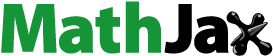
ABSTRACT
AISI 1045 steels are medium carbon steels used in applications that require greater strength and hardness such as gears, railway wheels and tracks, crankshafts, rolls, axles and other structural components. Although AISI 1045 steels are highly demanded engineering materials, they suffer from deformation and wear resistance when these applications are used. The hardness and wear resistance of the material are improved using a surface hardening method. Pack nitriding is the cheapest and easiest method of surface hardening method implemented in this work. The surface hardness of AISI 1045 steel is improved by the diffusion of nitrogen from the nitrogen-bearing material (melamine) to the surface of the steel and forms a metal nitride compound, which has strong hardness and wear resistance. This work reports that substantial surface hardening of AISI 1045 steel was achieved by the pack nitriding method using melamine obtained by in situ reaction of urea. The surface hardness increases manifold with the amount of melamine used, soaking time and temperature. The microscopic investigations of the nitrided surfaces of the metal show the formation of two distinct layers. The microstructural properties and hardness of the samples before and after nitriding were thoroughly investigated and interpreted.
Introduction
Surface hardening is a method of improving the hardness of the surface of metal objects by means of heat, thermochemical or mechanical methods. The main objective of surface/case hardening is to improve the surface properties of metals such as hardness, wear and corrosion resistance. Nowadays case hardening finds extensive applications in industries to increase the durability and strength of materials achieving new properties on the surface of the material [Citation1–4].
Hardness is an important term in materials’ mechanical properties. It prevents the material from scratches or indentation. The hardness of materials is directly related to tensile strength (resistance to deformation), fatigue limit, strength, coefficient of friction, abrasion and a host of other tribological properties [Citation5–8].
After the thermochemical surface hardening process, the surface of the metal objects becomes harder and forms a thin hard compound layer zone of 5–20 μm thickness and a diffusion zone next to the thin skin layer with 100s of μicrometer thickness [Citation9,Citation10]. Nitriding is one of the most commonly used thermochemical surface hardening [Citation11–15].
Nitriding is the diffusion of nitrogen to the surface of metals at a temperature between 500590°C. At this temperature range, steel remains in an α-ferrite phase. At the time of nitriding, the diffusion of nitrogen forms a hardened surface. The compound layer includes ϵ–Fe2-3N, γ′–Fe4N or a mixture of (ϵ+γ′). The compound layer with ϵ–Fe2-3N has good wear resistance, friction and corrosion resistance characteristics. The next layer to the compound layer is a diffusion zone, in which nitrogen diffuses interstitially to the steel and forms metallic precipitates with high fatigue resistance properties [Citation9,Citation10,Citation13,Citation16–21].
In the case of this work, the pack nitriding method, which is the cheapest (it does not require expensive equipment/machines for the processing method and ammonia gas) and nontoxic surface treatment used to harden AISI 1045 steel by packing the AISI 1045 steel samples in nitrogen-bearing materials (melamine) and heating to the ferritic temperature up to 590°C.
The melamine for nitriding is obtained by the thermal decomposition of urea, (NH2)2CO, which decomposes to ammonia and isocyanic acid at 350°C and subsequently to melamine, (NH2CN)3, at 400°C. Melamine, (NH2CN)3, on heating until the ferritic temperature liberates only nitrogen [Citation22–25].
(1)
(1) The nascent nitrogen from melamine is then diffused to the surface of the steel and reacts with the iron and other alloying elements in the steel to form metal nitrides, such as Fe2N, Fe3N and CrN, which are hard and resistant to wear.
Experimental procedures
Sample preparation
Round commercial AISI 1045 steel bars with a diameter of 26 mm (whose chemical composition is given in ) were collected from Burka Gibe Metal Manufacturing Company, Jimma, Ethiopia. The diameter was reduced to 8 mm using the lathe machine and then cut into smaller pieces of 4 mm height and subsequently polished using SiC emery papers from the coarsest 120 to the finest 2400. Then the samples were polished using 1.0, 0.5 and 0.1 μm of corundum or α-alumina (Al2O3) paste to create smooth and mirror-finish surfaces free of any scratches. For metallographic samples were etched with Nital (a mixture of 95% by weight of ethanol and 5% concentrated HNO3).
Table 1. Chemical composition of AISI 1045 (wt.%).
Nitriding treatment
The samples were immersed in a bed of melamine that is obtained by the thermal decomposition of urea at the temperature of 400°C at the rate of 10°C/min and kept at that temperature for 1 h. Melamine on further heating liberates nitrogen gas which will gradually diffuse to the surface of the steel to form metal nitride.
The prepared samples were buried and heated in a dry melamine powder inside a silica crucible kept in a stainless steel box sealed tightly with fire clay to prevent the leaking of gases during the heating process. The temperature is raised to 580°C with a heating rate of 5°C/min to decompose the melamine into nascent nitrogen. In this study, the chosen nitriding temperatures are 500, 520, 550 and 580°C. At each temperature, samples were soaked for 2, 3, 4 and –5 h.
Vickers microhardness
Vickers microhardness tester machine (HVs-50) connected with the optical lens was used to investigate the surface hardness of the samples. The load used to test samples in this research was 1 kg and the loading time of 15 s.
Microstructure
LH100-1 type optical microscope, having ScopeImage software, was used to investigate the microstructure with a magnification of 200×. Samples were cross-sectioned after nitriding to display the compound layer, diffusion zone and base metal on the computer screen.
X-ray diffraction
The X-ray diffractogram of each sample were recorded in a Drawell diffractometer (operating at 25 KV and 25 mA) in the range of 2θ = 20° to 85° with a step size of 0.01 degree using CuKα radiation before and after surface hardening (nitriding).
Results and discussions
Microstructure
The microstructures of the untreated () and treated () specimens were investigated using a metallurgical optical microscope.
The treated (nitride) specimen has two layers. The upper layer (the edge of the image) is called the compound layer and mainly contains epsilon iron nitrides and a small gamma prime. It is a white part of the layer. The next layer is called the diffusion layer. It contains precipitates of iron nitrides and other metallic nitrides such as titanium nitride, aluminum nitride and chromium nitride. These metallic nitrides in the compound layer and diffusion zone result in the increment of the hardness of the surface of the AISI 1045 steel samples.
X-ray diffraction
The phases in both untreated and treated (nitrided) samples were studied using the X-ray diffractometer (XRD 7000). γ'-Fe4N and ϵ-Fe2-3N phases were identified in the compound layer of the steel and metal nitride precipitates in the diffusion zone. New peaks that were not in the untreated AISI 1045 steel were observed in the nitrided (treated) AISI 1045 steels. These are epsilon iron nitride and gamma iron nitride (ϵ-Fe2-3N, Fe8N and γ'-Fe4N) peaks. Other metallic nitrides, such as Cr2N, CrN and AlN, may also be formed but most of their peaks could not be detected from the powder diffractogram. These metallic nitrides provide further surface hardness to the AISI 1045 steels. The XRD of untreated AISI 1045 is shown in and the nitrided AISI 1045 is in .
Experimentally observed hardness
The surface hardness of AISI 1045 increases from 238HV1 to 724HV1 with the increase in the nitrogen potential used (melamine) and the temperature. The nitrided surface hardness shows 204% increment to the untreated sample. This great increase in hardness is due to the presence of alloying elements such as Al, Mo and Cr in the AISI 1045 steel. These elements form metal nitride hard precipitates which give high strength and hardness to steels.
The hardness of the steel increases with the increase in the amount of melamine. Melamine shows a nitrogen potential for the nitriding process. An optimized hardness was observed at 20 g of melamine when it was treated at 590°C. The hardness before nitriding was 238HV1 and then the hardness rose with the increase in the amount of melamine until it reached optimum hardness. When the sample was treated with 550°C, an optimum hardness of 705HV1 was recorded. In both temperatures (550°C and 590°C) the hardness became nearly constant, right to 20 g of melamine.
The increment of the hardness is due to the formation of ϵ–Fe2-3N and other metallic nitrides. The amount of melamine and the temperatures were varying parameters in this experiment and time was held constant. The nitriding temperatures were 550°C and 590°C and time was held constant for 5 h in all experiments ().
Figure 5. Increase in hardness of AISI 1045 steel with the amount of melamine used and the temperature.

With the increasing in temperature in the range of ferritic temperature, the hardness of the samples increased due to the formation of a very thin compound layer dominated by ϵ–Fe2-3N. An increase in the ferritic temperature causes phase change and material distortion.
The effect of the soaking time during the surface hardening was also studied. Hardness increases with the increase in time.
To investigate the effect of soaking time on hardness, we used 20 g of melamine heat treated the samples at a temperature of 590°C and the time varied from 1 to 5 h. Holding the temperature constant at 590°C and varying the soaking time, the hardness increases from 238HV1 to 345HV1 at 1 h soaking time, 460HV1 at 2 h, 570HV1 at 3 h, 680HV1 at 4 h and 724HV1 at 5 h. With the increase in the soaking time more nitrogen diffuses interstitially to the surface of the sample and forms hard precipitates and metallic nitrides which result in an increment of surface hardness ().
The hardness decreases from the surface of materials as it goes to the inner core of the material. The hardness of nitrided steels decreases with an increase in the case depth explained by Hernandez et al. [Citation26]. The sample, which was nitrided at 590°C for 4 and 5 h, was studied for its hardness from its surface to the inner core of the samples. The hardness at the surface of the nitrided AISI 1045 steel at 590°C was 724HV1 and 680HV1 for 5 and 4 h soaking time, respectively. After the measurement of the surface hardness, the hardness differences of the sample at different depths were also measured. In the depth of 0.3 mm from the surface of the sample, the hardness as 660HV1 and 615HV1 for 5 and 4 h soaking time, respectively. In the depth of 0.8 mm from the surface of the sample, the hardness was 590HV1 and 525HV1 for 5 and 4 h, respectively. Then, in the depths of 1.2, 1.5 and 1.8 mm from the surface of the sample, the hardness was 478HV1 and 418HV1; 357HV1 and 306HV1; and 256HV1 and 243HV1 for 5 and 4 h soaking time, respectively ().
Conclusion
AISI 1045 steel is one of the most important metal alloys used in many applications such as gears, railway wheels, crankshafts, axles and structural components. One of the methods to improve the surface hardness of AISI 1045 steel is pack nitriding using melamine derived from urea. During pack nitriding of the steel two layers, called compound layer and diffusion zone, are generated. These two layers help to improve the mechanical properties of the steel. Soaking temperature, time and amount of melamine all have a great effect on the surface hardening of AISI 1045 steels. The hardness of the surface of the steel increases with the increase in all three parameters until it reaches optimum and then goes constant.
The hardness of the AISI 1045 steel samples decreases from the surface to the inner core of the sample. There is also a faster decrease of hardness in the diffusion zone than in the compound layer.
Disclosure statement
No potential conflict of interest was reported by the author(s).
References
- Davis JR. Surface hardening of steels: understanding the basics. Ohio: ASM International; 2002.
- Dossett J, Totten GE. Introduction to surface hardening of steels. In: ASM handbook. 4. Ohio: ASM International; 2013. p. 389–398.
- Bataev IA, et al. Surface hardening of steels with carbon by non-vacuum electron-beam processing. Surf Coat Technol. 2014; 242:164–169. doi:10.1016/j.surfcoat.2014.01.038
- Aghajani H, Behrangi S. Pulsed DC glow discharge plasma nitriding. In: Plasma nitriding of steels. Springer; 2017. p. 71–125.
- Callister Jr WD, Rethwisch DG. Callister's materials science and engineering. John Wiley & Sons; 2020.
- Pavlina EJ, Van Tyne CJ. Correlation of yield strength and tensile strength with hardness for steels. J Mater Eng Perform. 2008;17(6):888–893. doi:10.1007/s11665-008-9225-5
- Casagrande A, Cammarota GP, Micele L. Relationship between fatigue limit and Vickers hardness in steels. Mater Sci Eng A. 2011; 528(9):3468–3473. doi:10.1016/j.msea.2011.01.040
- Solis Romero J, Medina Flores A, Roblero Aguilar O. Tribological evaluation of plasma nitride H13 steel. Superficies y vacío. 2013; 26(4):131–138.
- Almeida EADSD, Costa CSED, Milan JLCSG. Study of the nitrided layer obtained by different nitriding methods. Matéria (Rio J). 2015; 20(2):460–465. doi:10.1590/S1517-707620150002.0046
- Aparecida dos Santos de Almeida E, Milan JLCSG, Edil da Costa CS. Acquired properties comparison of solid nitriding, gas nitriding and plasma nitriding in tool steels. Mater Res. 2015; 18(1):27–35. doi:10.1590/1516-1439.255513
- Wang X. Activated atmosphere case hardening of steels. Worcester Polytechnic Institute; 2011.
- Zhang C. Assessment of depth of case-hardening in steel rods by electromagnetic methods. Iowa: Iowa State University; 2009.
- Romanenko DN, Kolmykov VI, Ermolaev DV, et al. Increasing of fatigue limit and efficiency of constructional steels through additional chemical and thermal treating using nitrogenous carbonaceous carburizers. J Chem Technol Metall. 2017; 52(5):990–995.
- Agarwal PK. Thermo-chemical surface hardening treatment of steels. Jamshedpur: NML; 1994.
- Akhtar SS, Arif AFM, Yilbas BS. Influence of multiple nitriding on the case hardening of H13 tool steel: experimental and numerical investigation. Int J Adv Manuf Technol. 2012; 58(1):57–70.
- Somers MAJ. Nitriding and nitrocarburizing: status and future challenges. In: Proceedings of Heat Treat & Surface Engineering Conference & Expo, Chennai, India; 2013.
- Somers MAJ, Mittemeijer EJ. Formation and growth of compound layer on nitrocarburizing iron: kinetics and microstructural evolution. Surf Eng. 1987;3(2):123–137. doi:10.1179/sur.1987.3.2.123
- Calliari I, et al. Microstructure of a nitrided steel previously decarburized. J Mater Eng Perform. 2006;15(6):693–698. doi:10.1361/105994906X150740
- Ortiz-Domínguez M, et al. Analysis of nitride layers on ARMCO pure iron: the powder-pack nitriding process. Microsc Microanal. 2018; 24(S1):1076–1077. doi:10.1017/S143192761800586X
- Li KY, Xiang ZD. Increasing surface hardness of austenitic stainless steels by pack nitriding process. Surf Coat Technol. 2010; 204(14):2268–2272.
- Ito A, et al. Fabrication of high-entropy alloy CrMnFeCoNi with bimodal structure and its fatigue crack propagation properties バイモーダル構造を有するハイエントロピー合金CrMnFeCoNiの創製とその疲労き裂伝ぱ特性. J Soc Mater Sci Jpn. 2022; 71:961–968. doi:10.2472/jsms.71.961
- Bernhard AM, et al. Hydrolysis and thermolysis of urea and its decomposition byproducts biuret, cyanuric acid and melamine over anatase TiO2. Appl Catal B. 2012; 115:129–137.
- Yim SD, Kim SJ, Baik JH, et al. Decomposition of urea into NH3 for the SCR process. Ind Eng Chem Res. 2004;43(16):4856–4863. doi:10.1021/ie034052j
- Wynne AM. The thermal decomposition of urea: an undergraduate thermal analysis experiment. J Chem Educ. 1987;64(2):180. doi:10.1021/ed064p180
- Costa L, Camino G. Thermal behaviour of melamine. J Therm Anal. 1988;34(2):423–429. doi:10.1007/BF01913181
- Hernandez M, Staia MH, Puchi-Cabrera ES. Evaluation of microstructure and mechanical properties of nitrided steels. Surf Coat Technol. 2008;202(10):1935–1943. doi:10.1016/j.surfcoat.2007.08.018