm/min. However, the charring rate slightly increased to 0.39 mm/min in the first 20 mm of layer-2 at 60 mm depth from the face of the panel near to the fire. From , it can be seen that the slope of charred depth vs time curve is almost constant throughout the test due to the fact that the cladding of 15 mm Fireline gypsum plasterboard remained intact on the panel. The charred depth of 80 mm was measured after the fire test time of 210 mins which gives a charring rate of 0.45 mm/min. During the test, the protective cladding at the fire-exposed face of the CLT panel remained intact on the CLT panel and thus no delamination of the CLT panel could occur.
W-15FPJ
The furnace temperatures and the temperatures measured at various depths within the CLT panel, which was protected with a 15 mm Fireline gypsum plasterboard with joints in it, are shown in . The furnace temperature closely followed the Standard ISO 834 fire curve throughout the test.
Figure 13. Temperature-time curves of test specimen W-15FPJ at different depths based on TC locations.

In W-15FPJ, the charring rate for the first 20 mm of the CLT panel was quite low at 0.26 mm/min as shown in . However, the charring rate for the next 20 mm of the W-15FPJ increased substantially to 0.65 mm/min which could be due to the opening of the vertical joint in the cladding causing the heat to be directed into the CLT panel at that location. This can also be confirmed by the different sets of TCs installed at the same depths but at different locations as illustrated in . The charring rates measured by Set-2 and Set-3 thermocouples remained almost constant throughout the test. The charred depth of 80 mm was measured after the fire test time of 200 mins which gives a charring rate of 0.46 mm/min as shown in . The protective cladding of the Fireline gypsum plasterboard remained intact on the CLT panel throughout the test. This kept the charring of the CLT panel low as fall off of CLT layers did not occur.
W-12.5FP25PW
The furnace temperatures and the temperatures measured at various depths within the CLT panel, protected with a 12.5 mm Fireline gypsum plasterboard and a 25 mm plywood layer with joints in both, are shown in . A slight reduction in the furnace temperature at 20–40 mins of the test was observed but overall, the furnace temperature closely followed the Standard ISO 834 fire curve throughout the test.
Figure 16. Temperature-time curves of test specimen W-15FPJ at different depths based on TC locations.

In this wall panel W-12.5FP25PW, the initial charring rate for the first 20 mm of the panel was 0.45 mm/min which then slightly increase to 0.47 mm/min up to the 40 mm depth of the panel as shown in . In CLT layer 2 of W-12.5FP25PW, the charring rate increased significantly to 1.03 mm/min which might be due to the fall-off of a charred layer 1 which exposed the fresh timber of layer 2 directly to the fire.
In W-12.5FP25PW, the slope of the charred depth and time curve is almost constant for the first 40 mm depth of the CLT panel. However, the slope of the charring depth time curve increased significantly after 40 mm of the CLT panel was charred. This is because the cladding which was intact with the CLT panel for more than 120 mins of the test then fell off which directly exposed the CLT panel to the fire. Furthermore, after the removal of the CLT panel from the furnace, it was observed that the fire-exposed layer of the CLT panel was delaminated during the test as shown in . The charred depth of 75 mm was measured after the fire test time of 156 mins which gives a charring rate of 0.67 mm/min.
Actual char-depth and its comparison with thermocouples data
In , the time to reach the final char depth based on the actual measurements after the test, and the charring rates calculated between the locations of the thermocouples, are both given. The final char depths of the panels are measurements at the location of maximum char after the fire tests were finished. The actual charring rate was calculated by taking the ratio of the maximum charred depth and total fire time of the test. For specimen W-1, the charred rate calculated using Set-1 TC is 0.63 mm/min while the charring rate calculated after measuring the final charring depth was 0.66 mm/min. For specimens W-15FP, W-15FPJ, and W-12.5FP25P, the charring rates calculated from the installed thermocouples (Set-1 TC) were 0.35, 0.37, and 0.56 mm/min respectively. The charring rate calculated after the actual measurement of charred depth for these specimens was 0.44, 0.46 and 0.67 mm/min. The actual charred depth is slightly higher than the charring depth calculated from the data recorded by thermocouples due to the following reasons:
The removal of the panel from the furnace and cooling it down takes approximately 12-15 minutes, which would give an additional charred depth.
The actual charred depth calculation was considered at the location with the maximum char and not an average charred depth at different points.
The insertion of thermocouples from the unexposed face of the CLT panel may also influence the low charred depth, as studied in the literature (Pope et al., Citation2021).
Fire resistance
The criteria for stopping the test were based on any of the following conditions occurring:
Once integrity failure had occurred, i.e. if flames break through the external surface of the CLT panel (i.e. the side not directly exposed to the flames inside the furnace).
When structural failure has occurred. The wall panel is unable to sustain the applied load at which point it will have failed structurally.
If a cotton pad is ignited on the cool side of the panel (In accordance with IS EN 13501-1).
If there is an integrity failure allow a gap gauge to be inserted into the sample from the cold side (In accordance with IS EN 13501-1).
All the fire tests were stopped due to the integrity failure in which the fire from the edges of the panel broke through except for the W-1 panel. An excessive deflection was observed in the W-1 panel as soon as the third layer of the panel started charring. The rest of the test panels which were protected with different cladding configurations were stopped before any structural failure could occur in which the panels are unable to sustain the load. The horizontal mid-height deflection on the un-exposed face of the panel to fire was recorded and is shown in . In wall panel W-1, which was directly exposed to fire, the deflection increased at the beginning and was followed by a plateau and then a more abrupt increase in the deflection. The initial increase in deflection was due to the charring of the fire-exposed outer load-bearing layer. The plateau shows the charring of the inner weak layer (perpendicular to the grain) that is followed by an increase in deflection due to the charring of the third vertically load-bearing layer. In the panels which were initially protected by cladding, the deflection time curves are similar to the un-protected panel except that the plateau was observed earlier due to the protection provided by the cladding on the exposed side to fire.
Effect of protective cladding on delay in charring
The delay in charring of CLT panels provided by different protective cladding configurations is given in . The protective cladding in W-15FP delayed the charring of the CLT panel by 30 mins when it was protected by a 15 mm Fireline gypsum plasterboard (Type F) with no joints. While, in panel W-15FPJ, the charring of the CLT panel was delayed by 25 mins, when it was protected with a 15 mm Fireline gypsum plasterboard with joints. EN 1995-1-2 provides the formulae as given in Equation 1 to measure the start of charring of the CLT panel behind the gypsum type A or F plasterboards at internal locations or the perimeter adjacent to filled joints. The delay in charring of the CLT panels (tch) calculated using Equation 1 for type F plasterboards with 15 mm thickness is 28 mins, which is very close to the experimental values of 25 mins and 30 mins as calculated in this research for 15 mm thick Type F plasterboard.
Where hp is the thickness of the protective cladding.
The overall delay in charring of the CLT panel was 44 min in W-12.5FP25PW, which was protected with a 12.5 mm Fireline gypsum plasterboard and a 25 mm plywood layer. The temperature behind the plywood reached 300°C in 28 minutes, which shows that the 25 mm plywood layer can significantly contribute to the delay of charring of the CLT panel. The charring rate of the plywood layer was found to be 0.89 mm/min which compares well to the one-dimensional charring rate of 1 mm/min provided by EN 1995-1-2 for the plywood panel having a thickness of 20 mm or more with a characteristic density of 450 kg/m3. However, it is important to note that the system presented above, inclusive of plywood and plasterboard, influences this result, and further testing is required to verify the delay in charring that could be achieved with plywood as the sole protective layer of load-bearing elements.
Comparison of different sets of thermocouples data
The three sets of thermocouples, which were drilled into the CLT panels at a similar depth from the exposed face to the fire but at different locations are compared in terms of the time to reach the charring temperature and are shown in . Overall, all three sets of thermocouples gave similar times to reach the char temperature ranging from 2 mins to 15 mins except for the 40 mm readings of W-15FPJ where the difference between the time to reach the char temperature was up to 26 mins. In the W-15FPJ panel, the vertical joint in the Fireline gypsum plasterboard appears to open as can be seen in . This caused the heat to pass through the joint directly and in turn, the temperature of the set-1 thermocouple (placed at a depth of 40 mm from the face of the CLT panel near the fire) increases more rapidly. On the other side, the two thermocouples at 40 mm from set-2 and set-3 were protected from direct exposure to fire as the plasterboard was in contact with the panel throughout the test.
Effect of joint location on fire performance
The effect of the joint location of the protective cladding on the fire performance of the CLT panel was studied. The W-15FP panel, which had no joint in the plasterboard remained in contact with the CLT panel throughout the test. The temperature measured and recorded by the thermocouple placed between the CLT panel and the plasterboard showed a lower temperature than the furnace temperature throughout the test. Thus the direct contact between the plasterboard and the CLT panel gave extra protection to the panel and consequently resulted in the lower char depth. Similarly, in Specimen W-15FPJ the measured temperature behind the protective cladding was lower than the furnace temperature throughout the test as shown in resulting in a lower char depth. In W-12.5FP25PW, which was protected with 25 mm plywood and 12.5 mm Fireline gypsum plasterboard, both with joints, resulted in the fall off of the cladding during the test. Due to the fall off of the cladding, the temperature recorded by a thermocouple placed between the plasterboard and CLT panel reached the furnace temperature resulting in a higher charring rate due to the direct exposure of the CLT panel to fire. The temperature-time curves recorded by the thermocouples which are installed between the CLT panel and the Fireline gypsum plasterboard are shown in . It can be seen from , that in specimen W-15FP the temperature between the plasterboard and the CLT panel was less than the temperature recorded by thermocouples in W-15FPJ and W-12.5FP25PW. In specimen W-15FPJ, the temperature recorded between the plasterboard was slightly higher than the W-15FP panel at the first 2.5 hours of the test. In the W-12.5FP25P specimen, the temperatures between the protective claddings and CLT panel followed a similar trend to that of the W-15FP and W-15FPJ panels for the first 2 hours of the test. However, a sharp increase in the temperature between the plasterboard and CLT panel was observed at around 120 minutes into the W-12.5FP25PW test and consequently, the temperature between the plasterboard and the CLT panel reached the furnace temperature. This sudden increase in the temperature behind the protective claddings at around 2 hours of the test shows that the fall-off of the plasterboard occurred at that time.
Conclusions
This paper presents fire tests on four 120 mm thick three-layer CLT wall panels which were exposed to fire from one side. The test series includes panels with, and without, protective cladding, each of which was vertically loaded. The following are concluded from the experimental fire testing of CLT wall panels under standard fire curve testing conditions:
The average charring rate of the Irish-grown Sitka spruce unprotected CLT wall panel is between 0.63 and 0.66 mm/min, which closely matches the one-dimensional charring rate of 0.65 mm/min in Eurocode 5 (Bartlett et al., Citation2015). Thus, these Eurocode one-dimensional charring rates can be used for Irish Spruce CLT wall panels for fire design.
Protective cladding with and without joints was examined and for a 15 mm Fireline gypsum plasterboard without joints charring of the CLT panel was delayed by 30 minutes, while for the same protection measure with a vertical joint in the middle and a joint along the perimeter of the exposed surface of the panel only delayed the charring of CLT panel by 25 minutes. This shows a reasonably close alignment with the EN 1995-1-2.
The CLT wall panel which was protected with a combination of 12.5 mm Fireline plasterboard and 25 mm plywood delayed the charring of the CLT panel by 44 minutes. This also shows a reasonably close alignment with the EN 1995-1-2.
The experimental testing in this paper shows that the protective claddings can significantly delay the onset of charring of CLT panels but joints in the protective cladding system reduce the fire performance of the wall system and should be considered during design.
The above research provides a foundation to study the performance of Irish Spruce CLT panels under significant loads to understand the fire behaviour of Irish spruce CLT panels in timber structures.
Acknowledgments
The MODCONS Project is a joint project between the Timber Engineering Research Group at the University of Galway and Munster Technological University focused on increasing the knowledge and use of timber in modular construction. The authors thank the project team from the University of Galway, in particular, Prof. Annette M. Harte and Dr Patrick McGetrick for providing us with the CLT panels for this research.
Disclosure statement
No potential conflict of interest was reported by the author(s).
Additional information
Funding
References
- Bartlett, A. I., Hadden, R. M., Bisby, L. A., & Law, A. (2015, February 2-4). Analysis of cross-laminated timber charring rates upon exposure to non-standard heating conditions [Paper presentation]. Fire and Materials, San Francisco, USA (pp. 667-681). Interscience Communications Ltd.
- Buchanan, A. H., & Abu, A. K. (2017). Structural design for fire safety (2nd ed.). Wiley.
- CEN. (2004). EN1995-1-2. In Eurocode 5. Design of timber structures. Part 1-2: General – Structural fire design. Comité Européen de Normalisation.
- Dârmon, R., & Lalu, O. (2019). The fire performance of cross laminated timber beams. Procedia Manufacturing, 32, 121–128. https://doi.org/10.1016/j.promfg.2019.02.192
- European Committee for Standardisation. (2015). EN 16351: Timber structures – Cross Laminated Timber – Requirements.
- Fragiacomo, M., Menis, A., Clemente, I., Bochicchio, G., and Tessadri, B. (2012). Experimental and numerical behaviour of cross- Laminated timber floors in fire conditions [Paper presentation]. World Conference on Timber Engineering, Auckland New Zealand, 36–43.
- Frangi, A., Fontana, M., Hugi, E., & Jübstl, R. (2009, November). Experimental analysis of cross-laminated timber panels in fire. Fire Safety Journal, 44(8), 1078–1087. https://doi.org/10.1016/j.firesaf.2009.07.007
- Frangi, A., Fontana, M., Knobloch, M., & Bochicchio, G. (2008). Fire behaviour of cross-laminated solid timber panels. Fire Safety Science, 9, 1279–1290. https://doi.org/10.3801/IAFSS.FSS.9-1279
- Friquin, K. L. (2011, August). Material properties and external factors influencing the charring rate of solid wood and glue-laminated timber. Fire and Materials, 35(5), 303–327. https://doi.org/10.1002/fam.1055
- Harte, A., McPolin, D., Sikora, K., O’Neill, C., & O’Ceallaigh, C. (2014, August 28-29). Irish timber – Characterisation, potential and innovation [Paper presentation]. Civil Engineering Research in Ireland, Belfast, UK (pp. 63–68). Civil Engineering Research Association of Ireland.
- ISO 834-1. (1999). Fire resistance test - elements of building construction, Part 1: General requirements. International Organization for Standardization.
- Kippel, M., Leyder, C., Frangi, A., & Fontana, M. (2014). Fire tests on loaded cross-laminated timber wall and floor elements. Fire Safety Science, 11, 626–639. https://doi.org/10.3801/IAFSS.FSS.11-626
- Lau, P. W. C., White, R., & Van Zeeland, I. (1999, September). Modelling the charring behaviour of structural lumber. Fire and Materials, 23(5), 209–216. https://doi.org/10.1002/(SICI)1099-1018(199909/10)23:5<209:AID-FAM685>3.0.CO;2-A
- Mestek, P., Kreuzinger, H., & Winter, S. (2008, June 2-5). Design of Cross Laminated Timber (CLT) [Paper presentation]. World Conference on Timber Engineering (p. 8), Miyazaki, Japan, 156–163.
- Miyamoto, B., Bechle, N. J., Rammer, D. R., & Zelinka, S. L. (2021, February). A small-scale test to examine heat delamination in Cross Laminated Timber (CLT). Forests, 12(2), 232. https://doi.org/10.3390/f12020232
- Muszyński, L., Gupta, R., Hyun Hong, S., Osborn, N., & Pickett, B. (2019, July). Fire resistance of unprotected cross-laminated timber (CLT) floor assemblies produced in the USA. Fire Safety Journal, 107, 126–136. https://doi.org/10.1016/j.firesaf.2018.12.008
- O’ceallaigh, C., Sikora, K., & Harte, A. (2018, August). The influence of panel lay-up on the characteristic bending and rolling shear strength of CLT. Buildings, 8(9), 114. https://doi.org/10.3390/buildings8090114
- Östman, B., Mikkola, E., Stein, R., Frangi, A., König, J., Dhima, D., Hakkarainen, T., & Bregulla, J. (2010). Fire safety in timber buildings: Technical guideline for Europe. In B. Östman (Ed.), SP report (pp. 140). SP Technical Research Institute of Sweden.
- Pope, I., Hidalgo, J. P., & Torero, J. L. (2021, March). A correction method for thermal disturbances induced by thermocouples in a low-conductivity charring material. Fire Safety Journal, 120, 103077. https://doi.org/10.1016/j.firesaf.2020.103077
- Sandoli, A., D’Ambra, C., Ceraldi, C., Calderoni, B., & Prota, A. (2021, February). Sustainable cross-laminated timber structures in a seismic area: Overview and future trends. Applied Sciences, 11(5), 2078. https://doi.org/10.3390/app11052078
- Schmid, J., Konig, J., and Kohler, J. (2010, June 20-24). Fire-exposed cross-laminated timber-modelling and tests. Presented at the World Conference on Timber Engineering, Trentino, Italy, 3268–3276.
- Suzuki, J., Mizukami, T., Naruse, T., & Araki, Y. (2016, Jul). Fire resistance of timber panel structures under standard fire exposure. Fire Technology, 52(4), 1015–1034. https://doi.org/10.1007/s10694-016-0578-2
- Wiesner, F., Bisby, L. A., Bartlett, A. I., Hidalgo, J. P., Santamaria, S., Deeny, S., & Hadden, R. M. (2019, January). Structural capacity in fire of laminated timber elements in compartments with exposed timber surfaces. Engineering Structures, 179, 284–295. https://doi.org/10.1016/j.engstruct.2018.10.084
- Wiesner, F., Hadden, R., Deeny, S., & Bisby, L. (2022, March). Structural fire engineering considerations for cross-laminated timber walls. Construction and Building Materials, 323, 126605. https://doi.org/10.1016/j.conbuildmat.2022.126605
- Wiesner, F., Randmael, F., Wan, W., Bisby, L., & Hadden, R. M. (2017, July). Structural response of cross-laminated timber compression elements exposed to fire. Fire Safety Journal, 91, 56–67. https://doi.org/10.1016/j.firesaf.2017.05.010
- Yang, T.-H., Wang, S.-Y., Tsai, M.-J., & Lin, C.-Y. (2009, February). The charring depth and charring rate of glued laminated timber after a standard fire exposure test. Building and Environment, 44(2), 231–236. https://doi.org/10.1016/j.buildenv.2008.02.010