ABSTRACT
OBJECTIVES: Alzheimer’s disease (AD) is a severe neurodegenerative disease with presentation of the neuronal death, memory loss and cognitive decline. The relationship between neuroinflammation and AD has been well documented. However, the options of anti-inflammatory treatment are very limited in patients with AD. Previous studies showed that flavonoids might be an effective treatment and thymoquinone (TQ), an aromatic hydrocarbon found in Nigella sativa suggested as a candidate molecule due to having strong anti-inflammatory effects. Our study aimed to investigate the effects of TQ on neuroinflammation and neuroprotection in Aβ(1–42) infused rat model of AD.
METHODS: A rat model of AD was established in 6 month-old rats (n = 23) by intra-hippocampal infusion during 14 days via a micro-osmotic pump containing aggregated Aβ(1–42). After model establishment, TQ at a dosage of 20 mg/kg/day was intubated intragastrically for 15 days. The functional recovery was determined using the Morris Water Maze task by measuring memory consolidation. The content of cytokine levels of Tumour Necrosis Factor-alpha (TNF-α), Interleukin-1 beta (IL-1β), Interleukin-1 alpha (IL-1α) and Interferon-gamma (IFN-γ) in the hippocampus was assessed by Magnetic Luminex assay. In order to reveal the functional molecular changes in hippocampal tissue upon TQ administration, the protein expression profile of neuronal migration protein Doublecortin (DCX), synaptic plasticity marker Mitogen Activated Protein Kinase2 (MAP2) and apoptosis related protein Poly (ADP-ribose) Polymerase (PARP) was analyzed by Western blotting.
RESULTS: Aβ(1–42) infused group had worse memory performance than sham control group on Day 4 with an amelioration in this behaviour by TQ. In our study, the levels of TNF-α, IL-1α and IL-1β did not significantly alter among groups. On the other hand, Aβ(1–42) infusion slightly decreased the level of IFN-γ compared to sham control group. TQ treatment ameliorated both impaired memory performance and IFN-γ levels. It was found that TQ treatment increased the protein levels of DCX compared to the sham control group. Also, the levels of MAP2 and the activation of PARP protein markedly decreased in both Aβ(1–42) and Aβ(1–42)+TQ groups compared to the sham control groups Pearson’s correlation test showed a positive relation between IL-1β and DCX in the Aβ(1–42) group.
DISCUSSION: Our data suggested that TQ-related functional improvement might result from the increasing level of neurogenesis and ameliorating the level of IFN-γ in the Aβ(1–42) infused rat model of AD.
Introduction
Alzheimer’s disease (AD) is a progressive neurodegenerative disorder which is characterized by two main pathological hallmarks including the formation of senile plaques and neurofibrillary tangles. The neurotoxicity due to these aberrant structures results in the loss of neuronal function and neuronal cell death by triggering the inflammatory activity of microglia [Citation1]. The response-driven mainly by activated microglial cells has been noticed in both animal models and patients with AD [Citation2]. The activation of microglia is accompanied by an activated complements system and high levels of specific cytokines and chemokines [Citation3].
Like other brain disorders [Citation4], a relationship between neuroinflammation and neurodegeneration in AD has been well documented since different cytokines such as Interleukins (ILs), Tumour Necrosis Factors (TNFs), Interferons (IFNs), and Transforming Growth Factors (TGFs) are actively participated in the pathogenesis of AD [Citation5–7]. However, there were contradictions among different research groups in terms of the level of cytokines and regulation on the expression of cytokines both in the AD animal models and the patients with AD [Citation5]. These contradictory results complicated to define the roles of cytokines and chemokines as diagnostic or therapeutic targets for AD neurodegeneration.
In the conventional treatment of AD, well-accepted clinical drugs such as acetylcholinesterase (AChE) inhibitors and N-methyl-D-aspartate (NMDA) receptor antagonists are only used for compensation of cholinergic dysfunction or improvement of cognitive functions [Citation8]. Therefore, current treatment strategies are limited to provide symptomatic relief by slowing the cognitive, behavioural, and psychological progression of AD [Citation9]. For that reason, alternative therapeutic strategies for AD are needed to prevent or delay the onset of the clinical symptoms and to remove the underlying pathology. Recently, a growing interest in the use of herbal medicine because of their neuroprotective properties has developed for AD treatment [Citation10].
Thymoquinone (TQ), one of the main active ingredients of the black cumin seeds (Nigella sativa), is a candidate molecule for herbal medicine due to its various therapeutic values. TQ may be a potential agent against to AD regarding the results of studies showing that its antioxidant, anti-inflammatory and neuroprotective properties concerning central nervous system disorders [Citation11–13]. For instance, TQ treatment of cultured hippocampal and cortical neurons efficiently improved cell viability by decreasing neurotoxicity via inhibition of mitochondrial membrane potential depolarization and reactive oxygen species (ROS) generation after amyloid beta induced neurotoxicity [Citation14]. Also, TQ prevented Aβ(1–42) aggregations in vitro and restored synaptic vesicle recycling inhibition [Citation14]. Furthermore, it was observed that TQ improved LPS-induced learning and memory deficiencies in rat hippocampus by decreasing inflammatory markers such as IL-6, TNF-α, and NO metabolites [Citation15]. In a streptozotocin-induced neurodegeneration model, TQ treatment showed its antioxidant and regenerative effects on the hippocampus through the inhibition of NOS enzymes and the activation of the MAPK pathway [Citation16].
While previous studies provide data for the therapeutic effect of TQ on AD, we aimed to investigate the effects of TQ on the cytokines and protein expression levels in Aβ(1–42) infused rat model of AD regarding its possible roles in neuroinflammation and neuroprotection.
Materials & methods
Animals
Adult female Sprague Dawley rats (6-month-old) were housed in a quiet, temperature and humidity-controlled room (21 ± 2°C; 62 ± 7% relative humidity; 12-h cycles dark/light) They were fed ad libitum with a standard dry rat diet and tap water. The Guide for the Care and Use of Laboratory Animals adopted by the National Institutes of Health (U.S.A.) and the Declaration of Helsinki were followed during experiments. Experimental protocol of this study was approved by the local scientific ethical committee of Bezmialem Vakif University, Istanbul, Turkey (2015/229).
Aβ(1–42) infused rat model
Lyophilized Aβ(1–42) peptide (SCP0038, Sigma Aldrich) was reconstituted in stock solution (0.1% TFA, 35% acetonitrile and 64,9% dH2O). Then, soluble peptide suspensions were incubated at 37°C for 72 h with gentle shaking for fibril formation [Citation17]. An infusion cannula was implanted into the right hippocampus (AP:−3,60: L:−2,00: V:−4,00 from bregma) [Citation18] and it was fixed in place with Loctite contact cement. Subsequently, the skin incision was sutured and the rats were injected with saline and 0.1 ml of Sefazone S (Astech Pharma) in order to rehydration and prevention from infection. Following surgery, the rats were placed in their home cages for a continual infusion for two weeks by attaching an infusion cannula to a mini-osmotic pump (Alza, Palo Alto, CA, U.S.A.) containing Aβ(1–42) (300 pmol/day) or 0.9% NaCl saline solution [Citation19].
Thymoquinone administration
At the 15th day of surgery, rats (n = 23) were randomly divided into three groups; Sham control (n = 8, 0.9% NaCl saline solution infusion via osmotic pump and corn oil administration via intragastric infusion for the following two weeks), Aβ(1–42) (n = 8, Aβ1–42 infusion), and Aβ(1–42) +TQ (n = 7, Aβ(1–42) infusion and TQ 20 mg/kg in corn oil) administration via intragastric intubation for the following two weeks.
Behavioural analysis
After TQ administration, Morris Water Maze (MWM) was applied to observe changes in the learning and memory after Aβ infusion [Citation16,Citation20]. MWM, a circular, stainless pool (a height of 51 cm and a diameter of 210 cm) filled with water containing a non-toxic paint. The maze was divided into four equal quadrants and a hidden platform (11 × 11 cm) was centred 2 cm below the surface of the water. A daily session of four trials was given to all groups for four consecutive days. The trial was finished when the animal found the platform or 60 s passed. The video-tracking system (EthoVision XT11 software, Noldus Information Technology, The Netherlands) was automatically recording the escape latency to reach the invisible platform.
Hippocampal tissue analysis
After behavioural assessments, rats were sacrificed under the anaesthesia and their brains were quickly removed to dissect their hippocampi. The obtained hippocampal tissues were stored at −80°C until analyses. Frozen hippocampal tissues were homogenized with RIPA lysis buffer in the presence of protease and phosphatase inhibitor cocktail. Homogenized hippocampal tissues were centrifuged 14000 rpm for 15 min at 4°C and supernatants were collected for further analysis.
Relative expression of proteins including neuronal migration protein Doublecortin (DCX), synaptic plasticity marker Microtubule Associated Protein 2 (MAP2) and apoptosis related protein Poly (ADP-ribose) Polymerase (PARP) were investigated in order to reveal the functional molecular changes in the hippocampal tissue upon TQ administration in the Aβ(1–42) infused rats. Protein concentrations were determined using BCA assay kit in Multiskan™ GO Microplate Spectrophotometer (Thermo Fisher Scientific; Paisley, England). An equal amount of proteins were separated by sodium dodecyl sulfate polyacrylamide gels (SDS-PAGE) and subsequently transferred to a PVDF membrane. Then, the blots were incubated with primary antibodies: anti-DCX (Thermo Scientific), anti-PARP (Cell Signaling Technologies), anti-MAP-2 (Thermo Scientific) diluted in 5% milk powder in 0.1% Tween 20/0.1 M Tris-buffered saline (TBST) at 4°C overnight. The following day, membranes were washed and incubated in peroxidase-conjugated secondary antibodies which were also diluted in the same solution as the primary antibodies. Signal detection was obtained with luminol substrate (Advansta, San Francisco, U.S.A.) under CCD camera with Fusion FX7 (Vilber Lourmat) system. The loading control was checked with a monoclonal mouse antibody against β-actin and β-tubulin (Thermo Fisher Scientific, Paisley, England). Immunoreactive protein bands were quantified densitometrically using the ImageJ programme (NIH; Washington, U.S.A.).
The cytokine levels of tumour necrosis factor alpha (TNF-α), interferon gamma (IFN-γ), interleukin1 alpha and beta (IL-1α and IL-1β) in the content of hippocampus were evaluated in order to determine the effects of TQ on inflammation by utilizing Magnetic Luminex Screening Assay (LXSARM-04, R&D Systems, Minneapolis, MN, U.S.A.) according to the manufacturer’s instructions. Briefly, 50 μl of lysate prepared from hippocampus dissected from rats or standards were incubated with antibody-linked beads for two h on shaker, afterwards incubated 1 h with biotinylated secondary antibodies before 30 min incubation with streptavidin-phycoerythrin. Acquisitions were performed by MULTIPLEX Analyst through MAGPIX system (Luminex).
Statistical analysis
Significant differences among the groups were determined using the one-way ANOVA analysis (SPSS for Windows, version 18.0, Chicago, IL, U.S.A.). Post-hoc comparisons were made using the least significant difference (LSD) tests. Pearson’s correlation test was also applied to determine the relationship between the cytokines levels of inflammation (SPSS for Windows, version 18.0, Chicago, IL, U.S.A.). Effect sizes (η2) for statistically significant values were estimated by Cohen’s d [Citation21]. d ≤ 0.2 accepted as small effect size, d = 0.5 accepted as mean effect size, d ≥ 0.8 accepted as large effect size. All values are reported as mean ± standard error of the mean (SEM). Statistical significance was set at p < 0.05.
Results
Effect of TQ administration on behaviour in Aβ(1–42) infused rats
The MWM training to test for spatial reference learning was assessed using statistical analysis by one way ANOVA was revealed insignificant differences among groups on Day 1 (F(2:19) = 0.009, p = 0.991) and a marginally significant group differences on Day 4 (F(2:19) = 2.858, p = 0.097). During training in the MWM, as expected, a decrease in the latency to reach the hidden platform was observed from the first day to the fourth day of training in all groups (). However, this decrease was slow in the Aβ(1–42) group than the sham control group on Day 4 according to the post hoc LSD test (p = 0.040) (). TQ administration ameliorated this effect because there was no significant difference between Aβ(1–42)+TQ group and sham control group (p = 0.559) and learning and memory performance of Aβ(1–42)+TQ group was better than Aβ(1–42) group on the fourth day of the MWM training ().
Effect of TQ administration on inflammation in Aβ(1–42) infused rats
In our study, the levels of any cytokine did not significantly alter among groups (F (2:19) = 2.178, p = 0.144; effect size (η2) = 0.204 for IFN-γ; F (2:21) = 0.442, p = 0.649 for IL-1α; F (2:22) = 0.163, p = 0.850 for IL-1β; F(2:22) = 0.137, p = 0.872 for TNF-α) (). On the other hand, multiple comparisons according to the post hoc LSD test showed that Aβ(1–42) infusion slightly decreased the level of IFN-γ compared to the sham control group (p = 0.053). Furthermore, TQ treatment ameliorated this effect ((A)). However, there was no change in the levels of IL-1α ((B)), IL-1β ((C)) and TNF-α ((D)). According to the Pearson’s correlation test, a negative correlation between IL-1β and IFN-γ (p = 0.019) was noted in the Aβ(1–42) infusion group. However, TQ treatment produced a positive correlation between IL-1β and IFN-γ (p = 0.005) ().
Figure 2. The concentrations of selected inflammatory markers (A) IFN-γ (B) IL-1α (C) IL-1β (D) TNF-α.which were measured by Magnetic Luminex system for all groups. Error bars denote SEM. The degree of significance is denoted as * for p ≤ 0.05.
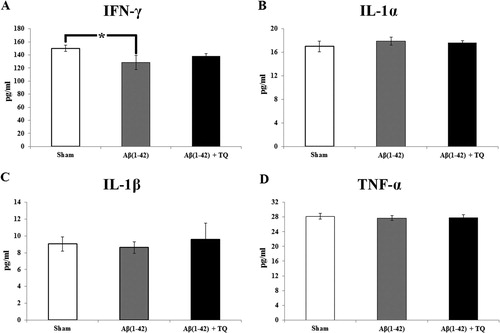
Table 1. The results of Pearson's correlation of the levels of IFN-γ, IL-1α, IL-1β and TNF-α with each other among sham, Aβ(1–42) and Aβ(1–42) + TQ groups.
Effect of TQ administration on protein expression in Aβ(1–42) infused rats
According to one way ANOVA, there was a significant between group differences in the levels of MAP-2 (F(2:8) = 15.696, p = 0.004, effect size (η2) = 0.840) and PARP (F(2:8) = 31.346, p = 0.001, effect size (η2) = 0.913) with a marginal between group differences in the level of DCX (F(2:8) = 4.359, p = 0.068, effect size (η2) = 0.592). Post hoc LSD test showed that the protein levels of DCX in Aβ(1–42)+TQ group significantly increased compared to the sham control group (p = 0.026) ((A)). Also, MAP2 protein expression markedly decreased in both Aβ(1–42) and Aβ(1–42) +TQ groups compared to sham control groups (p = 0.002 and p = 0.004, respectively) ((B)). Furthermore, the levels of protein expression of PARP decreased in both Aβ(1–42) and Aβ(1–42) +TQ groups compared to the sham control groups (p = 0.002 and p ≤ 0.001, respectively) ((C)). Pearson’s correlation test showed a positive relation between IL-1β and DCX in the Aβ(1–42) group (p = 0.024) ().
Figure 3. Representative pictures and relative amounts of (A) DCX to beta tubulin (B) MAP-2 to beta tubulin, and (C) PARP 89/116protein expressions analyzed by Western blot for all groups. Error bars indicate SEM. The degree of significance is denoted as * for p ≤ 0.05, ** for p ≤ 0.01, and *** for p ≤ 0.001.
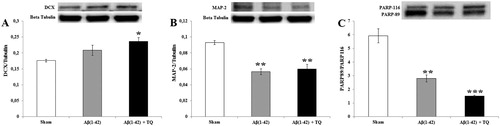
Table 2. The results of Pearson's correlation of the levels of inflammatory markers with DCX, MAP2 and PARP in the sham, Aβ(1–42) and Aβ(1–42) + TQ groups.
Discussion
The relationship between neuroinflammation and neurodegenerative disorders including AD has been studied by many investigators and suggested that the molecular mechanism of AD pathophysiology on learning, memory and neuronal plasticity might occur under the influence of inflammation [Citation22,Citation23]. Much of the attention has been devoted to natural anti-inflammatory substances to improve the cognitive impairments in AD-like pathology [Citation24,Citation25]. TQ might be a strong candidate for preventing or delaying the symptoms of AD by reducing neurotoxicity via its anti-inflammatory activity [Citation26]. However, the molecular effect of TQ on AD has not yet been established in detail. Here, we aimed to investigate the mechanism of TQ in terms of neuroinflammation and neuroprotection in the hippocampus of Aβ(1–42) infused rat model of AD.
In our AD model, a decline in the learning and memory performance at the last day of training was observed depending on Aβ infusion as shown in the previous studies [Citation27–29]. The rats with AD learnt slowly and showed worse performance in the memory task. However, TQ treatment increased the level of memory performance to control levels on Day 4, suggesting the ameliorative effect of TQ in spatial learning. The recovery role of TQ in cognitive functions was also demonstrated in distinct models of neurodegeneration [Citation11,Citation13,Citation30].
In previous studies, the neuroprotective role of TQ was associated with its modulatory effects on inflammation [Citation31]. Also, it was noted that inflammation had a crucial role in the initiation and deterioration of neurodegeneration in AD [Citation32]. TNF-α,IFN-γ and IL-1 act as either pro-or anti-inflammatory having some sort of a dual function. In our AD model, a significant decrease was observed in the levels of IFN-γ which is a cytokine that plays an essential role in inducing and modulating of immune responses and an effector of inflammation [Citation33,Citation34]. While there has been inadequate information related to the alterations in the level of IFN-γ among AD patients, a decrease in IFN-γ level in transgenic mice model of AD was obtained in parallel to our findings [Citation35]. In the present study, TQ administration ameliorated the levels of IFN-γ in rats with AD that pointing out the anti-inflammatory effect of TQ. In a previous study, it was observed that IFN-γ increases the Aβ uptake by microglia for synaptic integrity [Citation36]. It was also proposed that the enhancing effect on the IFN-γ levels might be as a result of the Th1 and NK cells activation [Citation31]. Therefore, we can suggest that TQ may show its anti-inflammatory activity by increasing the levels of IFN-γ for activation of microglia in the treatment of AD.
In addition to IFN-γ, other cytokines, including interleukins, TNF-α, and TGF-β, are actively participated in the pathogenesis of AD [Citation32]. In our model, there was no change in the levels of IL-1α, IL-1β, and TNF-α, while their concentrations increased in the AD patients [Citation37,Citation38]. It was previously suggested that TQ exhibited anti-inflammatory effects by decreasing several cytokines, including TNF-α, NF-κB, IL-6, IL-1β, and iNOS [Citation39,Citation40]. On the other hand, we noted that a significant negative correlation between IFN-γ and IL-1β in our AD model, suggesting that Aβ-related decreased level of the IFN-γ might increase the level of IL-1β. This result may be responsible for the well-known neuroinflammatory process in the AD as the activation of glial cells, and release of IL-1β contribute to neuronal dysfunction [Citation41]. In healthy conditions, it was shown that IFN-γ potentiates IL-1β release from human cells [Citation42]. In parallel to this finding, we observed a significant positive correlation between IFN-γ and IL-1β levels upon TQ treatment suggesting the TQ's ameliorative effect on the neuroinflammation. Previously, the anti-inflammatory effect of TQ also found as a decline of iNOS protein expression and attenuation of proinflammatory cytokines/chemokines levels [Citation40]. Therefore, both high levels of IFN-γ and positive correlation between the levels of IFN-γ and IL-1β may generate a TQ- dependent clearance of Aβ plaques in the AD.
Besides, we also noted a significant negative correlation between IL-1β and DCX, a neuronal migration protein and suggested an inverse relation between inflammation and neurogenesis. In a recent study, the changes in interleukin-1β (IL-1β) have been associated with cognitive dysfunction, and its increased concentration significantly decreased DCX positive cells in the hippocampus [Citation43]. Researchers have claimed that IL-1β can bind directly to neural precursors to cause cell cycle arrest in vitro [Citation44]. Besides, IL-1β indirectly activated microglia and astrocytes by recruiting peripheral leukocytes to invade the brain and reduce neurogenesis [Citation45,Citation46]. In the current study, we found that TQ treatment significantly increased the expression of DCX protein. This result confirmed our previous findings that TQ administration had a positive effect on the neurogenesis [Citation47]. As seen, it can be interpreted elevated neurogenesis in the hippocampus could be as a result of TQ-triggered protective effect against the pathophysiology of AD. Correspondingly, a study showed that Nigella sativa that contains TQ promoted neurite outgrowth, which is an essential event for neuro-regeneration [Citation48].
Interestingly, unlike DCX, another microtubule-associated protein, MAP-2, was significantly affected by Aβ infusion in our AD model, and TQ remained incapable of restoring this effect. MAP-2, a microtubule binding protein, aggregates and forms Alzheimer-type neurofibrillary tangles by altering neurofilamentous elements [Citation49]. Upregulation of MAP-2 is required for activity-dependent stabilization of new dendritic arbours [Citation50] In an in vitro retinal neuron culture, exposure to physiological amounts of Aβ1–42 for 24 h resulted in impairment to neuronal MAP-2 by transient downregulation [Citation51]. Also, the decrease in the MAP-2 protein varied depending on the type of plaque in AD. For example, in AD brains, MAP-2 protein was lower in the Aβ-positive dense-core plaques which are fibrillary deposits of Aβ showing all the classical properties of amyloid including beta-sheet secondary structure than diffuse plaques which are amorphous deposits [Citation52,Citation53]. These results show that Aβ infusion disrupts the cytoskeletal elements in the AD brain by worsening the neurodegeneration, and the regenerative activity of TQ can find no way out to fix this problem in our model.
Lastly, to observe the effect of TQ on the level of nuclear proteins, it was investigated the level of PARP protein, which has a role in nucleic acid metabolism, modulation of chromatin structure, DNA synthesis, and DNA repair [Citation54]. In our model, we observed a significant decrease in the activation of PARP and TQ treatment did not affect the activation of PARP. The decrease of the PARP activation could be related to the role of PARP in cell proliferation and cell death processes [Citation55]. In a study a reduced level, nucleolar immunohistochemical staining of PARP in hippocampal pyramidal cells was noted as a sensitive marker of functional changes on the postmortem hippocampus of individuals with AD [Citation56]. It may be suggested that memory deficiency in AD can be correlated to decrease in the level of this chromatin-remodelling enzyme (PARP) due to its role in synaptic plasticity and memory consolidation which was noted in both Aplysia and rodents [Citation57,Citation58]. Using nicotinamide adenine dinucleotide (NAD+), ribosylation by PARP forms branched ADP-ribose polymers on DNA polymerases, ligases, and histones and allows repair proteins and transcription factors to access the DNA by epigenetic modifications [Citation54]. However, a decrease in the activation of PARP resulted in the reduced expression of genes required for memory consolidation [Citation59].
In summary, in the current study, we observed a TQ-related functional recovery in our AD model by increasing neurogenesis and inhibiting neuroinflammation through amelioration of IFN-γ levels.
Acknowledgements
This study was supported by the grant from Bezmialem Vakif University Scientific Research Found (BVU-BAP) (12.2014/2).
Disclosure statement
No potential conflict of interest was reported by the authors.
ORCID
Birsen Elibol http://orcid.org/0000-0002-9462-0862
Sule Terzioglu-Usak http://orcid.org/0000-0002-4594-2697
Additional information
Funding
References
- Ransohoff RM. How neuroinflammation contributes to neurodegeneration. Science. 2016;353:777–783. doi: 10.1126/science.aag2590
- Cagnin A, Brooks DJ, Kennedy AM, et al. In vivo measurement of activated microglia in dementia. Lancet. 2001;358:461–467. doi: 10.1016/S0140-6736(01)05625-2
- Swardfager W, Lanctot K, Rothenburg L, et al. A meta-analysis of cytokines in Alzheimer’s disease. Biol Psychiatry. 2010;68:930–941. doi: 10.1016/j.biopsych.2010.06.012
- Sahbaz C, Zibandeyeh N, Kurtulmuş A, et al. Role of lymphocyte subsets and T-cell profiles in the immune dysfunction of sSchizophrenia. Biol Psychiatry. 2018;83:S331–S332. doi: 10.1016/j.biopsych.2018.02.853
- Su F, Bai F, Zhang Z. Inflammatory cytokines and Alzheimer’s disease: a review from the perspective of genetic polymorphisms. Neurosci Bull. 2016;32(5):469–480. doi: 10.1007/s12264-016-0055-4
- Karpenko MN, Vasilishina AA, Gromova EA, et al. Interleukin-1β, interleukin-1 receptor antagonist, interleukin-6, interleukin-10, and tumor necrosis factor-α levels in CSF and serum in relation to the clinical diversity of Parkinson’s disease. Cell Immunol. 2018;327:77–82. doi: 10.1016/j.cellimm.2018.02.011
- van Vliet EA, Aronica E, Vezzani A, et al. Review: neuroinflammatory pathways as treatment targets and biomarker candidates in epilepsy: emerging evidence from preclinical and clinical studies. Neuropathol Appl Neurobiol. 2018;44(1):91–111. doi: 10.1111/nan.12444
- Schmidt R, Hofer E, Bouwman FH, et al. EFNS-ENS/EAN Guideline on concomitant use of cholinesterase inhibitors and memantine in moderate to severe Alzheimer’s disease. Eur J Neurol. 2015;22:889–898. doi: 10.1111/ene.12707
- Riedel G, Bergman J, Vanderschuren L, et al. The behavioural pharmacology of dementia. Behav Pharmacol. 2017;27(2 and 3- Special Issue):91–93.
- Laver K, Dyer S, Whitehead C, et al. Interventions to delay functional decline in people with dementia: a systematic review of systematic reviews. BMJ Open. 2016;6(4):e010767. DOI:10.1136/bmjopen-2015-010767.
- Dariani S, Baluchnejadmojarad T, Roghani M. Thymoquinone attenuates astrogliosis, neurodegeneration, mossy fiber sprouting, and oxidative stress in a model of temporal lobe epilepsy. J Mol Neurosci. 2013;51:679–686. doi: 10.1007/s12031-013-0043-3
- Gokce EC, Kahveci R, Gokce A, et al. Neuroprotective effects of thymoquinone against spinal cord ischemia-reperfusion injury by attenuation of inflammation, oxidative stress, and apoptosis. J Neurosurg Spine. 2016;24:949–959. doi: 10.3171/2015.10.SPINE15612
- Ullah I, Ullah N, Naseer MI, et al. Neuroprotection with metformin and thymoquinone against ethanol-induced apoptotic neurodegeneration in prenatal rat cortical neurons. BMC Neurosci. 2012;13:11. doi: 10.1186/1471-2202-13-11
- Alhebshi AH, Gotoh M, Suzuki I. Thymoquinone protects cultured rat primary neurons against amyloid β-induced neurotoxicity. Biochem Biophys Res Commun. 2013;433(4):362–367. doi: 10.1016/j.bbrc.2012.11.139
- Bargi R, Asgharzadeh F, Beheshti F, et al. The effects of thymoquinone on hippocampal cytokine level, brain oxidative stress status and memory deficits induced by lipopolysaccharide in rats. Cytokine. 2017;96:173–184. doi: 10.1016/j.cyto.2017.04.015
- Dalli T, Beker M, Terzioglu-Usak S, et al. Thymoquinone activates MAPK pathway in hippocampus of streptozotocin-treated rat model. Biomed Pharmacother. 2018;99:391–401. doi: 10.1016/j.biopha.2018.01.047
- Terzioglu-Usak S, Negis Y, Karabulut DS, et al. Cellular model of Alzheimer’s disease: Aβ1–42 Peptide induces amyloid deposition and a decrease in topo isomerase IIβ and nurr1 expression. Curr Alzheimer Res. 2017;14(6):636–644. doi: 10.2174/1567205014666170117103217
- Paxinos G, Watson C. The rat brain in stereotaxiccoordinates. San Diego: Academic Press; 1998.
- Vorobyov V, Kaptsov V, Gordon R, et al. Neuroprotective effects of hydrated fullerene C60: cortical and hippocampal EEG interplay in an amyloid-infused rat model of Alzheimer’s disease. J Alzheimers Dis. 2015;45:217–233. doi: 10.3233/JAD-142469
- Barnhart CD, Yang D, Lein PJ, et al. Using the Morris water maze to assess spatial learning and memory in weanling mice. PLoS One. 2015;10(4):e0124521. DOI:10.1371/journal.pone.0124521.
- Cohen J. The analysis of variance. In: Statistical power analysis for the behavioral sciences. 2nd ed. Hillsdale (NJ): Lawrence Erlbaum Associates; 1988. p. 274–287.
- Yirmiya R, Goshen I. Immune modulation of learning, memory, neural plasticity and neurogenesis. Brain Behav. Immun. 2011;25(2):181–213. doi: 10.1016/j.bbi.2010.10.015
- Chesnokova V, Pechnick RN, Wawrowsky K. Chronic peripheral inflammation, hippocampal neurogenesis, and behavior. Brain Behav Immun. 2016;58:1–8. doi: 10.1016/j.bbi.2016.01.017
- Panche AN, Diwan AD, Chandra SR. Flavonoids: an overview. J Nutr Sci. 2016;5:e47. DOI:10.1017/jns.2016.41.
- Wang D, Hu M, Li X, et al. Design, synthesis, and evaluation of isoflavone analogs as multifunctional agents for the treatment of Alzheimer’s disease. Eur J Med Chem. 2019;168:207–220. DOI:10.1016/j.ejmech.2019.02.053.
- McNamara CE, Larsen L, Perry NB, et al. Anti-inflammatory sesquiterpene-quinones from the New Zealand sponge Dysidea cf. cristagalli. J Nat Prod. 2005;68:1431–1433. doi: 10.1021/np050171n
- Sirvanci-Yalabik M, Sehirli AÖ, Utkan T, et al. A Metabolite of arginine, improves learning and memory in streptozotocin-induced Alzheimer’s disease model in rats. Bull Clin Psychopharmacol. 2016;26(4):342–354. doi: 10.5455/bcp.20161121125642
- Noshita T, Murayama N, Nakamura S. Effect of nicotine on neuronal dysfunction induced by intracerebroventricular infusion of amyloid-β peptide in rats. Eur Rev Med Pharmacol Sci. 2015;19(2):334–343.
- Campolongo P, Ratano P, Ciotti MT, et al. Systemic administration of substance P recovers beta amyloid-induced cognitive deficits in rat: involvement of Kv potassium channels. PLoS One. 2013;8(11):e78036. doi: 10.1371/journal.pone.0078036
- Abdel-Zaher AO, Mostafa MG, Farghly HM, et al. Inhibition of brain oxidative stress and inducible nitric oxide synthase expression by thymoquinone attenuates the development of morphine tolerance and dependence in mice. Eur J Pharmacol. 2013;702:62–70. doi: 10.1016/j.ejphar.2013.01.036
- Majdalawieh AF, Fayyad MW. Immunomodulatory and anti-inflammatory action of Nigella sativa and thymoquinone: a comprehensive review. Int Immunopharmacol. 2015;28:295–304. doi: 10.1016/j.intimp.2015.06.023
- Zheng C, Zhou XW, Wang JZ. The dual roles of cytokines in Alzheimer's disease: update on interleukins, TNF-α, TGF-β and IFN-γ. Transl Neurodegener. 2016;5:7. doi: 10.1186/s40035-016-0054-4
- Tau G, Rothman P. Biologic functions of the IFN-gamma receptors. Allergy. 1999;54(12):1233–1251. doi: 10.1034/j.1398-9995.1999.00099.x
- Huchet R, Bruley-Rosset M, Mathiot C, et al. Involvement of IFN-gamma and transforming growth factor-beta in graft-vs-host reaction-associated immunosuppression. J Immunol. 1993;150:2517–2524.
- Baruch K, Rosenzweig N, Kertser A, et al. Breaking immune tolerance by targeting Foxp3(+) regulatory T cells mitigates Alzheimer’s disease pathology. Nat Commun. 2015 Aug 18;6:7967. doi: 10.1038/ncomms8967
- Monsonego A, Imitola J, Petrovic S, et al. Abeta-induced meningoencephalitis is IFN-gamma-dependent and is associated with T cell-dependent clearance of Abeta in a mouse model of Alzheimer’s disease. Proc Natl Acad Sci USA. 2006;103(13):5048–5053. doi: 10.1073/pnas.0506209103
- Italiani P, Puxeddu I, Napoletano S, et al. Circulating levels of IL-1 family cytokines and receptors in Alzheimer’s disease: new markers of disease progression? J Neuroinflammation. 2018;15(1):342. doi: 10.1186/s12974-018-1376-1
- Decourt B, Lahiri DK, Sabbagh MN. Targeting tumor necrosis factor alpha for Alzheimer’s disease. Curr Alzheimer Res. 2017;14(4):412–425.
- Velagapudi R, Kumar A, Bhatia HS, et al. Inhibition of neuroinflammation by thymoquinone requires activation of Nrf2/ARE signalling. Int Immunopharmacol. 2017;48:17–29. doi: 10.1016/j.intimp.2017.04.018
- Taka E, Mazzio EA, Goodman CB, et al. Anti-inflammatory effects of thymoquinone in activated BV-2 microglial cells. J Neuroimmunol. 2015;286:5–12. doi: 10.1016/j.jneuroim.2015.06.011
- Rai S, Kamat PK, Nath C, et al. Glial activation and post-synaptic neurotoxicity: the key events in Streptozotocin (ICV) induced memory impairment in rats. Pharmacol Biochem Behav. 2014;117:104–117. doi: 10.1016/j.pbb.2013.11.035
- Masters SL, Mielke LA, Cornish AL, et al. Regulation of interleukin-1beta by interferon-gamma is species specific, limited by suppressor of cytokine signalling 1 and influences interleukin-17 production. EMBO Rep. 2010;11(8):640–646. doi: 10.1038/embor.2010.93
- Lin Q, Shen F, Zhou Q, et al. Interleukin-1β Disturbs the Proliferation and Differentiation of Neural Precursor Cells in the Hippocampus via Activation of Notch Signaling in Postnatal Rats Exposed to Lipopolysaccharide. ACS Chem Neurosci. 2019;10(5):2560–2575. doi: 10.1021/acschemneuro.9b00051
- Koo JW. Duman RS IL-1beta is an essential mediator of the antineurogenic and anhedonic effects of stress. Proc Natl Acad Sci USA. 2008;105(2):751–756. doi: 10.1073/pnas.0708092105
- Wu MD, Hein AM, Moravan MJ, et al. Adult murine hippocampal neurogenesis is inhibited by sustained IL-1β and not rescued by voluntary running. Brain Behav Immun. 2012;26(2):292–300. doi: 10.1016/j.bbi.2011.09.012
- Wu MD, Montgomery SL, Rivera-Escalera F, et al. Sustained IL-1β expression impairs adult hippocampal neurogenesis independent of IL-1 signaling in nestin+ neural precursor cells. Brain Behav Immun. 2013;32:9–18. doi: 10.1016/j.bbi.2013.03.003
- Beker M, Dallı T, Elibol B. Thymoquinone can improve neuronal survival and promote neurogenesis in rat hippocampal neurons. Mol Nutr Food Res. 2018;62(5):1700768. doi: 10.1002/mnfr.201700768
- Doolaanea AA, Mansor N, Mohd Nor NH, et al. Co-encapsulation of Nigella sativa oil and plasmid DNA for enhanced gene therapy of Alzheimer’s disease. J Microencapsul. 2016;33(2):114–126. doi: 10.3109/02652048.2015.1134689
- Kosik KS, Duffy LK, Dowling MM, et al. Microtubule-associated protein 2: monoclonal antibodies demonstrate the selective incorporation of certain epitopes into Alzheimer neurofibrillary tangles. Proc Natl Acad Sci USA. 1984;81:7941–7945. doi: 10.1073/pnas.81.24.7941
- Vaillant AR, Zanassi P, Walsh GS, et al. Signaling mechanisms underlying reversible, activity-dependent dendrite formation. Neuron. 2002;34:985–998. doi: 10.1016/S0896-6273(02)00717-1
- Taylor-Walker G, Lynn SA, Keeling E, et al. The Alzheimer’s-related amyloid beta peptide is internalised by R28 neuroretinal cells and disrupts the microtubule associated protein 2 (MAP-2). Exp Eye Res. 2016;153:110–121. doi: 10.1016/j.exer.2016.10.013
- Rak M, Del Bigio MR, Mai S, et al. Dense-core and diffuse Abeta plaques in TgCRND8 mice studied with synchrotron FTIR microspectroscopy. Biopolymers. 2007;87(4):207–217. doi: 10.1002/bip.20820
- D'Andrea MR, Nagele RG. MAP-2 immunolabeling can distinguish diffuse from dense-core amyloid plaques in brains with Alzheimer’s disease. Biotech Histochem. 2002;77(2):95–103. doi: 10.1080/bih.77.2.95.103
- D'Amours D, Desnoyers S, D'Silva I, et al. Poly(ADP-ribosyl)ation reactions in the regulation of nuclear functions. Biochem J. 1999;342(Pt 2):249–268. doi: 10.1042/bj3420249
- Amé JC, Spenlehauer C, de Murcia G. The PARP superfamily. Bioessays. 2004;26(8):882–893. doi: 10.1002/bies.20085
- Zeng J, Libien J, Shaik F, et al. Nucleolar PARP-1 expression is decreased in Alzheimer’s disease: consequences for epigenetic regulation of rDNA and cognition. Neural Plast. 2016;2016:8987928. doi: 10.1155/2016/8987928
- Hernández AI W, Hu JY, et al. Poly-(ADP-ribose) polymerase-1 is necessary for long-term facilitation in Aplysia. J Neurosci. 2009;29(30):9553–9562. doi: 10.1523/JNEUROSCI.1512-09.2009
- Goldberg S, Visochek L, Giladi E, et al. PolyADP-ribosylation is required for long-term memory formation in mammals. J Neurochem. 2009;111(1):72–79. doi: 10.1111/j.1471-4159.2009.06296.x
- Fontán-Lozano Á, Suárez-Pereira I, Horrillo A, et al. Histone H1 poly[ADP]-ribosylation regulates the chromatin alterations required for learning consolidation. J Neurosci. 2010;30(40):13305–13313. doi: 10.1523/JNEUROSCI.3010-10.2010