To the Editor,
Intensity-modulated radiation therapy (IMRT) is particularly useful for treating irregularly shaped planning target volumes (PTVs) whilst minimizing doses to organs at risk (OARs). Volumetric modulated arc therapy (VMAT) is a form of rotational IMRT that typically requires less delivery time and monitor units (MU) compared to static IMRT beams. Treatment planning aims to create plans with an optimal trade-off between PTV coverage and OAR sparing [Citation1–3]. In the search for better VMAT plans there are many parameters that the planner can modify, including the number of arcs. We previously demonstrated that RapidArc® (Varian Medical Systems, Palo Alto, CA, USA) with two arcs was associated with improved plan quality over a single arc and increased agreement between calculated and measured doses, while beam-on time was still less than 3 minutes [Citation4]. Since the RapidArc optimization algorithm tries to maximize gantry speed we hypothesized that using more than two arcs, which would allow for a longer delivery time and more opportunity for modulation, might translate into further gains in plan quality. We investigated the relationship between the number of arcs, PTV dose homogeneity and OAR sparing.
Material and methods
General considerations
Planning CT scans from 10 patients with head and neck cancer previously treated using RapidArc were selected for this retrospective planning study. The head and neck location was selected because it is a challenging scenario for treatment planning. Plans were created using the current institutional simultaneous integrated boost (SIB) technique described in detail previously [Citation5]. In brief, this aims to deliver 54.25 Gy/70 Gy to the elective/boost PTV (PTVE/PTVB) in 35 fractions. PTVB consisted of the gross tumor volume and biopsy-proven positive lymph nodes with a 5 mm margin for microscopic disease (edited for anatomical boundaries) and a 4–5 mm PTV margin. PTVE consisted of elective nodal regions, with a 4–5mm PTV margin, minus a 5mm transition zone (PTVT), which was created between PTVB and PTVE to allow for a steep dose falloff.
OARs with only a maximum dose objective taken into account in the optimization included the brainstem, spinal cord, and their planning at risk volumes (PRV) after 3mm expansion. OAR for which the aim was to reduce the mean dose included salivary structures (e.g. the ipsilateral and contralateral parotid and submandibular glands) and swallowing structures (e.g. the upper esophageal sphincter, upper and lower larynx, the superior, medial and inferior pharyngeal constrictor muscle, cricopharyngeal muscle and esophagus). The treating clinician may have decided not to spare an individual OAR, for example if there was substantial overlap with the PTV. Composite salivary (compsal) and swallowing (compswal) structures (consisting of individually optimized OARs) were created for OAR dose reporting.
Treatment planning
Coplanar, 6MV RapidArc plans were created for all 10 patients using two, four, six and eight full arcs optimized using the progressive resolution optimizer (PRO, version 10.0.28; Eclipse treatment planning Varian Medical Systems, Palo Alto, CA, USA). Collimator angles were chosen with a 5° difference between individual arcs. Volume dose was calculated using the Anisotropic Analytical Algorithm (AAA, version 10.0.28) with a 2.5mm dose grid. After a first optimization and dose calculation, a ‘continue previous optimization’ (CPO) was performed to improve PTV dose homogeneity [Citation5]. All plans were created following our institutional approach to interactive planning [Citation6,Citation7]. Briefly, the spinal cord, brainstem and their PRVs were each constrained well below their respective tolerance levels using single maximum dose objectives (optimization priority 120). As shoulder position can vary slightly during and between fractions, direct irradiation through them was limited (using a maximum dose objective) to increase the robustness of the plan. To minimize the mean dose to the individual salivary glands and swallowing muscles, 4–5 objectives with typical optimization priorities of 80–90 were used for each structure. The dose-volume location of these objectives was regularly adjusted during the optimization process, aiming to keep them at a constant distance from the OAR dose-volume histogram line that is displayed during optimization. The boost and elective PTVs had minimum and maximum dose objectives placed respectively 1 Gy lower and higher than the prescription dose, with PTV optimization priorities (POP) of 150 (POP150).
Plans were evaluated using institutional guidelines. The goal was that at least 99% of the boost volume should be covered by 95% of the prescribed dose (V95% ≥ 99%) and no more than 4% should receive a dose greater than 107% (V107% ≤ 4%). The goals for PTVE dose homogeneity were V95% ≥ 98% and V107% ≤ 15%.
Study endpoints
Dose inhomogeneity (IH) was calculated in all plans for both PTVB (IHB) and PTVE (IHE) using the equation IH = (100%–V95%)+ V107%. This metric for PTV dose homogeneity consists of the PTV dose values that are analyzed clinically in our institute (V95% and V107%) and is consistent with ICRU reports 50 and 62. To allow for a broader comparison of our results, the homogeneity index (HI) was also calculated for both the boost (HIB) and elective (HIE) PTVs using the equation suggested by ICRU 83 HI = (D2%–D98%)/D50%. Mean doses to compsal and compswal were averaged to provide a composite mean OAR (OARcomp) dose. To investigate whether additional arcs increase dose deposition in the remaining body, the body minus PTV volume receiving a dose ≥ 5Gy (V5Gy), ≥ 30Gy (V30Gy) and ≥ 50Gy (V50Gy) was determined. An ANOVA test with post hoc Bonferroni analysis was performed (using IBM SPSS, Armonk, NY, USA) to investigate which of the analyzed parameters improved significantly using more arcs. Finally, we reported the number of monitor units (MU) as a surrogate for plan modulation.
To investigate the effect of using more arcs for different PTV optimization priorities, 1–8 arc plans were made for patients 1–3 at three POP levels: POP130, POP150 and POP170. The boost and elective PTVs used the same POP value.
Dosimetric validation was performed for the two, four, six and eight arc plans made with POP150 of patients 1–3. Delivery time was measured for the two, four, six and eight arc plans of patient 1 on the TrueBeam (Varian Medical Systems). A more detailed explanation is found in the Appendix, available online at http://informahealthcare.com/doi/abs/10.3109/0284186X.2014.934968.
Results
Data on disease site, stage, PTV and OAR volumes is reported in Supplementary Table I (available online at http://informahealthcare.com/doi/abs/10.3109/0284186X.2014.934968). Maximum dose constraints to the brainstem and spinal cord were met in all plans. Increasing the number of arcs increased PTV dose homogeneity, OAR sparing and MU (). The gains in OAR sparing were similar for the individual salivary structures (supporting the use of composite salivary structures and OARcomp for OAR dose reporting). Except for body-PTV doses, IHB and PTVB V95% and V107%, all parameters improved significantly when four instead of two arcs were used. The magnitude of the gains decreased going from four to six and four to eight arcs. No statistically significant differences were obtained when increasing from six to eight arcs. shows the effect of increasing the number of arcs on IHB, IHE, compsal and compswal for all individual patients. Although the initial value and subsequent gains in these parameters vary, all plans benefitted from additional arcs either by lowering IHB/IHE, or OAR dose, or both. When a homogeneous PTV dose was already obtained with two arcs, more arcs could still improve OAR dose (e.g. in patient 1). In contrast, in some cases where there was only a minor reduction in OAR dose, bigger gains in IHB and IHE were possible (e.g. in patient 9). For some patients with large PTVB volumes (e.g. patient 2, 8 and 9), it was hard to obtain adequate PTVB coverage using two arcs and IHB values were higher. In this case adding more arcs resulted in improvements in IHB. HIB and HIE were also consistently lower using additional arcs. V5Gy and V30Gy both tended to increase using more arcs, although this increase was no more than 1.7% and 0.4%, respectively, and therefore unlikely to be clinically relevant. V50Gy decreased by less than 0.02% when using more arcs.
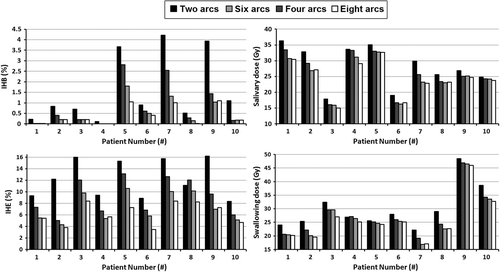
Table I. The effect of arc number on boost and elective planning target volume (PTV) dose inhomogeneity (IHB/HIB and IHE/HIE respectively) and organ-at-risk (OAR) mean dose.
In the Appendix, gamma analysis of the POP150 plans of patients 1–3 can be found, along with delivery times. Supplementary Figure 1 (available online at http://informahealthcare.com/doi/abs/10.3109/0284186X.2014.934968) illustrates for three patients the PTV homogeneity and OARcomp dose for 1–8 arcs and POP130, POP150 and POP170.
Discussion
In this systematic investigation of the effect of arc number on VMAT plan quality using RapidArc we found a clear trend for improved OAR sparing and more homogeneous PTV doses going from one to eight arcs in head and neck VMAT plans with a simultaneous integrated boost. The largest absolute gains were found when increasing the number of arcs from two, which is mostly used in clinical practice, to four. Although the improvements were smaller, further gains were still possible using more than four arcs. Taking into account the available literature regarding normal tissue toxicity [Citation8–11], we think that the dosimetric gains are sufficient to be clinically relevant. The resulting increase in plan quality comes at the expense of increased delivery time, and a modest increase in MU, both of which we consider clinically acceptable. To put this increased delivery time into context, our four arc plans were delivered in under 4.5 minutes whereas the single arc SmartArc plans in the study of Lechner et al. [Citation3] could take more than 6 minutes. This illustrates that data concerning the use of multiple arcs from other systems, e.g. SmartArc (Philips Healthcare) or VMAT (Elekta) cannot be extrapolated to RapidArc because of fundamental differences in the treatment planning/delivery system [Citation12–18]. This makes it necessary to perform system-specific treatment planning studies like the present report. Whether these results can be generalized to other anatomical locations or to other versions of the treatment planning system requires further e valuation.
One reason for improved plan quality when using more arcs might be specific characteristics of the RapidArc optimizer. It tends to create a treatment plan in which the gantry rotates at maximum speed, while dose rate and leaf positions are modulated. Only if the maximum dose rate prevents sufficient delivery of dose, is the gantry speed reduced. Our results suggest that two arcs delivered at maximum gantry speed cannot achieve sufficient fluence modulation to create an optimal plan. It is therefore possible that RapidArc plans with one or two arcs could be created with similar quality to four or more arcs if the optimizer allowed gantry slowdown.
In conclusion, using the clinical scenario of complex head and neck radiotherapy, we have quantitatively demonstrated increased plan quality when more than two arcs are used. The four arc plans seemed to provide a good trade-off between increased delivery time and improved plan quality. Data such as this illustrate that gains in complex photon plans are still possible using existing technology.
Supplementary material available online
Supplementary Appendix, Figure 1 and Table I to be found online at http://informahealthcare.com/doi/abs/10.3109/0284186X.2014.934968).
ionc_a_934968_sm8554.pdf
Download PDF (293.4 KB)Acknowledgments
For input on the statistical analysis we would like to thank Dr. Alexander Louie.
Declaration of interest: The authors alone are responsible for the content and writing of the paper.
This work has been funded by Varian Medical Systems. The department has a research collaboration with Varian Medical Systems and Dahele, Slotman, and Verbakel have received honoraria/travel support from Varian.
References
- Ottosson RO, Engstrom PE, Sjöström D, Behrens CF, Karlsson A, Knöös T, et al. The feasibility of using Pareto fronts for comparison of treatment planning systems and delivery techniques. Acta Oncol 2009;48:233–7.
- Craft DL, Halabi TF, Shih HA, Bortfeld TR. Approximating convex pareto surfaces in multiobjective radiotherapy planning. Med Phys 2006;33:3399–407.
- Lechner W, Kragl G, Georg D. Evaluation of treatment plan quality of IMRT and VMAT with and without flattening filter using Pareto optimal fronts. Radiother Oncol 2013; 109:437–41.
- Verbakel WF, Cuijpers JP, Hoffmans D, Bieker M, Slotman BJ, Senan S. Volumetric intensity-modulated arc therapy vs. conventional IMRT in head-and-neck cancer: A comparative planning and dosimetric study. Int J Radiat Oncol Biol Phys 2009;74:252–9.
- Tol JP, Dahele M, Doornaert P, Slotman BJ, Verbakel WF. Toward optimal organ at risk sparing in complex volumetric modulated arc therapy: An exponential trade-off with target volume dose homogeneity. Med Phys 2014;41:021722.
- Doornaert P, Verbakel WF, Rietveld DHF, Slotman BJ, Senan S. Sparing the contralateral submandibular gland without compromising PTV coverage by using volumetric modulated arc therapy. Radiat Oncol 2011;6:74.
- Doornaert P, Verbakel WF, Bieker M, Slotman BJ, Senan S. RapidArc planning and delivery in patients with locally advanced head-and-neck cancer undergoing chemoradiotherapy. Int J Radiat Oncol Biol Phys 2011;79:429–35.
- Beetz I, Schilstra C, van der Schaaf A, van den Heuvel ER, Doornaert P, van Luijk P, et al. NTCP models for patient-rated xerostomia and sticky saliva after treatment with intensity modulated radiotherapy for head and neck cancer: The role of dosimetric and clinical factors. Radiother Oncol 2012;105:101–6.
- Christianen MEMC, Schilstra C, Beetz I, Muijs CT, Chouvalova O, Burlage FR, et al. Predictive modelling for swallowing dysfunction after primary (chemo)radiation: Results of a prospective observational study. Radiother Oncol 2012;105:107–14.
- Dijkema T, Raaijmakers CPJ, Ten Haken RK, Roesink JM, Braam PM, Houweling AC, et al. Parotid gland function after radiotherapy: The combined michigan and utrecht experience. Int J Radiat Oncol Biol Phys 2010;78:449–53.
- Deasy JO, Moiseenko V, Marks L, Chao KSC, Nam J, Eisbruch A. Radiotherapy dose-volume effects on salivary gland function. Int J Radiat Oncol Biol Phys 2010;76: S58–63.
- Bedford JL. Treatment planning for volumetric modulated arc therapy. Med Phys 2009;36:5128.
- Cao D, Afghan MKN, Ye J, Chen F, Shepard DM. A generalized inverse planning tool for volumetric-modulated arc therapy. Phys Med Biol 2009;54:6725–38.
- Cameron C. Sweeping-window arc therapy: An implementation of rotational IMRT with automatic beam-weight calculation. Phys Med Biol 2005;50:4317–36.
- Chi A, Ma P, Fu G, Hobbs G, Welsh JS, Nguyen NP, et al. Critical structure sparing in stereotactic ablative radiotherapy for central lung lesions: helical tomotherapy vs. volumetric modulated arc therapy. PLoS One 2013;8:e59729.
- Guckenberger M, Richter A, Krieger T, Wilbert J, Baier K, Flentje M. Is a single arc sufficient in volumetric-modulated arc therapy (VMAT) for complex-shaped target volumes?Radiother Oncol 2009;93:259–65.
- Vanetti E, Nicolini G, Nord J, Peltola J, Clivio A, Fogliata A, et al. On the role of the optimization algorithm of RapidArc(®) volumetric modulated arc therapy on plan quality and efficiency. Med Phys 2011;38:5844–56.
- Bzdusek K, Friberger H, Eriksson K, Ha rdemark B, Robinson D, Kaus M. Development and evaluation of an efficient approach to volumetric arc therapy planning. Med Phys 2009;36:2328.
- Ong CL, Cuijpers JP, Senan S, Slotman BJ, Verbakel WFAR. Impact of the calculation resolution of AAA for small fields and RapidArc treatment plans. Med Phys 2011;38:4471–9.