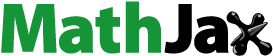
ABSTRACT
Farmers increasingly have to navigate through a complex web of agricultural and environmental regulations. In the EU, for example, the Nitrate Directive aims to control the nutrient levels in the water and agri-environmental schemes aim to protect habitats and increase biodiversity. The trade-offs between such environmental policies are not clear. Therefore, this paper studies the interaction between manure policy, especially derogation policy, and meadow bird schemes for the Netherlands. We develop a mathematical programming farm model with nine representative dairy farm types, based on location and economic size, that gain utility from both profit and agri-environmental services production. We show that the abolishment of derogation and the introduction of a low-protein feeding measure – both aimed at reducing nitrogen emissions – reduce total land contracted for meadow birds. This is because (1) without derogation makes farms switch to more maize production, hence reducing grassland, and (2) the protein measure requires extra feed production on-farm which most farms cannot accomplish and which makes contracting for the meadow bird scheme less attractive. Especially farms on peat and more intensive farms, who are more dependent on buying feed, reduce the amount of land contracted. We advocate that policy makers should become aware of how policies interact and that researchers do not study them in isolation.
1. Introduction
Farmers increasingly have to navigate through a complex web of agricultural and environmental regulations. Farmers have to comply with regulations that are concerned with water quality, nitrogen and phosphate emissions, wildlife habitats and animal welfare (Schils et al., Citation2007). Many of these regulations follow from EU policy and the international Climate Agreement, which are then translated into national policies. For example, in the Netherlands national manure and nutrient policies are grounded in the EU Nitrate Directive (EC/91/676) (Bouma, Citation2011; RVO, Citation2020). These policies include manure and nutrient application limits. An important aspect herein is derogation, which permits Dutch farmers to use more nitrogen (i.e. apply more manure to their land) if they adhere to stricter management rules (RVO, Citationn.d.).
Moreover, in the EU Common Agricultural Policy Pillar II so-called agri-environmental schemes (AES) are used to subsidise farms to engage in nature conservation and habitat creation (Bignal & McCracken, Citation2000; EC, 2005). AES can also be used by member states to mitigate climate change (Franks, Citation2011). In the Netherlands the meadow bird scheme, which is concerned with creating habitat for meadow birds, is the most relevant scheme for Dutch dairy farms (Westerink et al., Citation2015).
Policy evaluation is usually done in isolation. Little is known about how environmental policies interact and what the interlinkages are with other policies (e.g. De W. D. Vries, Citation2020). However, if one set of measures offsets the effects of another set, this will lead to disappointing environmental outcomes and misjudgements on their potential effect.
We mention three studies in the context of Dutch dairy farms that are close to what we intend to do in this paper. Peerlings and Polman (Citation2009) investigate the interaction of two types of agri-environmental contracts with Dutch manure policies. They find that both the AES contracts and stricter nitrogen application limits increase nitrogen emissions and reduce feed production on the dairy farm. In a similar study, Peerlings and Polman (Citation2008) conclude that the opportunity cost of AES contracting is lower under stricter manure application limits. Both studies assume profit maximisation ignoring alternative farmers’ objectives. Yet, agricultural studies have pointed out that farmers weigh multiple objectives that may contradict each other (Wallace & Moss, Citation2002). Groeneveld et al. (Citation2019) model the implementation of AES, via spatially interconnected mathematical programming models of Dutch dairy farms, using endogenous biodiversity goal weights in the objective functions of individual farms. However, they do not explicitly focus on the interaction between manure policies and AES.
Our objective is to analyse the interaction of the Dutch manure and AES policy measures for the Dutch dairy sector. We specifically study the trade-offs between the Dutch nitrate derogation (hereafter: derogation) and the meadow bird scheme policy, and the impact on farm income and agri-environmental services production. To reach the objective we develop a mathematical programming farm model wherein three farm size types have the possibility to produce milk and agri-environmental services for the meadow bird scheme. The model includes the phosphate and nitrogen cycles of the dairy farm. Herein we follow Peerlings and Polman (Citation2008), except that we assume that farmers have multiple objectives so that agri-environmental services production does not only depend on income but also on farmers’ preferences for producing these services. The weights in the multiple objective function are endogenously determined using a procedure developed by Manos et al. (Citation2009) and applied by Groeneveld et al. (Citation2019). Data for the model are farm data for three average farm sizes, which are further disaggregated based on soil type to take into account regional differences in application limits. This paper contributes to the literature by explicitly focusing on policy interaction in the context of Dutch manure policy and AES, taking into account multiple objectives of farmers.
The rest of the paper is organised as follows. First in section 2 we explain the two Dutch environmental policies that we examine in this paper. In section 3 the data are outlined. Section 4 describes the mathematical programming farm model. In section 5 we present the policy scenarios and results. Finally, a general discussion and conclusions are given in section 6.
2. Dutch environmental policies
In the Netherlands agri-environmental policy aims to counteract problems stemming from agricultural production, such as environmental pollution, climate change and health issues. In this section we describe the Dutch manure and AES policies.
2.1. Dutch manure policy
The EU Nitrate Directive (91/676/EC) and EU Water Framework Directive (2000/60/EC) aim to reduce the negative effects from agriculture on the environment by limiting the nutrient concentrations in soil and surface water, especially that of nitrogen (N) and phosphate (P2O5) (EC, Citation1991; RVO, Citation2020). These directives are translated into national policy by each member state. In the Netherlands since 1986 manure policy is described in the Fertiliser Nutrient Act (MSW, Dutch: Meststoffenwet). The MSW is complex, we introduce some of the main measures next. Discussed are national ceilings for nitrogen and phosphate emissions, derogation, application limits, compulsory manure removal, phosphate rights and additional measures.
2.1.1. National ceilings
The MSW prescribes national ceilings for nitrogen and phosphate emissions from livestock manure. These are 172.9 million kg phosphate and 504.4 million kg nitrogen for all “farm” animals in the Netherlands as a whole in 2021. For dairy this is 84.9 million kg phosphate and 281.8 million kg nitrogen. For pigs 39.7 million kg phosphate and 99.1 million kg nitrogen and for poultry this is 27.4 million kg phosphate and 60.3 million kg nitrogen.
2.1.2. Derogation
Derogation means that Dutch farmers can use a higher nitrogen application limit for grazing livestock manure as an exception from EU policy. Derogation is important for the Netherlands given its large dairy herd and long growing season (Schröder et al., Citation2007). Without derogation farms will have a larger manure surplus and possibly smaller grass yield (Henkens & Van Keulen, Citation2001; Koeijer et al., Citation2017; Schröder et al., Citation2007). To get derogation the EU prescribes additional measures. The most important one is industry-specific production ceilings for nitrogen and phosphate, for the agricultural sector these are the aggregate values mentioned above. Other conditions are that at least 80% of the farm is grassland, the farmer has to write a manure management plan, and no synthetic phosphate fertiliser may be used. However, because of EU concerns about the height of the Dutch nitrogen emissions the Dutch action programme with respect to the Nitrate Directive has been rejected, thus derogation will be phased out by 2026 (RVO, Citationn.d.).
2.1.3. Application limits
Application limits were introduced in 2006 to reduce nitrate and phosphate leaching from the agricultural sector into ground and surface water (Blanken et al., Citation2019). In the MSW, three manure application limits are dictated per annum. First, a nitrogen limit for grazing cattle manure of 170 kg N per hectare (on both grassland and arable land). In case of derogation, the application limit for nitrogen from grazing livestock manure is 230 (in regions with high livestock density, the so-called surplus regions) or 250 kg per hectare on grassland. Second, a nitrogen limit for all types of fertiliser that varies per crop and soil type. Finally, a phosphate limit for all fertilisers of 75 kg per hectare for grassland and 40 kg per hectare for arable land. However, in some cases limits can differ, e.g., in the case of high phosphate concentration in the soil the application norms are lower.
2.1.4. Manure surplus and removal
Because of the application limits, not all Dutch farms can apply all the manure they produce to their land. Farms are obliged to remove this manure surplus from their farm. To calculate the farm’s manure surplus, one has to calculate both the production and allowance based on the nitrogen and phosphate content of manure. For manure production per livestock type the government provides standard coefficients (RVO, Citationn.d.). To calculate the amount of phosphate fertiliser allowed to be used on the farmland, the so-called phosphate allowance, the number of hectares per crop are multiplied by the corresponding phosphate application limits per crop and then summed for all crops. For the allowance of nitrogen one must:
(1) calculate the nitrogen allowance from grazing livestock manure, based on the application limit of 170 kg N per hectare without derogation and 230 or 250 kg N per hectare with derogation;
(2) calculate the nitrogen allowance from all fertilisers, based on the application limit per hectare that varies with the crop and soil type (e.g. sandy or clay soils);
(3) calculate the actual application, taking into account gasification.
If the outcome of (2) is larger than (1), the farm can apply the full allowance of livestock manure to the land and additional organic or artificial fertilisers may be used up to the allowance as calculated under (2). However, when the allowance for all fertilisers is lower than that of livestock manure, the amount of livestock manure that can be applied is restricted to this allowance and no additional fertiliser can be used. Besides, not all nitrogen ends up in the soil and crops because of gaseous nitrogen losses from animal housing and manure storage, through a process called denitrification (3) (Schröder et al., Citation2007). For this reason total application is not equal to the actual or effective application. To calculate the amount of nitrogen that is effectively applied, working coefficients are available for any fertiliser type. For example, for manure from grazing dairy cows the working value is 45%, so the coefficient is 0.45, but for chemical fertiliser the working is 100%, so the coefficient is 1.0. The Dutch government has listed these coefficients on its website for farmers to use (RVO, Citationn.d.). Once the manure surpluses are known (i.e. production minus allowance), either the one based on phosphate or nitrogen is larger. An amount of manure corresponding to the larger surplus has to be removed from the farm. In this way either nitrogen or phosphate is most restrictive for the farm. Removal costs vary by region and manure type. Manure can be transported to e.g. arable farms, exported or processed. Processing is compulsory for dairy farms that are expanding or that already have a relatively large surplus. For monitoring streams of nutrients, farmers and transporters have to employ a bookkeeping system.
2.1.5. Additional measures
After abolition of the Dutch milk quota system in 2015, some additional policies have been introduced. The Dairy Act policy aimed to limit phosphate production on expanding dairy farms, to ensure that phosphate production stays under the national ceiling as required from the derogation policy (Klootwijk et al., Citation2016). The Integrated Approach Nitrogen policy was introduced to regulate nitrogen emissions from agriculture and the construction sector in the vicinity of Natura 2000 areas. It included measures on animal housing, feed and management, and manure application techniques (De Heer et al., Citation2017). However, the policy has not fulfiled the standard as set by the European Habitats Directive (EU, Citation1992). Therefore the Netherlands experiences a nitrogen crisis. To reduce nitrogen emissions further, the Ministry of Agriculture has to formulate additional environmental measures (for more details see Appendix A).
Furthermore, a system of phosphate rights was implemented in 2018. A dairy farm is not allowed to produce more phosphate than the number of rights it holds. These rights were assigned on the basis of farm size, soil conditions, and phosphate emissions from cattle. The phosphate rights correspond to the amount of livestock manure, expressed in kg phosphate, that can be produced yearly. The phosphate rights trading system has resulted in a quota system for dairy cattle. Farmers have to pay for additional rights to increase their herd and trade is allowed between farmers (RVO, Citationn.d.).
2.2. Dutch agri-environmental schemes
As part of the second pillar of the EU’s CAP, AES entails contracts between individual farmers who are the producers of environmental services and the government who pays for these services (OECD, 2013). In the Netherlands the contracts are collectively concluded by farmer collectives (i.e. farmer groups) with the provincial government (Terwan et al., Citation2016).
The largest share of Dutch grassland is owned by the agricultural sector, specifically the dairy sector. The open grassland found on Dutch dairy farms is an important breeding and feeding habitat for meadow bird populations. This makes the Netherlands of international importance for the preservation of these bird species (Kleijn & Sutherland, Citation2003). For this reason the meadow bird scheme is one of the main types of AES in the Netherlands. The scheme consists of several measures that entail varying degrees of “light” or “heavy” packages (). Farmers can choose among the various packages according to what suits their business best. The meadow bird management focuses on drainage, manure application, resting periods, differential heights in vegetation, extensive grazing, and herb-rich meadows. These packages can also be combined. For example, a farm can adopt the measure “resting period until 15th June” and adopt thereafter on the same plot the “solid manure” measure. Since heavy measures are needed for creating a suitable bird habitat, coordinators of meadow bird schemes stimulate farmers to adopt these types of measures (Van der Pol, Citation2021). Heavy management restricts farm activities and reduces the grass yield – particularly the farm loses a major part of the first grass’ harvest that is rich in protein. Moreover, for some heavy packages it holds that the application of nitrogen fertiliser is restricted to these plots. In particular, the application of slurry is forbidden and the application of solid manure (manure from the stable that contains straw and no urine) is restricted (NFW, Citation2016 Van der Pol, Citation2021). These conditions interact with the manure application limits as found in the MSW.
Table 1. Dutch meadow bird scheme measures.
3. Data
Data needed to calibrate the model come from various sources and largely reflect the year 2018. Data on output and input prices as well as on technical relations concerning the production of milk, feed and cattle are from the handbook “Quantitative Information Livestock Sector” (Blanken et al., Citation2019). Furthermore, we used for each simulated farm the nitrogen and phosphate content of grass, maize and feed bought from Blanken et al. (Citation2019) and Wageningen Economic Research (Citation2020, May 31), nitrogen and phosphorus in milk and cattle from Netherlands Enterprise Agency (Citation2020; RVO, Citation2018), labour requirements per cow and per hectare of grassland and maize from Meetjesland (Citation2016), labour hours for maintaining one hectare contracted under the meadow bird scheme from Remmelink et al. (Citation2019). Additionally, we made assumptions on some farm characteristics such as the farming system (). The fixed cost for AES contracting is the collective fee, which is 35 euro annually. Grass yield loss stemming from the AES is estimated at 19% of total energy from all harvests combined (referred to as VEM, a Dutch energy parameter), based on Louis Bolk Institute (Citation2019) and interviews with three dairy farmers. The AES payment is taken from the price list of a Friesian farmer collective (NFW, Citation2016). lists some of the data for the model parameters (for a complete list see Appendix B).
Table 2. Technical coefficients that are the same for all farms.
Specific data on three representative Dutch dairy farms (small, medium and large) is taken from Vrolijk et al. (Citation2020) (). The Agrimatie database is used to create three dairy farm classes based on economic size measured in 1,000 standard output (kSO) in the following way: small between 0–300 kSO, medium 300–600 kSO, and large>600 kSO. The three farm sizes differ amongst others in the mean number of dairy cows and current production plan, including the average amount of AES hectares contracted. Average family labour hours for each farm type were obtained from Agrimatie (Wageningen Economic Research, Citation2020, May 31), because this was missing in Vrolijk et al. (Citation2020).
Table 3. Three representative farms.
Finally, the weights of the goals vary between farms. Weights attached to the production of agri-environmental services are derived from the current production plan, where we assume that the estimated weights reflect the preference for producing for the meadow bird scheme. We formulate a triangular distribution for each farm from which the weights are drawn in such a way that the average weight from these draws is equal to the estimated weight (last row, ). The distribution form is
with mode
, and minimum and maximum values
and
. We know that a farmer’s preference for producing environmental services can be zero at least, therefore
. The formula for the mean of the distribution is
. Using the estimated weight
and assuming the mean is equal to the mode,
can be calculated. Because the estimated weights
are low, this means the weights drawn will often be low. By using Monte Carlo simulations, we vary the preferences for the consumption of agri-environmental services versus farm income.
4. The farm model
We use a mathematical programming farm model to analyse farm decision making in light of manure and agri-environmental policies. The theoretical model, the farm’s optimisation problem, is given in Appendix C. We model a stylised Dutch dairy farm in which the most important mechanisms related to the topic of this paper are included, without claiming it is a full representation a real farm. Next, we describe some of the main elements of the model: the assumed farm behaviour, the utility function with goal weights, feed requirements, labour and land constraints, the limits to the number of cows, and phosphate and nitrogen constraints. A complete overview of the farm model can be found in Appendix D.
4.1. Farms
We consider utility maximising farmers (farm sizes small, medium, large), who gain utility from profit and possibly the production of agri-environmental services and who make decisions based on one year. Within Dutch regions, farms differ in soil type (peat, sand, clay) and thus in the nitrogen application limits for all fertiliser types. Thus, we have in total nine farms. The farms earn income from milk production, the selling of cows, the selling of maize, and possibly agri-environmental services production for the meadow bird scheme through hectares contracted. This means that one hectare of grassland simultaneously generates income through milk production and agri-environmental services production.
In the model the farms can choose to contract land under a heavy package from the meadow bird scheme, which consists of postponing the mowing date and reducing manure application. In that case, the farm loses part of the first grass’ harvest and can make up for this loss by buying additional feed. Moreover, in case of a manure surplus excess manure has to be transported off-farm. When a farm enters the meadow bird scheme it is faced with (i) variable (yearly) costs, depending on the measures carried out per hectare, and (ii) fixed costs, which consist of one-off costs related to creating the natural space needed for the meadow birds and other investment costs. The variable costs lower the net price that the farm receives per hectare contracted, while the fixed costs lower the farm’s profit. From a farmer collective representative we know that these fixed costs are largely non-existent because those plots that are contracted are suited already, and if otherwise the collective reimburses any adjustments that have to be made (Velde Vinken, Citation2021). Thus, the only fixed cost remaining is the yearly membership fee to the collective.
4.2. Utility function with goal weights
The farm has two main objectives or goals, that is the maximisation of profit ( and the maximisation of the consumption of agri-environmental services (
measured in meadow bird scheme hectares contracted), but these objectives may not be weighted equally. By using the farms’ actual production plan and Goal Programming, we are able to estimate these weights for each farm
’s’ utility function (Manos et al., Citation2009). In this way the weight of objective
is endogenously determined (for details see Appendix E). The derived goal weights are used in the model simulations. For the small, medium and large farm size these are 0.06, 0.028 and 0.017, respectively.
4.3. Feed requirements
The farms produce roughage in the form of maize silage and grass when they are on sandy or clay soil. The farms who are on peat do not grow maize. The average yields per hectare of maize and grass are assumed fixed. Dairy cattle need a certain amount of energy (VEM) and protein (DVE) to stay healthy, to grow and to produce milk – expressed per calf, heifer (young cattle>1 year) and dairy cow respectively. Therefore on the farm the total VEM and DVE required per calf, heifer and dairy cow needs to be equal to the VEM and DVE produced on-farm as well as additional VEM and DVE that the farm buys. Furthermore, for each animal there is a ratio of VEM from roughage and from feed concentrates required, so the farm is restricted in what source of feed it can give to each animal (Blanken et al., Citation2019). The ratio of roughage to concentrates for calves is 90:10, for heifers 100:0 and for dairy cows 80:20 (G. D. Vries, Citationn.d.; Remmelink et al., Citation2019). Moreover, the surplus or deficit of roughage (maize) can be sold or bought respectively, although these amounts are restricted to 375 kilo VEM per cow to reflect real market conditions.
4.4. Labour and land constraints
The labour used for maintaining the cows, maize, grassland and agri-environmental services should be equal to the available labour on the farm plus any hired labour. Land used for production of maize and grassland cannot exceed the amount of available arable land and grassland. The total amount of land is assumed fixed. It is common practice that dairy farms on peat land do not grow maize, because the soil does not allow for a long growing season (and hence yields will be low). When we run simulations for the farms on peat land, the restriction is added that the area of grassland is equal to the total land.
4.5. Limits to the number of cows
There is a limit to the number of cows that can be held on the farm due to capacity constraints and phosphate rights. Calves are born at a certain rate per cow and replace the older cows (which are sold). The farm can also buy new cows. The total new cows on the farm equals the newly bought and newly born cows. The total cows on the farm equals the initial number of cows plus new cows minus sold cows.
4.6. Phosphate and nitrogen constraints
We model the phosphate and nitrogen cycle on the farm. Dairy cattle is fed with grass, maize and concentrated feed, which amongst others contain phosphate and nitrogen that are partly stored in the cattle. The cows produce milk and excrete phosphate and nitrogen. Milk leaves the farm. Grazing livestock manure (usually slurry) is used on-farm as fertiliser. Phosphate, nitrogen and other nutrients from the slurry are absorbed by the soil and crops (grass and maize). These crops are used to feed the dairy cattle – and the circle is complete. However, according to government rule farms cannot apply more phosphate on maize and grassland than 40 and 75 kg per hectare respectively (i.e. phosphate from manure). In addition, the nitrogen limits for grazing cattle manure are 170 kg per hectare and for all categories of manure 250 kg N per hectare on grassland and 140 kg N per hectare of maize in the case of sandy soil (for the other soil types this is higher, see Appendix B). When farms make use of derogation, the nitrogen application limit for grazing cattle manure is 250 or 230 (instead of 170) kg per hectare, but the farm has to have at least 80% grassland. Yet, the crops may need more nitrogen than the grazing cattle manure limit allows. In this case the farm can use chemical fertiliser, which adds costs. If the amount of manure produced exceeds either the phosphate or nitrogen limits, the farm pays for the removal of the surplus (Blanken et al., Citation2019). These are additional costs that the farmer wants to keep at a minimum.
Finally, other restrictions are that decision variables such as land allocated to grass production are nonzero. In sum, the base model includes i) the current agri-environmental policy, ii) the nitrogen and phosphate application limits that make up the total manure allowances, and iii) the derogation policy, which implies a 250 kg N limit per ha of grazing cattle manure and a minimum requirement of 80% grassland. Furthermore, the nitrogen application limits for all fertiliser types are included to reflect different soil conditions on the farm (peat, sand, clay) and the goal weights are varied using Monte Carlo simulations per farm to see the impact of different preferences for producing agri-environmental services. Thus, simulations are done for 3 farm sizes, 3 soil types and 5 goal weights making in total 3 × 3 * 5 = 45 artificial farms (). We use the software package GAMS to run the model simulations (Rosenthal, Citation2017).
5. Scenarios and results
Given our objective to understand the interaction between manure and agri-environmental policies, we formulated besides the base scenario two additional scenarios. We discuss these scenarios first and the results next. In addition, we performed a sensitivity analysis for the base model.
5.1. Scenarios
5.1.1. Scenario I
In the base model we assumed that all farms make use of the derogation policy. In Scenario I we simulate derogation abolishment. Therefore, the nitrogen limit for grazing cattle manure becomes 170 kg per hectare and we remove the restriction of minimum 80% grassland. In reality, dairy farms can convert temporary grassland into arable land (for growing maize) and vice versa but not permanent grassland. However, we do not know the average amount of permanent grassland for the three farm size types, and so a distinction between temporary and permanent grassland is not made in the model (in the sense that each farm has one decision variable for grassland). However, we do know that the total area of permanent grassland in the Netherlands is not allowed to decrease by more than 5% compared to the reference year 2012 - when it was 40.97% (RVO, Citationn.d.). If this limit is exceeded, the government will introduce a ban to switch from permanent to temporal grassland or arable land and an obligation to restore permanent grassland. Therefore, for Scenario I the share of grassland for each farm is set to be at least 40%. In this way the national average will certainly not exceed the −5% limit.
5.1.2. Scenario II. Low-protein feeding
One nitrogen-reducing measure that the Dutch Ministry considers, is to reduce the raw protein content of feed for dairy cattle. This will directly reduce the production of nitrogen and hence emissions. However, it is possible that due to less protein intake the cow’s productivity will decrease and milk composition will change (Mutsvangwa et al., Citation2016). In the literature low-protein feeding of dairy cows is reported to result in a 6 to 11% lower milk production (e.g. Frank & Swensson, Citation2002; Nielsen et al., Citation2003), although some authors also conclude that there is no loss in production if the feeding strategy is better finetuned (Børsting et al., Citation2003; Frank et al., Citation2002; Jonker et al., Citation2002). In the model we have not included the relationship between protein intake and milk production, but we can simulate the effect of a 20% nitrogen reduction in feed concentrates. We do this by reducing the amount of feed concentrates that can be bought by 20%.
5.2. Results
5.2.1. Base scenario
In the base scenario the farms produce milk, grass and maize and contract between 7% and 51% of the grassland for the meadow bird scheme, depending on farm size and soil type (, for detailed results see Appendix F). Even when preferences for agri-environmental services are zero or low, all farms participate in the AES. So, a profit can be gained by participating, independent of the farm size and soil type. In the case that simulated preferences for agri-environmental services are above 0.09, farms on clay and sand contract all the grassland (). Farms on peat also increase contracted land, but this stays at 16%, 32% or 53% of total grassland for the large, medium or small farms respectively. Farms on peat solely depend on grassland for roughage to feed the cattle. It appears that the grass yield loss due to AES contracting limits these farms in entering more land in the meadow bird scheme, because this loss needs to be compensated by buying feed.
Figure 2. Role of the weight for agri-environmental services (base model). (a) Farms on sand and clay, (b) Farms on peat.

Table 4. Nitrogen allowance.
Table 5. Outcomes scenarios versus base model, for soil types sand and clay (shown for weights .
With respect to the economic size of the farm, we observe that small farms on peat are more inclined to producing agri-environmental services. This is because these farms are more extensive – the number of dairy cows per ha is only 1.5 (versus 2.1 and 2.5 for medium and large farms, respectively) and they require less feed. For farms on sand and clay we do not see a large difference between sizes regarding land contracted (between 31–35%). Furthermore, large farms have relatively higher marginal feed and manure removal costs per cow.
5.2.2. Scenario I : No derogation
The results of Scenario I, a switch to stricter nitrogen application limits but no 80% grassland, are identical for all farm sizes (). In sum, without derogation farms want to increase maize production. This is done by switching grassland into land for maize production, leading to a reduction in grassland contracted. However, all the remaining grassland is AES contracted. In the base scenario, total contracted land ranged between 56.3–164.8 hectares (for both sand and clay) and 30.9–55.3 hectares (peat). Without derogation, this amount remains 55.3 hectares for peat, but for sand and clay it has reduced to 82.4 hectares. This shows that nitrogen policies can have an opposite effect on contracting, less grassland leads to a reduction of land contracted but at the same time more of the remaining grassland is contracted.
Table 6. Outcomes scenarios versus base model, for peat (shown for weights .
Abolishing derogation results in farms on clay sand switching to 40% grassland (the minimum) and 60% maize land. Besides an increase in feed production, this modifies their manure allowance. This has two advantages. First, the cows excrete less nitrogen through their manure, by 14–22% less, because maize contains less protein, while it does contain more matter per hectare than grassland and is less labour-intensive (Hoogeveen et al., Citationn.d.). Second, maize needs less nitrogen to grow (it has a nitrogen application limit for all fertilisers of 140–160 kg per ha depending on soil type). The use of maize instead of grass, then, shrinks the allowance for all fertilisers so that it comes closer to the allowance for grazing livestock manure, leaving less room for applying chemical fertiliser (see for an example ). This means a reduction in the cost for chemical fertiliser (). Overall the effect on income of no derogation for farms on sand and clay is negligible.
The opposite effect happens on peat soils because they cannot switch to maize. No derogation widens the gap between the allowances. The farm has to apply less livestock manure and more chemical fertiliser (compare ). As a consequence farms on peat soils face higher manure removal and chemical fertiliser costs.
5.2.3. Scenario II : Low-protein feeding
When the nitrogen intake through feed concentrates is reduced by 20%, initially, running this scenario turned out to be infeasible for most farm types – meaning that these farms cannot comply with the protein policy through on-farm feed production. Therefore, in the model we relaxed the restriction on maize bought so that these farms at least can make up for the loss in energy from concentrates feed through buying maize instead. We also adjusted the protein requirement for the cattle accordingly, thereby ignoring that a protein deficit influences milk productivity. After these adjustments, the result is that farms tend to reduce the production of environmental services to almost zero (, last column). The reason is that contracting for the meadow bird scheme entails buying extra feed concentrates to make up for the grass yield loss. The low-protein feeding measure thus hits where it already hurts. Especially farms on peat, who are more dependent on buying feed, reduce the land contracted. The exception are small farms; since they are more extensive they still substantially produce agri-environmental services. Overall AES contracted land, summed for the three sizes, reduces due to the introduction of the protein measure. In the base model the small, medium and large farm types on peat contracted 16.8, 4.2 and 7.9 ha, respectively, in the AES. Summing over the three farm types gives 28.9 ha in total. In comparison to the base scenario, in scenario II in total on peat farms 12.0 ha is contracted, which is a decrease of 18.9 ha (−61%). Similarly for farms on sand and clay this is a decrease of 29.5 ha. and 26.8 ha (−48%), respectively. Furthermore, the role of preferences is less prominent in this scenario. A higher preference for producing agri-environmental services does not make farms increase the production of these services. The utility of maximising income is decisive in this case.
As aimed for by the protein policy, nitrogen production is reduced for most farms, which in turn reduces manure removal costs. The exception again are small farms on sand and clay, who increase nitrogen production. We give two explanations for this unexpected result. First, these farms still contract for the meadow bird scheme, which results in higher manure surplus. Second, these farms already did not buy a large amount of concentrates so the protein policy does not affect their buying much in the model. When farms exhibit relatively few dairy cows per unit of land and have the opportunity to grow maize, they can be more flexible by feeding the cows roughage produced on-farm.
Finally, farms have more profit in this scenario because they have reduced feed and manure removal costs. This is partly an artificial outcome, since we allowed farms in this scenario to exchange more expensive concentrates for buying cheaper maize. Also, concerns related to milk production and health due to a changed energy and protein intake remain out of the scope of this research. It is possible that due to less protein intake the cow’s productivity will decrease and milk composition will change, resulting in less production (Mutsvangwa et al., Citation2016). For example, low-protein feeding of dairy cows is reported to result in 6–11% lower milk production (Frank & Swensson, Citation2002; Nielsen et al., Citation2003). Thus, it must be borne in mind that any profit gain may be offset due to a reduction in the value of milk produced.
5.3. Sensitivity analyses
Additional simulations were carried out to check the robustness of the base model outcomes with respect to AES contracting. The following conditions were varied: i) increased manure removal costs, ii) increased feed price, and iii) lower and higher milk price. First, manure removal costs were increased from the initial 12 euro up to 36 euro per 1000 kg. In Dutch regions where manure surplus is low, costs are around 12 euro per 1000 kg. In contrast, other regions that experience high surplus face costs of 22–24 euro per 1000 kg (Blanken et al., Citation2019). When the pressure on the manure market increases (a likely scenario in case of derogation), manure removal costs will probably increase beyond 24 euro per 1000 kg. The base model results are the same with increased manure removal costs with respect to AES contracting, but farms with low and medium preferences (up to around 0.2) decrease land contracted as the manure removal costs increase. Thus, the opportunity cost of AES contracting increases.
Second, the feed concentrates price was raised by 20% up to 150%. Not surprisingly, participation in the meadow bird scheme decreases when the price is increased by 40–60%. This effect is larger for farms with a low preference and for those that depend more on buying feed. If feed prices increase (e.g. due to war in Ukraine), the AES compensation has to increase to prevent a drop in participation.
Third, milk prices were drawn eight times from a normal distribution with mean milk price 36.85 per 100 kg and standard deviation 3.82 (calculated from Blanken et al. (Citation2019)). The outcomes remain robust with respect to AES contracting despite changes in milk price.
6. Discussion and conclusions
Farmers face multiple environmental regulations, which are concerned with reducing negative externalities from agriculture and also with nature conservation and biodiversity. However, these policies are not always aligned. The Dutch manure policies, for example, may interact with the contract terms as found in the Dutch AES policy, leading to suboptimal outcomes (Lastra-Bravo et al., Citation2015). This paper investigates this interaction. We simulated the following policy scenarios in the context of Dutch dairy farms: a base model with current Dutch manure policy including derogation and a package from the meadow bird scheme, a scenario without Dutch derogation, and a scenario introducing a low-protein feeding measure. We used multiple-objective optimisation and simulated preferences for producing agri-environmental services by using Monte Carlo simulations.
We discuss some caveats related to our analysis. First, because we formulated a linear model the optimal outcomes jump from one (extreme) solution to another. For example, if farms have a slight preference for producing agri-environmental services, 100% of the land is selected for the meadow bird scheme, while in reality much less land is entered. More realistic solutions can be attained with a nonlinear model, such as by formulating a log function for yield loss to reflect that the more the mowing of the grass is prolonged the larger the loss in protein or energy. Second, due to lack of data we included average farm types in our analysis. Using more “real” farms could have resulted in more realistic outcomes, we did not capture the large heterogeneity of farms out there. For example, not all farms apply for derogation as is assumed in the base model. Third, we looked at the interaction of certain aspects of manure and environmental policies but these policies are more diverse than we simulate. For example, we ignored the variety in agri-environmental contracts, the effects of grazing, etc. Moreover, effects of less protein in feed on milk production and the effect of derogation on soil fertility were not taken into account. Finally, we ignored long-term effects in the model. Total land, capital and the number of cattle are fixed. Despite these caveats the model presented remains a flexible tool to investigate various aspects of the interaction between manure and agri-environmental policies. Moreover, the linear model can serve as a basis to build more complex models and to compare the outcomes of several model scenarios.
We recap our findings. In the base scenario all farms participated in the meadow bird scheme, regardless of their economic size. The endogenous goal weights for agri-environmental services, which we calculated from the actual production plans of three average Dutch dairy farm sizes, appear to be low, suggesting that for farmers agri-environmental services are especially important as an income source and less so for environmental reasons. The possibility to grow maize for additional feed seems important to offset the decrease in grass yield due to AES contracting. In Scenario I without derogation the farms adjusted their production plan: they switched to more maize production and entered all of the remaining grassland under the meadow bird scheme. The latter is in line with the finding of Peerlings and Polman (Citation2008, p. 186) that “stricter manure policies increase contracting because they reduce the opportunity cost of contracting”. With this strategy, the farms can better deal with the lower nitrogen limits, thus reducing manure surplus (measured in nitrogen). Farms that cannot adjust the feeding strategy, such as farms on peat in our model, are the losers here. We conclude that the abolishment of derogation does not make the meadow bird scheme less attractive on grassland to the farm. However, the total amount of grassland and, therefore, land entered for the meadow bird scheme falls due to a switch to maize. Thus, the present 80% permanent grassland criterion for derogation is especially important for meadow birds but negatively affects the needed flexibility to switch to feed crops like maize to deal with stricter environmental regulations.
Finally, scenario II has shown that the low-protein feeding measure requires extra feed production on-farm. This makes AES contracting less attractive. Moreover, it is questionable whether farms are able to deal with this policy in the first place without a fundamental change in the way they farm. In conclusion, the policy succeeds in reducing nitrogen emissions but it reduces the production of agri-environmental services.
A general conclusion that can be drawn from this paper is that policy makers should be aware of how policies interact to better understand their outcomes and researchers should be careful not to study policies in isolation.
Acknowledgments
This work was supported by the European Union’s Horizon 2020 research and Innovation programme under Grant N°817903.
Disclosure statement
No potential conflict of interest was reported by the authors.
Additional information
Funding
References
- Bignal, E. M., & McCracken, D. I. (2000). The nature conservation value of European traditional farming systems. Environmental Reviews, 8(3), 149–39. https://doi.org/10.1139/a00-009
- Blanken, K., Buisonje, F., Evers, A., Ouweltjes, W., Van Schooten, H., Verkaik, J., Vermeij, I., & Wemmenhove, H. (2019). KWIN ‘Quantitative Information Livestock Sector’. Wageningen Livestock Research.
- Børsting, C. F., Kristensen, T., Misciattelli, L., Hvelplund, T., & Weisbjerg, M. R. (2003). Reducing nitrogen surplus from dairy farms. Effects of feeding and management. Livestock Production Science, 83, 165–178. https://doi.org/10.1016/S0301-6226(03)00099-X
- Bouma, J. (2011). Applying indicators, threshold values and proxies in environmental legislation: A case study for Dutch dairy farming. Environmental Science & Policy, 14(3), 231–238. https://doi.org/10.1016/j.envsci.2010.12.001
- EC. (1991). Directive 91/676/EEC Concerning the protection of water against the pollution caused by nitrates from agricultural sources. Brussels Official Journal of the European Communities, 34(L), 375.
- EU, (1992). Council directive 92/43/EEC of 21 May 1992 on the conservation of natural habitats and of wild fauna and flora. OJ L 206, 22.7.1992, p. 7–50.
- Frank, B., & Swensson, C. (2002). Relationship Between Content of Crude Protein in Rations for Dairy Cows and Milk Yield, Concentration of Urea in Milk and Ammonia Emissions. Journal of Dairy Science, 85(7), 1829–1838. https://doi.org/10.3168/jds.S0022-0302(02)74257-4
- Frank, B., Persson, M., & Gustafsson, G. (2002). Feeding dairy cows for decreased ammonia emission. Livestock Production Science, 76, 171–179. https://doi.org/10.1016/S0301-6226(02)00021-0
- Franks, J. R. (2011). The collective provision of environmental goods: A discussion of contractual issues. Journal of Environmental Planning and Management, 54(5), 637–660. https://doi.org/10.1080/09640568.2010.526380
- Groeneveld, A. N., Peerlings, J. H. M., Bakker, M. M., Polman, N. B. P., & Heijman, W. J. M. (2019). Effects on participation and biodiversity of reforming the implementation of agri-environmental schemes in the Netherlands. Ecological Complexity, 40(2019), 1–17. https://doi.org/10.1016/j.ecocom.2018.08.003
- Heer, M. D., Roozen, F., & Maas, R. (2017). The Integrated Approach to Nitrogen in the Netherlands: A preliminary review from a societal, scientific, juridical and practical perspective. Journal for Nature Conservation, 35(2017), 101–111. https://doi.org/10.1016/j.jnc.2016.11.006
- Henkens, P. L. C. M., & Van Keulen, H. (2001). Mineral policy in the Netherlands and nitrate policy within the European Community. NJAS: Wageningen Journal of Life Sciences, 49(2–3), 117–134. https://doi.org/10.1016/S1573-5214(01)80002-6
- Hoogeveen, M., Daatselaar, C., & Prins, H., (n.d.). Fall derogation: Exploration size and manager’s motivations (Dutch: Afname derogatie: Verkenning omvang en beweegredenen ondernemers.) Retrieved August 21, 2020, from https://edepot.wur.nl/494727.
- Jonker, J. S., Kohn, R. A., & High, J. (2002). Dairy Herd Management Practices that Impact Nitrogen Utilization Efficiency. Journal of Dairy Science, 85, 1218–1226. https://doi.org/10.3168/jds.S0022-0302(02)74185-4
- Kleijn, D., & Sutherland, W. J. (2003). How effective are European agri-environment schemes in conserving and promoting biodiversity? The Journal of Applied Ecology, 40(6), 947–969. https://doi.org/10.1111/j.1365-2664.2003.00868.x
- Klootwijk, C. W., van Middelaar, C. E., Berentsen, P. B. M., & de Boer, I. J. M. (2016). Dutch dairy farms after milk quota abolition: Economic and environmental consequences of a new manure policy. Journal of Dairy Science, 99(10), 8384–8396. https://doi.org/10.3168/jds.2015-10781
- Koeijer, T. J., de Luesink, H. H., & Prins, H., (2017). Herd size, manure production, manure market and costs manure disposal; Evaluation Fertiliser Nutrient Act 2016 (Dutch: Dieraantallen, mestproductie, mestmarkt en kosten mestafzet; Evaluatie Meststoffenwet 2016). Wageningen Economic Research, Wageningen University and Research. Retrieved June 7, 2021, from https://doi.org/10.18174/407574.
- Lastra-Bravo, X. B., Hubbard, C., Garrod, G., & Tolón-Becerra, A. (2015). What drives farmers’ participation in EU agri-environmental schemes?: Results from a qualitative meta-analysis. Environmental Science & Policy, 54, 1–9. https://doi.org/10.1016/j.envsci.2015.06.002
- Louis Bolk Institute, (2019). Voorweiden voor vogels (Report V-focus October 2019). Louis Bolk Instituut. Retrieved August 1, 2020, from https://louis-bolk.nl/publicaties/voorweiden-voor-weidevogels
- Manos, B., Papathanasiou, J., Bournaris, T., Paparrizou, A., & Arabatzis, G. (2009). Simulation of impacts of irrigated agriculture on income, employment and environment. Operational Research, 9(3), 251–266. https://doi.org/10.1007/s12351-008-0030-6
- Meetjesland, (2016). Labour efficiency at dairy farms (Dutch: Arbeidsefficiëntie op melkveebedrijven). Brugse Ommeland and Meetjesland. Retrieved April 15, 2020, from https://docplayer.nl/14841808-Arbeidsefficientie-op-melkveebedrijven.html.
- Mutsvangwa, T., Davies, K. L., McKinnon, J. J., & Christensen, D. A. (2016). Effects of dietary crude protein and rumen-degradable protein concentrations on urea recycling, nitrogen balance, omasal nutrient flow, and milk production in dairy cows. Journal of Dairy Science, 99(8), 6298–6310. https://doi.org/10.3168/jds.2016-10917
- NFW, (2016). Management packages and prices for open grassland. Farmer collective Noardlike Fryske Wâlden, the Netherlands. Retrieved May 8, 2020, from https://www.noardlikefryskewalden.nl/wp-content/uploads/2016/10/Tarieven-Beheerpakketten-ANLb2016-Open-Grasland.pdf.
- Nielsen, N. M., Kristensen, T., Nørgaard, P., & Hansen, H. (2003). The effect of low protein supplementation to dairy cows grazing clover grass during half of the day. Livestock Production Science, 81, 293–306. https://doi.org/10.1016/S0301-6226(02)00229-4
- Peerlings, J. H. M., & Polman, N. B. P. (2008). Agri-environmental contracting of Dutch dairy farms: The role of manure policies and the occurrence of lock-in. European Review of Agricultural Economics, 34(2), 167–191. https://doi.org/10.1093/erae/jbn022
- Peerlings, J. H. M., & Polman, N. B. P. (2009). Farm choice between agri-environmental contracts in the European Union. Journal of Environmental Planning and Management, 52(5), 593–612. https://doi.org/10.1080/09640560902958131
- Pol, B. van der, (2021). Bird management coordinator affiliated with farmer collective Noardlike Fryske Wâlden, personal communication, 15 January, 2021.
- Remmelink, G., Van Middelkoop, J., Ouweltjes, W., & Wemmenhove, H. (2019). Guide Dairy Sector (Dutch: Handboek Melkveehouderij) 2019-2020. Wageningen Livestock Research.
- Rosenthal, R. E., (2017). GAMS a user’s guide. Retrieved August 11, 2020, from https://www.scribd.com/document/360403453/GAMSUsersGuide-pdf
- RVO, (2018). Guide company-specific excretion dairy cattle. The Netherlands Enterprise Agency, version 2010.(Dutch: Handreiking bedrijfsspecifieke excretie melkvee, Rijksdienst voor Ondernemend Nederland). Retrieved April 15, 2020, from http://www.rvo.nl/file/handreiking-bedrijfsspecifieke-excretie-melkvee/ ( 2010).
- RVO, (2020). Guide company-specific excretion dairy cattle. The Netherlands Enterprise Agency, version 2018. (Dutch: Handreiking bedrijfsspecifieke excretie melkvee, Rijksdienst voor Ondernemend Nederland). Retrieved April 15, 2020, from http://www.rvo.nl/file/handreiking-bedrijfsspecifieke-excretie-melkvee ( 2019).
- RVO, (n.d.). The Netherlands Enterprise Agency (executive agency for agricultural policy of the Dutch Ministry of Agriculture, Nature and Food quality). https://english.rvo.nl/Onmanurepolicy;https://www.rvo.nl/onderwerpen/agrarisch-ondernemen/mest.Onnitrogenlimits;https://www.rvo.nl/sites/default/files/2018/03/Tabel-1-Stikstofgebruiksnormen-2018.pdfOnworkingcoeffcients;https://www.rvo.nl/sites/default/files/2015/04/Tabel%203%20Werkingsco%C3%ABffici%C3%ABnt%20dierlijk%20e%20andere%20organische%20meststoffen%202014-20171.pdfOnpermanentgrassland;https://www.rvo.nl/subsidie-en-financieringswijzer/vergroeningsbetaling/blijvend-grasland;https://www.rvo.nl/onderwerpen/agrarisch-ondernemen/mest/tabellen
- Schils, R. L. M., de Haan, M. H. A., Hemmer, J. G. A., van den Pol, A., de Boer, J. A., Evers, A. G., Holshof, G., van Middelkoop, J. C., & Zom, R. L. G. (2007). DairyWise, a Whole Farm Dairy Model. Journal of Dairy Science, 90, 5334–5346. https://doi.org/10.3168/jds.2006-842
- Schröder, J. J., Aarts, H. F. M., van Middelkoop, J. C., Schils, R. L. M., Velthof, G. L., Fraters, B., & Willems, W. J. (2007). Permissible manure and fertiliser use in dairy farming systems on sandy soils in the Netherlands to comply with the Nitrates Directive target. European Journal of Agronomy, 27, 102–114.
- Terwan, P., Deelen, J. G., Mulders, A., & Peeters, E., (2016). The cooperative approach under the new Dutch agri-environment-climate scheme. Background, procedures and legal and institutional implications. Ministry of Economic Affairs: The Hague, The Netherlands. Retrieved August 2, 2021, from https://enrd.ec.europa.eu/sites/default/files/w12_collective-approach_nl.pdf
- Velde Vinken, E. V. D., (2021). Coordinator from farmer collective Noardlike Fryske Wâlden, personal communication, March. 2021.
- Vries, G. D., (n.d.). What does the cow want on her plate (Dutch: Aan tafel! Wat wil de koe op haar bord?). https://edepot.wur.nl/504583.
- Vries, W. D., (2020). Building blocks for new nitrogen policy (Dutch: Bouwstenen voor nieuw stikstofbeleid). Magazine Milieu, April. 2020. Retrieved from https://www.wur.nl/upload_mm/8/8/7/6c616079-61b6-4a8b-91fd-b5544e1babe7_De%20Vries%202020%20Milieu%20%282%29%20Dossier%2037-43.pdf.
- Vrolijk, H., Reijs, J., & Dijkshoorn Dekker, M., (2020). Towards sustainable and circular farming in the Netherlands; Lessons from the socio-economic perspective. Wageningen Economic Research. Retrieved from: https://research.wur.nl/en/publications/towards-sustainable-and-circular-farming-in-the-netherlands-lesso.
- Wallace, M. T., & Moss, J. E. (2002). Farmer Decision-Making with Conflicting Goals: A Recursive Strategic Programming Analysis. Journal of Agricultural Economics, 53(1), 82–100.
- Wageningen Economic Research, (2020, May 31). Dutch FADN, dairy sector 2018. Wageningen Economic Research. Retrieved May 31, 2020, from https://www.agrimatie.nl/binternet.aspx?ID=4&bedrijfstype=2.
- Westerink, J., Melman, D. C. P., & Schrijver, R. A. M. (2015). Scale and self-governance in agri-environment schemes: Experiences with two alternative approaches in the Netherlands. Journal of Environmental Planning and Management, 58(8), 1490–1508. https://doi.org/10.1080/09640568.2014.932762
Appendix A:
Additional nitrogen policies
The European Habitats Directive (Directive 92/43/EEG) aims to stop biodiversity loss in the EU through the network of protected European nature areas called Natura-2000 (EU, 1992; EC, 2011). In July 2015 an additional Dutch nitrogen policy called Integrated Approach Nitrogen (PAS, Dutch: Programma Aanpak Stikstof), was introduced to regulate nitrogen emissions from agriculture and the construction sector in the neighbourhood of Natura-2000 areas (de Heer et al., 2017). In May 2019 the Dutch Council of State concluded that the PAS policy was not up to the standard as set by the European Habitats Directive. This was the beginning of the Dutch nitrogen crisis. The regulations from the PAS have not prevented a too high level of nitrogen deposition, in the form of compounds such as ammonia (NH3) and nitrogen oxide (NOx), in about three quarters of Dutch nature areas, causing eutrophication and acidification (Wamelink et al., 2013, Gies et al., 2019, de Vries, 2020). The verdict of the Council of State implies many economic consequences for the Dutch industry, agricultural sector, traffic and transport (Gies et al., 2019). To reduce nitrogen emissions, the Ministry of Agriculture considers additional environmental measures to be implemented, such as reducing the raw protein content of feed for dairy cattle, buying up farms in the neighbourhood of Natura-2000 areas, and additional stable measures preventing emissions. The reserved budget for nitrogen reducing measures as well as nature conservation and restoration measures is 25 billion euro until 2035 (Dutch Ministry of Agriculture, 2019, 2022a, 2022b). Since these measures are largely aimed at lowering ammonia deposits and the dairy sector is the main contributor of ammonia emissions within the agricultural sector, this means that especially dairy farmers will be faced with additional policies (Sutton et al., 2015; Gies et al., 2019; de Vries, 2020).
References
Dutch Ministry of Agriculture, (2019). Questions and answers nitrogen (policy document). Retrieved from: https://www.rijksoverheid.nl/documenten/publicaties/2019/10/04/vragen-en-antwoorden-over-stikstof
Dutch Ministry of Agriculture, (2022a). Approach regarding the nitrogen crisis (government letter). Retrieved from: https://www.rijksoverheid.nl/documenten/kamerstukken/2022/04/01/hoofdlijnen-van-de-gecombineerde-aanpak-van-natuur-water-en-klimaat-in-het-landelijk-gebied-en-van-het-bredere-stikstofbeleid
Dutch Ministry of Agriculture, (2022b). Memorandum national programme rural area (government letter), 24-04-2020. Retrieved from: https://www.rijksoverheid.nl/documenten/kamerstukken/2022/06/10/startnotitie-nationaal-programma-landelijk-gebied
EC. (1991). Directive 91/676/EEC Concerning the protection of water against the pollution caused by nitrates from agricultural sources. Brussels Official Journal of the European Communities, 34(L), 375.
EU, (1992). Council directive 92/43/EEC of 21 May 1992 on the conservation of natural habitats and of wild fauna and flora. OJ L 206, 22.7.1992, p. 7–50.
Gies, E., Kros, H., & Voogd, J. C., (2019). Insights nitrogen deposits on nature (Dutch: Inzichten stikstofdepositie op natuur). Memo Insights Nitrogen (9 October. 2019). Wageningen Environmental Research, Wageningen. Retrieved from: https://www.wur.nl/upload_mm/9/7/b/0e3bba36-5a6f-46c7-b442-ef7d64ebd93c_inzichten-stikstofdepositie-op-natuur-wenr.pdf
Heer, M. D., Roozen, F., & Maas, R. (2017). The Integrated Approach to Nitrogen in the Netherlands: A preliminary review from a societal, scientific, juridical and practical perspective. Journal for Nature Conservation, 35(2017), 101–111. https://doi.org/10.1016/j.jnc.2016.11.006
Sutton, M. A., Dragosits, U., Geels, C., Gyldenkærne, S., Misselbrook, T. H., & Bussink, W., (2015). Review on the scientific underpinning of calculation of ammonia emission and deposition in the Netherlands. Retrieved July 1, 2020, from https://edepot.wur.nl/357694.
Vries, W. D., (2020). Building blocks for new nitrogen policy (Dutch: Bouwstenen voor nieuw stikstofbeleid). Magazine Milieu, April. 2020. Retrieved from https://www.wur.nl/upload_mm/8/8/7/6c616079-61b6-4a8b-91fd-b5544e1babe7_De%20Vries%202020%20Milieu%20%282%29%20Dossier%2037-43.pdf.
Wamelink, G. W. W., de Knegt, B., Pouwels, R., Schuiling, C., Wegman, R. M. A., & Schmidt, A. M.van Dobben, H. F., Sanders, M. E. (2013). Considerable environmental bottlenecks for species listed in the Habitats and Birds Directives in the Netherlands. Biological Conservation, 165(2013), 43–53. https://doi.org/10.1016/j.biocon.2013.05.012
Appendix B:
Data
Appendix C:
Theoretical model
This appendix describes a theoretical model of the farm’s optimisation problem, for which we assume that farm maximises utility based on the production of milk and the possible production (and consumption) of agri-environmental services by contracting hectares of grassland.
The farm’s utility maximisation problem
Farms weigh multiple goals or objectives in allocating their resources, with the ultimate aim to maximise utility (Wallace and Moss, 2002). The utility function belonging to this utility maximisation problem contains besides profit, several attributes (Robinson, 1982). Thus the farm’s maximisation problem in general form is:
with
the expected value operator (Gómez-Limón et al., 2004). In our model farm
maximises profit based on the production of two goods: milk,
(including the sale of livestock), and agri-environmental services from entering an AES contract,
. We assume that
is measured in hectares contracted. We further assume that the farm possibly gains utility from consuming agri-environmental services. Thus, the farm enjoys agri-environmental services even without receiving a compensation for it.
The farm ’s utility (
maximisation problem is defined as:
wherein the weights and
determine how much each objective is valued at farm
.
presents the utility function for profit of farm
and
the utility function of agri-environmental services of farm
. Furthermore,
is the profit of farm
. The weights are assumed to sum up to one and the separate utilities are scaled from zero to one, i.e. the utility function is additive and has nonlinear indifference curves (Hardaker et al., 2015). This form is chosen because some farmers may not derive utility from producing agri-environmental services. Moreover, the additive utility function has been shown to be representative for how people think about preferences and has been commonly applied to the modelling of farmers’ decision-making (Gómez-Limón et al., 2004).
Farm’s objective 1: profit
We start by formulating objective 1:
in which is a vector of the quantities of (quasi-)fixed inputs of farm
,
a price vector of variable inputs (including those for producing the agri-environmental services),
is total fixed costs of farm
for entering an AES contract, and
the payment from the AES subsidy. Quasi-fixed inputs are inputs that are fixed, e.g. capital and total land. Notice that the farmer is a price-taker.
The first-order conditions are:
or price equals marginal cost of dairy production, and
or the AES payment equals marginal cost of agri-environmental services production.
The first-order conditions (4) and (5) state that marginal cost equal marginal benefits. Therefore, only variable input prices and output prices influence decision-making. Moreover, AES participation is only attractive if it leads to an increase in profit.
Farm’s objective 2: consuming agri-environmental services
For objective 2 we assume that is increasing, continuous and quasiconcave in
, i.e. the farmer gains utility from consuming agri-environmental services and marginal utility diminishes as consumption increases. This utility is besides the utility derived from the profit earned by producing agri-environmental services.
References
Gómez-Limón, J. A., Riesgo, L., & Arriaza, M. (2004). Multi-Criteria Analysis of Input Use in Agriculture. Journal of Agricultural Economics, 55(3), 541–564. https://doi.org/10.1111/j.1477-9552.2004.tb00114.x
Hardaker, J. B., Lien, G., Anderson, J. R., & Huirne, R. (2015). Coping with Risk in Agriculture: Applied Decision Analysis (3rd ed.). CABI Publishing. https://doi.org/10.1079/9781780645742.0000
Robinson, L. J. (1982). An appraisal of expected utility hypothesis tests constructed from responses to hypothetical questions and experimental choices. American Journal of Agricultural Economics, 64(2), 367–375.
Wallace, M. T., & Moss, J. E. (2002). Farmer Decision-Making with Conflicting Goals: A Recursive Strategic Programming Analysis. Journal of Agricultural Economics, 53(1), 82–100.
Appendix D:
The farm model
The objective function to be optimised is the utility function in [1]. All farms gain utility from profit and possibly nature consumption, depending on how much weight is given to these objectives. New profit is divided by the initial profit (derived from the base model) to scale utility around 1. Production for the AES is scaled to 1 by dividing the amount of hectares contracted by all grassland. The weight is drawn from a triangular distribution of the form
. Using the average weight
that we calculated for each farm size
, wherein
= small, medium, or large farm type (and assuming the mean is equal to the mode
), the maximum value
can be constructed. When we calculate this, for example for farm size small with mean weight 0.06, we get
, hence the distribution to draw weights from is:
. Although this works nicely in theory, given that the calculated average weights are very low, the drawn weights (for the simulations) are also low. In a linear model this does not give many different outcomes. Therefore, when running the model we allowed as maximum value
, thus using the form
, to get more than 0.10 or 0.20 as value for
. The goal weights
and
together have to sum up to one [2].
subject to (1)
with corresponding to farm sizes small, medium and large, and
, corresponding to farm’s goal profit maximisation and maximisation of agri-environmental services production.
Equation [3] is the profit function, revenue minus all costs. Equation [4] calculates total milk production .
Limits to the number of cows. Calves and heifers are born or grow at a certain rate per dairy cow [6,7] and replace the older cows (which are sold). In the short run, it is common that farms do not buy cows but only replace the old dairy cows with new ones from the herd (heifers that have matured), thus will be zero. The total number of cows
then equals the initial number of cows,
, plus new cows minus sold cows,
[8]. Equation [5] states that the number of cows is equal to the farm’s capacity,
. This means the initial herd size on the farm is fixed in the model. (This restriction is made because we assume capacity is fixed in the short run. In addition, when we allow the number of cows to become smaller than the capacity, the optimisation outcome is to shrink the herd size by more than 50% - which is not a realistic outcome.) Equation [5] can be changed to read ‘equal or lower than’. In case the farm reduces its capacity, it will sell more old cows than are replaced. The number of sold cows is equal to [11]. EquationEquation [9
(9)
(9) ,Equation10
(10)
(10) ,Equation11]
(11)
(11) make sure that the extra sold cows are limited to 10% (c4).
Land and crops. The total land available to the farm is fixed. Therefore, the land used for production of maize and grassland cannot exceed this amount [12]. The derogation policy requires farms to have at least 80% grassland [13a]. However farms on peat only own grassland and thus their grassland equals total land [13b]. The average dairy farm grows maize for feed. In the model we set maize to be at least 15% of total land [14a], except for farms on peat, which cannot grow maize [14b]. Furthermore, for all farms it holds that the amount of land contracted for the meadow bird scheme,
, cannot exceed the amount of grassland available [15].
if farm’s soil is peat (13b)
if farm’s soil is peat (14b)
Labour. The total labour required for maintaining the cows, maize, grassland and agri-environmental services is summed in [16]. This should be equal to the available labour on the farm plus any hired labour [17].
Feed requirements. Cattle need feed in the form of roughage and concentrates for growth and health. Concentrates and additional roughage (maize) are bought on the market to supplement the cows’ dietary need. This need is expressed in the feed’s energy (VEM) and protein (DVE) content. EquationEquations [19](19)
(19) and Equation[20]
(20)
(20) express the total need of VEM and DVE respectively. These needs have to be equal to the VEM and DVE produced by the crops, as well as additional feed that is bought (concentrates and maize) [21-23]. In case the farm produces AE services the grass yield loss
needs to be calculated [18] so that it can be deducted from the total yield [21 and 23]. Moreover, each animal needs a certain minimum percentage of concentrates feed
[22], so the farmer is restricted in what source of feed can be given to which animal. Calves are fed at least 10% of concentrates and dairy cows at least 20% (Remmelink et al., 2017; De Vries, n.d.). Any additional maize bought,
, is then the difference between the on-farm production (average yield per ha are used) minus possible yield loss minus the minimum amount of concentrates feed needed [21]. EquationEquation [24
(24)
(24) and 25] make sure that the concentrates
bought meets the requirements. EquationEquation [25]
(25)
(25) states that the total amount of concentrates feed bought, expressed in VEM, has to at least meet the minimum share in the cows’ diet plus any protein deficit that may be present (according to [23]). To a certain extent maize can be a good part of the cows’ diet. However, the amount of maize bought is restricted to reflect the actual Dutch maize market [26].
Production and use of phosphate and nitrogen. Simply put, phosphate and nitrogen are fed to cows through their feed (grass, maize and concentrates) and partly are stored inside them and excreted via milk production. The remainder is excreted through their manure. Whether the farm has a surplus of manure, depends on the phosphate and nitrogen application limits. The surplus of phosphate and nitrogen is calculated by Equationequations [27(27)
(27) -Equation29]
(29)
(29) and Equation[30
(30)
(30) -Equation39]
(39)
(39) respectively.
The farm’s phosphate production [27] and the allowance for phosphate
[28] are compared to give the (possible) surplus of phosphate
[29]. To arrive at the nitrogen surplus more equations are needed. First, the difference between the nitrogen stored in the cows [30] and nitrogen produced from feed [31] gives the farm’s net nitrogen production [32]. Then this amount has to be compared to the allowance for nitrogen from grazing cattle manure and that for nitrogen from all fertilisers, which are calculated by [33a,b] and [34] respectively.
, with
corresponding to the soil types farms reside on (34)
The allowance for all nitrogen fertilisers is determined by the nitrogen application limits per soil type. Parameters
and
depend on the soil the farm resides on. Hence this equation implies that the model is solved multiple times for 3
3 (size
soil) farms. When the farm chooses to adopt heavy measures for the meadow bird scheme, for which nitrogen application is restricted, this reduces the allowance
[33b]. In the case other measures or none is adopted, there is no such restriction and Equationequation [33a]
(33a)
(33a) should be used instead. Furthermore, the nitrogen that the farm applies to the land
cannot exceed the amount produced on the farm [35], nor the two nitrogen allowances [36,37]. The nitrogen surplus is determined by the difference between nitrogen production and the nitrogen allowances, depending on whichever of these is restrictive [38,39].
Chemical fertiliser and manure surplus removal. When the farm still has allowance for all fertilisers (i.e. this allowance is larger than that for grazing livestock manure), synthetic nitrogen fertiliser can be applied to the land [40]. This is equal to the allowance of grazing cattle manure minus applied manure multiplied by a working coefficient. Finally, the manure surplus that has to be removed from the farm is translated into 1000 kilograms manure. The amount
depends on whether the nitrogen [41] or phosphate surplus [42] is the larger one. Finally other restrictions are that inputs should be positive.
, if
(40)
Scenarios I and II
For scenario I, no derogation, Equationequation [13a](13a)
(13a) is replaced by [13c] and the nitrogen limit of grazing cattle manure, parameter
, is set to 170. Furthermore the amount of maize farms can sell on the market is restricted [43], because they are foremost dairy farms and thus producing maize cannot be a major source of income.
(43)
For scenario II, the low-protein feeding measure, Equationequations [19](19)
(19) and Equation[20]
(20)
(20) were set to ‘greater or equal to’ and [22] to be exactly ‘equal to’ and the coefficients c41, c42, c51, c52 and c53 were reduced by 20%.
EquationEquation [26](26)
(26) on the amount of maize bought on the market,
, was changed for the farm types as follows:
, so for small farms only, (26a)
, for medium farms on sand and clay, (26b)
, for medium farms on peat, (26c)
, for large farms on sand and clay, (26d)
, for large farms on peat (26e)
These numbers were chosen on the basis of permitting farms to make up for the lost amount of energy from concentrates feed so as to still meet cattle energy need (i.e. to not run into model errors).
Appendix E:
Estimating weights using Goal Programming
The steps are outlined for estimating the weights for each farm ’s’ utility function according to Manos et al. (2009).
Step 1: The first step is to determine a pay-off matrix with rows (attributes) and columns
(the objectives) based on the optimisation of each one of the two objectives. We run the farm model with the following objective function:
whereby each one of the two farmer’s goals is optimised (and the other goal is not), by setting the weight of this goal in the objective to 1, while we set the other to 0. When we have run the farm model with the farm’s weight solely on profit, , or on nature,
, we save the obtained data for the payoff matrix. Thus
is the value of the
-th attribute when the
-th objective (j = 1,2) is optimised. In our case h = 1,2, namely farm income in euros and the number of hectares contracted under the AES. In this way we get a 2 by 2 pay-off matrix for each farm
The diagonal of the pay-off matrix then gives the optimal values for each of the two objectives, while each column represents the optimal production plan when objective
is preferred.
Step 2: Farm ’s current production plan
and its corresponding payoff matrix can be used to estimate the weights attached to each goal. The average amount of land contracted under the AES per farm type is known for the year 2020,
, but we do not have the farm’s average profit. We do know however the average allocation of grass and maize, the number of cows, and average milk production in 2020. We use this information to calculate current profit
of farm
, with the farm model that includes the dairy farm’s technical constraints and as objective function profit maximisation.
The production plan is now complete for all farm types. Therefore, the pay-off matrices can be used to estimate a set of weights
that reflect the farms’ preferences. Typically, when using linear optimisation the pay-off matrix will have in the first column (only preference for profit maximization) zero hectares contracted for nature consumption and in the second column (only preference for nature consumption) all land contracted for nature consumption. Hence, it will look something akin to:
.
For each farm we solve a Goal Programming (GP) model whereby the objective is to minimise the positive and negative deviations from each goal. Based on Manos et al. (2009) the GP model is:
and
where is the weight that is attached to each objective
of farm
,
are the elements of the pay-off matrix (estimated in the previous step),
is the value that is achieved for the
-th attribute according to the farm’s current production plan,
represents the positive deviation from the
-th objective and
the negative deviation. The first constraint implies that the pay-off of each goal weighted by the farm plus or minus deviations from that goal value has to be equal to the real production value. The other constraint implies that the weights
are normalised (they have to sum up to 1). In this way, by solving the GP model, the weight of objective
is endogenously determined. The derived weights are used in the model simulations.
Appendix F:
Model outcomes
The results of the simulated scenarios are given for selected variables; in for farms on sand and clay and for farms on peat.
Table A1. Base Scenario for farms on sand and clay, for weights .
Table A2. Base Scenario for farms on sand and clay, for weights .
Table A3. Scenario I No derogation for farms on sand and clay (all weights).
Table A4. Scenario II Low-protein feeding for farms on sand and clay (all weights).
Table A5. Base scenario for farms on peat, for weights .
Table A6. Base scenario for farms on peat, for weights .
Table A7. Base scenario for farms on peat, for weights .
Table A8. Base scenario for farms on peat, for weights .
Table A9. Scenario I no derogation for farms on Peat (all weights).
Table A10. Scenario II low-protein feeding for farms on peat (all weights).