Abstract
Introduction
Neutrophil predominant airway inflammation is associated with severe and steroid-resistant asthma clusters. Previously, we reported efficacy of ASHMI, a three-herb TCM asthma formula in a steroid-resistant neutrophil-dominant murine asthma model and further identified Ganoderic Acid C1 (GAC1) as a key ASHMI active compound in vitro. The objective of this study is to investigate GAC1 effect on neutrophil-dominant, steroid-resistant asthma in a murine model.
Methods
In this study, Balb/c mice were systematically sensitized with ragweed (RW) and alum and intranasally challenged with ragweed. Unsensitized/PBS challenged mice served as normal controls. Post sensitization, mice were given 4 weeks of oral treatment with GAC1 or acute dexamethasone (Dex) treatment at 48 hours prior to challenge. Pulmonary cytokines were measured by ELISA, and lung sections were processed for histology by H&E staining. Furthermore, GAC1 effect on MUC5AC expression and on reactive oxygen species (ROS) production in human lung epithelial cell line (NCI-H292) was determined by qRT-PCR and ROS assay kit, respectively. Computational analysis was applied to select potential targets of GAC1 in steroid-resistant neutrophil-dominant asthma. Molecular docking was performed to predict binding modes between GAC1 and Dex with TNF-α.
Results
The result of the study showed that chronic GAC1 treatment, significantly reduced pulmonary inflammation (P < 0.01–0.001 vs Sham) and airway neutrophilia (P < 0.01 vs Sham), inhibited TNF-α, IL-4 and IL-5 levels (P < 0.05–0.001 vs Sham). Acute Dex treatment reduced eosinophilic inflammation and IL-4, IL-5 levels, but had no effect on neutrophilia and TNF-α production. GAC1 treated H292 cells showed decreased MUC5AC gene expression and production of ROS (P < 0.001 vs stimulated/untreated cells). Molecular docking results showed binding energy of complex GAC1-TNF was −10.8 kcal/mol.
Discussion
GAC1 may be a promising anti-asthma botanical drug for treatment of steroid-resistant asthma.
Introduction
Patients with severe asthma account for approximately 10% of the total asthma population but represent a high proportion of asthma morbidity and health care costs.Citation1 Presence of neutrophils in airway inflammation is associated with the most severe asthma clusters as defined by recent the Severe Asthma Research Program (SARP) findings.Citation2–4 Neutrophil-dominant asthma, which occurs in 15–25% of the asthma cases, represents a severe clinical problem. It is steroid-resistantCitation5–8 and is not addressed by recent novel biologic therapies, which mostly target eosinophilic (T2 high) asthma.Citation9,Citation10 It has been reported that steroid-resistant neutrophil dominant asthma may be associated with tumor necrosis factor α (TNF-α), which is a pleiotropic cytokine that can promote mucus secretion, airway inflammation and enhance airway smooth muscle cells (ASMC) contractility.Citation11–13 TNF-α elevation and mutations in tumor necrosis factor receptor (TNF-R) are associated with asthma exacerbations and severe asthma.Citation14,Citation15 It has been reported that TNF-α is involved in the recruitment of neutrophils and eosinophils in the inflammatory response during asthma.Citation16 TNF-α increased endogenous TNF-α, interleukin-6 (IL-6), interleukin-1β (IL-1β), interleukin-8 (IL-8), thymic stromal lymphopoietin, and pro-matrix metalloprotease 9 release in severe asthmatic airways.Citation17 TNF-α reduces the responsiveness to steroid treatment and TNFα blockade restores the therapeutic effects of glucocorticoids (GCs) in the steroid-resistant model.Citation18 Clinical trials of anti-TNF biologics in carefully selected patients are efficacious. However, serious adverse effects include increased susceptibility to infections, cancer and suicide.Citation9,Citation19 Most anti-TNF biologics are injectable and not indicated for use in children. Thus, safer and more patient-friendly alternatives to treatment of T2-low asthma are desirable.
In previous studies, we showed that ASHMI, a three-herb TCM asthma formula comprised Ganoderma lucidum, Glycyrrhiza uralensis and Sophora flavesence, suppressed IgE, Th2 cytokines and eosinophilic inflammation (characteristics of T2 high asthma) in a clinical trialCitation20 and in an eosinophilic ovalbumin (OVA)-asthma murine model.Citation21 Subsequently, we showed that ASHMI was also effective in neutrophil-dominant asthma using a murine ragweed asthma model characterized by neutrophil dominant mixed granulocytic inflammation with involvement of TNF-α and IL-8 (mimicking human T2 low asthma).Citation22 Acute steroid treatments in this model failed to reduce neutrophilic airway inflammation or airway hyperresponsiveness (AHR).Citation22,Citation23 Various steroid-resistant murine asthma models insensitive to acute Dex treatment have been developed. Chronic aerosol OVA exposure of OVA/alum sensitized BALB/c mice displayed steroid-resistant neutrophilic inflammation. Acute Dex treatment did not suppress neutrophil accumulation and development of airway hyperresponsiveness.Citation23 OVA/ complete Freund’s adjuvant (CFA)-based sensitization followed by allergen challenge elicited a neutrophilic pulmonary inflammation in C57BL/6 mice, which was poorly controlled by acute DEX treatment.Citation24 Chlamydia infection induced steroid-insensitive neutrophilic allergic airway disease using BALB/c mice. Both inflammation and AHR were insensitive to acute Dex treatment.Citation25 These models provided useful tools to study steroid-resistant asthma in association with neutrophilic airway inflammation.
Ganoderic acid C1 (GAC1), a lanostane triterpenoid isolated from ASHMI constituent Ganoderma lucidum, shows marked anti-inflammatory activities by inhibiting the generation of reactive oxygen species (ROS) and the expression of p21 and p16 proteins.Citation22 We reported GAC1 as a potent suppressor of TNF-α production in lipopolysaccharide (LPS)-stimulated macrophages in vitro, human peripheral blood mononuclear cells (PBMCs) from asthma patients and biopsy samples from inflammatory bowel disease subjects.Citation26,Citation27 These findings prompted us to hypothesize that GAC1 may be an effective therapy for severe asthma endotypes featuring a prominent role for TNF-α, such as neutrophil-dominant and steroid-resistant asthma. Considering the complexity of pathological mechanisms affected by GAC1 on neutrophil-dominant asthma, computational analysis – system pharmacology and in silico molecular docking – was applied to estimate the potential targets of GAC1 on asthma and glucocorticoid resistance.
In the current study, using a ragweed-induced T2 low asthma model published previous,Citation22 we showed that oral chronic GAC1 therapy significantly reduced the percentage of neutrophils and eosinophils in airways and alleviated asthma pathology associated with suppression of TNF-A, in addition to suppressing Th2 cytokines and increasing interferon-gamma (IFN-γ). In contrast, acute Dex treatment only reduced eosinophils but showed no reduction in neutrophils, tissue pathology or TNF-α. Downstream regulation of TNF-α might be key contributors in treating steroid-resistant neutrophil-predominant asthma. This work further implies that levels of TNF-α are closely associated with steroid-resistant neutrophil predominant airway inflammation. GAC1 is a potentially promising therapy for steroid-resistant neutrophil predominant asthma by targeting TNF-α.
Materials and Methods
GAC1 Isolation and HPLC
ASHMI and G. lucidum (GL) aqueous extracts were manufactured by the Sino-Lion Pharmaceutical Company (a GMP certified facility in Weifang, China) as previously described.Citation28 GAC1 (chemical structure shown in ) was isolated from GL aqueous extracts as previously described.Citation26 High performance liquid chromatography (HPLC) chromatogram of GAC1, ASHMI and GL are shown in Supplementary E–Figure 1.
Figure 1 Chemical Structure of GAC1 and Experimental Design. (A) Chemical structure of Ganoderic acid C1. (B) Experimental design. Mice sensitized with RW (and alum) intraperitoneally on days 0 and day 7 received intranasal RW on day 14. GAC1 (20 mg/kg) were given in 0.5 mL of water twice daily by intragastric administration from D15 to D42. Dex (1 mg/kg, dissolved in 0.5 mL water were given at 24 hours and 2 hours prior to the second RW challenge. Mice in all groups except Naïve group were intranasally challenged with RW on days 43 and 44. Naïve mice were challenged with PBS. Terminal analysis was performed on day 46. D= Day, i.p.= intraperitoneal, i.n.= intranasal.
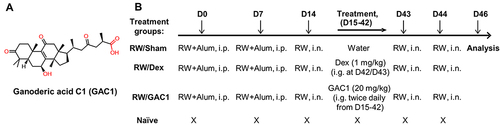
Animal Studies
Female BALB/c mice were purchased from Jackson Laboratory (Bar Harbor, ME) and maintained in pathogen-free facilities at the Mount Sinai vivarium in accordance with standard guidelines for the care and use of animals.Citation29 All animal procedures were approved by the Institutional Animal Care and Use Committee. This project has been approved by the Institutional Animal Care and Use Committee, IACUC, at Icahn School of Medicine at Mount Sinai, New York (IACUC#-LA11-00082).
Antigen Sensitization, Challenge, and Treatment Protocols
RW sensitization, challenge and treatment protocols were similar to those described previously.Citation22 The endotoxin level of RW extract (low-endotoxin source material, Greer Laboratories, Lenoir, North Carolina) was measured as previously described and verified to be 9 pg/mL.Citation22 As shown in , mice were sensitized by intraperitoneal (i.p.) injections of RW (100 µg) in 0.4 mL PBS containing 2 mg alum as adjuvant (Thermo Scientific, Waltham, MA) on days 0 and 7 according to the protocol in . Mice then received an intranasal (i.n.) RW challenge (100 µg in 50 µL PBS) on day 14 (RW mice). One day later, RW-sensitized mice received 400 μg GAC1 (20 mg/kg, RW/GAC1 mice) in 0.5 mL of water or water alone as the sham treatment (RW/sham mice) twice daily by intragastric administration (i.g.) from day 15 to day 42. Another group of RW-sensitized mice was given oral DexCitation22,Citation23 (1 mg/kg, D4902, Sigma-Aldrich, St. Louis, MO), which was dissolved in 0.5 mL water at 24 hours and 2 hours prior to the second RW challenge. After completing treatment, a second set of intranasal challenges consisting of 2 consecutive daily doses (days 43 and 44) with RW (150 µg) in 50 µL PBS was administered. Naïve mice without treatment (naïve group) served as negative controls.
Evaluation of BALF Cells, Cytokines
BALF was collected by lavaging airways as previously described.Citation22 BALF was centrifuged at 1200 rpm for 15 minutes at 4 °C to isolate cells. After counting, approximately 4 × 104 cells were cytospun onto glass slides for differential analysis by HEMA-3 staining. Eosinophils, neutrophils and macrophage numbers per 500 cells were counted using standard criteria.Citation22 Cytokine levels in BALF supernatants were assayed by commercial ELISA kits according to the manufacturer’s instructions (BD Biosciences, San Diego, CA) for TNF-α, IL-4, IL-5 and IFN-γ.
Scoring of Pulmonary Inflammation
Formalin fixed paraffin-embedded lung sections were stained with hematoxylin and eosin stain. Inflammation scores were assigned using criteria described previously.Citation22 Briefly, peribronchial and perivascular inflammations were evaluated on a scale from 0 to 4 as follows. 0 – No inflammation was detectable around the airway or blood vessel; 1-Occasional presence of inflammatory cells around airway or blood vessel; 2 – Most airways/blood vessels were surrounded by a ring of inflammatory cells that were one cell layer deep; 3-Most airways/blood vessels surrounded by a ring of inflammatory cells that were two to four cells deep; 4-Most airways/blood vessels were surrounded by a thick layer of inflammatory cells (more than four cells deep). Morphometric analysis was performed in a blinded fashion.
Measurement of MUC5AC in Human Lung Epithelial Cell Line
NCI-H292 cells, a human lung epithelial cell line, were purchased from the American Type Culture Collection (ATCC, Manassas, VA). The cells were cultured as previously described.Citation30 Briefly, cells were serum deprived, washed twice with PBS and confluent cells (5 x 105 cells/well in 24-well) were cultured in RPMI 1640 with 0.2% fetal bovine serum (FBS) for 24 hrs. After 24 hrs. of serum deprivation, cells were pre-treated with GAC1 (40 μg/mL) for 30 mins, after which the cells were stimulated with Phorbol 12-myristate 13-acetate (PMA,10 ng/mL) for 24 hrs. After 24 hrs., the cells were lysed in buffer RLT (Qiagen), and RNA was extracted using Qiagen RNA extraction kit as per manufacturer protocol. The total RNA was reverse transcribed to cDNA using RevertAid RT kit (Thermo Scientific, MA) as per manufacturer’s instructions. For each qRT-PCR, a 25 μL reaction was run with 12.5 μL maxima SYBR Green/ROX qPCR Master Mix 2x (Thermo Fisher, MA), 1.8 μL of 0.3 μM assays on demand primer, and 300 ng of RNA. The qRT-PCR was performed at 40 cycles of 25 °C for 5 mins, 42 °C for 60 mins and 70 °C for 15 mins. The Ct values for each gene were normalized by subtracting the Ct values for the housekeeping gene GAPDH (ΔCT). The relative fold change in mRNA expression between groups was calculated and expressed as 2−ΔΔCT. The following primers were used as previously described.Citation31 h-MUC5AC (forward) 5’-TGATCATCCAGCAGGGCT-3’ and (reverse) 5’-CCGAGCTCAGAGGACATATGGG-3’; h-GAPDH (forward) 5’-CAAAAGGGTCATCTCTG-3’ and (reverse) 5’-CCTGCTTCACCACCTTCTTG-3’.
Evaluation of Intracellular ROS Levels
Intracellular reactive oxygen species (ROS) levels were measured using the DCFDA/H2DCFDA-Cellular ROS assay kit (Abcam, MA) as per the manufacturer protocol. Briefly, 25,000 NCI-H292 cells were serum starved for 24 hrs. and seeded on a dark and clear bottom 96-well microplate overnight to allow the cells adhere. After 24 hrs., the cells were washed with PBS twice and pre-treated with GAC1 (40 μg/mL) for 30 mins after which the cells were stimulated with PMA (10 ng/mL). Twenty-four hours later, DCFDA (20 μM) was overlayed on top of the treated cells for 45 mins at 37° C in the dark after which the plate was read in an end point setting using a fluorescent reader (with excitation and emission wavelength of 485 and 535 nm, respectively). Data are represented as relative fluorescence unit (RFU).
Computational Analysis
Targets of GAC1 were mined from published databases including Swiss Target Prediction,Citation32,Citation33 Similarity Ensemble Approach,Citation34,Citation35 PubChem,Citation36,Citation37 PharmMapperCitation38 and DrugBank.Citation39,Citation40 The relevant human genes of asthma and glucocorticoid resistance were obtained from various databases including Therapeutic Target Database,Citation41,Citation42 Malacards,Citation43 GeneCards,Citation44,Citation45 and Open Targets Platform.Citation46,Citation47 The shared targets of GAC1 with asthma and glucocorticoid resistance were selected. Pathways and GO were conducted by mapping targets to KOBAS 3.0Citation48,Citation49 and DAVID database,Citation50,Citation51 respectively. Protein–Protein interaction (PPI) was constructed from String database.
Molecular Docking Analysis Predicts the Binding Modes of GAC1 with TNF-α
Molecular docking was performed on GAC1 and Dex with TNF-α (pdb: 2AZ5) by AutoDock VinaCitation52 to further explore and predict their binding modes. Protein crystal structures of STAT6 were downloaded from RCSB protein data bank. The structure of GAC1 and Dex was directly downloaded from PubChemCitation37,Citation53 without further optimization. TNF-α and Dex were prepared by AutoDockTools (v1.5.6).Citation54 The three dimensional molecular graphics were prepared by PyMOL systemCitation55 (http://www.pymol.org) and Discovery Studio.Citation56 Generally, all hydrogens and Gasteiger charges were added to protein and ligands.
Statistical Analysis
Data were analyzed by GraphPad Prism (San Diego, CA) using analysis of Variance (One way ANOVA) followed by a Bonferroni t-test for all pair-wise comparisons of all normally distributed data. The differences between groups were analyzed using the Wilcoxon Signed Rank test, where data were not normally distributed. The significant differences between groups for MUC5AC expression and ROS production were analyzed by Student’s t-test followed by Tukey’s post-test. P values ≤0.05 were considered significant.
Results
Treatment with GAC1 reduced airway neutrophil and eosinophil recruitment in RW sensitized and challenged mice
RW sensitization and challenge were performed as described in . Total BALF cell numbers () in the Sham group (2.87 × 105 ± 0.28 × 105) was significantly elevated compared to the naïve group (6.00 × 104 ± 0.19 × 104, P < 0.0001 vs Sham); however, acute Dex treatment did not affect total BALF cell counts (2.85 × 105 ± 0.21 × 105). Chronic GAC1 treated mice significantly decreased total BALF cell count (1.94 × 105 ± 0.35 × 105, P < 0.001 vs Sham). Neutrophil numbers () were similar in RW/Sham (0.97x105±0.25 x105/mL) and RW/Dex (1.12 x105± 0.112 × 105) but significantly reduced in the RW/GAC1 group (0.26 × 105 ± 0.10 × 105, P < 0.0001 vs RW/Sham and RW/Dex). Eosinophil numbers () were significantly reduced in RW/GAC1 (0.11 × 105 ± 0.10 × 105 P < 0.05 vs RW/Sham) and RW/Dex (0.14 × 105 ± 0.02 × 105, P < 0.05 vs RW/sham) when compared to RW/Sham mice (0.53 × 105 ± 0.24 × 105). Macrophage numbers () were similar across RW/Sham, RW/GAC1 and RW/Dex groups. Together, these findings demonstrated that RW challenge of RW-sensitized mice induced a predominantly neutrophilic airway inflammation, and whereas acute Dex treatment only suppressed eosinophilic inflammation, GAC1 suppressed neutrophilic as well as eosinophilic inflammation in this asthma model.
GAC1 Treatment Downregulated Th2 and Innate BALF Cytokines
Consistent with the anti-inflammatory profile of GAC1, we found significant inhibition of TNF-α, IL-4 and IL-5 in BALF from chronic GAC1 treated mice with inhibition rates over 60% (), however IFN-γ levels () were elevated suggesting GAC1 regulates Th2/Th1 innate immune responses without global immune-suppression. Acute Dex treatment was associated with reduction of IL-4 and IL-5 but no effect on TNF-α and IFN-γ.
Figure 3 Treatment with ASHMI and GAC1 Inhibited Th2 and Increased Th1 Responses. BAL fluid TNF-α (A) IL-4 (B), IL-5 (C) and IFN-γ (D) were measured by ELISA. Data expressed as Mean ± SD. N=3-5mice/group. *P<0.05, **P<0.01, ***P<0.001, compared with RW/Sham group; #P<0.05 compared with RW/Dex group.
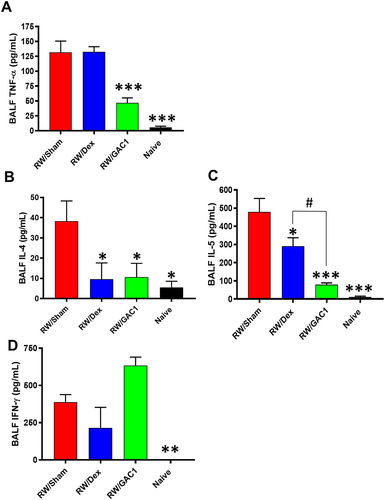
Mice Treated with GAC1 but Not Dex Showed Decreased Pulmonary Inflammation
Examination of lung histology showed peribronchial and perivascular inflammation in mice sensitized and challenged with RW, which was absent in lung sections from naïve mice (). Perivascular inflammation in particular was very pronounced with organized inflammatory lesions that were many cell layers deep ( lower panels). No change in either peribronchial or perivascular inflammation was observed in RW-sensitized mice treated with Dex. In contrast, such inflammatory lesions were markedly reduced and affected fewer airways (P < 0.01 vs Sham) and blood vessels (P < 0.001 vs Sham) in RW-sensitized mice treated with GAC1 ( and ).
Figure 4 Peribronchial and Perivascular Inflammation were Reduced in GAC1 but not Dex-Treated Mice. (A) 5-micron lung sections were stained with H&E stain and observed using 20x objective for airways (upper panels) and 40x objective for blood vessels (Lower panels); Peribronchial (B) and perivascular (C) inflammation scores were assigned using criteria described in Methods. Data shown as group means ± SD. N=3-5 mice/group. **P<0.01; ***P<0.001 compared with RW/Sham group; ##P<0.01; ###P<0.001 compared with RW/Sham group.
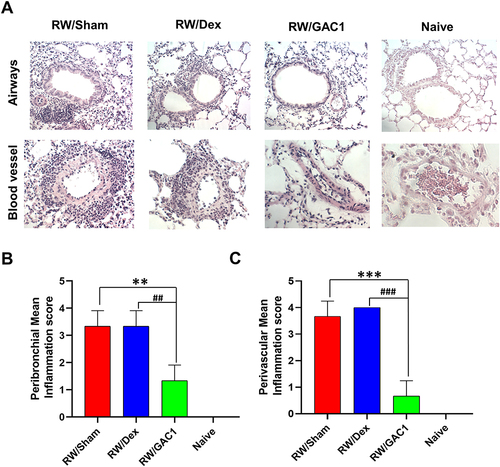
GAC1 Suppressed the Expression of MUC5AC and Decreased Intracellular ROS Levels
Mucin5ac (MUC5AC), as predominant mucin in asthma, has been associated with reduced pulmonary function and asthma exacerbation.Citation57 Moreover, oxidative stress has been identified as a main reason for this respiratory inflammation and plays a critical role in the pathogenesis of asthma.Citation58 To provide direct evidence whether GAC1 suppresses mucus cell hyperplasia and oxidative stress, we next test GAC1 effect on MUCAC gene expression and ROS production using lung epithelial cell line NCI-H292 in response to PMA stimulation. Significant inhibition of GAC1 on MUC5AC expression () was identified, which indicated that GAC1 may inhibit mucus production by regulating MUC5AC transcription. Moreover, intracellular ROS levels generated in response PMA stimulated H292 cells were suppressed significantly after GAC1 treatment ().
Figure 5 GAC1 Suppressed the Expression of MUC5AC and Decreased Intracellular ROS Levels. (A) Serum-deprived NCI-H292 cells were pretreated with GAC1 (40 ug/mL) for 30 mins followed by stimulation with PMA (10 ng/mL) and the relative expression of MUC5AC was measured. (B) GAC1 pre-treatment reduces PMA induced intracellular ROS production by H292 cells. After treatment, serum-starved cells were overlayed with DCFDA and the fluorescence intensity was evaluated. Data represents means ± SD. ***p<0.001 vs PMA stimulation group. N= three independent experiments performed in triplicate.
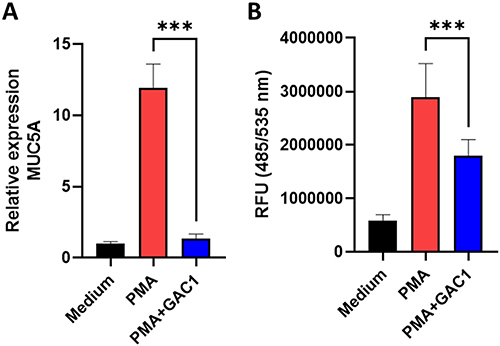
Computational analysis revealed that TNF-α might be critical target of GAC1 in relieving neutrophil-predominant airway inflammation
The compound-target-disease network in shows that 8 targets including TNF, AKT1, STAT6, HDAC2, IL17A, IFNG, NOS2 and NR3C1 are closely associated with GAC1 and both asthma and glucocorticoid resistance. Additionally, 21 other targets of GAC1 are considered as potentially regulated medium for asthma. PI3K-Akt response was associated with TNF-α levels, which play an important role in the pathogenesis of experimental and clinical steroid-resistant asthma. Pro-inflammatory factors such as IL17A, and IFNG undoubtedly participated in the regulation of TNF-α. Moreover, it’s widely known that the STAT6 and HDAC2 can regulate the expression and effects of TNF-α. Considering the extensive downstream regulated effects of TNF-α, it might be a promising therapeutic target in steroid-resistant asthma.Citation59
GAC1 Binding with TNF-α to Relieve Neutrophilic Airway Inflammation
Chronic GAC1 therapy significantly inhibited the level of TNF-α, while acute Dex showed no regulation in the level of TNF-α. To investigate the mechanism of such differentiation, molecular docking was applied to predict the binding modes of GAC1 and Dex with TNF-α, respectively. The binding energy of complex GAC1-TNF and Dex-TNF are −10.8 kcal/mol and −8.3 kcal/mol, respectively (), indicating higher stability of the GAC1-TNF complex. The conformations and binding interactions of GAC1-TNF and Dex-TNF are illustrated in . Both GAC1 and Dex can recognize and bind with inhibited pocket of TNF-α (). For GAC1, hydrogen bonds with TYR151 and the hydrophobic force with TYR59, and HIS15 stabilize the conformation of GAC1-TNF (). On the other hand, only hydrophobic force with TYR119 and TYR59 was formed in complex Dex-TNF. The binding affinity of GAC1 with TNF-α is higher than Dex with TNF-α. With higher binding affinity, GAC1 might be a stronger inhibitor for the TNF-α.
Table 1 Molecular Docking Results Between Compounds with TNF-α
Figure 7 Binding conformations of GAC1 and Dex with TNF-α. Binding conformation of GAC1 (A) and Dex (C) with TNF-α. The configuration and binding interactions of GAC1 (B) and Dex (D) with key amino acids. For GAC1 and Dex, carbon, oxygen and fluorine are highlighted in yellow, red and blue, respectively. For amino acids of protein, carbon, oxygen and nitrogen are displayed by green, red and blue, respectively. The green and pink lines indicate hydrogen bonds and hydrophobic interactions.
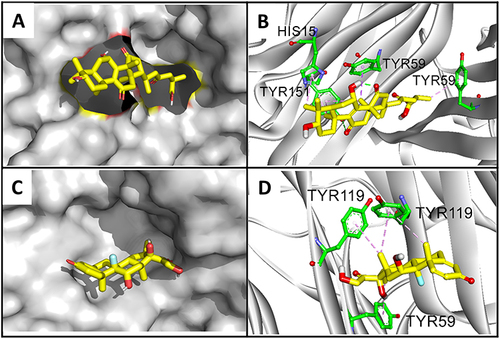
Discussion
Stimulated by TNF-α, ROS is generated from multiple cells in lung such as macrophages. Moreover, mucous is secreted from epithelial cells regulated by TNF-α. TNF-α, ROS, mucous, and other cytokines will help recruit neutrophiles to migrate to lung. In addition, the neutrophile activation and oxidative stress of ROS will further stimulate the generation of TNF-α, ROS, and mucous, which aggravate lung damage. By inhibiting the downstream signal TNF-α, GAC1 will improve neutrophilic inflammation by relieving oxidative stress, neutrophil recruitment, and mucous secretion ().
Figure 8 The Mechanism of GAC1 in Regulating Steroid-resistant Neutrophil Predominant Asthma. ROS are generated from multiple cells in lung such as epithelial cells, macrophages, and mast cells stimulated by TNF. Mucous is secreted from epithelial cells regulated by TNF-α. TNF-α, ROS, mucous, and other cytokines released by epithelial cells help recruit neutrophils to the lung. In addition, the neutrophil activation and oxidative stress of ROS will further stimulate the generation of TNF-α, ROS, and mucous, aggravating lung damage.
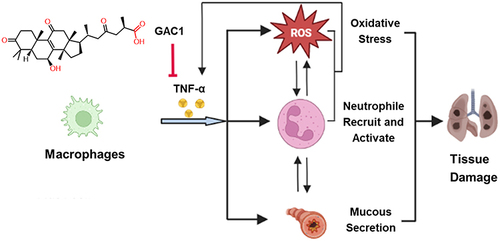
Neutrophilic airway inflammation is observed in the majority of patients belonging to the most severe asthma clusters, as defined in the SARP study.Citation2,Citation4 Recent reports have described a functional role for neutrophils in asthma pathology via the release of pro-inflammatory mediators and formation of Neutrophil Extracellular Traps (NETs), which lead to epithelial cell dysfunction and degradation.Citation60–65 Persistence of neutrophils has been observed in adult and pediatric patients with severe asthma.Citation66,Citation67 However, treatment of patients with neutrophil-dominant asthma is still limited by the availability of few therapeutic options.Citation9
Neutrophil-predominant murine asthma models are helpful for exploring possible treatments for T2 low severe asthma. We previously generated an RW-sensitized and RW-challenged murine asthma model characterized by neutrophil-predominant, mixed granulocytic airway inflammation.Citation22
We demonstrated that ASHMITM and refined ASHMI (ASHMIII) could significantly suppress both neutrophil and eosinophil inflammation, regulate Th1/Th2/innate immune responses, and reduce AHR and RW specific IgE. In the present study, we found that GAC1 isolated from Ganoderma lucidum, a component herb of ASHMI, has similar ability to suppress not only eosinophilic but also neutrophilic inflammation in the RW asthma model. We were the first to describe TNF-α reducing activity of Ganoderic acid C1 in a macrophage cell line, human PBMCs and IBD patient biopsies. Ganoderic acids are lanostane triterpenoids, and multiple isoforms have been described with varying biological activity.Citation68 They are chiefly valued for their anti-cancer properties,Citation69 and considerable research has been devoted to enhancing ganoderic acid content of Ganoderma species.Citation70 RW is a more clinically relevant antigen than ovalbumin, but ovalbumin is more commonly used in the experimental studies than RW.Citation22 Our previous study defined that RW induced neutrophilic inflammation in this model was not due to endotoxin, because RW induced a significantly higher (>10-fold) BALF neutrophil percentage than ovalbumin following a suboptimal protocol in our preliminary study, whereas the RW endotoxin level (9 pg/mL) was only 0.001% that of ovalbumin (12 ng/mL). The ability of RW to induce neutrophilic inflammation has been previously demonstrated to be attributable to intrinsic NADPH oxidases.Citation71
To investigate molecular mechanisms that would explain GAC1 efficacy in neutrophil-dominant asthma, computational analysis was utilized to predict the potential targets of GAC1 with asthma under the restriction of glucocorticoid resistance. GAC1 potential targets including TNF, AKT1, STAT6, HDAC2, IL17A, IFNG, NOS2 and NR3C1 were strongly associated with asthma and steroid resistance. A key finding of GAC1 activity in the current study is that GAC1, but not Dex, treatment reduced pulmonary TNF-α production. TNF-α is considered to play an important role in the recruitment of neutrophils and induce steroid resistance in severe asthma.Citation10,Citation72 TNF-α blockade has been shown to restore steroid sensitivity in a mouse model of neutrophilic-predominant inflammation.Citation18 Failure to reduce TNF-α may be a major reason underlying the inability of acute Dex treatment to decrease neutrophilic inflammation in this steroid-resistant asthma model despite Dex’s ability to reduce eosinophil numbers. The binding affinities of GAC1 with TNF-αt are clearly higher than with Dex. GAC1-reduction of TNF-α in vivo in the current study confirms our earlier in vitro studies showing that GAC1 could inhibit RW or LPS induced TNF-α production in a mouse macrophage cell line and PBMCs from asthma patients and decrease TNF-α production by PBMCs and inflammation in colonic biopsies from Crohn’s Disease patients.Citation22,Citation26,Citation27
In addition, we, for the first time, provided direct evidence that GAC1 reduced MUC5AC, a marker of mucus cell hyperplasia, and reduced ROS production, a marker of oxidative stress.Citation73 It has been shown that mucus hyperplasia is one of the markers of airway remodeling, and ROS has been shown to be involved in both eosinophilic and neutrophilic inflammation, and airway remodeling.Citation74 These findings further suggested that GAC1 suppression of MU5AC and ROS may coordinate with its anti-TNF-a effect leading to protection against neutrophilic asthma. However, the effect of GAC1 on MUC5AC in vivo has not been evaluated. Further studies are needed to understand GAC1 anti-airway remodeling, and the effect of GAC1 on MUC5AC and ROS production in treating neutrophilic asthma. Moreover, the regulation of GAC1 on neutrophil survival and functions has not been investigated in this study. In the future work, we will focus on the regulation of GAC1 on neutrophils.
Conclusion
In summary, we showed for the first time that GAC1, an active compound isolated from ASHMI, inhibited neutrophil-predominant airway inflammation in vivo, using an RW sensitized and challenged steroid-resistant murine asthma model. GAC1 benefits were associated with reduction of Th2 cytokines and TNFα. GAC1 administration was via the oral route, implying satisfactory bioavailability and increasing feasibility of future development as a botanical anti-asthma drug tailored to target neutrophil-dominant asthma, which is often severe and currently underserved by advances in asthma therapeutics. The chronic DEX treatment and inflammatory cell kinetic infiltration of the model have not been investigated in this study. They will be explored to further characterize the neutrophil-predominant mouse asthma model in our future work. Moreover, the content of GAC1 is low in Ganoderma lucidum, which limits the application of GAC1. However, chemical synthetic GAC1 will provide an opportunity to ensure the medicinal sourcing for developing GAC1 as anti-inflammatory drug for asthma therapy.
Disclosure
Xiu-Min Li received research support to her institution from the National Institutes of Health (NIH)/National Center for Complementary and Integrative Health (NCCAM) # 1P01 AT002644725-01 “Center for Chinese Herbal Therapy (CHT) for Asthma”, and grant #1R01AT001495-01A1 and 2R01 AT001495- 05A2, NIH/NIAID R43AI148039, NIH/NIAID 1R21AI176061-01, NIH/NIAID 1R44AI177183-01, NIH/NIAID 1R41AI172572-01A1, Food Allergy Research and Education (FARE), Winston Wolkoff Integrative Medicine Fund for Allergies and Wellness, the Parker Foundation and Henan University of Chinese Medicine; received consultancy fees from FARE and Johnson & Johnson Pharmaceutical Research & Development, L.L.C. Bayer Global Health LLC; received royalties from UpToDate; received travel expenses from the NCCAM and FARE; share US patent US7820175B2 (FAHF-2), US10500169B2 (XPP), US10406191B2 (S. Flavescens), US10028985B2 (WL); US11351157B2 (nanoBBR); take compensation from her practice at Center for Integrative Health and Acupuncture PC; US Times Technology Inc is managed by her related party; is a member of General Nutraceutical Technology LLC. Nan Yang received research support to his institute from the National Institutes of Health (NIH)/ National Center for Complementary and Alternative Medicine (NCCAM), NIH/NIAID R43AI148039, NIH/NIAID 1R21AI176061- 01, NIH/NIAID 1R44AI177183-01, NIH/NIAID 1R41AI172572- 01A1; shares US patent: US10500169B2 (XPP), US10406191B2 (S. Flavescens), US10028985B2 (WL); and is a member of General Nutraceutical Technology, LLC; receives a salary from General Nutraceutical Technology, LLC; Kamal Srivastava shares US11351157B2 (nanoBBR); Hugh Sampson shares US patent PCT/ AQ7 US05/008417 (FAHF-2), PCT/US2014/012306 (S. Flavescens). He also reports personal fees for consulting and stock options from DBV Technologies and N-Fold, LLC; personal fees for Scientific Advisory Board from Siolta Therapeutics; and personal fees for serving on a Anaphylaxis Adjudication Committee from AbbVie, Inc, outside the submitted work. The remaining authors declare that the research was conducted in the absence of any commercial or financial relationships that could be construed as a potential conflict of interest.
Acknowledgments
The interim findings of the abstract of this paper were presented at the American Society of Biochemistry and Molecular Biology held in Seattle, WA, from March 25-28,2023 as a poster presentation. The abstract has been published in Journal of Biological Chemistry. The DOI of this abstract is 10.1016/j.jbc.2023.103268.
Additional information
Funding
References
- Lang DM. Severe asthma: epidemiology, burden of illness, and heterogeneity. Allergy Asthma Proc 2015;36:418–424.
- Backman H, Jansson SA, Stridsman C, et al. Severe asthma-A population study perspective. Clin Exp Allergy. 2019;49:819–828. doi:10.1111/cea.13378
- Fitzpatrick AM, Moore WC. Severe asthma phenotypes - how should they guide evaluation and treatment? J Allergy Clin Immunol Pract. 2017;5:901–908. doi:10.1016/j.jaip.2017.05.015
- Moore WC, Hastie AT, Li X, et al. Sputum neutrophil counts are associated with more severe asthma phenotypes using cluster analysis. J Allergy Clin Immunol. 2014;133:1557–1563.e1555. doi:10.1016/j.jaci.2013.10.011
- Panettieri RA. The role of neutrophils in asthma. Immunol Allergy Clin North Am. 2018;38:629–638. doi:10.1016/j.iac.2018.06.005
- Panettieri RA. Neutrophilic and pauci-immune phenotypes in severe asthma. Immunol Allergy Clin North Am. 2016;36:569–579. doi:10.1016/j.iac.2016.03.007
- Bruijnzeel PL, Uddin M, Koenderman L. Targeting neutrophilic inflammation in severe neutrophilic asthma: can we target the disease-relevant neutrophil phenotype? J Leukoc Biol. 2015;98:549–556. doi:10.1189/jlb.3VMR1214-600RR
- Seys SF, Lokwani R, Simpson JL, Bullens DMA. New insights in neutrophilic asthma. Curr Opin Pulm Med. 2019;25(1):113–120. doi:10.1097/MCP.0000000000000543
- Pepper AN, Renz H, Casale TB, Garn H. Biologic therapy and novel molecular targets of severe asthma. J Allergy Clin Immunol Pract. 2017;5(4):909–916. doi:10.1016/j.jaip.2017.04.038
- Svenningsen S, Nair P. Asthma endotypes and an overview of targeted therapy for asthma. Front Med Lausanne. 2017;4:158. doi:10.3389/fmed.2017.00158
- Malaviya R, Laskin JD, Laskin DL. Anti-TNFalpha therapy in inflammatory lung diseases. Pharmacol Ther. 2017;180:90–98. doi:10.1016/j.pharmthera.2017.06.008
- Lee HS, Park HW, Song WJ, et al. TNF-alpha enhance Th2 and Th17 immune responses regulating by IL23 during sensitization in asthma model. Cytokine. 2016;79:23–30. doi:10.1016/j.cyto.2015.12.001
- Berry M, Brightling C, Pavord I, Wardlaw A. TNF-alpha in asthma. Curr Opin Pharmacol. 2007;7:279–282. doi:10.1016/j.coph.2007.03.001
- Huang H, Nie W, Qian J, et al. Effects of TNF-alpha polymorphisms on asthma risk: a systematic review and meta-analysis. J Investig Allergol Clin Immunol. 2014;24:406–417.
- Manthei DM, Schwantes EA, Mathur SK, et al. Nasal lavage VEGF and TNF-alpha levels during a natural cold predict asthma exacerbations. Clin Exp Allergy. 2014;44:1484–1493. doi:10.1111/cea.12387
- Lukacs NW, Strieter RM, Chensue SW, Widmer M, Kunkel SL. TNF-alpha mediates recruitment of neutrophils and eosinophils during airway inflammation. J Immunol. 1995;154:5411–5417. doi:10.4049/jimmunol.154.10.5411
- Hardyman MA, Wilkinson E, Martin E, et al. TNF-α-mediated bronchial barrier disruption and regulation by src-family kinase activation. J Allergy Clin Immunol. 2013;132:665–675.e668. doi:10.1016/j.jaci.2013.03.005
- Dejager L, Dendoncker K, Eggermont M, et al. Neutralizing TNFα restores glucocorticoid sensitivity in a mouse model of neutrophilic airway inflammation. Mucosal Immunol. 2015;8:1212–1225. doi:10.1038/mi.2015.12
- Taille C, Poulet C, Marchand-Adam S, et al. Monoclonal anti-TNF-alpha antibodies for severe steroid-dependent asthma: a case series. Open Respir Med J. 2013;7:21–25. doi:10.2174/1874306401307010021
- Wen MC, Wei CH, Hu ZQ, et al. Efficacy and tolerability of anti-asthma herbal medicine intervention in adult patients with moderate-severe allergic asthma. J Allergy Clin Immunol. 2005;116:517–524. doi:10.1016/j.jaci.2005.05.029
- Zhang T, Srivastava K, Wen MC, et al. Pharmacology and immunological actions of a herbal medicine ASHMI on allergic asthma. Phytother Res. 2010;24:1047–1055. doi:10.1002/ptr.3077
- Srivastava KD, Dunkin D, Liu C, et al. Effect of antiasthma simplified herbal medicine intervention on neutrophil predominant airway inflammation in a ragweed sensitized murine asthma model. Ann Allergy Asthma Immunol. 2014;112:339–347. doi:10.1016/j.anai.2014.01.021
- Ito K, Herbert C, Siegle JS, et al. Steroid-resistant neutrophilic inflammation in a mouse model of an acute exacerbation of asthma. Am J Respir Cell Mol Biol. 2008;39:543–550. doi:10.1165/rcmb.2008-0028OC
- Bogaert P, Naessens T, De Koker S, et al. Inflammatory signatures for eosinophilic vs. neutrophilic allergic pulmonary inflammation reveal critical regulatory checkpoints. Am J Physiol Lung Cell Mol Physiol. 2011;300:L679–690.
- Essilfie AT, Horvat JC, Kim RY, et al. Macrolide therapy suppresses key features of experimental steroid-sensitive and steroid-insensitive asthma. Thorax. 2015;70:458–467. doi:10.1136/thoraxjnl-2014-206067
- Liu C, Yang N, Song Y, et al. Ganoderic acid C1 isolated from the anti-asthma formula, ASHMI suppresses TNF-alpha production by mouse macrophages and peripheral blood mononuclear cells from asthma patients. Int Immunopharmacol. 2015;27:224–231. doi:10.1016/j.intimp.2015.05.018
- Liu C, Dunkin D, Lai J, et al. Anti-inflammatory effects of Ganoderma lucidum triterpenoid in human crohn’s disease associated with downregulation of NF-κB signaling. Inflamm Bowel Dis. 2015;21:1918–1925. doi:10.1097/MIB.0000000000000439
- Kelly-Pieper K, Patil SP, Busse P, et al. Safety and tolerability of an antiasthma herbal formula (ASHMI™) in adult subjects with asthma: a randomized, double-blinded, placebo-controlled, dose-escalation Phase I study. J Altern Complementary Med. 2009;15:735–743. doi:10.1089/acm.2008.0543
- Institute of Laboratory Animal Resources Commission of Life Sciences NRC. Guide for the Care and Use of Laboratory Animals. National Academic Press; 1996.
- Liu C, Weir D, Busse P, et al. The flavonoid 7,4’-dihydroxyflavone inhibits muc5ac gene expression, production, and secretion via regulation of NF-kappaB, STAT6, and HDAC2. Phytother Res. 2015;29:925–932. doi:10.1002/ptr.5334
- Lee HJ, Lee SY, Bae HS, et al. Inhibition of airway MUC5AC mucin production and gene expression induced by epidermal growth factor or phorbol ester by glycyrrhizin and carbenoxolone. Phytomedicine. 2011;18:743–747. doi:10.1016/j.phymed.2010.11.003
- Swiss Target Prediction. Available from: https://swisstargetprediction.ch
- Daina A, Michielin O, Zoete V. SwissTargetPrediction: updated data and new features for efficient prediction of protein targets of small molecules. Nucleic Acids Res. 2019;47:W357–w364.
- Similarity Ensemble Approach. Available from: https://sea.bkslab.org
- Keiser MJ, Roth BL, Armbruster BN, Ernsberger P, Irwin JJ, Shoichet BK. Relating protein pharmacology by ligand chemistry. Nat Biotech. 2007;25(2):197–206. doi:10.1038/nbt1284
- PubChem. Available from: https://pubmed.ncbi.nlm.nih.gov/
- Kim S, Chen J, Cheng T, et al. PubChem 2019 update: improved access to chemical data. Nucleic Acids Res. 2019;47:D1102–d1109.
- Wang X, Shen Y, Wang S, et al. PharmMapper 2017 update: a web server for potential drug target identification with a comprehensive target pharmacophore database. Nucleic Acids Res. 2017;45:W356–w360.
- DrugBank. Available from: https://go.drugbank.com
- Wishart DS, Knox C, Guo AC, et al. DrugBank: a knowledgebase for drugs, drug actions and drug targets. Nucleic Acids Res. 2008;36:D901–906. doi:10.1093/nar/gkm958
- Therapeutic target database.
- Wang Y, Zhang S, Li F, et al. Therapeutic target database 2020: enriched resource for facilitating research and early development of targeted therapeutics. Nucleic Acids Res. 2020;48:D1031–d1041.
- Rappaport N, Twik M, Plaschkes I, et al. MalaCards: an amalgamated human disease compendium with diverse clinical and genetic annotation and structured search. Nucleic Acids Res. 2017;45:D877–d887.
- GeneCards. Available from: https://genecards.org
- Safran M, Solomon I, Shmueli O, et al. GeneCards 2002: towards a complete, object-oriented, human gene compendium. Bioinformatics. 2002;18:1542–1543. doi:10.1093/bioinformatics/18.11.1542
- Open targets platform. Available from: https://platform.opentargets.org
- Carvalho-Silva D, Pierleoni A, Pignatelli M, et al. Open Targets Platform: new developments and updates two years on. Nucleic Acids Res. 2019;47:D1056–d1065.
- Xie C, Mao X, Huang J, et al. KOBAS 2.0: a web server for annotation and identification of enriched pathways and diseases. Nucleic Acids Res. 2011;39:W316–W322. doi:10.1093/nar/gkr483
- KOBAS 3.0; 2020. Available from: http://kobas.cbi.pku.edu.cn
- Huang DW, Sherman BT, Lempicki RA. Bioinformatics enrichment tools: paths toward the comprehensive functional analysis of large gene lists. Nucleic Acids Res. 2009;37(1):1–13. doi:10.1093/nar/gkn923
- DAVID. Available from: http://david.ncifcrf.gov
- Trott O, Olson AJ. AutoDock Vina: improving the speed and accuracy of docking with a new scoring function, efficient optimization, and multithreading. J Comput Chem. 2010;31:455–461. doi:10.1002/jcc.21334
- Müller CW, Rey FA, Sodeoka M, Verdine GL, Harrison SC. Structure of the NF-kappa B p50 homodimer bound to DNA. Nature. 1995;373:311–317. doi:10.1038/373311a0
- Sanner MF. Python: a programming language for software integration and development. J Mol Graph Model. 1999;17:57–61.
- DeLano WL. Pymol: an open-source molecular graphics tool. Newsletter on Protein Crysta. 2002;40:82–92.
- DassaultSystèmesBIOVIAD. Discovery Studio; 2020.
- Chen M, Lv Z, Zhang W, et al. Triptolide suppresses airway goblet cell hyperplasia and Muc5ac expression via NF-κB in a murine model of asthma. Mol Immunol. 2015;64:99–105. doi:10.1016/j.molimm.2014.11.001
- Cho YS, Moon HB. The role of oxidative stress in the pathogenesis of asthma. Allergy Asthma Immunol Res. 2010;2:183–187. doi:10.4168/aair.2010.2.3.183
- Brightling C, Berry M, Amrani Y. Targeting TNF-α: a novel therapeutic approach for asthma. J Allergy Clin Immunol. 2008;121(1):5–12. doi:10.1016/j.jaci.2007.10.028
- Thomson NC. Novel approaches to the management of noneosinophilic asthma. Ther Adv Respir Dis. 2016;10:211–234. doi:10.1177/1753465816632638
- Nair P, Aziz-Ur-Rehman A, Radford K. Therapeutic implications of ‘neutrophilic asthma’. Curr Opin Pulm Med. 2015;21:33–38. doi:10.1097/MCP.0000000000000120
- Halwani R, Sultana A, Vazquez-Tello A, Jamhawi A, Al-Masri AA, Al-Muhsen S. Th-17 regulatory cytokines IL-21, IL-23, and IL-6 enhance neutrophil production of IL-17 cytokines during asthma. J Asthma. 2017;54:893–904. doi:10.1080/02770903.2017.1283696
- Kim RY, Pinkerton JW, Essilfie AT, et al. Role for NLRP3 inflammasome-mediated, il-1beta-dependent responses in severe, steroid-resistant asthma. Am J Respir Crit Care Med. 2017;196:283–297. doi:10.1164/rccm.201609-1830OC
- Lachowicz-Scroggins ME, Dunican EM, Charbit AR, et al. Extracellular DNA, neutrophil extracellular traps, and inflammasome activation in severe asthma. Am J Respir Crit Care Med. 2019;199:1076–1085. doi:10.1164/rccm.201810-1869OC
- Uddin M, Watz H, Malmgren A, Pedersen F. NETopathic inflammation in chronic obstructive pulmonary disease and severe asthma. Front Immunol. 2019;10:47. doi:10.3389/fimmu.2019.00047
- Grunwell JR, Stephenson ST, Tirouvanziam R, Brown LAS, Brown MR, Fitzpatrick AM. Children with neutrophil-predominant severe asthma have proinflammatory neutrophils with enhanced survival and impaired clearance. J Allergy Clin Immunol Pract. 2019;7:516–525.e516. doi:10.1016/j.jaip.2018.08.024
- Uddin M, Nong G, Ward J, et al. Prosurvival activity for airway neutrophils in severe asthma. Thorax. 2010;65:684–689. doi:10.1136/thx.2009.120741
- Hsu CL, Yen GC. Ganoderic acid and lucidenic acid (Triterpenoid). Enzymes. 2014;36:33–56.
- Ruan W, Wei Y, Popovich DG. Distinct responses of cytotoxic Ganoderma lucidum triterpenoids in human carcinoma cells. Phytother Res. 2015;29:1744–1752. doi:10.1002/ptr.5426
- Xu JW, Zhong JJ. Genetic engineering of Ganoderma lucidum for the efficient production of ganoderic acids. Bioengineered. 2015;6:357–360. doi:10.1080/21655979.2015.1119341
- Boldogh I. ROS generated by pollen NADPH oxidase provide a signal that augments antigen-induced allergic airway inflammation. J Clin Invest. 2005;115(8):2169–2179. doi:10.1172/JCI24422
- Berry MA, Hargadon B, Shelley M, et al. Evidence of a role of tumor necrosis factor alpha in refractory asthma. N Engl J Med. 2006;354:697–708. doi:10.1056/NEJMoa050580
- Bonser LR, Erle DJ, Iwata N. Airway Mucus and Asthma: the Role of MUC5AC and MUC5B. J Clin Med. 2017;6(1):6. doi:10.3390/jcm6010006
- Michaeloudes C, Abubakar-Waziri H, Lakhdar R, et al. Molecular mechanisms of oxidative stress in asthma. Mol Aspects Med. 2022;85:101026. doi:10.1016/j.mam.2021.101026