Abstract
Sepsis is a common and fatal disease, especially in critically ill patients. Sepsis-associated encephalopathy (SAE) is a diffuse brain dysfunction with acute altered consciousness, permanent cognitive impairment, and even coma, accompanied by sepsis, without direct central nervous system infection. When managing SAE, early identification and quantification of axonal damage facilitate faster and more accurate diagnosis and prognosis. Although no specific markers for SAE have been identified, several biomarkers have been proposed. Neurofilament light chain (NFL) is a highly expressed cytoskeletal component of neurofilament (NF) proteins that can be found in blood and cerebrospinal fluid (CSF) after exposure to axonal injury. NFs can be used as diagnostic and prognostic biomarkers for sepsis-related brain injury. Phosphorylation of NFs contributes to the maturation and stabilization of cytoskeletal structures, especially axons, and facilitates axonal transport, including mitochondrial transport and energy transport. The stability of NF proteins can be assessed by monitoring the expression of NF genes. Furthermore, phosphorylation levels of NFs can be monitored to determine mitochondrial axonal transport associated with cellular energy metabolism at distal axons to assess progression during SAE treatment. This paper provides new insights into the biological characteristics, detection techniques, and scientific achievements of NFs, and discusses the underlying mechanisms and future research directions of NFs in SAE.
Graphical Abstract
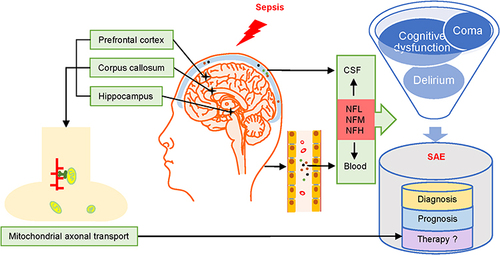
Introduction
Sepsis is life-threatening organ dysfunction caused by dysregulated host response to infection.Citation1 It is also the main cause of morbidity and mortality in critically ill patients.Citation2 The central nervous system (CNS) is one of the most vulnerable organs to systemic lesions caused by sepsis. Sepsis-associated encephalopathy (SAE) is one of the most serious clinical manifestations seen in patients with sepsis.Citation3 SAE is often accompanied by delirium and various degrees of cognitive dysfunction, including chronic neurological dysfunction.Citation4 Its surveillance, screening, and early diagnosis are crucial for improving prognosis and treatment management. However, despite monitoring methods and comprehensive treatment strategies that have been summarized after years of research on sepsis, sensitive and specific biomarkers for detection of SAE are lacking.Citation5
Neurofilament light chain (NFL) has been identified as a prognostic and diagnostic biomarker for neurological disorders, including multiple sclerosis, Alzheimer’s disease, postoperative delirium, and traumatic brain injury.Citation6–9 Research confirmed that NFL in CSF and blood has similar pathophysiological processes and identical prognostic effects on cognitive function and neurodegeneration. Progression of NFL levels can predict conversion from the pre-symptomatic to symptomatic stage and time of survival.Citation10,Citation11 In patients with SAE, elevated NFL levels indicate adverse neurological outcomes such as cognitive impairment. The close link between NFL, reduced brain volume, and neuronal damage also supports the feasibility of monitoring NFL levels to predict neurological damage in SAE patients.Citation12,Citation13 Moreover, elevated plasma NFL values were significantly correlated with SAE severity and poorer neurocognitive outcomes. However, plasma NFL is consistent with CSF-NFL; elevated levels in both plasma and CSF represent poorer clinical outcomes.Citation14
In this paper, we reviewed the biological structure, type of measurements, and research background of neurofilaments (NFs). We also discussed the mechanisms of NFs, phosphorylation changes, and their association with mitochondria during SAE. In addition, the potential implications of NFs in the surveillance of SAE are discussed.
Biological Structure and Measurements of NFs
NFs, a basic component of the cytoskeleton of neurons, provide the structural basis for axons and regulate axonal diameter to affect neuronal conduction velocity and the transportation of essential organelles.Citation15–17 NFs belong to the intermediate filaments (IFs) family, with diameters ranging between actin (6 nm) and myosin (15 nm).Citation18 They are major neuronal supporting proteins comprising five subunits (neurofilament triplet proteins): neurofilament light chain (NFL, 68 kDa), neurofilament medium chain (NFM, 160 kDa), neurofilament heavy chain (NFH, 200 kDa), α-internexin (66 kDa), and peripherin (57 kDa).Citation19 The NF protein is rigorously assembled from dimer to tetramer, then an octamer, forming a mature long NF polymer of 10 nm diameter ().Citation20 All NF subunits contain a conserved A-helical rod domain connected by several helical coils, a variable amino-terminal head region, and a carboxy-terminal tail region. Different types of NFs have different number of repeats containing Lysr-Ser-Pro sequences at the carboxy terminal. Epigenetic modifications of NF genes include methylation, acetylation, and phosphorylation.Citation21–23 Phosphorylation of NF subunits is the most important factor in stabilizing the axonal cytoskeleton.
Figure 1 Neurofilament assembly in CNS. The mature long NF polymer has a diameter of 10 nm. NFL (yellow) is the most abundant quantifiable subunit. Tail domains of NFM (green) and NFH (blue) protrude outward from the neurofilament core for phosphorylation of the carboxy-terminal tail region. In addition to the neurofilament triplet proteins (NFL, NFM, and NFH), α-internexin (purple) is also present in the CNS. A magnified image of myelinated axon is shown on the left.

NFL, as the most abundant quantifiable subunit in NFs, can be measured in blood and cerebrospinal fluid (CSF).Citation24 Kuhle et al suggested using blood NFL levels for real-time monitoring of disease activity and response to drugs.Citation25 Previous studies have analyzed three approaches to reliably measure NFL levels in blood samples. The fourth-generation single-molecule array (Simoa) assay for NFL was found to be 126-fold more sensitive than the conventional ELISA and 25 times more sensitive than electrochemiluminescence (ECL).Citation26 Continuous measurement of blood NFL is clinically feasible, with minimal collateral damage to the patient. It also provides more possibilities for the early diagnosis and prognostic analysis of neurological diseases. However, Shahim et al observed the dynamic release of NFs in serum and CSF, which increased over the first 12 days post-injury.Citation27 The mean NFs in patients with SAE showed a time-dependent increase from day 1 to day 7.Citation14 Therefore, these studies suggested that the measurement of NFs should be initiated from the first day after injury and may be influenced by time. Timely repeat measurements are of great significance for assessing NF levels.
NF Response to Nerve System Injury
Clinical Research
Several studies provided evidence that disorganization of the neuronal IF network may be directly involved in neuronal dysfunction. Depending on the extent of neuronal dysfunction, NFs can easily diffuse into the extracellular fluid and subsequently into CSF and peripheral blood via the blood–brain barrier (BBB).Citation25
Recently, the application of NFs in SAE has attracted extensive interest. The elevated NFL value and soluble triggering receptor expressed on myeloid cells 2 (sTREM2) in CSF predicted early brain damage caused by sepsis and neuroinflammation before the onset of SAE symptoms. Moreover, a positive correlation was found between CSF NFL and sTREM2 levels (p = 0.005, R = 0.706).Citation28 Thus, NFL and sTREM2 levels have been suggested as diagnostic biomarkers for early brain damage and prognostic biomarkers for long-term cognitive dysfunction in SAE patients. Besides NFL, human septic brains postmortem demonstrated that the deterioration of brain homeostasis could contribute to diffuse and/or ischemic axonal damage during SAE. The immunohistochemical staining for non-phosphorylated NFH SMI32 revealed numerous axonal endbulbs accompanied by bead-like swelling, indicating axonal degeneration.Citation29 Moreover, Ehler et al found elevated levels of NFL and NFH in plasma and CSF of SAE patients. The plasma NFL values were significantly related to the severity of SAE (R = 0.534, p = 0.022) and a poorer functional outcome after 100 days (R = −0.535, p = 0.0003). Also, CSF NFL levels were significantly correlated with the last lifetime (R = −0.932, p < 0.0001) and functional outcome after 100 days (R = −0.749, p < 0.0001) in contrast to CSF NFH levels ().Citation14 This prospective, longitudinal study suggested for the first time that NF levels in plasma and CSF provide prognostic information on the severity of SAE and long-term cognitive outcome in survivors.
Table 1 Research of NF Response to SAE in Clinical and Preclinical Findings
NFL is a novel and less invasive biomarker for clinical assessment of patients with neurodegenerative diseases.Citation30,Citation31 However, NFL levels are affected by factors such as age, renal function, surgery, and anesthesia.Citation32–36 In addition, recent evidence reported a correlation between NFL and SARS-COV-2 infection. However, whether NFL can be used as a definitive biomarker for COVID-19 patient needs to be further explored.Citation37 Therefore, severe sepsis patients with the above-mentioned high-risk factors may be more likely to develop delirium and SAE.
NF is a neuronal cytoskeletal protein that increases in certain PNS lesions, such as amyotrophic lateral sclerosis (ALS), Parkinson’s disease (PD), Charcot–Marie–Tooth (CMT) disease, giant axonal neuropathy, and COVID-related critical neuropathy/myopathy.Citation20,Citation38 However, NFL can reflect the intensity of neurodegeneration in PNS and neurodegeneration-related cognitive decline in CNS.Citation39 The abnormal accumulation of NFs is consistent with indicators such as GFAP and Tau proteins.Citation40,Citation41 Thus, we concluded that the measurement of NFs in CNS and PNS diseases is equally reliable. Therefore, monitoring NFs to reflect neuronal damage may be useful for individual clinical treatment decisions and prognostic assessment.
Preclinical Research
The use of lipopolysaccharide (LPS) and cecal ligation and puncture (CLP) are the two most common methods used to simulate sepsis models. CLP-induced SAE model is characterized by septic brain injury with cognitive impairment.Citation42 However, no studies have investigated the role of NFs in the CLP-induced SAE model.
Due to the altered regulation of NFL gene expression caused by experimental LPS administration, resulting in structural abnormalities in neurons and synapses, the application of LPS in models involving the study of NFs may have certain advantages.Citation43 In the LPS-induced sepsis model, the expression of NFL, NFM, and NFH proteins was significantly decreased in the corpus callosum, while the axonal injury involved decreased mean axonal diameter, decreased axonal myelination levels, and even no myelin sheaths. Additionally, in primary cultured cortical neuron models with IL-1β treatment, IL-1β activates the phosphorylation of the P38-MAPK signaling pathway to inhibit the protein expressions of NFL, NFM, NFH, and synaptophysin.Citation44 In vivo, IL-1β produced by activated microglia enters the brain through BBB and induces the decrease of NF expression and synaptic damage through the inflammatory pathway. Similarly, the significantly reduced expressions of NFL, NFM, and NFH in neonatal rats induced by LPS injection resulted in reduced number of myelinated axons and impaired nodes of Ranvier, which were associated with axonal development disorders manifested as movement disorders in later life.Citation45 Furthermore, in an LPS-induced neonatal rat model of sepsis, significant down-regulation of NFL, NFM, and NFH expressions in the prefrontal cortex and hippocampus was associated with axonal dysfunction, which may be related to motor dysfunction and cognitive dysfunction.Citation46 In addition to the LPS modeling, there are two types of ischemic and diffuse axonal damage in the septic rat model induced by intraperitoneal injection of fecal slurry. The mild correlation between NFHSMI35 levels and the time to sepsis induction accompanied by abnormal staining of β-amyloid precursor protein (βAPP) and severe disruption of the β-tubulin network was reported ().Citation29 The above preclinical studies provided evidence at the protein level for NFs as a non-specific biomarker for supplementary monitoring to predict the occurrence of axonal damage and related neurocognitive dysfunction during sepsis. However, the gene transcription or mRNA stability is responsible for normal levels of NF proteins.Citation47–49 Current samples are insufficient to provide direct evidence of reduced NF gene transcription or mRNA stability in SAE patients. Therefore, more information is needed from subsequent experiments in different sepsis models in order to translate discoveries into human setting.
Pathological Mechanisms Associated with NFs and SAE
Current hypotheses suggest that neuroinflammation, activation of microglia cells, destruction of BBB, mitochondrial dysfunction, dysregulation of oxygen and energy metabolism, and neuroaxonal damage can lead to cerebral dysfunction in sepsis.Citation29,Citation50–55 Besides the pro-inflammatory cytokines that are produced during sepsis can inhibit the expression of NF subunits while penetrating the BBB and activating microglia.Citation44,Citation56 NF-related axonal transport mechanism contributes to mitochondrial translocation and energy transport that are worth exploring in SAE.
The oxidative stress in sepsis affects the intra-mitochondrial biogenesis, which finally causes reduced energy production.Citation54 Since synapses consume most of the energy in the brain and synaptic energy is mainly provided by mitochondria,Citation57 transporting mitochondria to distal neuronal axons may be an important measure to improve the synaptic energy supply and even reverse the SAE.
The transport of mitochondria in CNS involves neuronal cytoskeletal components such as microtubules, actin and NFs, the driving force system, and the mitochondrial membrane potential. Here, we focused on how NF injury affects mitochondrial axonal transport in SAE. First, the driving force for NFs to participate in axonal transport comes from the combination of dynein/dynactin, kinesin, myosin and NFs, based on the microtubules.Citation58–61 In addition, mitochondrial association with IFs alters mitochondrial membrane potential, energy production and transport.Citation62 The phosphorylated NFLs are more likely to bind stationary mitochondria with higher membrane potentials.Citation63 Furthermore, the phosphorylation of NFH and NFM protrude their side arms from the trunk to increase the negative charge and then form the regular cross-bridge between mitochondria and NFs, promoting the translocation of mitochondria to distal axons and facilitating the acquisition of energy in neurons, which is required for maintaining neuronal resting potential, synaptic transmission and ATP for the action potentialCitation63,Citation64 (). Therefore, efficient binding of NFs to mitochondria mediated by phosphorylated NFs is essential for normal mitochondrial translocation in axons. In sepsis, the disruption of NFs leads to reduced axonal diameter, atrophy of NFs and decreased conduction velocity, which contributes to axonal transport disorder. Therefore, monitoring NFs, even the phosphorylation status, may effectively assess the treatment of SAE, which is helpful for recovery.
Figure 2 Mitochondrial axonal transport associated with NFs. The blue arrow indicates the anterograde axonal transport of mitochondria towards the distal axon. The driving force comes from the combination of dynein/dynactin, kinesin, myosin and phosphorylated NFs based on the microtubules. The elements that participate in the rapid axonal transport include mitochondria, neurotransmitter vesicles, and secretory particles. In addition, the direct combination of NFL and myosin Va plays a critical role in slow axonal transport for local mitochondrial movement.

NF is a biomarker that contributes to the diagnosis of SAE and the prediction of disease severity. Maintaining mRNA stability by targeting miRNA is conducive to normal protein expression and reduced abnormal aggregation of NFs.Citation65 Meanwhile, reversal of translational dysregulation of NF mRNAs in the ALS/frontotemporal dementia (FTD) mouse model contributes to improving cognitive impairment associated with restored axonal integrity and synaptic function.Citation66 Moreover, assessment of mitochondrial function related to NFs may be useful for assessing brain energy metabolism.Citation67 These studies support the importance of repair of neuroaxonal function associated with mitochondrial transport mediated by NFs and provide a new idea for NFs as potential therapeutic targets for SAE.
Conclusions
NFs can be used as diagnostic and prognostic biomarkers for SAE to assess cognitive function. Monitoring mRNA expression levels of NFs is useful for assessing neuronal cytoskeleton stability. Monitoring NF phosphorylation levels may be used to assess mitochondrial axonal transport, which has important implications for cellular energy metabolism at distal axons. The next step is to use NFs as biomarkers in practical studies and combine with pathophysiological changes under different etiologies, to explore whether NF monitoring can play a more active role in the clinical management of SAE.
Disclosure
The authors report no conflicts of interest in this work.
Additional information
Funding
References
- Singer M, Deutschman CS, Seymour CW, et al. The third international consensus definitions for sepsis and septic shock (Sepsis-3). JAMA. 2016;315(8):801–810. doi:10.1001/jama.2016.0287
- Feng Q, Ai YH, Gong H, et al. Characterization of sepsis and sepsis-associated encephalopathy. J Intensive Care Med. 2019;34(11–12):938–945. doi:10.1177/0885066617719750
- Zhang LN, Wang XT, Ai YH, et al. Epidemiological features and risk factors of sepsis-associated encephalopathy in intensive care unit patients: 2008–2011. Chin Med J. 2012;125(5):828–831.
- Mazeraud A, Righy C, Bouchereau E, et al. Septic-associated encephalopathy: a comprehensive review. Neurotherapeutics. 2020;17(2):392–403. doi:10.1007/s13311-020-00862-1
- Rhodes A, Evans LE, Alhazzani W, et al. Surviving sepsis campaign: international guidelines for management of sepsis and septic shock: 2016. Intensive Care Med. 2017;43(3):304–377. doi:10.1007/s00134-017-4683-6
- Shahim P, Zetterberg H, Tegner Y, Blennow K. Serum neurofilament light as a biomarker for mild traumatic brain injury in contact sports. Neurology. 2017;88(19):1788–1794. doi:10.1212/WNL.0000000000003912
- Barro C, Benkert P, Disanto G, et al. Serum neurofilament as a predictor of disease worsening and brain and spinal cord atrophy in multiple sclerosis. Brain. 2018;141(8):2382–2391. doi:10.1093/brain/awy154
- Alirezaei Z, Pourhanifeh MH, Borran S, et al. Neurofilament light chain as a biomarker, and correlation with magnetic resonance imaging in diagnosis of CNS-related disorders. Mol Neurobiol. 2020;57(1):469–491. doi:10.1007/s12035-019-01698-3
- Casey CP, Lindroth H, Mohanty R, et al. Postoperative delirium is associated with increased plasma neurofilament light. Brain. 2020;143(1):47–54. doi:10.1093/brain/awz354
- Mielke MM, Syrjanen JA, Blennow K, et al. Plasma and CSF neurofilament light: relation to longitudinal neuroimaging and cognitive measures. Neurology. 2019;93(3):e252–e260. doi:10.1212/WNL.0000000000007767
- Bacioglu M, Maia LF, Preische O, et al. Neurofilament light chain in blood and CSF as marker of disease progression in mouse models and in neurodegenerative diseases. Neuron. 2016;91(1):56–66. doi:10.1016/j.neuron.2016.05.018
- Orhun G, Tuzun E, Bilgic B, et al. Brain volume changes in patients with acute brain dysfunction due to sepsis. Neurocrit Care. 2020;32(2):459–468. doi:10.1007/s12028-019-00759-8
- Khalil M, Pirpamer L, Hofer E, et al. Serum neurofilament light levels in normal aging and their association with morphologic brain changes. Nat Commun. 2020;11(1):812. doi:10.1038/s41467-020-14612-6
- Ehler J, Petzold A, Wittstock M, et al. The prognostic value of neurofilament levels in patients with sepsis-associated encephalopathy - a prospective, pilot observational study. PLoS One. 2019;14(1):e0211184. doi:10.1371/journal.pone.0211184
- Barry DM, Stevenson W, Bober BG, et al. Expansion of neurofilament medium C terminus increases axonal diameter independent of increases in conduction velocity or myelin thickness. J Neurosci. 2012;32(18):6209–6219. doi:10.1523/JNEUROSCI.0647-12.2012
- Jones MR, Villalon E, Garcia ML. Genetic manipulation of neurofilament protein phosphorylation. Methods Enzymol. 2016;568:461–476.
- Garcia ML, Lobsiger CS, Shah SB, et al. NF-M is an essential target for the myelin-directed “outside-in” signaling cascade that mediates radial axonal growth. J Cell Biol. 2003;163(5):1011–1020. doi:10.1083/jcb.200308159
- Renner W, Franke WW, Schmid E, et al. Reconstitution of intermediate-sized filaments from denatured monomeric vimentin. J Mol Biol. 1981;149(2):285–306. doi:10.1016/0022-2836(81)90303-X
- Yuan A, Hassinger L, Rao MV, et al. Dissociation of axonal neurofilament content from its transport rate. PLoS One. 2015;10(7):e0133848. doi:10.1371/journal.pone.0133848
- Yuan A, Rao MV, Nixon V. Neurofilaments and neurofilament proteins in health and disease. Cold Spring Harb Perspect Biol. 2017;9(4):a018309. doi:10.1101/cshperspect.a018309
- Lei R, Lee JP, Francis MB, Kumar S. Structural regulation of a neurofilament-inspired intrinsically disordered protein brush by multisite phosphorylation. Biochemistry. 2018;57(27):4019–4028. doi:10.1021/acs.biochem.8b00007
- Abu Hamdeh S, Ciuculete DM, Sarkisyan D, et al. Differential DNA methylation of the genes for amyloid precursor protein, tau, and neurofilaments in human traumatic brain injury. J Neurotrauma. 2021;38(12):1679–1688. doi:10.1089/neu.2020.7283
- Shen M, Chen Z, Ming M, et al. The acetylome of adult mouse sciatic nerve. J Neurochem. 2022;162(3):262–275. doi:10.1111/jnc.15648
- Giovannoni G. Peripheral blood neurofilament light chain levels: the neurologist’s C-reactive protein? Brain. 2018;141(8):2235–2237. doi:10.1093/brain/awy200
- Kuhle J, Kropshofer H, Haering DA, et al. Blood neurofilament light chain as a biomarker of MS disease activity and treatment response. Neurology. 2019;92(10):e1007–e1015. doi:10.1212/WNL.0000000000007032
- Kuhle J, Barro C, Andreasson U, et al. Comparison of three analytical platforms for quantification of the neurofilament light chain in blood samples: ELISA, electrochemiluminescence immunoassay and simoa. Clin Chem Lab Med. 2016;54(10):1655–1661. doi:10.1515/cclm-2015-1195
- Shahim P, Gren M, Liman V, et al. Serum neurofilament light protein predicts clinical outcome in traumatic brain injury. Sci Rep. 2016;6(1):36791. doi:10.1038/srep36791
- Orhun G, Esen F, Yilmaz V, et al. Elevated sTREM2 and NFL levels in patients with sepsis associated encephalopathy. Int J Neurosci. 2021:1–7. doi:10.1080/00207454.2021.1916489
- Ehler J, Barrett LK, Taylor V, et al. Translational evidence for two distinct patterns of neuroaxonal injury in sepsis: a longitudinal, prospective translational study. Crit Care. 2017;21(1):262. doi:10.1186/s13054-017-1850-7
- Shin HR, Moon J, Lee WJ, et al. Serum neurofilament light chain as a severity marker for spinocerebellar ataxia. Sci Rep. 2021;11(1):13517. doi:10.1038/s41598-021-92855-z
- Aamodt WW, Waligorska T, Shen J, et al. Neurofilament light chain as a biomarker for cognitive decline in Parkinson disease. Mov Disord. 2021;36(12):2945–2950. doi:10.1002/mds.28779
- Schulte PJ, Roberts RO, Knopman DS, et al. Association between exposure to anaesthesia and surgery and long-term cognitive trajectories in older adults: report from the mayo clinic study of aging. Br J Anaesth. 2018;121(2):398–405. doi:10.1016/j.bja.2018.05.060
- Brown CH, Lewis A, Probert J, et al. Perioperative neurofilament light plasma concentrations and cognition before and after cardiac surgery: a prospective nested cohort study. Anesthesiology. 2022;137(3):303–314. doi:10.1097/ALN.0000000000004327
- Bornhorst JA, Figdore D, Campbell MR, et al. Plasma neurofilament light chain (NfL) reference interval determination in an age-stratified cognitively unimpaired cohort. Clin Chim Acta. 2022;535:153–156. doi:10.1016/j.cca.2022.08.017
- Akamine S, Marutani N, Kanayama D, et al. Renal function is associated with blood neurofilament light chain level in older adults. Sci Rep. 2020;10(1):20350. doi:10.1038/s41598-020-76990-7
- Guasp M, Munoz-Sanchez G, Martinez-Hernandez E, et al. CSF biomarkers in COVID-19 associated encephalopathy and encephalitis predict long-term outcome. Front Immunol. 2022;13:866153. doi:10.3389/fimmu.2022.866153
- Chung HY, Neu C, Wickel J, Kuckertz SL, Coldewey SM. Neurofilament light chain in patients with COVID-19 and bacterial pneumonia. Ann Neurol. 2021;90(1):174–175. doi:10.1002/ana.26135
- Frithiof R, Rostami E, Kumlien E, et al. Critical illness polyneuropathy, myopathy and neuronal biomarkers in COVID-19 patients: a prospective study. Clin Neurophysiol. 2021;132(7):1733–1740. doi:10.1016/j.clinph.2021.03.016
- Olsson B, Portelius E, Cullen NC, et al. Association of cerebrospinal fluid neurofilament light protein levels with cognition in patients with dementia, motor neuron disease, and movement disorders. JAMA Neurol. 2019;76(3):318–325. doi:10.1001/jamaneurol.2018.3746
- Aamodt AH, Hogestol EA, Popperud TH, et al. Blood neurofilament light concentration at admittance: a potential prognostic marker in COVID-19. J Neurol. 2021;268(10):3574–3583. doi:10.1007/s00415-021-10517-6
- Kanberg N, Ashton NJ, Andersson LM, et al. Neurochemical evidence of astrocytic and neuronal injury commonly found in COVID-19. Neurology. 2020;95(12):e1754–e1759. doi:10.1212/WNL.0000000000010111
- Huerta PT, Robbiati S, Huerta TS, et al. Preclinical models of overwhelming sepsis implicate the neural system that encodes contextual fear memory. Mol Med. 2016;22(1):789–799. doi:10.2119/molmed.2015.00201
- Lukiw WJ, Cong L, Jaber V, Zhao Y. Microbiome-derived Lipopolysaccharide (LPS) selectively inhibits Neurofilament Light chain (NF-L) gene expression in Human Neuronal-Glial (HNG) cells in primary culture. Front Neurosci. 2018;12:896. doi:10.3389/fnins.2018.00896
- Han Q, Lin Q, Huang P, et al. Microglia-derived IL-1beta contributes to axon development disorders and synaptic deficit through p38-MAPK signal pathway in septic neonatal rats. J Neuroinflammation. 2017;14(1):52. doi:10.1186/s12974-017-0805-x
- Deng Y, Han Q, Shen F, Chen M, Zeng H. Effect of axonal developmental disorders in the corpus callosum on the neurological function after birth in septic neonatal rats. Zhonghua Wei Zhong Bing Ji Jiu Yi Xue. 2016;28(8):683–687. Chinese. doi:10.3760/cma.j.issn.2095-4352.2016.08.003
- Huang P, Chen X, Hu X, et al. Experimentally induced sepsis causes extensive hypomyelination in the prefrontal cortex and hippocampus in neonatal rats. Neuromolecular Med. 2020;22(3):420–436. doi:10.1007/s12017-020-08602-6
- Campos-Melo D, Hawley ZCE, Strong MJ. Dysregulation of human NEFM and NEFH mRNA stability by ALS-linked miRNAs. Mol Brain. 2018;11(1):43. doi:10.1186/s13041-018-0386-3
- Mages B, Aleithe S, Altmann S, et al. Impaired neurofilament integrity and neuronal morphology in different models of focal cerebral ischemia and human stroke tissue. Front Cell Neurosci. 2018;12:161. doi:10.3389/fncel.2018.00161
- Pogue AI, Jaber VR, Sharfman NM, Zhao Y, Lukiw WJ. Downregulation of neurofilament light chain expression in human neuronal-glial cell co-cultures by a microbiome-derived lipopolysaccharide-induced miRNA-30b-5p. Front Neurol. 2022;13:900048. doi:10.3389/fneur.2022.900048
- Moraes CA, Santos G, de Sampaio e Spohr TC, et al. Activated microglia-induced deficits in excitatory synapses through IL-1beta: implications for cognitive impairment in sepsis. Mol Neurobiol. 2015;52(1):653–663. doi:10.1007/s12035-014-8868-5
- Mei B, Li J, Zuo Z. Dexmedetomidine attenuates sepsis-associated inflammation and encephalopathy via central alpha2A adrenoceptor. Brain Behav Immun. 2021;91:296–314. doi:10.1016/j.bbi.2020.10.008
- Li Y, Yin L, Fan Z, et al. Microglia: a potential therapeutic target for sepsis-associated encephalopathy and sepsis-associated chronic pain. Front Pharmacol. 2020;11:600421. doi:10.3389/fphar.2020.600421
- Xu XE, Liu L, Wang YC, et al. Caspase-1 inhibitor exerts brain-protective effects against sepsis-associated encephalopathy and cognitive impairments in a mouse model of sepsis. Brain Behav Immun. 2019;80:859–870. doi:10.1016/j.bbi.2019.05.038
- Sun J, Zhang J, Tian J, et al. Mitochondria in sepsis-induced AKI. J Am Soc Nephrol. 2019;30(7):1151–1161. doi:10.1681/ASN.2018111126
- Vandewalle J, Libert C. Sepsis: a failing starvation response. Trends Endocrinol Metab. 2022;33(4):292–304. doi:10.1016/j.tem.2022.01.006
- Basu S, Agarwal P, Anupurba S, Shukla R, Kumar A. Elevated plasma and cerebrospinal fluid interleukin-1 beta and tumor necrosis factor-alpha concentration and combined outcome of death or abnormal neuroimaging in preterm neonates with early-onset clinical sepsis. J Perinatol. 2015;35(10):855–861. doi:10.1038/jp.2015.86
- Harris JJ, Jolivet R, Attwell D. Synaptic energy use and supply. Neuron. 2012;75(5):762–777. doi:10.1016/j.neuron.2012.08.019
- Wagner OI, Ascano J, Tokito M, et al. The interaction of neurofilaments with the microtubule motor cytoplasmic dynein. Mol Biol Cell. 2004;15(11):5092–5100. doi:10.1091/mbc.e04-05-0401
- Kimura N, Okabayashi S, Ono F. Dynein dysfunction disrupts intracellular vesicle trafficking bidirectionally and perturbs synaptic vesicle docking via endocytic disturbances a potential mechanism underlying age-dependent impairment of cognitive function. Am J Pathol. 2012;180(2):550–561. doi:10.1016/j.ajpath.2011.10.037
- Rohn JL, Patel JV, Neumann B, et al. Myo19 ensures symmetric partitioning of mitochondria and coupling of mitochondrial segregation to cell division. Curr Biol. 2014;24(21):2598–2605. doi:10.1016/j.cub.2014.09.045
- Rao MV, Mohan PS, Kumar A, et al. The myosin Va head domain binds to the neurofilament-L rod and modulates endoplasmic reticulum (ER) content and distribution within axons. PLoS One. 2011;6(2):e17087. doi:10.1371/journal.pone.0017087
- Shah M, Chacko LA, Joseph JP, Ananthanarayanan V. Mitochondrial dynamics, positioning and function mediated by cytoskeletal interactions. Cell Mol Life Sci. 2021;78(8):3969–3986. doi:10.1007/s00018-021-03762-5
- Wagner OI, Lifshitz J, Janmey PA, et al. Mechanisms of mitochondria-neurofilament interactions. J Neurosci. 2003;23(27):9046–9058. doi:10.1523/JNEUROSCI.23-27-09046.2003
- Perrot R, Julien JP. Real-time imaging reveals defects of fast axonal transport induced by disorganization of intermediate filaments. FASEB J. 2009;23(9):3213–3225. doi:10.1096/fj.09-129585
- Campos-Melo D, Droppelmann CA, He Z, Volkening K, Strong MJ. Altered microRNA expression profile in amyotrophic lateral sclerosis: a role in the regulation of NFL mRNA levels. Mol Brain. 2013;6:26. doi:10.1186/1756-6606-6-26
- Kumar S, Phaneuf D, Cordeau P Jr, et al. Induction of autophagy mitigates TDP-43 pathology and translational repression of neurofilament mRNAs in mouse models of ALS/FTD. Mol Neurodegener. 2021;16(1):1. doi:10.1186/s13024-020-00420-5
- Obrador E, Salvador-Palmer R, Lopez-Blanch R, et al. The link between oxidative stress, redox status, bioenergetics and mitochondria in the pathophysiology of ALS. Int J Mol Sci. 2021;22(12):6352. doi:10.3390/ijms22126352