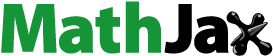
ABSTRACT
Climate change is a current global issue which must be addressed in order to create a sustainable future. While European countries have embraced the use of sustainable, naturally sourced materials, such as cross-laminated timber (CLT), their use in the UK and Ireland is much more limited. Currently, CLT is only manufactured in mainland Europe using C24 timber. This has higher characteristic properties than the most common grade of timber grown in the UK and Ireland. The UK climate generally results in fast growing trees, leading to lower density timber which is often correlated to lower strength properties. This research investigates the drying and conditioning methods used to achieve a moisture content of 12%, which is required to produce CLT panels according to European standards. The addition of a strengthening material, glass fibre mesh produces a novel product for the construction industry while improving a CLT panels overall performance in bending and shear, examined through a 4-point bending test. The research also focuses on finding the most suitable way of incorporating the glass fibre mesh between the bottom two layers of CLT panels with various adhesive techniques, examined through shear tests.
Introduction
The use of natural resources as a reputable building material is rapidly developing, particularly with timber. Cross-laminated timber (CLT) is becoming an increasingly favourable building material within Europe (CBI Ministry of Foreign Affairs [CBI], Citation2017).
CLT is an engineered product of timber which is formed by the arrangement of lengths of timber placed in layers, with each layer positioned perpendicular to one another. CLT panels are composed of an odd number of layers, typically three or five layers (Swedish Wood, Citation2019). The principal benefit of the use of CLT as a building material within the construction industry is the ability it has to replace traditional building materials such as steel or concrete products. These materials commonly have higher levels of carbon embedded within them relative to CLT. The continued use of high carbon materials would drastically impact the sustainability of the future due to global warming (Younger et al., Citation2008). It is important that the construction industry develop the use of a suitable alternative with a less detrimental impact on the environment.
However, with no suitable CLT production plant in the UK and Ireland, the product cannot be sourced locally and must be imported from other parts of Europe. This transportation adds to the overall carbon footprint of the product as well as adding approximately 10% to the costs, deeming it less sustainable as initially anticipated (Waugh Thistleton Architects, Citation2018).
To overcome this problem, CLT can be manufactured using timber supplies sourced locally within the UK and Ireland (Sikora et al., Citation2016). Sitka spruce has been regarded as a favourable timber source for CLT manufacture through past research due to its abundance in regions of Ireland and Scotland (Crawford et al., Citation2015). According to Ireland’s National Forest Inventory, 11.6% of Ireland alone is under forestry. This equates to 808,848 hectares of forest land at present which is a significant increase since 2006 when the total forestry area was 697,842 hectares (Ireland’s National Forest Inventory, Citation2022). It is important that there is a form of sustainable forest management in place with groups such as Coford, responsible for exploring the potential of the Irish forest sector in the emerging bioeconomy (Coford, Citation2017).
This study investigates the feasibility of the use of timber of strength class C16 rather than commonly used C24 as a source material for the production of CLT. Timber within a strength class of C16 typically has less favourable properties than C24 timber, such as strength, level of imperfections, density and elastic modulus. With the strength characteristics being reduced with the use of the lower strength class (C16) home-grown timber, the panel will benefit from another source of strength, such as a layer of glass fibre mesh reinforcement. Similar work has previously been undertaken involving the use of basalt fibreas a reinforcing material in timber elements which then use the layered beam theory, gamma beam theory and shear analogy theory to determine strength characteristics of the panels (O’Ceallaigh et al. Citation2014; Sikora et al., Citation2016).
Initial research will be based on finding the suitable drying and conditioning techniques of C16 Irish Sitka Spruce timber to achieve the suitable raw material to manufacture CLT panels with. Once this is established, CLT panels will be manufactured and tested in four-point bending tests to determine the panel strength characteristics such as modulus of elasticity. Research will also be conducted with the aid of a theoretical modelling programme to determine the benefit of adding an additional strengthening material, glass fibre mesh. The research will investigate the most appropriate way of incorporating this material into the CLT panel. The composite CLT panel (with addition of mesh) will then be tested with the same tests and results compared to determine the benefit the additional layer has on strength characteristics.
The importance of the construction industry within the United Kingdom and Ireland
The current shortage of housing units within the UK has reached over one million. More than 69,000 houses need to be constructed every year for the next 15 years to eradicate this demanding issue (BBC Briefing-Housing, Citation2020).
With these vast shortages, new-builds and existing households are becoming increasingly unaffordable. According to Sirius, a property finance company, a traditional build with a timeframe of 12 months would have an estimated loan value of £252,000 over a year whereas a modular build with a timeframe of 6 months would have an estimated loan value of £126,000 (Sirius, Citation2021). Any existing houses and new-builds rely on heavily on “man-made” materials such as steel and concrete blockwork, which have an immense carbon footprint, directly associating today’s construction with climate change due to carbon dioxide emissions. The process of concrete production alone is currently responsible for 5% of anthropogenic global CO2 production per annum (Chemistry World, Citation2008).
The use of timber in construction has a plethora of benefits including a low carbon production, high insulating properties and when used correctly has similar structural abilities to blockwork (BK Structures, Citation2022). With a great focus being placed on the search for a low carbon building materials, there has been an increase in timber import levels to the UK and Ireland since 2007, signifying the demand for timber in the construction industry (Federation TT, Citation2021).
According to a study carried out in 2021, the predicted demand for CLT use in the US and Canada alone was estimated to be 800,000 m3/year by the year 2030 (Brandt et al., Citation2021). According to “Checkatrade UK”, a CLT panel would typically cost £30/m2 to manufacture for a typical house in the UK and Ireland (Checkatrade, Citation2022). With domestic manufacture of the CLT panels, some production and transportation costs may be eliminated and so CLT manufactured using home-grown timber sources may be much more viable for housing in terms of costs while also improving the environmental footprint of the product. In addition, it has the significant benefit of arriving on-site fully machined allowing for rapid on-site assembly.
Requirements of raw timber for cross-laminated timber manufacture
In the past, CLT panels have been manufactured in the laboratory to replicate those manufactured in eastern European factories such as “KLH Massivholz” and “Metsawood”, and is governed by BS EN 16351 (Citation2021). Before the panels are assembled, the raw timber should be dried and conditioned under highly controlled settings to ensure the timber has a suitable moisture content and has dried at the correct pace to allow the moisture to fully leave without distorting the timber. Panels manufactured in industrial settings are subject to high amounts of vertical and lateral pressure to ensure maximum bonding between the joint of timber sections. Both these factors are of high importance in the manufacture of CLT panels. However, with past investigations, the drying and conditioning phase appears to be the control which is the most difficult to adapt. Studies show that the fundamental rule for timber drying is that the end quality of timber depends on the rate of drying (Reeb & Brown, Citation2007).
However, in an industrial setting, adding to manufacturing time respectively loses profits and so there is a balance between the drying time and condition of the timber. The required moisture content for timber used in CLT manufacture is 6–15% (BS EN 16351, Citation2021). However, it is important that this moisture content is the same throughout the whole section of the timber and not only at the section extremities. Trada explains, the moisture first evaporates from the surface of the timber, creating a gradient in the moisture content with a high degree of moisture in the core of the section. The moisture gradient then causes the water to be drawn to the surface which in turn evaporates (Trada, Citation2011).
Preliminary test: manufacture of CLT panels using ambient dried timber
An initial test set of CLT panels were manufactured in the laboratory. The C16 Irish Sitka Spruce timber was allowed to air dry in an open area of high ventilation and temperature of 18–20°C within the laboratory. The moisture content of the timber was checked daily using a “Protimeter” industry type hand held moisture probe which penetrated into the timber to a depth of around 10 mm. Once the probe gave a moisture content reading of 12–15% the panels were manufactured in line with guidance from BS EN 16351.
The chosen adhesive for the manufacture of the panels was Solfre 2261, a one component polyurethane wood glue supplied by a local company, Action Adhesives, and fulfils the requirements of CLT manufacture, stated in BS EN 16351 (British Standards Institutions [BSI], Citation2021). According to the Solfre 2261 Technical Data Sheet (TDS) (Chemique Adhesives, Citation2018), the open time at this temperature is 23 min. The required press time is 47 min and the cure time is a further 70 min. The panels were face bonded. Lateral and vertical pressure was applied during press time to allow successful bonding between the lamella and to close any lateral gaps. The lateral pressure was loaded to a maximum of 0.1N/mm2 using a hand hydraulic pump and the vertical loading was applied at 0.9N/mm2 (the required loading for the chosen adhesive), using a Dartec hydraulic loading machine.
Within hours after manufacture, gaps started appearing in the panels as shown in . The gaps between the lamella were measured daily using a feeler gauge and grew over time as shown in . When moisture testing using the moisture probe, the timber appeared to be successfully dried, however the inner core of the timber remained high in moisture. During the manufacture, with the pressure being applied for successful adhesion of the glue, the moisture was forced to the outer parts of each lamella, which then subsequently dried off with time, resulting in the shrinkage. These gaps resulted in insufficient bonding between the layers, indicating the high importance of the drying and conditioning stage in order to manufacture CLT panels with small lamella gaps. However, according to the US CLT Handbook, gaps in CLT up to 6 mm are not uncommon and so joints are typically assumed to be “tight-fitting” (CLT Handbook US, Citation2013).
The modulus of elasticity was established for the “preliminary” test panels made using the timber dried in ambient conditions, through four-point bending tests in accordance with BS EN 16351 (Citation2021). shows the modulus of elasticity calculated for the three layer and five layer panels, with total thicknesses of 90 mm and 150 mm respectively and spans 2250 and 3750 mm respectively. The values can be compared to that of three and five layer panels with similar thicknesses produced in an industrial setting, MetsäWood, manufactured using common grade C24 Spruce timber sources (Metsawood, Citation2012). In both panel sizes, panels made in the laboratory proved to have a significantly lower modulus of elasticity compared to those commercially made by MetsäWood. This reduced modulus of elasticity in the laboratory manufactured panels was due to the use of a lower strength raw material (C16) instead of the typically chosen C24 strength class. The insufficient drying leading to gaps in the lamella may also be a factor to consider in the reduction of panel stiffness. Through the manufacture and testing during this preliminary test, it can be determined that the drying and conditioning stage needs to be reconsidered.
Table 1. Laboratory data and data provided by Metsawood Leno on the moisture content of timber used to manufacture CLT panels along with corresponding modulus of elasticity values (Leno Metsawood, Citation2012).
Methods of drying and conditioning
In order to get an understanding of the patterns in timber drying and the rate it occurs in different conditions, some timber samples were dried in ambient conditions and some in a humidity and temperature controlled cabinet. The purpose of this was to determine if timber dried in ambient conditions could be suitable for manufacturing CLT. This was of interest, particularly given the experience and results of the preliminary test panels. The preliminary test panels were dried in ambient conditions, but the moisture content measured only using a handheld pinned probe and so a more detailed investigation was warranted.
Drying in ambient conditions
As shown in , timber samples were arranged and left to naturally dry in ambient conditions, as done in with the manufacture of the preliminary test panel. The room temperature fluctuated between 18°C and 22°C. The moisture content was determined using the oven drying method, as explained in Section 5.2. With the ambient conditions, the timber samples took around 20 days to reach the equilibrium moisture content of 12% and it was observed that distortion had occurred in the timber during this time. The rate of drying of the timber samples is as shown in . The rate at which the timber dried at within the first 10 days was the quickest and after this, the drying slowed off to a steady rate.
Drying in controlled conditions
An airtight drying cabinet was constructed to dry the timber in a temperature and humidity controlled environment. This allows for a common moisture content of the timber to be achieved to ease with comparison of results. According to the U.S. Department of Agriculture, a temperature of 20°C and 65% relative humidity would dry timber to an equilibrium moisture content of 12%, a suitable moisture content for timber used in the production of CLT (Simpson, Citation1998). Therefore, the drying cabinet controlling unit was set to a temperature of 20°C and 65% relative humidity and the timber was strapped using small ratchet straps to reduce twisting during the drying process. The drying set-up is as shown in . Moisture testing shows that after 12 days in the controlled drying cabinet, the timber reaches an equilibrium moisture content of 12% and remains at this level as long as temperature and relative humidity levels are untouched, shown in .
Methods of measuring moisture content
Use of handheld device
A handheld device can be used to get instant readings of moisture content from a sample of timber. Moisture meters can either have probes to penetrate the timber or can be pinless and non-invasive to the timber using electromagnetic sensors to read the moisture content. With the testing of timber of the preliminary tests a pinned probe was used. For readings in the next tests, an Orion 910 pin-less moisture meter was used. This meter was suitable for timber with moisture between 4.0% and 32.0%. To ensure accuracy of the hand held moisture meter it was verified with the oven drying method, as outlined in Section 5.2.
Oven drying method
The oven drying method is the most accurate form of testing the moisture content of a piece of raw timber as it directly measures the mass of moisture in the sample. The accuracy of other methods of determining moisture content can be verified by comparison with the oven drying method (Boone & Wengert, Citation1998). The method is described in BS EN 13183–1 (Citation2005) and involves taking samples of a length of timber, each being 20 mm in thickness. The first sample should be taken 300 mm in from the end of the sample and this piece should be discarded. In this case, a selection of 10 samples were cut. Immediately after being cut, these samples were weighed and recorded and then placed in an oven at 103°C (±2°C) and left for two hours, as shown in . The samples were weighed every 2 h until the difference between two successive weighings is less than 0.1%. The moisture content of the timber to which the samples were cut from can be calculated using EquationEquation (1)(1)
(1) from BS EN 13,183–1 (Citation2005).
Figure 7. Oven drying method used to determine moisture content of timber before manufacture of CLT panels.

where, ω is the moisture content as a percentage; m1 is the mass of the test slice before drying (in grams); m0 is the mass of the test slice after being oven dried (in grams) (BS EN 13,183–1, Citation2005).
Methods of incorporating a strengthening material into a CLT panel-adhesion techniques
With the expected panel strength characteristics being reduced due to the use of C16 timber for the manufacture of CLT panels, an additional strengthening material will be incorporated into the panel. This material will be added between the bottom two layers of timber. As an example, a three-layer panel will have a layer build up as follows:
Layer 4: Timber
Layer 3: Timber
Layer 2: Reinforcing material (GRP mesh)
Layer 1: Timber
A variety of GRP mesh mats were assessed on their suitability of providing added strength to a CLT panel. Consideration was based on; the compatibility of the mesh material with timber, the additional costs associated with the mesh, the material sustainability, the additional time taken to introduce the additional material during panel manufacture and the ease of manufacture in an industrial setting. With the main focus being on strengthening the CLT panel, it is important to ensure that a weak bond is not formed between the layer of timber and the mesh layer. The chosen material was a 450 gm powder bound fibreglass chopped strand mesh mat.
Four CLT samples were manufactured using various adhesion techniques to incorporate the added layer of GRP mesh into a CLT panel. In all cases, the sample panels were made to the standard outlined in BS EN 16351 and with an adhesive vertical pressure of 0.9N/mm2 for a duration of 47 min to achieve successful bonding. The pressure and duration is as specified in the adhesive data sheet and is used for all CLT manufacture in this research. The data sheet also specifies that the open time for this selected adhesive is 23 min and so the rate of application, including the time taken to apply the loading must be within this timescale (Chemique Adhesives, Citation2018).
In order to determine the suitability of adhesion types between the fibreglass layer and timber, shear tests were carried out on the small CLT panel samples.
Option 1: Manufacture of unreinforced CLT panel using one-component polyurethane wood glue
An unreinforced panel was manufactured as a control test to show how the CLT panel reacts in shear tests without the presence of the added strengthening material. This panel is manufactured to the standard outlined in BS EN 16,351 and one component polyurethane wood glue is used to bond the layers.
Option 2: Fibreglass mat with one-component polyurethane wood glue
Another option was to use the same wood glue as used throughout the full CLT panel to incorporate the fibreglass layer into the panel. By using this same glue as the adhesion for the mat this would reduce costs and time of manufacture. For manufacture of the samples, the glue was applied to the timber layer to which the mat was added followed by the application of more glue until the mat was fully covered. The next layer of timber was then added and normal procedures were followed with the remaining layers of timber. This option is shown in . The fibreglass layer is placed on top of the bottom layer of timber and then covered in the wood glue before the nect layers are added.
Option 3: Fully cured layer of fibreglass with epoxy resin bonded to timber using one component polyurethane wood glue
This option involved separately making a layer of resin bonded fibreglass, allowing it to cure and adding it to the CLT panel by bonding it with one-component polyurethane wood glue. The resin used was Sicomin SR5550/SD5503 SLOW Epoxy Resin and was sourced from a local company MB Fibreglass. This method increases time of manufacture, thus adding to manufacture costs and therefore would not be favoured in an industrial setting.
Option 4: Fibreglass mat with epoxy resin system
The chosen fibreglass is designed to be used with an epoxy resin system, Sicomin SR5550, which penetrates the mat to form a high strength material. This involved mixing the resin and hardener to form a compound. The manufacturer describes the epoxy resin system to be “excellent for bonding reinforcement materials such as glass or carbon onto wood”. This compound was poured onto the bottom layer of timber, to which the mat was then added and covered again using the resin compound, followed by another layer of timber. This sample was allowed to cure for 3.30 h, as specified by the manufacturer.
Comparison of adhesion techniques
Each panel was cut into eight evenly sized samples and tested under shear. This involved supporting the sample and loading vertically upon one lamination as shown in .
The first set of samples involved the unreinforced panel. This was the highest achiever under the shear test with a maximum average load of 15683N before failure occurred. The failure occurred in the timber showing that the bond between the timber and the glue was stronger than the timber itself.
The second set of samples tested were the panels manufactured using the one-component polyurethane wood glue to bond timber and mesh. These samples achieved the highest average maximum load of 15,500N and the failure was due to a split in the timber while the bond line remained intact. This illustrated a successful adhesion between the timber, glue and mesh. This failure is shown in .
The third set of samples tested were the panels manufactured using wood glue to bond timber and the hardened sheet of mesh. These samples achieved an average maximum load of 9634N. Failure again occurred along the timber to mesh glue line indicating that the wood glue cannot penetrate into the hardened mesh and resin sheet to form a successful bond between the timber and mesh.
The final set of samples tested were from the panels with the layer of mesh which was bonded using the epoxy resin system. The average maximum load the samples could withstand before failure was 5,585N, as shown in , which is equivalent to a shear stress on the sample of 2.98N/mm2. The split formed on the bond line of the mesh. This shows the lack of successful adhesion between the epoxy resin and the timber.
Figure 10. Average load (standard deviation on error bars) at point of failure for a range of CLT panels manufactured using a range of adhesion techniques.

Comparing the set of results for each of the four adhesion techniques, the addition of the mesh layer using the wood glue only would be the most suitable method which does not affect the shear strength of the panel. This is also the preferred method of adding the mesh layer when focusing on ease of manufacture and could be implemented in an industrial setting in the future without difficulty.
Determining the performance of a CLT panel manufactured using fully dried and conditioned timber
Testing of panels and calculations
In order to determine how feasible it is to replace traditional materials such as steel or concrete with CLT made from C16 home-grown timber sources, the mechanical and strength characteristics of the panels must be identified. This was carried out by manufacturing a new set of full sized CLT panels with timber dried and conditioned a controlled environment until 12% moisture content is achieved. This will allow the capabilities to be compared with traditional materials. shows the elastic modulus of the materials which can be used in CLT panels, with the chosen 450 gm glass fibre mesh from Section 6 being the additional material. This mesh will be incorporated into the panel using one-component polyurethane wood glue.
Table 2. Elastic modulus of various materials (Martynova et al., Citation2018) (BS EN 338, Citation2016).
In past research, the bending capacity of CLT panels has been found using the stiffness values of each individual length of timber in the CLT panel (Sikora et al., Citation2016; O’Ceallaigh et al., 2018). Using this method, the global and local stiffness of any “unreinforced” CLT panel can be calculated at a predictive level. With timber being a natural product, there will be variations in the density of the which would lead to small variations in the modulus of elasticity throughout the panel, as researched in the past (Tapia, Citation2022).
Panel spans will depend on the required testing outcome and can be determined in accordance with BS EN 16,351. When calculating only the shear strength of the panel, the span must be greater than 12 times the depth of the panel. When calculating the bending strength of the panel, the span must be between 24 and 30 times the depth of the panel.
EquationEquation (2)(2)
(2) based on BS EN 408, can be used to obtain the LOCAL modulus of elasticity (in N/mm2) of CLT panels (BS EN 408, 2010). This can then be used to calculate the bending strength.
In addition to this, EquationEquation (3)(3)
(3) can be used to obtain the GLOBAL modulus of elasticity of a panel (BS EN 408, 2010). This can then be used to calculate the rolling shear strength and stiffness of the panel.
However, different theoretical calculations were used in past research including the layered beam theory, gamma beam theory and shear analogy theory to determine strength characteristics of the panels to calculate a panels Young’s modulus value (O’Ceallaigh et al., Citation2016; Sikora et al., Citation2016).
In most previous studies, strength and stiffness characteristics were only determined on a panel manufactured from timber only and do not allow for consideration if an additional material is introduced in the panel (Sikora et al., Citation2016). Within this study, it is anticipated that by adding a layer of strengthening mesh, the overall performance of the panel will be improved; thus improving the panel stiffness.
The theoretical strength and stiffness of a CLT/GRP composite panel strength can be achieved by homogenizing the composite using the Young’s modulus of the materials in each layer of the panel and transforming it into a single material using modular ratios, as previously used by O’Ceallaigh (2018). From this, the parallel axis theorem can then be used to calculate the moment of inertia for the cross section of the panel. Thus, bending stresses on positions of the panel can simply be calculated for various loads. However, it is important to note that at this point this theoretical modelling is only suitable for the calculation of strength characteristics up to the moment of serviceability failure of the outermost component in the CLT panel. Post elastic behaviour, most notably in the outmost compression zone is not yet considered in the model. This theoretical modelling technique can also be used to calculate the same characteristics when the panel has an existing strengthening layer.
A similar model has been presented by Blank and Frangi in past research which has the ability to calculate the bending resistance of fibre reinforced glulam panels (glass and carbon fibre) in the post-elastic range and the elastic range. The outcomes from this research showed that by using fibre reinforcement in the tension area of a glulam panel, the bending resistance is increased (Blank et al., Citation2020).
shows the experimental and theoretical mid span deflections at neutral axis results for two similarly sized panels. Both panels are of 1560 mm in span with a depth of 120 mm, determining the global modulus of elasticity and allowing the rolling shear strength and stiffness to be calculated, in accordance with BS EN 16,351 and BS EN 408. One panel will be reinforced with the GRP mesh and the other will be an unreinforced CLT panel.
Both panels were subject to test loading. The unreinforced panel was loaded to a maximum of 20kN and the reinforced panel was loaded to a maximum of 25kN (safe loads before any chance of failure), and their deflections recorded. Six LVDTs were positioned at the mid span of the panel, in accordance with BS EN 16351. Two recorded the mid span deflection at the neutral axis, two recorded the deflection of the full panel (global deflection) and two recorded the deflection between the loading positions (local deflection). Plates were used to prevent indentation at the load and support points. The hanging bars to which the LVDTs are mounted on are freely hanging and so do not affect the panels overall stiffness. These hanging bars were used in accordance to the guidance given in BS EN 16351. The four point being test set-up is as shown in .
The theoretical modelling programme was then used to determine the theoretical deflection for both panels under a load of 15kN. This is a load that both panels reached and subsequently exceeded allowing for direct comparison. The results in illustrate the accuracy which can be achieved by using the theoretical model to get an idea of the physical performance of a CLT panel during experimental testing. The results show that the theoretical model over estimates the deflection values allowing for a safe determination of results. It can also be seen that through experimental testing, the addition of the layer of GRP mesh to create a reinforced panel results in a reduction of deflection of 3.5%. This is as to be expected, however theoretical results expect a greater reduction.
Figure 12. Experimental and theoretical global deflections at the mid span neutral axis of a 1560 mm panel under a load of 15kN.

Results from the other transducers measuring the global and local deflections are shown in . As illustrated in , the global deflection values are much greater than the local deflection values which is to be expected as the deflection is being taken over a greater span in the global reading. It is also important to note that the global deflection is greater in the panel with no mesh however the difference between the local deflections for the panels with and with no mesh is small. It should be noted that for shorter panels, deflection values are low, establishing a modest effect on stiffness.
Figure 13. The local and global deflections of reinforced and non-reinforced CLT panels of span 1560mm at various loads achieved through EXPERIMENTAL testing.

From this, the same theoretical modelling programme was then used to determine the performance for a broad range of panel spans including composite panels with the added strengthening layer. shows how various panels would THEORETICALLY deflect when a load of 15kN is applied. The panels compared are reinforced and unreinforced versions of panels with spans 1560 mm and 3000 mm, all with the same panel depth (120 mm) made up of three layers of 40 mm thicknesses. The layer of GRP mesh in the composite panel is 10 mm in thickness – a typical grade of mesh used in construction works involving plasterboard.
Figure 14. The comparison of THEORETICAL deflection results of panels with and without a layer of GRP mesh under a load of 15kN.

The theoretical results show that with the presence of the additional material layer, the same load applied results in a reduced deflection of 32%. This indicates that with the additional strengthening layer, the stiffness performance of the panel is improved. The theoretical results in also illustrate how the deflection due to a load increases significantly as the panel span increases which is as expected. The effect of the reinforcement within theoretical calculations is much greater than within experimental test results.
Improving the performance of CLT using an additional layer
As determined from the predicted values from the theoretical modelling programme, the addition of a layer of GRP mesh to create a reinforced CLT panel improves the stiffness; thus reducing the deflection caused upon loading.
With the same load of 15kN applied each time, the presence of the additional 10 mm layer of GRP mesh resulted in a theoretical mid-span deflection at the neutral axis reduction of 32% on average. The panel stiffness is improved as the tensile forces acting on the bottom of the panel are not only resisted by the overlapping pattern of the CLT but also by the additional material which has a high tensile force resistance. The use of GRP mesh between the bottom two layers of timber can be replaced with a variety of layering materials and the modelling programme can calculate the strength parameters when the value for the Young’s modulus for the additional material is imputed. This programme can therefore determine the combination of materials which produces panels with the highest performance, saving time and waste of timber and other materials.
Conclusions
The following main conclusions have been drawn from this study:
The timber must be at the correct moisture content to ensure stability or it risks shrinkage or swelling potentially leading to gaps forming in the CLT panel.
It is confirmed that the addition of a layer of GRP mesh increases the overall CLT panel stiffness with the panels selected, based on the experimental testing on a single CLT panel.
Through theoretical calculations, it is confirmed that with the addition of a layer of GRP mesh, a panel deflects on average 32% less during a loading of 15kN.
Shear tests on the small samples of varying adhesion techniques show that the best way of incorporating GRP mesh into a CLT panel is through the use of wood glue (one-component polyurethane).
It has been confirmed that theoretical calculations based on linear elastic theory of curvature are relevant for calculating deflections in CLT panels, as confirmed by the experimental data.
It is also important to note the importance of the drying and conditioning stage before manufacture of the CLT panels. In order to get the timber to a moisture content of 12%, the timber must be placed in an environment of 20°C and 65% relative humidity or can be kiln dried as typically done in industrial factories.
It has also been determined that the most accurate method of determining the moisture content in a timber sample is by using the oven drying method as outlined in BS EN 13183-1.
It is important to ensure the mesh layer is completely covered in this glue before continuing with the addition of timber layers. This method would be favoured in an industrial setting due to the ease of addition during manufacture.
Disclosure statement
No potential conflict of interest was reported by the authors.
References
- BBC Briefing-Housing. (2020). [Online]. Retrieved September 10, 2021, from bbci.Co.uk
- BK Structures. (2022). CLT [online]. Retrieved January 20, 2022, from bkstructures.co.uk
- Blank, L., & Frangi, A. (2020). Design model for the bending resistance of fibre reinforced glulam. [online]. Retrieved June 10, 2023, from https://www.sciencedirect.com/science/article/pii/S0141029619315202
- Boone, R. S., & Wengert, E. M. (1998). Guide for using the oven-dry method for determining the moisture content of wood. (Forestry facts) [Online]. Retrieved February 5, 2022, from Microsoft Word-89.d https://forestandwildlifeecology.wisc.edu/wp-content/uploads/sites/111/2017/07/89.pdf oc (wisc.edu)
- Brandt, K., Latta, G., Camenzind, D., Dolan, J., Bender, D., Wilson, A., & Wolcott, M. (2021). Projected cross-laminated timber demand and lumber supply analysis. Retrieved February 20, 2022, from ncsu.edu
- British Standards Institutions. (2005). BS EN 13183-1 – Moisture content of a piece of sawn timber.
- British Standards Institutions. (2016). BS EN 338 structural timber strength classes.
- British Standards Institutions. (2021). BS EN 16351 - Timber structures — Cross laminated timber — Requirements.
- CBI Ministry of Foreign Affairs. (2017). Exporting cross laminated timber (CLT) to Western Europe. [online]. Retrieved June 22, 2022, from https://www.cbi.eu/market-information/timber-products/cross-laminated-timber/europe
- Checkatrade. (2022). Cross laminated timber cost. [online]. Retrieved June 13, 2022, from https://www.checkatrade.com/blog/cost-guides/cross-laminated-timber-cost/
- Chemique Adhesives. (2018). Solfre 2261 product technical data sheet. Retrieved April 12, 2022.
- Chemistry World. (2008). The concrete conundrum. [Online]. Retrieved September 14, 2021, from rsc.org
- CLT Handbook US. (2013). [Online]. Retrieved June 5, 2023 from https://cdn2.hubspot.net/hubfs/5577290/PDFs/CLT%20Handbook/CLT_USA-Complete-document-Think_Wood.pdf
- Coford. (2017). Growing the irish forest bioeconomy. [Online] Retrieved February 3, 2023, from assets.gov.ie
- Crawford, D., Hairstans, R., Smith, S., & Papastavrou, P. (2015). Viability of cross-laminated timber from UK resources. [online]. Retrieved April 14, 2021 icevirtuallibrary.com
- Federation TT. (2011). Statistical review. [online]. Retrieved January 5, 2022, from https://ttf.co.uk/timber-industry-well-positioned-to-meet-demand-in-2022-says-ttf/
- Ireland’s National Forest Inventory. (2022). Main findings. [online]. Retrieved June 7, 2023, from www.gov.ie
- Martynova, E., & Cebulla, H. (2018). Inorganic and composite fibers. [online]. Retrieved March 26, 2022, from https://www.sciencedirect.com/topics/engineering/glass-fibre#:~:text=Glass%20fibres%20are%20most%20commonly,modulus%20is%2070%2C000%20N%2Fmm%C2%B2
- Metsawood, L. (2012). Technical knowledge 2012-cross laminated timber CLT. Retrieved November 4, 2021, from archiexpo.com
- O’Ceallaigh, D. O., & Harte, A. M. (2016). An investigation of the viscoelastic and mechano-sorptive creep behaviour of reinforced timber elements. [Online]. https://www.researchgate.net/publication/334139043_An_Investigation_of_the_Viscoelastic_and_Mechano-sorptive_Creep_Behaviour_of_Reinforced_Timber_Elements/link/5ee0eeb945851516e665b41e/download
- O’Ceallaigh, C., Harte, A. M., Sikora, K. S., & McPolin, D. O. (2014, May 5). Enhancing Low Grade Sitka Spruce Glulam Beams with Bonded-in BFRP Rods. In COST Action FP1004 Conference, Experimental Research with Timber, Prague, Czech Republic Retrieved August 8, 2023, from https://www.researchgate.net/publication/334139043_An_Investigation_of_the_Viscoelastic_and_Mechano-sorptive_Creep_Behaviour_of_Reinforced_Timber_Elements
- Reeb, J. E., & Brown, T. D. (2007). Air- and Shed-drying Lumber. [Online]. Retrieved December 14, 2021, from oregonstate.edu
- Sikora, K. S., McPolin, D. O., & Harte, A. M. (2016). Effects of the thickness of cross-laminated timber (CLT) panels made from Irish sitka spruce on mechanical performance in bending and shear. [Online]. Retrieved January 10, 2021, from https://www.sciencedirect.com/science/article/pii/S0950061816307000
- Simpson, W. T. (1998). Equilibrium moisture content of wood in outdoor locations in the United States and worldwide. [Online]. Retrieved January 3, 2021, from usda.gov
- Sirius Property Finance. (2021). Modular building could save developers hundreds of thousands in funding interest. [Online]. Retrieved June 25, 2022, from siriusfinance.Co.uk
- Swedish Wood. (2019). The CLT handbook. [online]. Retrieved June 22, 2022, from woodcampus.co.uk
- Tapia, C. (2022). Variation of mechanical properties in oak boards and its effect on glued laminated timber. Retrieved June 14, 2023.
- Trada Technology. (2011). Drying and moisture content of timber.
- Waugh Thistleton Architects. (2018). 100 PROJECTS UK CLT. [online]. Retrieved March 26, 2022, from thinkwood.com
- Younger, M., Morrow-Almeida, H. R., Vindigni, S. M., & Dannenberg, A. L. (2008). The built environment, climate change, and health opportunities for co-benefits. [Online]. Retrieved June 22, 2022, from https://doi.org/10.1016/j.amepre.2008.08.017