ABSTRACT
Background
Macroautophagy/autophagy is an essential recycling process that is involved in a wide range of biological functions as well as in diseases. The regulation of autophagy by exercise and the associated health benefits have been revealed by rodent studies over the past decade, but the evidence from human studies remains inconclusive.
Methods
The MEDLINE, Embase, Cochrane, Scopus, and Web of Science databases were systematically searched from inception until September 2022. Human studies that explored potential effects of physical exercise on autophagy at the protein level were selected according to the Preferred Reporting Items for Systematic Reviews and Meta-Analyses guidelines. A random-effects model was used for the meta-analysis.
Results
Twenty-six studies were included in the meta-analysis. Subgroup analyses revealed that an acute bout of resistance exercise attenuated autophagy, as characterized by lower levels of microtubule-associated proteins 1A/1B light chain 3B (LC3-II) and higher levels of sequestosome 1 (SQSTM1). In contrast, the long-term resistance exercise elevated autophagy, as shown by higher levels of LC3-II and lower levels of SQSTM1. No significant changes in LC3-II levels were observed with moderate- or vigorous-intensity endurance exercise either as an acute bout or long-term. In terms of tissue types, exercise exerted opposite effects between skeletal muscles and peripheral blood mononuclear cells (PBMCs), whereby autophagy was suppressed in skeletal muscles when activated in the PBMCs. Other meta-analyses have also shown significant alterations in the level of many canonical autophagic and mitophagic proteins, including unc-51 like autophagy activating kinase (ULK1)S317, ULK1S757, Beclin-1, ATG12, BCL2/adenovirus E1B 19 kDa protein-interacting protein 3, and PARKIN following exercise, suggesting the activation of canonical autophagy and mitophagy, although the scope of those analyses was more limited.
Conclusion
Our findings demonstrate that physical exercise probably regulates autophagy in an exercise modality- and tissue-dependent manner in humans, although further investigation is needed. Customized exercise prescriptions should be aimed for when implementing exercise to regulate autophagy in humans.
Abbreviations: ATG: autophagy-related gene; BCL2L13: BCL2-like 13; BECN1: beclin1; BNIP3: BCL2/adenovirus E1B 19 kDa protein-interacting protein 3; GABARAP: gamma-aminobutyric acid receptor-associated protein; GAPDH: glyceraldehyde 3-phosphate dehydrogenase; LAMP2: lysosome-associated membrane protein 2; LC3B: microtubule-associated proteins 1A/1B light chain 3B; MD: mean difference; mTOR: mammalian target of rapamycin; PBMC: peripheral blood mononuclear cells; PINK1: PTEN-induced kinase 1; PRISMA: preferred reporting items for systematic review and meta-analysis; SD: standard deviation; SQSTM1: sequestosome 1; ULK1: unc-51 like autophagy activating kinase 1; VDAC1: voltage-dependent anion-selective channel 1.
1. Introduction
Macroautophagy/autophagy (Greek for “self-eating”) is a crucial cellular recycling process maintaining homeostasis, in which redundant or faulty cytoplasmic components undergo autophagy-related gene (ATG)-regulated degradation in the autophagosome and lysosome machineries Citation1. The disruption of autophagy has been implicated in a range of diseases, including metabolic diseases, cardiovascular diseases, infectious diseases, and cancer Citation2. However, the mechanisms underlying its role in these diseases are still not fully elucidated, as autophagy usually plays a double-edged-sword role in the development and progression of disease. In addition, boosting of autophagy is proposed to be associated with delayed aging and a prolonged life- and healthspan in mammals Citation3,Citation4, although adverse effects of hyperactivated autophagy have also been reported Citation5,Citation6. Given the essential and complex nature of autophagy, its proper and accurate regulation is likely to be required for the promotion of health and treatment of specific diseases. Owing to limited and inconclusive clinical evidence, approved drugs and therapies that primarily target autophagy are rare. Nevertheless, autophagy is thought to be a critical mechanism underlying health-promoting strategies, including intermittent fasting Citation7, particular nutritional supplements Citation8, and physical exercise Citation9.
Physical exercise has long been recognized as a robust activator of autophagy in various organs and tissues in animal models Citation10,Citation11. Deficient autophagy in turn may account for inactivity-related diseases and conditions, such as accelerated aging, obesity, and cancer Citation12,Citation13. In the past decade, mounting evidence has shown that autophagy and mitophagy, a selective form of autophagy for removal of mitochondria, are indispensable not only for exercise-induced adaptations of muscle and brain but also for improvements in exercise capacity Citation14–17. More importantly, physical exercise acts as a form of “autophagy pills” in the treatment of diverse diseases, including metabolic, neurodegenerative, and cardiovascular diseases Citation10,Citation18–20. Despite well-documented autophagic responses to exercise and the associated health benefits in rodent studies, it remains unclear whether exercise regulates autophagy in humans. In various studies, activation, repression, or no change in autophagy has been observed following exercise in humans Citation21–24, a difference that is probably determined by exercise modality Citation22, tissue type Citation24, or participant characteristics Citation25. This inconsistency has limited translation of findings from animal models to humans and implementation of physical exercise as an autophagy modulator in humans. Therefore, a more conclusive picture of the autophagic response to exercise in humans is required.
Despite extensive narrative reviews on exercise-induced autophagy in both animal and human studies Citation9,Citation26–29, the regulatory effects of physical exercise on autophagy in humans are still unclear. To the best of our knowledge, no systematic review or meta-analysis has examined the effects of physical exercise on autophagy in humans. The purpose of this review was to systematically assess human studies that explored autophagic responses to exercise and the impacts of potential determinants, such as exercise modality, tissue type, and participant characteristics. A more conclusive picture will be generated by synthesizing findings regarding autophagic responses to exercise in human studies using meta-analysis.
2. Methods
2.1. Search strategy and study selection
This systematic review and meta-analysis was conducted in accordance with Preferred Reporting Items for Systematic Review and Meta-analysis (PRISMA) guidelines Citation30 and was prospectively registered with the International Prospective Register of Systematic Reviews (PROSPERO) database (CRD42020200823). The MEDLINE, Embase, Cochrane, Scopus, and Web of Science databases were systematically searched from inception until September 01, 2022. The Medical Subject Headings (MeSH terms) of “exercise” and “autophagy” and core ATG names were employed in the search without date limit. A full list of search items is presented in Table S1. The search was limited to (a) articles written in English, (b) original studies, and (c) peer-reviewed articles. Duplicates were removed using Mendeley Reference Management software (Elsevier, USA). The initial and full-text screening of all records was performed independently by two reviewers and verified by a third reviewer. Studies that meet the following inclusion criteria were selected: (a) human study, (b) “exercise” is “physical exercise”, (c) exercise is the sole intervention, and (d) the protein level of microtubule-associated proteins 1A/1B light chain 3B (LC3B), the most commonly used indicator of autophagy Citation2, is reported.
2.2. Data extraction
The characteristics of articles selected were extracted using a standard extraction form Citation31, with following information: (a) author and publication year, (b) characteristics of participants, (c) exercise modality, (d) tissue type, and (e) autophagic proteins, with their changes following exercise. For meta-analysis, mean and standard deviation (SD) were extracted for the control and exercise groups. A relative level of autophagic proteins to glyceraldehyde 3-phosphate dehydrogenase (GAPDH) or other housekeeping proteins was extracted for western blot results. WebPlotDigitizer software was used if data were only reported on graphs Citation32. The original author was contacted if data presentation was incomplete.
2.3. Statistical analysis
A meta-analysis was performed using Review Manager 5.4 software (Cochrane Collaboration, Oxford, UK). Pooled effects were estimated on the basis of intervention effects (mean difference [MD] between groups) using a random-effects model. Subgroup analysis was applied to examine the effect of exercise modality, tissue type, or participant characteristic on autophagic responses to exercise in humans. Statistical significance was set at p-value < 0.05. I2 value was used to determine the heterogeneity Citation33.
3. Results
3.1. Study characteristics
A total of 4086 articles were identified through a systematic search of the five databases and a manual search of the reference list. After removal of duplicates, 2078 articles were selected for title and abstract screening, and 72 of these were further screened using the full text. Twenty-seven articles were found to meet the inclusion criteria Citation21–25,Citation34–55. Figure S1 outlines the PRISMA flow chart of article selection and the number of excluded articles, with reasons given.
The characteristics of the articles selected are given in . All selected articles were published between 2010 and 2022. Seven were published by United States institutions Citation23,Citation24,Citation35,Citation39,Citation40,Citation53,Citation54 and two by Australian institutions Citation46,Citation48. All others were published by European institutions, including four each from Denmark Citation22,Citation25,Citation37,Citation52 and Belgium Citation36,Citation38,Citation44,Citation45 and two each from Germany Citation47,Citation51, Spain Citation41,Citation42, Finland Citation34,Citation55, Sweden Citation43,Citation49, and Switzerland Citation21,Citation50. None were published by Asian, South American, or African institutions. A total of 519 participants aged 18–76 years were classified into five categories: (a) healthy untrained young adults, (b) trained adults, athletes Citation36,Citation44,Citation45,Citation55 or soldiers Citation43, (c) older adults Citation34,Citation41,Citation42,Citation53,Citation55, (d) adults with obesity or type 2 diabetes Citation35,Citation37,Citation40,Citation51, and (e) adults with essential hypertension Citation25.
Table 1. Characteristics of available evidence on autophagic responses to exercise in humans.
Various types of physical exercise were undertaken by the participants, including (a) an acute bout of exercise Citation23,Citation24,Citation35–40,Citation45,Citation46,Citation49,Citation50,Citation53,Citation54,Citation43,Citation44, (b) long-term exercise (3–21 weeks) Citation21,Citation25,Citation41,Citation42,Citation51,Citation55, and (c) both Citation22,Citation34,Citation47,Citation48,Citation52. In addition, moderate-intensity endurance exercise Citation21,Citation22,Citation36,Citation38–40,Citation44,Citation50, vigorous-intensity endurance exercise Citation23–25,Citation35,Citation37,Citation43,Citation45,Citation46,Citation48,Citation51, resistance exercise Citation34,Citation39,Citation41,Citation42,Citation47,Citation49,Citation52–55, and mixed-type exercise Citation43,Citation51 were used. The exercises were performed using cycle ergometers Citation22,Citation25,Citation37–40,Citation48,Citation50,Citation42,Citation44–46, treadmills Citation23,Citation24,Citation35,Citation36, strength training equipment Citation34,Citation39,Citation41,Citation42,Citation47,Citation49,Citation52–55, and mixed modes Citation21,Citation43,Citation51. In terms of the tissue types, skeletal muscles Citation21,Citation22,Citation45–49,Citation51–55,Citation25,Citation34,Citation36–39,Citation43,Citation44, peripheral blood mononuclear cells (PBMCs) Citation23,Citation35,Citation40–42,Citation50, or both Citation24 were sampled for autophagy analysis, using muscle biopsy and/or venous blood collection.
The levels of a range of canonical autophagic proteins were determined in the studies assessed, including LC3, sequestosome 1 (SQSTM1), gamma-aminobutyric acid receptor-associated protein (GABARAP), lysosome-associated membrane protein 2 (LAMP2), total unc-51 like autophagy activating kinase 1 (ULK1), ULK1S317, ULK1S638, ULK1S555, ULK1S757, beclin1 (BECN1), ATG7, ATG12, ATG5, ATG16, and ATG3. Mitophagic proteins were also examined in some studies, including BCL2/adenovirus E1B 19 kDa protein-interacting protein 3 (BNIP3), PARKIN, PTEN-induced kinase 1 (PINK1), voltage-dependent anion-selective channel 1 (VDAC1), and BCL2-like 13 (BCL2L13). Discrepant alterations in the levels of these autophagic or mitophagic proteins following exercise in humans were observed among the studies (). Only markers reported in at least four studies were further included in the meta-analysis, comprising LC3, SQSTM1, BECN1, ULK1S317, ULK1S555, ULK1S757, ATG12, BNIP3, and PARKIN.
3.2. Meta-analysis
Overall, 26 studies were included in the meta-analysis, with one study excluded due to lack of internal controls for western blot data Citation54. Synthesizing data from 26 studies, we found no significant change in LC3-II levels following exercise in humans, regardless of exercise modality (MD = –0.03; 95% CI = –0.10, 0.03; P = 0.29). Subgroup analyses revealed that no significant changes in LC3-II levels were observed following an acute bout of moderate-intensity or vigorous-intensity endurance exercise (), whereas LC3-II levels significantly declined following an acute bout of resistance exercise (MD = –0.31; 95% CI = –0.41, –0.20; P < 0.001) (). Furthermore, no significant alteration in the level of LC3-II was observed between pre- and post-exercise time points, including immediately, one hour, two hours, three hours, and four hours following an acute bout of moderate- or vigorous-intensity endurance exercise (Figure S2). With respect to long-term exercise, resistance exercise significantly increased the level of LC3-II (MD = 0.28; 95% CI = 0.04, 0.52; P = 0.02), while no significant effect was found in moderate-intensity or vigorous-intensity endurance exercise (). To confirm the autophagic responses suggested on the basis of changes in LC3-II, the levels of SQSTM1 were also assessed. As expected, no significant change was detected following an acute bout of moderate- or vigorous-intensity endurance exercise (), whereas a significant increase in the level of SQSTM1 was observed following an acute bout of resistance exercise (MD = 0.23; 95% CI = 0.11, 0.34; P < 0.001) (). However, SQSTM1 levels significantly altered following long-term resistance exercise (MD = –0.20; 95% CI = –0.32, –0.08; P = 0.001) and vigorous-intensity endurance exercise (MD = 0.24; 95% CI = 0.12, 0.36; P < 0.001) (). A separate grouping of exercise types (running, cycling, resistance exercise, and mixed-type exercise) was also analyzed. No significant changes were identified following cycling or running (Figure S3).
Figure 1. The effect of exercise modality on the levels of LC3-II in humans following exercise. (A): Acute bout of moderate-intensity endurance exercise. (B): Acute bout of vigorous-intensity endurance exercise. (C): Acute bout of resistance exercise. (D): Long-term moderate-intensity endurance exercise. (E): Long-term vigorous-intensity endurance exercise. (F): Long-term resistance exercise. CI: Confidence interval; MD: mean difference.
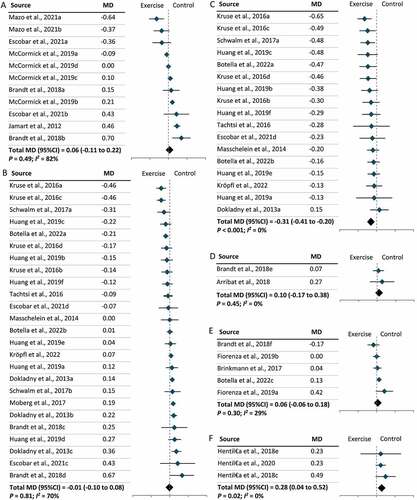
Figure 2. The effect of exercise modality on the levels of SQSTM1 in humans following exercise. (A): Acute bout of moderate-intensity endurance exercise. (B): Acute bout of vigorous-intensity endurance exercise. (C): Acute bout of resistance exercise. (D): Long-term moderate-intensity endurance exercise. (E): Long-term vigorous-intensity endurance exercise. (F): Long-term resistance exercise. CI: Confidence interval; MD: mean difference.
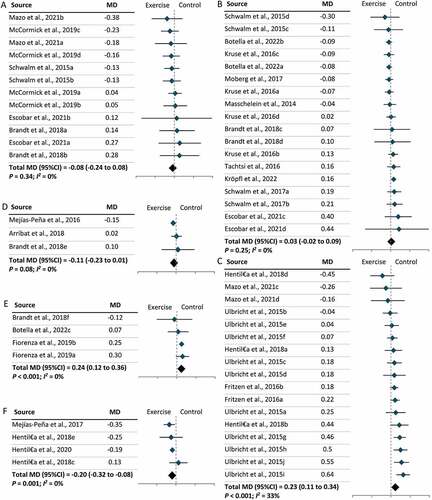
Furthermore, two types of tissue samples were included in our meta-analysis: skeletal muscles and PBMCs. We found that exercise lowered LC3-II levels in skeletal muscles (MD = –0.09; 95% CI = –0.18, 0.00; P = 0.04) but increased LC3-II levels in PBMCs (MD = 0.08; 95% CI = 0.00, 0.16; P = 0.049), independent of exercise modality (). Further subgroup analysis revealed that lower levels of LC3-II in skeletal muscles following exercise were largely due to the effect of resistance exercise on skeletal muscles (MD = –0.28; 95% CI = –0.39, –0.17; P < 0.001) () and (Figure S4). No significant effect of exercise modality on LC3-II levels was found in PBMCs owing to a limited number of studies (Figure S4). In parallel with the results for LC3-II, SQSTM1 levels increased in skeletal muscles (MD = 0.10; 95% CI = 0.04, 0.16; P < 0.001) and decreased in PBMCs (MD = –0.16; 95% CI = –0.24, –0.07; P = 0.049) following exercise (). Further subgroup analysis revealed that resistance exercise was the major exercise modality capable of increasing SQSTM1 levels in skeletal muscles (MD = 0.22; 95% CI = 0.11, 0.33; P < 0.001), while the effect of exercise modality on SQSTM1 levels in PBMCs remains unclear (Figure S5). Subgroup analysis by participant characteristics showed that only older adults had significantly lower LC3-II levels following exercise (MD = –0.38; 95% CI = –0.62, –0.15; P < 0.001), and this may be attributable to the consistent exercise modality (resistance exercise) used in the studies included (Figure S6). In terms of other autophagic proteins, significant changes were observed in the level of ULK1S317 (MD = 0.14; 95% CI = 0.12, 0.15; P < 0.001), ULK1S757 (MD = –0.11; 95% CI = –0.21, –0.01; P = 0.03), ATG12 (MD = 0.27; 95% CI = 0.06, 0.47; P = 0.01), and BECN1 (MD = 0.13; 95% CI = 0.08, 0.18; P < 0.001), but no change was observed in ULK1S555 (). Significant increases were also observed in the levels of two mitophagic proteins, BNIP3 (MD = 0.13; 95% CI = 0.06, 0.19; P < 0.001) and PARKIN (MD = 0.22; 95% CI = 0.09, 0.35; P < 0.001) () following exercise.
Figure 3. The effect of tissue type on the levels of LC3-II in humans following exercise. (A): Skeletal muscles. (B): Peripheral blood mononuclear cells (PBMCs). (C): The effect of resistance exercise on LC3-II levels in skeletal muscles. CI: Confidence interval; MD: mean difference.
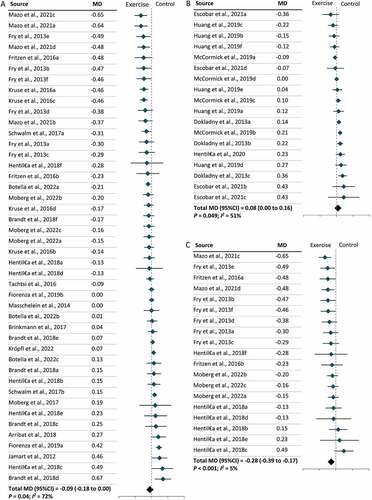
Figure 4. The effect of tissue type on the levels of SQSTM1 in humans following exercise. (A): Skeletal muscles. (B): Peripheral blood mononuclear cells (PBMCs). CI: Confidence interval; MD: mean difference.
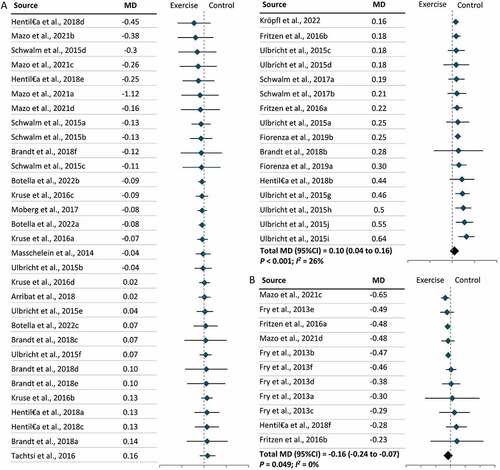
3.3. Study quality and publication bias
Risk of bias scores for individual studies are shown in Table S2–S4. The overall quality of the studies included was fair. The publication bias was detected for LC3-II but not for SQSTM1 by the asymmetry of the funnel plot, the Begg and Mazumdar’s rank correlation test, and the Egger’s regression test (Figure S7 and Table S5). Tweedie and Duval’s trim-and-fill method was used to generate a more symmetrical funnel plot for LC3-II by adding “missing” studies (Figure S7) .
4. Discussion
This is the first systematic review and meta-analysis to examine effects of physical exercise on autophagy in humans. Our findings provide a more conclusive picture of autophagic responses to exercise in humans by synthesizing evidence from 26 eligible studies (). Subgroup analyses revealed that an acute bout of resistance exercise attenuates autophagy, while long-term resistance exercise boosts autophagy, as characterized by changes in LC3-II and SQSTM1 levels, in human skeletal muscles. Conversely, moderate- and vigorous-intensity endurance exercise showed no effect on autophagy. In addition, activation of canonical autophagy and mitophagy, characterized by some other autophagic proteins including ULK1S317, ULK1S757, Beclin-1, ATG12, BNIP3, and PARKIN, was observed following exercise, regardless of exercise modality (); however, the results are somehow inconclusive because of the relatively small volume of evidence. Opposing effects of exercise on autophagy were determined when comparing the skeletal muscles and PBMCs in our meta-analysis, suggesting a discrepancy in autophagic responses to exercise in different tissues or cells. Therefore, the effects of physical exercise on autophagy in humans are likely to be exercise modality- and tissue-dependent.
It has long been recognized that exercise activates autophagy in a wide range of organs and tissues in animal models. Numerous studies have reported elevated levels of LC3-II in skeletal muscles following moderate- Citation10,Citation11,Citation14 and vigorous-intensity endurance exercise Citation56,Citation57, and resistance exercise Citation58–60, effects attributed to mechanical stress, metabolic responses, and nutritional alterations. Contradictory results have also been reported Citation61 and exercise-induced autophagy can be either beneficial or detrimental Citation56,Citation57, but a relatively consistent autophagic responses to exercise was found in rodent studies. However, autophagic responses to exercise in humans remain controversial Citation22,Citation24,Citation39. Using subgroup analyses, our systematic review and meta-analysis revealed that an acute bout of resistance exercise reduced autophagy while long-term resistance exercise enhanced autophagy in human skeletal muscles. Acute bouts of resistance exercise have been less studied in animal models because of difficulty in modeling static resistance training Citation62. Thus, the molecular mechanisms underlying autophagic responses to an acute bout of resistance exercise remain unclear. A previous study has reported that mammalian target of rapamycin (mTOR) is activated in the skeletal muscles during resistance exercise, which is essential for muscle hypertrophy and potentially accounts for inhibition of autophagy Citation63. In contrast, an elevated level of basal autophagy, probably due to the super-compensation in repeated resistance exercise, is required to preserve muscle mass following long-term resistance training Citation64. The findings suggest that long-term resistance exercise is an optimal form of exercise for boosting autophagy in human skeletal muscles. Equally, suppression of autophagy may be a mechanism underlying resistance exercise-induced muscle hypertrophy.
The effects of moderate- or vigorous-intensity endurance exercise on autophagy remain inconclusive within our meta-analysis. Several canonical autophagic (ULK1S317, Beclin-1, and ATG12) and mitophagic proteins (BNIP3 and PARKIN) were significantly increased following endurance exercise, suggesting a possible activation of autophagy and mitophagy, but LC3-II and SQSTM1 levels remained almost unchanged following exercise in meta-analyses. It remains unclear whether the health benefits of moderate- or vigorous-intensity endurance exercise in humans are mediated by autophagy. Since autophagy activated by an acute bout of endurance exercise returns to baseline rapidly following exercise Citation28,Citation65, a substantial increase in the levels of autophagic proteins may be required for subsequent detection. Thus, a discrepancy between rodents and humans in metabolic activation and subsequent autophagy activation following exercise could be due to the relatively higher intensity or dose of exercise used in animal studies Citation62. Importantly, aberrant autophagic responses to intense exercise are generally considered detrimental to human health. For instance, excessive autophagic cell death in cardiac or skeletal muscle can be involved in sports injuries Citation56,Citation57, which should be avoided in human studies. At the same time, the energy and nutrients can be adequately supplied by the blood and skeletal muscles during moderate-intensity endurance exercise over a time span from minutes to hours Citation66. Sufficient autophagic activation in humans may require a high-dose or prolonged endurance exercise, but the duration of the acute bout of endurance exercise was relatively short in existing human studies. Compared with an acute bout of exercise, long-term endurance exercise training represents a more effective health-promoting strategy in animal models; however, human studies on its effects on autophagy remain limited and further investigation is required.
Systemic effects of moderate-intensity endurance exercise have been reported in the last decade, in which autophagy is activated in a wide range of organs or tissues in animals, including skeletal muscles, brain, liver, and heart Citation10,Citation11. Metabolic alterations at the whole-body level are considered responsible for exercise-induced systemic autophagic responses Citation67,Citation68 and comprise an essential basis for treating diseases that occur in organs other than skeletal muscles via exercise, such as metabolic, neurodegenerative, and cardiovascular diseases Citation10,Citation19,Citation69. However, sampling of organs or tissues other than skeletal muscles and blood for autophagy measurements remain a major obstacle in human studies. In the present study, our meta-analysis indicated that autophagic responses to exercise varied between skeletal muscles and PBMCs. This implies a possible distinct regulation of autophagy in different tissues and cells. Autophagy was elevated in PBMCs following exercise, regardless of the exercise modality, which is probably due to metabolic or nutritional alterations. In contrast, mechanical stress may contribute to lower levels of autophagy in skeletal muscles following exercise. It is possible that other remote organs or tissues may also exhibit an increased level of autophagy following exercise, caused by metabolic or nutritional alterations, as has been shown in human blood cells and rodent organs or tissues Citation10,Citation11. Our results provide evidence that regulation of autophagy by exercise is likely to be tissue-dependent in humans, meaning that there may be a need for customized exercise prescriptions to regulate autophagy in different tissues or cells in the context of particular diseases.
The present study has several limitations. First, while we suggest that the effect of exercise on autophagy is likely to be tissue-dependent, our meta-analysis was restricted to skeletal muscles and PBMCs as the sampling of other organs and tissues is currently not feasible in human studies. Second, various standard methods for assaying autophagy and autophagic flux, including transmission electron microscopy and blockage of autophagic flux using autophagic inhibitors, have rarely been applied in human studies. The application of these methods and development of novel methods for in vivo measurement of autophagy and autophagic flux in specific human organs and tissues are needed. Third, western blot results for LC3-II and SQSTM1 were used as major outcomes in our meta-analysis, and this may lead to inappropriate interpretations of the results in the absence of adequate evidence from other assays or indicators Citation2, though some other markers of canonical autophagy and mitophagy were also analyzed. Fourth, studies involving older adults or individuals with obesity or other diseases, including type 2 diabetes and hypertension, remain limited. More importantly, no studies identified in our systematic search were performed by Asian, South American, or African institutions. Thus, whether autophagic responses to exercise are consistent under different health conditions and between different ethnic groups requires further evidence.
5. Summary
Physical exercise regulates autophagy in humans in a manner that is probably exercise modality- and tissue-dependent. Long-term resistance exercise is an recommended form of exercise to boost autophagy in human skeletal muscles. Further studies of the effects of exercise on autophagy using various autophagic markers, exercise modalities, tissue types, and participants under different health conditions are needed for the development of customized exercise prescriptions for boosting health or treating diseases in humans by regulating autophagy.
Conflict of Interest
The authors have declared that no conflict of interest exists.
Financial support
X.K.C. is supported by the Postdoctoral Matching Fund, The Hong Kong Polytechnic University, and C.Z. is supported by the Research Fellowship Scheme, The Chinese University of Hong Kong.
Authors’ contributions
S.H.W. and A.C.M. contributed to the conception and design of the study; X.C. and C.Z. performed the systematic search, data extraction, assessment of the risk of bias, and meta-analysis; P.M.S. contributed to data analysis and results interpretation; F.S. conducted the Begg and Mazumdar’ s rank correlation test and Egger’ s regression test. All authors have read and approved the final version of the manuscript, and agree with the order of presentation of the authors
Supplemental Material
Download ()Acknowledgments
We would like to thank contacted authors for taking the time to respond to data requests in such a kind and prompt manner.
SUPPLEMENTARY MATERIAL
Supplemental data for this article can be accessed online at https://doi.org/10.1080/27694127.2023.2190202
References
- Levine B, Kroemer G. Autophagy in the pathogenesis of disease. Cell 2008; 132:27–24.
- Klionsky DJ, Abdel-Aziz AK, Abdelfatah S, Abdellatif M, Abdoli A, Abel S, Abeliovich H, Abildgaard MH, Abudu YP, Acevedo-Arozena A, et al. Guidelines for the use and interpretation of assays for monitoring autophagy (4th edition). Autophagy 2021; 17:1–382.
- Pyo J-O, Yoo S-M, Ahn H-H, Nah J, Hong S-H, Kam T-I, Jung S, Jung Y-K. Overexpression of Atg5 in mice activates autophagy and extends lifespan. Nat Commun 2013; 4:2300.
- Fernández ÁF, Sebti S, Wei Y, Zou Z, Shi M, McMillan KL, He C, Ting T, Liu Y, Chiang W-C, et al. Disruption of the beclin 1-BCL2 autophagy regulatory complex promotes longevity in mice. Nature 2018; 558:136–40.
- Levine B. Cell biology: autophagy and cancer. Nature2007; 446:745–7.
- Zhou B, Kreuzer J, Kumsta C, Wu L, Kamer KJ, Cedillo L, Zhang Y, Li S, Kacergis MC, Webster CM, et al. Mitochondrial Permeability Uncouples Elevated Autophagy and Lifespan Extension. Cell 2019; 177:299–314.e16.
- Ulgherait M, Midoun AM, Park SJ, Gatto JA, Tener SJ, Siewert J, Klickstein N, Canman JC, Ja WW, Shirasu-Hiza M. Circadian autophagy drives iTRF-mediated longevity. Nature 2021; 598:353–8.
- Konings E, Timmers S, Boekschoten M V, Goossens GH, Jocken JW, Afman LA, Müller M, Schrauwen P, Mariman EC, Blaak EE. The effects of 30 days resveratrol supplementation on adipose tissue morphology and gene expression patterns in obese men. Int J Obes (Lond) 2014; 38:470–3.
- Halling JF, Pilegaard H. Autophagy-Dependent Beneficial Effects of Exercise. Cold Spring Harb Perspect Med 2017; 7.
- He C, Bassik MC, Moresi V, Sun K, Wei Y, Zou Z, An Z, Loh J, Fisher J, Sun Q. Exercise-induced BCL2-regulated autophagy is required for muscle glucose homeostasis. Nature 2012; 481:511–5.
- He C, Sumpter Rhea J, Levine B. Exercise induces autophagy in peripheral tissues and in the brain. Autophagy 2012; 8:1548–51.
- Organization WH. Global health risks: mortality and burden of disease attributable to selected major risks. World Health Organization; 2009.
- Drummond MJ, Addison O, Brunker L, Hopkins PN, McClain DA, LaStayo PC, Marcus RL. Downregulation of E3 ubiquitin ligases and mitophagy-related genes in skeletal muscle of physically inactive, frail older women: a cross-sectional comparison. J Gerontol A Biol Sci Med Sci 2014; 69:1040–8.
- Lira VA, Okutsu M, Zhang M, Greene NP, Laker RC, Breen DS, Hoehn KL, Yan Z. Autophagy is required for exercise training-induced skeletal muscle adaptation and improvement of physical performance. FASEB J Off Publ Fed Am Soc Exp Biol 2013; 27:4184–93.
- Codina-Martínez H, Fernández-García B, Díez-Planelles C, Fernández ÁF, Higarza SG, Fernández-Sanjurjo M, Díez-Robles S, Iglesias-Gutiérrez E, Tomás-Zapico C. Autophagy is required for performance adaptive response to resistance training and exercise-induced adult neurogenesis. Scand J Med Sci Sports 2020; 30:238–53.
- Lo Verso F, Carnio S, Vainshtein A, Sandri M. Autophagy is not required to sustain exercise and PRKAA1/AMPK activity but is important to prevent mitochondrial damage during physical activity. Autophagy 2014; 10:1883–94.
- Guan Y, Drake JC, Yan Z. Exercise-Induced Mitophagy in Skeletal Muscle and Heart. Exerc Sport Sci Rev 2019; 47:151–6.
- Kang E-B, Cho J-Y. Effect of treadmill exercise on PI3K/AKT/mTOR, autophagy, and Tau hyperphosphorylation in the cerebral cortex of NSE/htau23 transgenic mice. J Exerc Nutr Biochem 2015; 19:199–209.
- Rocchi A, Yamamoto S, Ting T, Fan Y, Sadleir K, Wang Y, Zhang W, Huang S, Levine B, Vassar R, et al. A Becn1 mutation mediates hyperactive autophagic sequestration of amyloid oligomers and improved cognition in Alzheimer’s disease. PLoS Genet 2017; 13:e1006962.
- Campos JC, Queliconi BB, Bozi LHM, Bechara LRG, Dourado PMM, Andres AM, Jannig PR, Gomes KMS, Zambelli VO, Rocha-Resende C, et al. Exercise reestablishes autophagic flux and mitochondrial quality control in heart failure. Autophagy 2017; 13:1304–17.
- Arribat Y, Broskey NT, Greggio C, Boutant M, Conde Alonso S, Kulkarni SS, Lagarrigue S, Carnero EA, Besson C, Cantó C, et al. Distinct patterns of skeletal muscle mitochondria fusion, fission and mitophagy upon duration of exercise training. Acta Physiol (Oxf) 2019; 225:e13179.
- Brandt N, Gunnarsson TP, Bangsbo J, Pilegaard H. Exercise and exercise training-induced increase in autophagy markers in human skeletal muscle. Physiol Rep 2018; 6:e13651.
- Dokladny K, Zuhl MN, Mandell M, Bhattacharya D, Schneider S, Deretic V, Moseley PL. Regulatory coordination between two major intracellular homeostatic systems: heat shock response and autophagy. J Biol Chem 2013; 288:14959–72.
- Escobar KA, Welch AM, Wells A, Fennel Z, Nava R, Li Z, Moriarty TA, Nitta CH, Zuhl MN, VanDusseldorp TA. Autophagy response to acute high-intensity interval training and moderate-intensity continuous training is dissimilar in skeletal muscle and peripheral blood mononuclear cells and is influenced by sex. Hum Nutr Metab 2021; 23:200118.
- Fiorenza M, Gunnarsson TP, Ehlers TS, Bangsbo J. High-intensity exercise training ameliorates aberrant expression of markers of mitochondrial turnover but not oxidative damage in skeletal muscle of men with essential hypertension. Acta Physiol (Oxf) 2019; 225:e13208.
- Tam BT, Siu PM. Autophagic cellular responses to physical exercise in skeletal muscle. Sports Med 2014; 44:625–40.
- Vainshtein A, Hood DA. The regulation of autophagy during exercise in skeletal muscle. J Appl Physiol 2016; 120:664–73.
- Martin-Rincon M, Morales-Alamo D, Calbet JAL. Exercise-mediated modulation of autophagy in skeletal muscle. Scand J Med Sci Sports 2018; 28:772–81.
- Liang J, Zeng Z, Zhang Y, Chen N. Regulatory role of exercise-induced autophagy for sarcopenia. Exp Gerontol 2020; 130:110789.
- Shamseer L, Moher D, Clarke M, Ghersi D, Liberati A, Petticrew M, Shekelle P, Stewart LA. Preferred reporting items for systematic review and meta-analysis protocols (PRISMA-P) 2015: elaboration and explanation. BMJ 2015; 350:g7647.
- Higgins JP. Cochrane handbook for systematic reviews of interventions. Version 5.1. 0 [updated March 2011]. The Cochrane Collaboration. www.cochrane-handbook.org 2011;
- Rohatgi A. WebPlotDigitizer. URL http://arohatgi. info. WebPlotDigitizer/app 2011;
- Higgins JPT, Thompson SG. Quantifying heterogeneity in a meta-analysis. Stat Med 2002; 21:1539–58.
- Hentilä J, Ahtiainen JP, Paulsen G, Raastad T, Häkkinen K, Mero AA, Hulmi JJ. Autophagy is induced by resistance exercise in young men, but unfolded protein response is induced regardless of age. Acta Physiol (Oxf) 2018; 224:e13069.
- Huang C-J, Rodriguez AL, Visavadiya NP, Fico BG, Slusher AL, Ferrandi PJ, Whitehurst M. An exploratory investigation of apoptotic and autophagic responses in peripheral blood mononuclear cells following maximal aerobic exercise in obese individuals. Arch Physiol Biochem 2019;:1–8.
- Jamart C, Francaux M, Millet GY, Deldicque L, Frère D, Féasson L. Modulation of autophagy and ubiquitin-proteasome pathways during ultra-endurance running. J Appl Physiol 2012; 112:1529–37.
- Kruse R, Pedersen AJT, Kristensen JM, Petersson SJ, Wojtaszewski JFP, Højlund K. Intact initiation of autophagy and mitochondrial fission by acute exercise in skeletal muscle of patients with Type 2 diabetes. Clin Sci (Lond) 2017; 131:37–47.
- Masschelein E, Van Thienen R, D’Hulst G, Hespel P, Thomis M, Deldicque L. Acute environmental hypoxia induces LC3 lipidation in a genotype-dependent manner. FASEB J Off Publ Fed Am Soc Exp Biol 2014; 28:1022–34.
- Mazo CE, D’Lugos AC, Sweeney KR, Haus JM, Angadi SS, Carroll CC, Dickinson JM. The effects of acute aerobic and resistance exercise on mTOR signaling and autophagy markers in untrained human skeletal muscle. Eur J Appl Physiol 2021; 121:2913–24.
- McCormick JJ, King KE, Dokladny K, Mermier CM. Effect of Acute Aerobic Exercise and Rapamycin Treatment on Autophagy in Peripheral Blood Mononuclear Cells of Adults With Prediabetes. Can J diabetes 2019; 43:457–63.
- Mejías-Peña Y, Estébanez B, Rodriguez-Miguelez P, Fernandez-Gonzalo R, Almar M, de Paz JA, González-Gallego J, Cuevas MJ. Impact of resistance training on the autophagy-inflammation-apoptosis crosstalk in elderly subjects. Aging (Albany NY) 2017; 9:408–18.
- Mejías-Peña Y, Rodriguez-Miguelez P, Fernandez-Gonzalo R, Martínez-Flórez S, Almar M, de Paz JA, Cuevas MJ, González-Gallego J. Effects of aerobic training on markers of autophagy in the elderly. Age (Dordr) 2016; 38:33.
- Moberg M, Hendo G, Jakobsson M, Mattsson CM, Ekblom-Bak E, Flockhart M, Pontén M, Söderlund K, Ekblom B. Increased autophagy signaling but not proteasome activity in human skeletal muscle after prolonged low-intensity exercise with negative energy balance. Physiol Rep 2017; 5.
- Schwalm C, Jamart C, Benoit N, Naslain D, Prémont C, Prévet J, Van Thienen R, Deldicque L, Francaux M. Activation of autophagy in human skeletal muscle is dependent on exercise intensity and AMPK activation. FASEB J Off Publ Fed Am Soc Exp Biol 2015; 29:3515–26.
- Schwalm C, Deldicque L, Francaux M. Lack of Activation of Mitophagy during Endurance Exercise in Human. Med Sci Sports Exerc 2017; 49:1552–61.
- Tachtsis B, Smiles WJ, Lane SC, Hawley JA, Camera DM. Acute Endurance Exercise Induces Nuclear p53 Abundance in Human Skeletal Muscle. Front Physiol 2016; 7:144.
- Ulbricht A, Gehlert S, Leciejewski B, Schiffer T, Bloch W, Höhfeld J. Induction and adaptation of chaperone-assisted selective autophagy CASA in response to resistance exercise in human skeletal muscle. Autophagy 2015; 11:538–46.
- Botella J, Jamnick NA, Granata C, Genders AJ, Perri E, Jabar T, Garnham A, Lazarou M, Bishop DJ. Exercise and Training Regulation of Autophagy Markers in Human and Rat Skeletal Muscle. Int J Mol Sci 2022; 23.
- Moberg M, Apró W, Horwath O, van Hall G, Blackwood SJ, Katz A. Acute normobaric hypoxia blunts contraction-mediated mTORC1- and JNK-signaling in human skeletal muscle. Acta Physiol (Oxf) 2022; 234:e13771.
- Kröpfl JM, Morandi C, Gasser BA, Schoch R, Schmidt-Trucksäss A, Brink M. Lymphocytes are less sensitive to autophagy than monocytes during fasting and exercise conditions. Apoptosis 2022; 27:730–9.
- Brinkmann C, Przyklenk A, Metten A, Schiffer T, Bloch W, Brixius K, Gehlert S. Influence of endurance training on skeletal muscle mitophagy regulatory proteins in type 2 diabetic men. Endocr Res 2017; 42:325–30.
- Fritzen AM, Madsen AB, Kleinert M, Treebak JT, Lundsgaard A-M, Jensen TE, Richter EA, Wojtaszewski J, Kiens B, Frøsig C. Regulation of autophagy in human skeletal muscle: effects of exercise, exercise training and insulin stimulation. J Physiol 2016; 594:745–61.
- Fry CS, Drummond MJ, Glynn EL, Dickinson JM, Gundermann DM, Timmerman KL, Walker DK, Volpi E, Rasmussen BB. Skeletal muscle autophagy and protein breakdown following resistance exercise are similar in younger and older adults. J Gerontol A Biol Sci Med Sci 2013; 68:599–607.
- Glynn EL, Fry CS, Drummond MJ, Dreyer HC, Dhanani S, Volpi E, Rasmussen BB. Muscle protein breakdown has a minor role in the protein anabolic response to essential amino acid and carbohydrate intake following resistance exercise. Am J Physiol Regul Integr Comp Physiol 2010; 299:R533–40.
- Hentilä J, Hulmi JJ, Laakkonen EK, Ahtiainen JP, Suominen H, Korhonen MT. Sprint and Strength Training Modulates Autophagy and Proteostasis in Aging Sprinters. Med Sci Sports Exerc 2020; 52:1948–59.
- Salminen A, Vihko V. Autophagic response to strenuous exercise in mouse skeletal muscle fibers. Virchows Arch B Cell Pathol Incl Mol Pathol 1984; 45:97–106.
- Liu H, Lei H, Shi Y, Wang J-J, Chen N, Li Z-H, Chen Y-F, Ye Q-F, Yang Y. Autophagy inhibitor 3-methyladenine alleviates overload-exercise-induced cardiac injury in rats. Acta Pharmacol Sin 2017; 38:990–7.
- Luo L, Lu A-M, Wang Y, Hong A, Chen Y, Hu J, Li X, Qin Z-H. Chronic resistance training activates autophagy and reduces apoptosis of muscle cells by modulating IGF-1 and its receptors, Akt/mTOR and Akt/FOXO3a signaling in aged rats. Exp Gerontol 2013; 48:427–36.
- White Z, Terrill J, White RB, McMahon C, Sheard P, Grounds MD, Shavlakadze T. Voluntary resistance wheel exercise from mid-life prevents sarcopenia and increases markers of mitochondrial function and autophagy in muscles of old male and female C57BL/6J mice. Skelet Muscle 2016; 6:45.
- Cui D, Drake JC, Wilson RJ, Shute RJ, Lewellen B, Zhang M, Zhao H, Sabik OL, Onengut S, Berr SS, et al. A novel voluntary weightlifting model in mice promotes muscle adaptation and insulin sensitivity with simultaneous enhancement of autophagy and mTOR pathway. FASEB J Off Publ Fed Am Soc Exp Biol 2020; 34:7330–44.
- Kim YA, Kim YS, Song W. Autophagic response to a single bout of moderate exercise in murine skeletal muscle. J Physiol Biochem 2012; 68:229–35.
- Fuller KNZ, Thyfault JP. Barriers in translating preclinical rodent exercise metabolism findings to human health. J Appl Physiol 2021; 130:182–92.
- Deldicque L, Theisen D, Francaux M. Regulation of mTOR by amino acids and resistance exercise in skeletal muscle. Eur J Appl Physiol 2005; 94:1–10.
- Masiero E, Agatea L, Mammucari C, Blaauw B, Loro E, Komatsu M, Metzger D, Reggiani C, Schiaffino S, Sandri M. Autophagy is required to maintain muscle mass. Cell Metab 2009; 10:507–15.
- Pagano AF, Py G, Bernardi H, Candau RB, Sanchez AMJ. Autophagy and protein turnover signaling in slow-twitch muscle during exercise. Med Sci Sports Exerc 2014; 46:1314–25.
- Hargreaves M, Spriet LL. Skeletal muscle energy metabolism during exercise. Nat Metab 2020; 2:817–28.
- Egan B, Zierath JR. Exercise metabolism and the molecular regulation of skeletal muscle adaptation. Cell Metab 2013; 17:162–84.
- Cassidy S, Thoma C, Houghton D, Trenell MI. High-intensity interval training: a review of its impact on glucose control and cardiometabolic health. Diabetologia 2017; 60:7–23.
- Wang L, Wang J, Cretoiu D, Li G, Xiao J. Exercise-mediated regulation of autophagy in the cardiovascular system. J Sport Heal Sci 2020; 9:203–10.