ABSTRACT
We review in this paper the hallmarks of cancer (described by Hanahan and Weinberg) from genomic perspective and we give examples of genomic alterations underlying each of the 10 biological characteristics. We explore examples of current FDA approved therapies that target specific pathways belonging to the different hallmarks of cancer. We also propose an addition to the 10 hallmarks of cancer.
Introduction
The hallmarks of cancer were first described by Hanahan and Weinberg in 2000Citation1 and updated in 2011.Citation2 Currently, the hallmarks of cancer include 10 pathways including proliferative signaling, evasion of suppression of growth, escaping immune response to cancer, escaping programmed cell death, cell replicative immortality, tumor promoting inflammation, activating invasion and metastasis, inducing angiogenesis, genome instability and mutations, and change of cellular energetics. Many suggestions have been published contesting or supporting the inclusion of the 10 hallmarks of cancer.Citation3–5 In this current manuscript, we rather approach the 10 hallmarks of cancer from a different perspective by systematically assigning a comprehensive growing list of cancer genomic alterations to each of the 10 hallmarks of cancer. Additionally, we suggest a new addition to the 10 hallmarks of cancer.
Methods
The Catalogue Of Somatic Mutations In Cancer (COSMIC)Citation6,Citation7 has over 700 cancer related genomic alterations. Each alteration is manually annotated with its relationship to none or at least one of the hallmarks of cancer. We manually searched the COSMIC database alteration by alteration and compiled and sorted the several hundred genomic alterations and divided them into 10 categories according to the 10 hallmarks of cancer.
Results
In this result section, each hallmark of cancer is listed with a descriptive summary followed by a list of genes (from COSMIC) associated with the particular hallmark, followed by examples of FDA approved targeted therapy corresponding to the particular hallmark (see also ).
Table 1. Summary of each hallmark of cancer and its associated genes, unique genes, and approved medications.
Proliferative Signalling
As opposed to non-cancerous cells in normal tissue, neoplastic cells acquire the potential of uncontrolled proliferation by upregulating specific signaling pathways. For example, the ROS1 gene, a member of the sevenless subfamily of tyrosine kinase insulin receptors genes, encodes a transmembrane protein with intracellular tyrosine kinase activity.Citation8,Citation9 ROS1 is often affected by mutations or chromosomal rearrangement such as fusions in different types of cancer and stimulates cell proliferation.Citation10 Crizotinib, a small-molecule tyrosine kinase inhibitor, has shown an objective response rate of approximately 72% in patients with advanced ROS1-rearranged non-small cell lung carcinoma. Median duration of response was 17.6 months.Citation11
According to COSMIC, alterations in the following genes are associated with proliferative signaling:
ABI1, ABL1, ACKR3, ACVR1, AFF4, AKT1, ALK, AR, BAP1, BCL10, BCL9, BCL9L, BRAF, BRD4, BUB1B, CACNA1D, CALR, CASP8, CBL, CCND2, CCNE1, CD79A, CDK6, CREB3L2, CREBBP, CRTC1, CTNNB1, CUX1, CXCR4, DAXX, DDB2, DDX5, DDX6, DICER1, DNM2, EGFR, EIF3E, ELF4, ELK4, EPAS1, EPS15, ERBB2, ERBB3, ERBB4, ESR1, EZR, FANCD2, FAS, FBXO11, FGFR1, FGFR2, FGFR3, FGFR4, FLT3, FLT4, FOXA1, FUBP1, GNA11, GNAQ, GNAS, GRIN2A, HEY1, HNRNPA2B1, HOXA11, HRAS, IL6ST, JAK1, JAK2, JAK3, KDM6A, KDR, KIT, KLF4, KMT2C, KMT2D, KRAS, LCK, LEF1, MAP3K13, MED12, MEN1, MTOR, MYC, MYD88, NDRG1, NONO, NOTCH1, NOTCH2, NRAS, NT5C2, NTRK1, NTRK2, NTRK3, PAX3, PAX5, PAX7, PAX8, PIK3CA, PIM1, PLCG1, POU2AF1, POU5F1, PPARG, PPM1D, PRKACA, PRKAR1A, PTK6, RAC1, RAF1, RAP1GDS1, ROS1, SIX1, SLC34A2, SMAD3, SUZ12, TBL1XR1, TCF7L2, TCL1A, TERT, TP63, UBR5, XPO1.
Genes that are unique to proliferative signaling:
CACNA1D, CCND2, CDK6, CREB3L2, CRTC1, EPS15, GNA11, GNAQ, JAK3, MAP3K13, NTRK1, NTRK2, NTRK3, ROS1.
Examples of gene mutations for which FDA approved medications are available according to OncoKBCitation12:
ABL1: imatinib, dasatinib, nilotinib.
BRAF: vemurafenib + cobimetinib, dabrafenib + trametinib, binimetinib + encorafenib.
EGFR: erlotinib, gefitinib, afatinib, osimertinib, dacomitinib.
ERBB2: trastuzumab, ado-trastuzumab emtansine, lapatinib, pertuzumab + trastuzumab, lapatinib + trastuzumab, neratinib.
FGFR2, FGFR3: erdafitinib
KIT: regorafenib, imatinib, sunitinib.
KRAS (wild type): cetuximab, panitumumab, regorafenib.
NTRK1, NTRK2, NTRK3 (fusions): larotrectinib
ROS1: crizotinib
The hallmark of proliferative signaling is characterized by the upregulation of specific signaling pathways that promote cell proliferation in cancer cells. For example, ROS1 is a transmembrane protein with intracellular tyrosine kinase activity that is often affected by mutations or chromosomal rearrangement in different types of cancer, leading to the activation of signaling pathways that stimulate cell proliferation. The use of Crizotinib, a small-molecule tyrosine kinase inhibitor, has shown an objective response rate in patients with advanced ROS1-rearranged non-small cell lung carcinoma.
Alterations in several genes have been associated with proliferative signaling, as listed in COSMIC. These genes include those involved in various signaling pathways, such as ABL1, AKT1, ALK, BRAF, CCNE1, CDK6, EGFR, ERBB2, KRAS, NRAS, PIK3CA, RAF1, and ROS1. Some of these genes have also been linked to clinical significance, such as mutations in EGFR, which are common in non-small cell lung cancer (NSCLC) and are associated with a better response to EGFR tyrosine kinase inhibitors (TKIs) like erlotinib, gefitinib, and osimertinib. Mutations in BRAF have been found in several cancer types, including melanoma and colorectal cancer, and are associated with a poor prognosis. FDA-approved medications are available for some of the gene mutations mentioned, such as imatinib, dasatinib, and nilotinib for ABL1 mutations and vemurafenib + cobimetinib, dabrafenib + trametinib, and binimetinib + encorafenib for BRAF mutations.
Moreover, some genes are unique to proliferative signaling, including CACNA1D, CCND2, CDK6, CREB3L2, CRTC1, EPS15, GNA11, GNAQ, JAK3, MAP3K13, NTRK1, NTRK2, NTRK3, and ROS1. Mutations in these genes may serve as potential therapeutic targets for developing new cancer treatments.
Evasion of Suppression of Growth
One of the hallmarks of cancer is evasion of suppression of growth. Normally, tumor suppressor genes have the role of preventing cancerous growth. Repression of tumor suppressor genes leads to cells acquiring the potential to overcome the signaling pathways that negatively control growth and proliferation.
According to COSMIC, alterations in the following genes are associated with evasion of suppression of growth:
ABI1, AKT1, AMER1, APC, ARID1A, ARID2, ATP2B3, ATR, AXIN1, AXIN2, BCOR, BLM, CAMTA1, CBFA2T3, CBLB, CCDC6, CDC73, CDKN2A, CIC, CNOT3, CTNNB1, CUX1, CYLD, DDX10, DDX3X, EBF1, ELF4, ELL, EP300, EPAS1, ERBB4, ESR1, FAT1, FAT4, FCGR2B, FLT3, FUS, GNAS, GPC3, GRIN2A, HNF1A, HOXA11, KLF4, KMT2C, LATS1, LATS2, LRP1B, MEN1, NCOR2, NDRG1, NF1, NF2, NOTCH2, PAX3, PDCD1LG2, PER1, PHOX2B, PIK3R1, PMS2, PPARG, PTEN, PTK6, PTPRT, QKI, RABEP1, RB1, RBM10, RHOA, RHOH, RNF43, RUNX1T1, SMAD2, SMAD3, SPOP, TBL1XR1, TET1, TGFBR2, TP53, TSC1, TSC2, VHL, ZFHX3.
Genes that are unique to evasion of suppression of growth:
AMER1, ATP2B3, AXIN2, BCOR, CAMTA1, EBF1, FCGR2B, PDCD1LG2, PHOX2B, PTPRT, TSC1, TSC2, ZFHX3.
Examples of gene mutations for which FDA approved medications are available according to OncoKBCitation12:
TSC1, TSC2: everolimus.
The evasion of suppression of growth hallmark is a critical characteristic of cancer cells that allows them to avoid the normal mechanisms of cell growth regulation. This hallmark category involves the loss or inactivation of tumor suppressor genes, which are responsible for controlling cell proliferation and apoptosis. The list of genes associated with evasion of suppression of growth provided by COSMIC includes many well-known tumor suppressor genes such as TP53, PTEN, and RB1, as well as several others not commonly associated with this hallmark.
Of particular interest are the genes unique to evasion of suppression of growth, as they may represent key drivers of this hallmark category. For example, TSC1 and TSC2 are two genes that are unique to this category and have FDA-approved targeted therapies available (everolimus). These genes are involved in the regulation of the mTOR signaling pathway, which is frequently dysregulated in cancer and plays a critical role in cell growth and survival. The identification of these genes as unique to this hallmark category suggests that targeting the mTOR pathway may be an effective strategy for treating cancers with mutations in these genes.
Other genes unique to evasion of suppression of growth include AMER1, ATP2B3, AXIN2, BCOR, CAMTA1, EBF1, FCGR2B, PDCD1LG2, PHOX2B, PTPRT, and ZFHX3. While the specific roles of these genes in evasion of suppression of growth are not fully understood, they represent potential targets for the development of new cancer therapies.
In terms of clinical significance, mutations in genes associated with evasion of suppression of growth are frequently observed in a variety of cancers, including breast, colorectal, lung, and pancreatic cancers. These mutations are often associated with a more aggressive tumor phenotype and poorer patient outcomes. Therefore, understanding the molecular mechanisms underlying evasion of suppression of growth and identifying novel targets for therapy is critical for improving cancer treatment and patient outcomes.
Escaping Immune Response to Cancer
Normally, any abnormal cell growth would be detected and dealt with by one or more of the different arms of the immune system. Cancerous cells can evade the immune system by “camouflage and sabotage.”Citation13 Cancerous cells can express surface antigens known to the immune system as “self” and can hide “foreign” antigens.
According to COSMIC, alterations in the following genes are associated with escaping immune response to cancer:
B2M, CBLB, CIITA, CTNNB1, CUX1, EGFR, ESR1, HIF1A, HRAS, JAK1, MYC, PTEN, RAC1, RB1, TNFAIP3, TP53.
Genes that are unique to escaping immune response to cancer:
B2M, CIITA.
Examples of gene mutations for which FDA approved medications are available according to OncoKBCitation12:
EGFR: Erlotinib, gefitinib, afatinib, osimertinib, dacomitinib.
The evasion of immune response to cancer is a hallmark of cancer that enables cancer cells to evade detection and destruction by the immune system. The immune system can recognize and eliminating cancer cells, but cancer cells can utilize various mechanisms to evade immune detection, including the expression of surface antigens known to the immune system as “self” and the suppression of immune response by various signaling pathways.
The COSMIC database lists several genes that are associated with escaping immune response to cancer, including B2M, CBLB, CIITA, CTNNB1, CUX1, EGFR, ESR1, HIF1A, HRAS, JAK1, MYC, PTEN, RAC1, RB1, TNFAIP3, and TP53. Among these genes, B2M and CIITA are unique to escaping immune response to cancer. B2M is a component of the MHC class I complex that presents antigens to the immune system, while CIITA is a transcriptional regulator that controls the expression of MHC class II genes.
Mutations in these genes can enable cancer cells to evade immune detection and contribute to tumor growth and progression. For instance, mutations in EGFR can activate signaling pathways that suppress immune response, while mutations in TP53 can lead to the upregulation of PD-L1, a checkpoint inhibitor that suppresses T cell activity.
OncoKB lists several FDA-approved medications that target gene mutations associated with escaping immune response to cancer. For instance, EGFR inhibitors such as erlotinib, gefitinib, afatinib, osimertinib, and dacomitinib can inhibit the EGFR signaling pathway and restore immune response to cancer cells expressing EGFR mutations. Other medications, such as checkpoint inhibitors that target PD-L1 or CTLA-4, can also restore immune response to cancer cells by blocking immune checkpoint pathways that suppress T cell activity.
Escaping Programmed Cell Death
The viability of a certain cell in a certain tissue depends on the balance between pro-apoptotic and anti-apoptotic factors influencing the cell fate. Cancer cells acquire properties that enable them to escape programmed cell death via different mechanisms that lead to an imbalance that favors anti-apoptotic trait.
According to COSMIC, alterations in the following genes are associated with escaping programmed cell death:
ABL1, ACKR3, ACVR1, AKT1, ALK, APC, AR, ARID1A, ATM, ATP1A1, ATR, AXIN1, BAP1, BCL10, BIRC3, BLM, BRAF, BRCA1, BRCA2, BRD4, BTK, BUB1B, CALR, CARD11, CARS, CASC5, CASP8, CCDC6, CD79B, CDC73, CDH11, CDKN2A, CHEK2, CNBP, CNOT3, CREBBP, CTNNB1, CUX1, CYLD, DAXX, DDB2, DDIT3, DDX3X, DDX5, DDX6, DICER1, EGFR, EIF3E, ELF4, ELK4, EP300, EPAS1, ERBB2, ERBB3, ERBB4, ERCC2, ERCC3, ERCC5, ETNK1, ETV6, EZR, FANCD2, FAS, FAT1, FBXO11, FCGR2B, FGFR2, FGFR3, FLT3, FLT4, FUBP1, HIF1A, HIP1, HNF1A, HNRNPA2B1, HOXA11, HRAS, JAK1, JAK2, KEAP1, KIT, KLF4, KRAS, LATS1, LATS2, LCK, MAPK1, MEN1, MTOR, MYC, MYD88, NCOR2, NF1, NFE2L2, NOTCH1, NOTCH2, NRAS, NT5C2, PAX3, PAX5, PAX8, PBRM1, PBX1, PER1, PIK3CA, PIK3CB, PIM1, PLCG1, PMS2, PPM1D, PRDM16, PRKACA, PRKAR1A, PSIP1, PTEN, PTK6, PTPN13, QKI, RAC1, RAD21, RAF1, RB1, RBM10, RECQL4, RHOA, RHOH, RNF43, SFPQ, SIX1, SMAD2, SMAD3, SPOP, TCF7L2, TCL1A, TERT, TET1, TP53, TP63, TRAF7, UBR5, VHL, XPO1.
Genes that are unique to escaping programmed cell death:
BIRC3, CARD11, CARS, CD79B, ETNK1, FCGR2B, HIP1, KEAP1, PRDM16, SFPQ, TRAF7.
Examples of gene mutations for which FDA approved medications are available according to OncoKBCitation12:
ABL1: imatinib, dasatinib, nilotinib.
ALK: crizotinib, ceritinib, alectinib, lorlatinib, brigatinib.
BRAF: vemurafenib + cobimetinib, dabrafenib + trametinib, binimetinib + encorafenib.
BRCA1, BRCA2: rucaparib, niraparib.
EGFR: erlotinib, gefitinib, afatinib, osimertinib, dacomitinib.
ERBB2: trastuzumab, ado-trastuzumab emtansine, lapatinib, pertuzumab + trastuzumab, lapatinib + trastuzumab, neratinib.
FGFR2: erdafitinib
FGFR3: erdafitinib
KIT: regorafenib, imatinib, sunitinib.
KRAS (wild type): cetuximab, panitumumab, regorafenib.
The Hallmark category “Escaping Programmed Cell Death” refers to the ability of cancer cells to avoid undergoing programmed cell death, or apoptosis, which is a natural process that eliminates damaged or abnormal cells. This hallmark is important for cancer development and progression because cancer cells acquire the ability to evade apoptosis through various mechanisms that favor anti-apoptotic traits.
The list of genes associated with this hallmark, as provided by COSMIC, includes many well-known cancer-related genes, such as TP53, PTEN, BRAF, KRAS, and EGFR. Some of these genes have FDA-approved drugs available for specific mutations, highlighting the clinical significance of targeting specific genetic alterations in cancer treatment.
Additionally, there are several genes unique to this hallmark, including BIRC3, CARD11, CARS, CD79B, ETNK1, FCGR2B, HIP1, KEAP1, PRDM16, SFPQ, and TRAF7. These genes may provide potential therapeutic targets for cancer treatment.
It is also interesting to note that some of the genes associated with this hallmark are involved in other hallmarks of cancer. For example, TP53 is also associated with genomic instability and mutation, and KRAS is associated with sustained proliferative signaling. This suggests that cancer is a complex disease with interconnected pathways and mechanisms.
Overall, the identification of genes associated with escaping programmed cell death provides insights into the mechanisms of cancer development and progression and highlights potential targets for cancer treatment.
Cell Replicative Immortality
Under normal circumstances, and after several rounds of replication, normal cells eventually reach senescence and then cell death. One of the mechanisms regulating this concept is the shortening telomeres. Cancer cells can manipulate this mechanism in order to acquire replicative immortality. For example, upregulation of telomerase in certain malignancies can lead to replicative immortality.
According to COSMIC, alterations in the following genes are associated with cell replicative immortality:
ARID1A, ATM, AXIN1, BCL11A, BRD4, BTK, CCNE1, CDK4, CTNNB1, DICER1, ELF4, ELL, ERG, FAS, FGFR1, HEY1, KDR, KLF4, KMT2C, KMT2D, KRAS, MLLT10, MYC, NDRG1, NOTCH1, NRAS, PIK3R1, POU5F1, PTEN, RNF43, SIX1, SLC34A2, SMAD2, STAG2, TERT, TP53, WIF1, ZBTB16.
Genes that are unique to cell replicative immortality:
BCL11A, CDK4, MLLT10, WIF1, ZBTB16.
Examples of gene mutations for which FDA approved medications are available according to OncoKBCitation12:
KRAS (wild type): cetuximab, panitumumab, regorafenib.
KRAS G12C: Sotorasib and adagrasib.
The Hallmark category “Cell Replicative Immortality” is an important biological process in cancer development. The list of genes associated with cell replicative immortality includes many well-known cancer genes, such as KRAS, TP53, and PTEN, as well as several less studied genes. The unique genes in this category, BCL11A, CDK4, MLLT10, WIF1, and ZBTB16, are interesting candidates for further study as they may play a more specific role in promoting replicative immortality.
Of particular interest are the genes that are correlated with clinical significance. In this regard, mutations in KRAS have been found in many different types of cancer and are associated with poor prognosis. However, FDA-approved medications such as cetuximab, panitumumab, and regorafenib are available for patients with wild-type KRAS. These drugs can help to target cancer cells by blocking the activity of the mutant KRAS protein. In addition, sotorasib and adagrasib are two recently developed targeted therapies for patients with KRAS-mutated cancers. Both drugs work by inhibiting KRAS G12C, a specific mutation in the KRAS gene that is present in about 13% of non-small cell lung cancers and other solid tumors. Sotorasib was approved by the US FDA in May 2021 for the treatment of advanced or metastatic non-small cell lung cancer with the KRAS G12C mutation, while adagrasib is currently being studied in clinical trials for various types of KRAS G12C-mutant cancers. These drugs have shown promising results in clinical trials, offering new treatment options for patients with this specific KRAS mutation.
Another gene of interest is TERT, which is a component of the telomerase enzyme that maintains the length of telomeres. Mutations in TERT have been linked to many different types of cancer, including melanoma, lung cancer, and bladder cancer. In some cases, these mutations can lead to the upregulation of telomerase activity, which can promote replicative immortality.
In conclusion, the genes associated with cell replicative immortality are diverse and include many well-studied cancer genes, as well as several less characterized genes. The clinical significance of these genes varies depending on the type of cancer and the specific mutation involved. Further research into these genes and their role in promoting replicative immortality may lead to the development of new targeted therapies for cancer patients.
Tumor Promoting Inflammation
Cells of the innate and adaptive immune system are found in the tumor bed, albeit with different degrees of involvement. Through cross-talk signaling, cancerous cells influence the inflammatory cells in the tumor bed to foster tumor growth. Ironically, the tumor ends up benefiting from cytokines and other growth factors secreted by the infiltrating immune cells.
According to COSMIC, alterations in the following genes are associated with tumor promoting inflammation:
APOBEC3B, ATM, CASP8, CYLD, DDIT3, EPAS1, FANCD2, HNRNPA2B1, HRAS, IL6ST, JAK2, KDR, KRAS, MYD88, PBRM1, RHOA, TNFAIP3, TP53.
Genes that are unique to tumor promoting inflammation:
None.
Examples of gene mutations for which FDA approved medications are available according to OncoKBCitation12:
JAK2: ruxolitinib
KRAS (wild type): cetuximab, panitumumab, regorafenib.
Several genes associated with tumor promoting inflammation have been found to be correlated with clinical significance. For instance, mutations in the TP53 gene are commonly found in many types of cancer and are associated with poorer prognosis and decreased survival rates. Mutations in KRAS are also prevalent in many types of cancer and are linked to a more aggressive disease course.
As stated earlier, recently, drugs targeting specific mutations in KRAS have shown promise in clinical trials. Sotorasib and adagrasib are two such drugs that have been approved by the FDA for the treatment of advanced non-small cell lung cancer (NSCLC) with KRAS mutations. These drugs are part of a new class of targeted therapies known as KRAS G12C inhibitors, which are designed to block the activity of the mutant KRAS protein and prevent it from promoting tumor growth. The development of these drugs represents a major breakthrough in the treatment of KRAS-mutant cancers, which have been historically difficult to treat with conventional therapies.
It is interesting to note that according to COSMIC, there are no genes that are unique to tumor promoting inflammation, which means that the genes involved in this hallmark category also play a role in other biological processes. This highlights the complexity of cancer and the fact that hallmark categories do not act independently, but rather interact with each other to promote tumor growth and progression.
Activating Invasion and Metastasis
By acquiring properties of invasion and metastasis, cancer cells can propagate and reach distant organs. One of the major mechanisms of acquiring this property is the revival and exploitation of the epithelial-mesenchymal transition (EMT) system.
According to COSMIC, alterations in the following genes are associated with invasion and metastasis:
ABI1, ABL1, ACKR3, ACVR1, AFF4, AKT1, ALK, APC, ARID1A, ARNT, ATM, ATP1A1, BAP1, BCL10, BCL9, BCL9L, BCORL1, BMPR1A, BRCA1, BUB1B, CALR, CASP8, CBFB, CBL, CBLB, CCNB1IP1, CCND1, CD79A, CDC73, CDH1, CDH11, CDKN2A, CIC, CLTCL1, CNBP, CREB3L1, CREBBP, CTNNB1, CUX1, CXCR4, CYLD, DAXX, DDB2, DDR2, DDX3X, DDX5, DICER1, DNM2, EGFR, EIF3E, EPAS1, ERBB2, ERBB3, ESR1, ETV6, EZR, FANCD2, FAS, FAT1, FAT4, FBXO11, FGFR1, FGFR2, FGFR4, FHIT, FLT4, FOXA1, FUBP1, FUS, GAS7, GPC3, GRIN2A, HIF1A, HNF1A, HNRNPA2B1, HOXA11, HRAS, KDM6A, KDR, KIT, KLF4, KMT2C, KMT2D, KRAS, LATS2, LEF1, LIFR, LRP1B, MAPK1, MED12, MTOR, MYC, MYD88, NDRG1, NF1, NF2, NONO, NOTCH2, NRAS, PAX3, PAX5, PAX7, PAX8, PBRM1, PHOX2B, PIK3CA, PIK3R1, PIM1, PLCG1, PMS2, POU5F1, PREX2, PRKAR1A, PSIP1, PTEN, PTK6, PTPN13, RABEP1, RAC1, RAD21, RAF1, RAP1GDS1, RB1, RECQL4, RHOA, RHOH, SIX1, SLC34A2, SMAD2, SMAD3, SPOP, SUZ12, TBL1XR1, TCF7L2, TERT, TET1, TGFBR2, TP53, TP63, UBR5, VHL, WAS.
Genes that are unique to invasion and metastasis:
BCORL1, BMPR1A, CBFB, CCNB1IP1, CLTCL1, DDR2, GAS7, LIFR, PREX2, WAS.
Examples of gene mutations for which FDA approved medications are available according to OncoKBCitation12:
ABL1: imatinib, dasatinib, nilotinib.
ALK: crizotinib, ceritinib, alectinib, lorlatinib, brigatinib.
BRCA1, BRCA2: rucaparib, niraparib.
EGFR: Erlotinib, gefitinib, afatinib, osimertinib, dacomitinib.
ERBB2: trastuzumab, ado-trastuzumab emtansine, lapatinib, pertuzumab + trastuzumab, lapatinib + trastuzumab, neratinib.
FGFR2: erdafitinib
KIT: regorafenib, imatinib, sunitinib.
KRAS (wild type): cetuximab, panitumumab, regorafenib.
The activating invasion and metastasis hallmark category refers to the acquisition of properties of invasion and metastasis by cancer cells, which enables them to propagate and reach distant organs. One of the primary mechanisms of acquiring these properties is the activation of the epithelial-mesenchymal transition (EMT) system.
The list of genes associated with invasion and metastasis, according to COSMIC, is quite extensive and diverse, encompassing a wide range of cellular processes such as signal transduction, DNA damage response, transcriptional regulation, cell cycle control, and epigenetic regulation. Among the genes listed, some have well-established clinical significance in various cancers. For example, mutations in ALK, EGFR, and ERBB2 are commonly observed in lung and breast cancers and are associated with targeted therapy response.
Interestingly, the list also includes genes that are unique to the invasion and metastasis hallmark category. These genes may represent potential targets for therapeutic interventions aimed at preventing or treating cancer metastasis.
In summary, the activating invasion and metastasis hallmark category involves a complex interplay of multiple genes and cellular processes. The genes identified in this category represent potential targets for developing novel therapies aimed at preventing or treating cancer metastasis, and some have well-established clinical significance in various cancers.
Inducing Angiogenesis
In order to keep up with supporting cell growth, tumors rely on angiogenesis to sustain the viability of cancerous cells. Neovascularization functions as an infrastructure that allows tumors to receive nutrients and to get rid of metabolic breakdown. In addition, newly formed endothelial cells contribute to the cross-talk signaling between the tumor cells and the cells of the microenvironment.
According to COSMIC, alterations in the following genes are associated with angiogenesis:
ABL1, ACKR3, AKT1, ARNT, BCL9, BRCA1, CALR, CASP8, CDKN2A, CREB3L1, CTNNB1, CUX1, CYLD, DDX6, DICER1, DNM2, EGFR, EIF3E, ELL, EPAS1, HIF1A, HRAS, KDR, KRAS, LCK, MTOR, MYC, NAB2, NF1, NOTCH1, PBX1, PIK3CA, PIM1, PLCG1, RAC1, RAF1, RNF213, RUNX1T1, SDHA, SIX1, TERT, TP53, UBR5, VHL.
Genes that are unique to angiogenesis:
NAB2, RNF213.
Examples of gene mutations for which FDA approved medications are available according to OncoKBCitation12:
ABL1: imatinib, dasatinib, nilotinib.
BRCA1, BRCA2: rucaparib, niraparib.
EGFR: erlotinib, gefitinib, afatinib, osimertinib, dacomitinib.
KRAS (wild type): cetuximab, panitumumab, regorafenib.
Angiogenesis is a complex biological process in which new blood vessels are formed from preexisting ones. This hallmark is critical for tumor growth and survival, as it enables the tumor to receive nutrients and oxygen necessary for its survival. The list of genes associated with angiogenesis provided by COSMIC includes several well-known oncogenes and tumor suppressor genes, such as EGFR, KRAS, TP53, and PIK3CA. Mutations in these genes have been implicated in a wide range of cancers, including lung, breast, colorectal, and pancreatic cancer.
Two genes, NAB2 and RNF213, were found to be unique to angiogenesis. NAB2 is a transcriptional repressor that has been implicated in the regulation of angiogenesis, and RNF213 is a gene that encodes a protein that is involved in the regulation of blood vessel formation.
Overall, these findings highlight the importance of angiogenesis in tumor growth and the potential for targeted therapies to disrupt this process and inhibit tumor growth. Additionally, the identification of unique genes such as NAB2 and RNF213 may provide new targets for the development of novel therapeutics.
Genome Instability and Mutations
Uncontrolled cancer cell growth leads to cells accumulating (often to a varying degree in heterogeneous sub-clones) a cascade of mutations that support tumor survival by inactivating tumor suppressor genes, leading to disruption of the DNA-machinery responsible for maintenance and repair of cells.
According to COSMIC, alterations in the following genes are associated with genome instability and mutations:
ABL1, AKT1, APC, APOBEC3B, ARID1A, ARID2, ATM, ATR, ATRX, BAP1, BCL9L, BCL10, BLM, BRCA1, BRCA2, BRIP1, BTK, BUB1B, CASC5, CCDC6, CCND1, CDK12, CHD4, CHEK2, CLTC, CREBBP, CTCF, CTNNB1, CUX1, DAXX, DDB2, DICER1, ERCC2, ERCC3, ERCC4, ERCC5, EWSR1, FANCD2, FBXW7, FHIT, HRAS, KMT2C, LATS1, LATS2, LMNA, MED12, MYC, NCOR2, PBRM1, PIK3CB, PMS2, POT1, PPM1D, PPP6C, PSIP1, PTEN, RAC1, RAD21, RANBP2, RB1, RECQL4, RHOA, SIX1, SPOP, STAG2, TERT, TP53, UBR5, USP8.
Genes that are unique to genome instability and mutations:
ATRX, BRIP1, CHD4, CLTC, CTCF, ERCC4, EWSR1, FBXW7, LMNA, POT1, PPP6C, RANBP2.
Examples of gene mutations for which FDA approved medications are available according to OncoKBCitation12:
ABL1: imatinib, dasatinib, nilotinib.
BRCA1, BRCA2: rucaparib, niraparib.
The Hallmark category of Genome Instability and Mutations is particularly important in the development and progression of cancer. The accumulation of mutations and genome instability are key features of cancer cells that enable them to evade growth control mechanisms and develop resistance to therapy.
The list of genes associated with genome instability and mutations provided by COSMIC includes many well-known tumor suppressor genes such as TP53, PTEN, RB1, and BRCA1/2. Mutations in these genes have been shown to increase the risk of developing cancer and are often associated with a poor prognosis. Other genes, such as ABL1, AKT1, and HRAS, have also been linked to cancer development and progression.
It is worth noting that several of the genes unique to this category, such as ATRX, BRIP1, and POT1, are involved in the maintenance of telomeres, the protective caps at the ends of chromosomes. Telomere dysfunction has been implicated in the development of cancer and is a potential therapeutic target.
Regarding the clinical significance of these genes, there are several FDA-approved drugs that target mutations in the genes listed in this category. For example, imatinib, dasatinib, and nilotinib target ABL1 mutations, while rucaparib and niraparib target BRCA1/2 mutations. This highlights the importance of identifying specific mutations in cancer patients to guide personalized treatment options.
In conclusion, the Hallmark category of Genome Instability and Mutations is a critical aspect of cancer biology, and the list of genes associated with this category provides important insights into the underlying mechanisms of cancer development and progression. The availability of FDA-approved drugs that target specific mutations in these genes highlights the importance of genomic profiling in guiding personalized treatment options for cancer patients.
Change of Cellular Energetics
Given the uncontrolled growth of cells crowding the tumor bed, one of the hallmarks of cancer is the ability of neoplasms to tap into alternative resources to fuel and sustain growth. Cancer cells often amplify aberrant metabolic pathways in order to keep up with growth demands.
According to COSMIC, alterations in the following genes are associated with change of cellular energetics:
ABL1, AKT1, ARNT, ATR, BAP1, BRCA1, CBFA2T3, CCDC6, CCND1, CDH1, CDK12, CIC, CTNNB1, DDX3X, EGFR, ERBB2, HIF1A, IDH1, IDH2, JAK2, KMT2D, KRAS, MAPK1, MTOR, MYC, NF2, NFE2L2, NONO, NOTCH1, PAFAH1B2, PBRM1, PBX1, PICALM, PIM1, PPARG, PTEN, RB1, SDHA, SIX1, TFE3, TP53, USP8, VHL.
Genes that are unique to change in cellular energetics:
IDH1, IDH2, PAFAH1B2, PICALM, TFE3.
Examples of gene mutations for which FDA approved medications are available according to OncoKBCitation12:
ABL1: imatinib, dasatinib, nilotinib.
BRCA1, BRCA2: rucaparib, niraparib.
EGFR: Erlotinib, gefitinib, afatinib, osimertinib, dacomitinib.
ERBB2: trastuzumab, ado-trastuzumab emtansine, lapatinib, pertuzumab + trastuzumab, lapatinib + trastuzumab, neratinib.
IDH1: ivosidenib
IDH2: enasidenib
KRAS (wild type): cetuximab, panitumumab, regorafenib.
The hallmark of Change of Cellular Energetics reflects the altered metabolism of cancer cells, which allows them to generate energy to support their growth and survival. Cancer cells undergo metabolic reprogramming to support their growth, proliferation, and survival in the hypoxic, nutrient-limited environment of the tumor. They preferentially use glycolysis to produce energy even under normal oxygen levels, known as the Warburg effect. In addition, cancer cells rely on alternative energy sources, such as glutamine and fatty acids, and suppress normal metabolic processes, such as oxidative phosphorylation.
Some of the unique genes in this category, such as IDH1, IDH2, PAFAH1B2, PICALM, and TFE3, are involved in more specific aspects of cellular metabolism. IDH1 and IDH2 are enzymes involved in the metabolism of isocitrate and have been implicated in the pathogenesis of some types of glioma and leukemia. Mutations in IDH1 and IDH2 result in the production of an oncometabolite, which can disrupt cellular metabolism and promote tumorigenesis. PAFAH1B2 and PICALM are involved in the regulation of lipid metabolism and may play a role in the metabolic reprogramming of cancer cells. TFE3 is a transcription factor that regulates lysosomal biogenesis and autophagy, which are important for cellular homeostasis and nutrient recycling.
The FDA-approved medications mentioned in this category target a variety of signaling pathways and metabolic enzymes. For example, Ivosidenib and enasidenib target IDH1 and IDH2, respectively, and inhibit the production of the oncometabolite that contributes to the pathogenesis of some types of cancer.
Overall, the genes associated with Change of Cellular Energetics reflect the complex metabolic adaptations that occur in cancer cells to support their growth and survival. Targeting these metabolic pathways and enzymes with specific inhibitors represents a promising therapeutic strategy for the treatment of cancer.
Discussion
The field of studying the omics of cancer is continuously evolving. The field encompasses the genome, the transcriptome, the proteome, as well as the microbiome and the influence of pharmacogenomics. Cell functionality relies on a network of signaling pathways. Cancer cells manipulate several of those pathways to acquire a survival advantage. Genomics alone do not give a full explanation of cancer behavior. For example, non-coding RNAs (ncRNA) have an essential role in cancer genesis. Those ncRNAs can potentially remodel signaling pathways by activating or repressing downstream effectors.Citation14 We realize the limitation of “genomic profiling” of the hallmarks of cancer. However, the 10 hallmarks of cancer could potentially help in categorizing the omics of cancer in order to find common ground and differences in deepening the understanding of targeted therapeutic approaches.
When we looked at the gene alterations associated with each of the 10 hallmarks of cancer, we identified genes unique to each hallmark and genes that are shared by more than one hallmark. (). This can be explained partly because of the overlap of different pathways at certain points in the cancer genesis and partly possibly because of redundancy in some of the hallmarks. Nine out of ten hallmarks of cancer did have association with alterations in genes unique to each specific hallmark of cancer. However, based on COSMIC data base, the only hallmark of cancer that did not have its own unique genes was “tumor promoting inflammation.” Perhaps, “tumor promoting inflammation” is a subcomponent of one of the other nine hallmarks of cancer. Another possibility to explain the lack of genes uniquely associated with this hallmark could be the insufficient gene annotation in COSMIC (vis-à-vis the 10 hallmarks of cancer). Further studies and annotation would be useful for this purpose. However, re-annotating all the genes in COSMIC is beyond the scope of this manuscript.
Although targeted therapy is a step forward in laying the ground for precision design for treatment of the different types of cancer, the explanation of relapse and recurrence, even years after remission, remains to be fully explored. Studies attributed relapse/recurrence to cancer cell dormancy (also described as latency)Citation15; some malignant cells remain quiescent for undetermined time then get activated and start to proliferate leading to the appearance of new foci of tumor in the patient. This state of dormancy is reversible and includes different stages, from establishment and maintenance to breaking the dormancy. Dormant cancerous cells often can resist chemotherapy and targeted therapies.Citation16 The molecular mechanism is not fully understood, nevertheless it was found that the transforming growth factor-B (TGF-B) family is involved in regulating this process of cancer cell dormancy.Citation15,Citation17 The TGF-B family includes TGF-Bs and bone morphogenetic proteins (BMPs). TGF-B has a double contradictive function: it acts as tumor promoter and tumor suppressor.Citation18 This capacity to regulate two opposing phenomenon makes of this molecular family a main regulator of dormancy (). Some mutations in the TGF-B gene are associated with cancer. In addition, TGF-B activates tumor suppressor genes such as p15, p21 and p27.Citation19,Citation20 The TGF-B pathway activates type I and type II kinases receptors which phosphorylates the complex of SMAD2/3 and SMAD1/5 which interact with SMAD4. This canonical pathway regulates transcription of certain genes. SMAD6/7 have an inhibitory effect on this pathway, which means that overexpression of SMAD6/7 may be related to the escape from dormancy and subsequently inducing proliferation of tumor. Alterations in TGF-B affects also non-canonical pathways such as the ones related to Ras, PI3K/AKT, p53, and epithelial mesenchymal transition (EMT).Citation21
Figure 1. Schematic summary of the different interactions leading to establishment and maintenance of dormancy versus breaking dormancy and activating proliferation
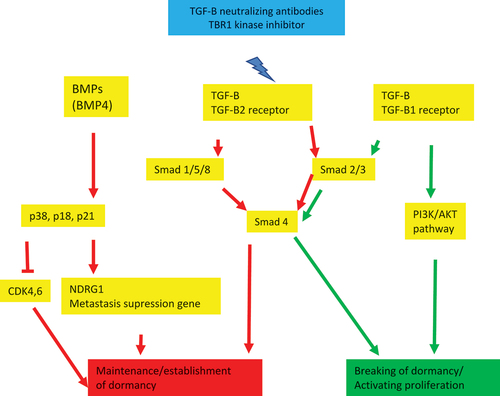
Experimental studies showed that TGF-B1 ligands inhibit cell dormancy and TGF-B2 ligands induce it. This reveals also that the state of latency of cancer cells depends on the type of TGF-B receptors found in tumor cells (TBR1, TBR2, TBR3). Thrombospondin 1 (TSP-1) is one of the TGF-B pathway regulators that inhibit cell proliferation by inducing tumor cell dormancy. BMPs (BMP4, BMP7) are mainly related to inducing dormancy and not inhibition of dormancy unlike TGF-B. This pathway activates agents such as p38 and p18 and p21 which induces CDK6 and CDK inhibition or upregulate the metastasis suppression gene NDRG1. Some BMPs such as BMP2 and BMP4 stimulate tumor growth. These ligands and receptors (TGF-B and BMP) are present in tumor cells and tumor niche which means that tumor dormancy phenomenon can be regulated by autocrine effects of tumors cells and by stromal cells in the tumor environment (metastasis sites such as bone marrow, lung, etc.).Citation15,Citation22
These findings opened the door to establish therapeutic theories targeting cell dormancy in cancer either by keeping the dormant cells in that state or eliminating them. The first theory would involve a lifetime treatment which may lead to serious adverse effects related to tissue and organ development and function such as the immune system. Targetable gene alterations and gene signatures specific to dormant cells are found. Nowadays strategies are being studied regarding the elimination of dormant cells such as TBR1 kinase inhibitor, TGF-B neutralizing antibody, BMP inhibitor, etc.Citation15,Citation23 Nevertheless, these options may still lead to uncontrolled cell proliferation and toxicity because the challenge would be in targeting single dormant cells. Perhaps, a more promising strategy would rely on targeting the niche (or “bed”) where dormant cells are present.
Mirroring the heterogeneous population of cancer cells, the microenvironment includes a variety of diverse host cells, cytokines, extracellular matrix proteins, and in specific organs, a plethora of microbiota. For example, the understanding of the role of cancer associated fibroblasts (CAFs), the blood and lymphatic vascular networks, and cells of the adipose tissue is also contributing to the complexity of the interplay and crosstalk between tumor cells and the microenvironment.Citation24–26 For instance, the adipose tissue influences the estrogen levels in postmenopausal patients with breast cancer.
In summary, the study of the omics of cancer is a rapidly evolving field that has the potential to provide a deeper understanding of cancer at the molecular level. While genomics alone cannot explain cancer behavior, integrating other omics such as the transcriptome, proteome, microbiome, and pharmacogenomics may help in identifying common ground and differences in targeted therapeutic approaches. In addition to identifying unique and shared genes associated with each of the 10 hallmarks of cancer, future research could explore the role of non-coding RNAs (ncRNA) in cancer genesis and how they can potentially remodel signaling pathways. Further annotation of genes in COSMIC, particularly related to “tumor promoting inflammation,” may also provide additional insights into the genomic basis of cancer.
Future Directions
Genomics, alone, certainly do not explain all aspects of the 10 hallmarks of cancer. Rather, the role of studies exploring the transcriptome, proteomics, epigenetic changes, non-coding RNAs, noncoding repeats such as ALU families, and the interplay between all those also remain to be analyzed and understood. In addition, non-tumor factors such as the tumor microenvironment cannot be ignored when studying cancer genesis.
Based on (see below), the hallmark entitled “tumor promoting inflammation” has 18 genomic alterations. However, none of those genomic alterations are unique to this particular hallmark (i.e. tumor promoting inflammation). As such, “tumor promoting inflammation” could represent a subset or a subgroup of one of the other nine hallmarks. Perhaps it can be combined with the hallmark titled “escaping immune response to cancer.” Subsequently, we would like to propose to declassify “tumor promoting inflammation” from being a stand-alone hallmark of cancer.
In 2022, HanahanCitation27 added 4 more hallmarks to the previous 10 hallmarks of cancer. Those are unlocking phenotypic plasticity, non-mutational epigenetic reprogramming, senescent cells, and polymorphic microbiomes. Those 4 additional hallmarks have not been added yet to the COSMIC database and as such, we look forward to update our report in the future once those 4 hallmarks are added to the COSMIC database.
In addition, we would like to propose “cancer cell dormancy” and its different interactions with the tumor microenvironment (including the microbiota and the immune system polymorphism) as an “emerging hallmark of cancer.” In terms of future directions, the study of cancer dormancy and its molecular mechanisms is a promising area of research that may help explain cancer relapse and recurrence. While the TGF-B family has been found to be involved in regulating the process of cancer cell dormancy, further studies are needed to fully understand the molecular mechanisms underlying dormancy and the escape from dormancy. Targeted therapeutic strategies aimed at eliminating dormant cells, such as TGF-B neutralizing antibodies,Citation28 are being studied. However, these approaches need further development and testing to ensure their safety and effectiveness in clinical settings. Ultimately, a better understanding of the molecular mechanisms of cancer dormancy could lead to the development of new and improved therapies for cancer patients.Citation29
Disclosure statement
No potential conflict of interest was reported by the author(s).
Correction Statement
This article has been republished with minor changes. These changes do not impact the academic content of the article.
References
- Hanahan D, Weinberg RA. The hallmarks of cancer. Cell. 2000;100:57–14.
- Hanahan D, Weinberg RA. Hallmarks of cancer: the next generation. Cell. 2011;144:646–74.
- Lazebnik Y. What are the hallmarks of cancer? Nat Rev Cancer. 2010;10:232–33.
- Fouad YA, Aanei C. Revisiting the hallmarks of cancer. Am J Cancer Res. 2017;7:1016–36.
- Sonnenschein C, Soto AM. The aging of the 2000 and 2011 hallmarks of cancer reviews: a critique. J Biosci. 2013;38:651–63.
- Tate JG, Bamford S, Jubb HC, Sondka Z, Beare DM, Bindal N, Boutselakis H, Cole CG, Creatore C, Dawson E, et al. COSMIC: the catalogue of somatic mutations in cancer. Nucleic Acids Res. 2019;47(D1):D941–D47.
- Forbes SA, Beare D, Bindal N, Bamford S, Ward S, Cole CG, Jia M, Kok C, Boutselakis H, De T, et al. COSMIC: high-resolution cancer genetics using the catalogue of somatic mutations in cancer. 2016.
- Uguen A, De Braekeleer M. ROS1 fusions in cancer: a review. Future Oncol. 2016;12:1911–28.
- Acquaviva J, Wong R, Charest A. The multifaceted roles of the receptor tyrosine kinase ROS in development and cancer. Biochim Biophys Acta. 2009;1795(1):37–52.
- Xiong Q, Chan JL, Zong CS, Wang LH. Two chimeric receptors of epidermal growth factor receptor and c-Ros that differ in their transmembrane domains have opposite effects on cell growth. Mol Cell Biol. 1996;16(4):1509–18.
- Shaw AT, Ou SH, Bang Y-J, Camidge DR, Solomon BJ, Salgia R, Riely GJ, Varella-Garcia M, Shapiro GI, Costa DB, et al. Crizotinib in ROS1 -rearranged non–small-cell lung cancer. N Engl J Med. 2014;371(21):1963–71. doi:10.1056/NEJMoa1406766.
- Chakravarty D, Gao J, Phillips S, Kundra R, Zhang H, Wang J, Rudolph JE, Yaeger R, Soumerai T, Nissan MH, et al. OncoKB: a precision oncology knowledge base. JCO Precis Oncol. 2017;1(1):1–16. doi:10.1200/PO.17.00011.
- Poschke I, Mougiakakos D, Kiessling R. Camouflage and sabotage: tumor escape from the immune system. Cancer Immunol Immunother. 2011;60(8):1161–71.
- Johnson SM, Grosshans H, Shingara J, Byrom M, Jarvis R, Cheng A, Labourier E, Reinert KL, Brown D, Slack FJ, et al. RAS is regulated by the let-7 microRNA family. Cell. 2005;120(5):635–47.
- Prunier C, Baker D, Ten Dijke P, Ritsma L. TGF-beta family signaling pathways in cellular dormancy. Trends Cancer. 2019;5(1):66–78.
- Naumov GN, Townson JL, MacDonald IC, Wilson SM, Bramwell VH, Groom AC, Chambers AF. Ineffectiveness of doxorubicin treatment on solitary dormant mammary carcinoma cells or late-developing metastases. Breast Cancer Res Treat. 2003;82(3):199–206.
- Oshimori N, Oristian D, Fuchs E. TGF-beta promotes heterogeneity and drug resistance in squamous cell carcinoma. Cell. 2015;160(5):963–76.
- Neel JC, Humbert L, Lebrun JJ. The dual role of TGF-beta in human cancer: from tumor suppression to cancer metastasis. ISRN Mol Biol. 2012;1–28. doi:10.5402/2012/381428.
- Hannon GJ, Beach D. p15INK4B is a potential effector of TGF-beta-induced cell cycle arrest. Nature. 1994;371:257–61.
- Polyak K, Kato JY, Solomon MJ, Sherr CJ, Massague J, Roberts JM, Koff A. p27Kip1, a cyclin-Cdk inhibitor, links transforming growth factor-beta and contact inhibition to cell cycle arrest. Genes Dev. 1994;8(1):9–22.
- Deckers M, van Dinther M, Buijs J, Que I, Lowik C, van der Pluijm G, ten Dijke P. The tumor suppressor Smad4 is required for transforming growth factor beta-induced epithelial to mesenchymal transition and bone metastasis of breast cancer cells. Cancer Res. 2006;66(4):2202–09.
- Suzuki M, Mose ES, Montel V, Tarin D. Dormant cancer cells retrieved from metastasis-free organs regain tumorigenic and metastatic potency. Am J Pathol. 2006;169(2):673–81.
- Gao H, Chakraborty G, Lee-Lim AP, Mo Q, Decker M, Vonica A, Shen R, Brogi E, Brivanlou AH. The BMP inhibitor Coco reactivates breast cancer cells at lung metastatic sites. Cell. 2012;150(4):764–79.
- Whiteside TL. The tumor microenvironment and its role in promoting tumor growth. Oncogene. 2008;27:5904–12.
- Wang M, Zhao J, Zhang L, Wei F, Lian Y, Wu Y, Gong Z, Zhang S, Zhou J, Cao K, et al. Role of tumor microenvironment in tumorigenesis. J Cancer. 2017;8(5):761–73.
- Roma-Rodrigues C, Mendes R, Baptista PV, Fernandes AR. Targeting tumor microenvironment for cancer therapy. Int J Mol Sci. 2019;20(4):840. doi:10.3390/ijms20040840.
- Hanahan D. Hallmarks of cancer: new dimensions. Cancer Discov. 2022;12(1):31–46. doi:10.1158/2159-8290.CD-21-1059.
- Huang C-Y, Chung C-L, Hu T-H, Chen J-J, Liu P-F, Chen C-L. Recent progress in TGF-β inhibitors for cancer therapy. Biomed Pharmacother Biomed Pharmacotherapie. 2021;134:111046. doi:10.1016/j.biopha.2020.111046.
- Batlle E, Massagué J. Transforming growth factor-β signaling in immunity and cancer. Immunity. 2019;50(4):924–40. doi:10.1016/j.immuni.2019.03.024.