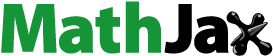
Abstract
4-nonylphenol (4-NP) a persistent and ubiquitous xenoestrogen with endocrine-disrupting activities, is present in varying concentrations in wastewater, ground water, treated drinking water and sediments. It is a biodegradation product of alkylphenolethoxylates used widely as a nonionic surfactant. In the present study, the presence of 4-NP in water samples and its toxicity and tissue accumulation were investigated in an air-breathing catfish Heteropneustes fossilis. The concentration of 4-NP varied between 12.40 ± 1.11 μg/L and 16.29 ± 1.18 μg/L in water samples drawn from river Ganga and river Varuna at 3 different sites that witness high anthropogenic activities or near a city wastewater treatment efflux point. Stored tap water contained 4-NP albeit at a low level (3.9 ± 0.23 μg/L). The median lethal concentrations (LC50) of 4-NP were 1600 μg/L and 1632 μg/L, respectively, in the gonad resting phase and gonad active (preparatory) phase. The concentration of 4-NP added to fish holding water was reduced by 67% over 10 hr of fish introduction suggesting a faster accumulation of the toxicant by fish. In a chronic study spanning 60 days, 4-NP added to the water was accumulated by tissues in a concentration (64 and 160 μg/L) and exposure time (15, 30, 45 and 60 days) – dependent manner. Brain accumulated the highest concentration of 4-NP while muscle showed the lowest uptake. Gill, liver, kidney, ovary and plasma showed intermediate ranges of accumulation. HPLC chromatogram profiles showed that 4-NP was metabolized in all tissues (brain, liver, kidney, gill and ovary) except muscle and plasma. A distinct seasonality of 4-NP was not discerned in this study, probably due to the fact that a steady state level was not reached up to 60 d of exposure. The study concludes that 4-NP is an environmentally relevant toxicant and the catfish has a high tolerance level, which may be due to the fact that 4-NP is metabolized by many tissues during its elimination.
abbreviations
APs | = | alkylphenols |
APEs | = | alkylphenolpolyethoxylates |
BPA | = | bisphenol A |
DDT | = | dichlorodiphenyltrichloroethane |
DWTPs | = | drinking water treatment plants |
HPLC | = | High Performance Liquid Chromatography |
LC50 | = | lethal concentration, 50% |
LC100 | = | lethal concentration, 100% |
NP | = | nonylphenol |
4-NP | = | 4-nonylphenol |
NPEs | = | nonylphenolpolyethoxylates |
OP | = | octylphenol |
OPEs | = | octylphenolpolyethoxylates |
PAHs | = | polyaromatic hydrocarbons |
t-OP | = | tertiary-octylphenol |
Vg | = | vitellogenin |
WWTPs | = | waste water treatment plants |
USEPA | = | United States Environment Protection Agency |
UV | = | ultra violet |
ZRP | = | zonaradiata protein. |
Introduction
Alkylphenolpolyethoxylates (APEs) are non-ionic surfactant, widely used in industries (pulp and paper, textile and petroleum production), agriculture (pesticide formulation) and domestic purposes (house hold cleaning agents, personal care products, etc.).Citation1 Nonylphenol and octylphenolpolyethoxylates (NPEs and OPEs) are the most common APEs, comprising 80% and 20%, respectively, of the global market production.Citation2,3 Most APEs are discharged to the sewer system and make their way into wastewater treatment plants (WWTPs). Under anaerobic conditions such as those found in sewers and WWTPs, the APEs are biodegraded to alkylphenols (APs), nonylphenol (NP) is one such AP, occurring in different isomeric forms, such as 4-NP.Citation4,5 NP is persistent and ubiquitous, detected in surface and ground water, sediments, aquatic organisms, waste water effluents, air and food products.Citation5-7 Unlike well known environmental contaminants such as polychlorinated biphenyls, DDT, and polyaromatic hydrocarbons (PAHs), that act directly,Citation8 NPs are highly toxic degradation products of less toxic NPE, by anaerobic biological activity.Citation6,9,10
In APEs, the alkyl phenol part is fairly non polar and can dissolve water-insoluble materials like grease and the ethoxylate portion is water soluble, helping transfer of materials into the aqueous phase.Citation4 Because of its lipophilic nature, NP is accumulated and persistent in aquatic organisms, and through the food chain, reaches higher animals and humans.
NP toxicity in aquatic organisms such as bacteria, algae, invertebrates and fishes has been documented and 96-hr LC50 values range greatly from 17 to 3000 μg/L for fishes.Citation6 NP causes a number of deleterious effects in fishes such as reduced fertilization, embryonic and larval toxicity, reduced growth and smoltification.Citation11-13 NP is a known endocrine-disrupting chemical, the best manifestation of which is its estrogenic properties, interfering with normal sexual differentiation and reproductive activities in fishes.Citation14-22 A few studies have shown that NP is present in drinking water and the chlorinated derivatives formed during chlorination step in drinking water treatment plants (DWTPs) show a higher level of estrogenic activity than parent compounds.Citation9,23 The presence of NP in drinking water is a major public concern of high human exposure and needs to be addressed in view of the reproductive and developmental toxicities described in animals.Citation9
In India, to our best knowledge, there are no detailed inventory on the usage and environmental contamination of APEs and APs. However in one study the toxic effects of NP and OP on behavior and selected hematological and biochemical parameters were reported in the African catfish Clarias gariepinus previously.Citation24 Recently, we have reported the adverse effects of 4-NP on fertilization, early development and embryo toxicity in Heteropneustes fossilis.Citation13 In the present study, we investigated the prevalence of 4-NP in freshwater bodies of Varanasi city by high performance liquid chromatography (HPLC). Secondly, 96-hr LC50 of 4-NP and bioaccumulation of sublethal doses of 4-NP were determined in different tissues of catfish Heteropneustes fossilis after chronic exposure under a static renewal system in 2 reproductive phases.
Results
4-NP extraction from water samples
4-NP was detected in all the water samples tested except in distilled water. The concentration of 4-NP in the sample collected at Dasashwamedhghat of river Ganges was the highest at 16.29 ± 1.18 μg/L that at Chowkaghat region of river Varuna was 12.40 ± 1.11 μg/L and that at Dhobi ghat region of river Varuna was 15.26 ± 1.26 μg/L. Stored tap water showed a concentration of 3.90 ± 0.23 μg/L 4-NP.
The concentration of 4-NP in the aquarium water holding 10 fish was at 0hr when 4-NP was just added to water was 24.29 ± 0.34 μg/L. After 10 hr of the treatment, the concentration was significantly reduced to 8.23 ± 0.24μg/L; Student's 2-tailed test (P < 0.001). There was no change in the concentration of 4-NP in the aquarium water devoid of any fish after 10 hr and the concentration was similar to the 0hr level.
LC50 value of 4-Nonylphenol
The 96- hr LC50 value of 4-NP during resting phase was 1600 μg/L and did not vary significantly from the value of the preparatory phase fish (1632 μg/L). Therefore, the value of 1600 μg/L was used for calculation of sublethal concentrations at 1/10th and 1/25th in the experimental study.
In the tested tissues and blood plasma, 4-NP was eluted at 23.65 min (), which was authenticated by spiking with the standard. The chromatograms show other peaks as well but could not be identified for lack of standards. A prominent peak that eluted at 27.23 ±0.2 min was found in brain, liver, gill, kidney and ovary. The peak was not detected in muscle and plasma. This peak was more prominent in samples of the preparatory- prespawning experiment.
Figure 1. A map of Varanasi city showing collection spots of water samples. 1-Ganga river (Dhashaswamedh ghat), 2-Varuna river (Chowka ghat), 3-Varuna river (Dhobi ghat).

Figure 2. Representative HPLC chromatograms showing elution profile of: (A) standard (4-NP), (B) sample (Dashaswamedh ghat) and (C) standard + sample. Chromatographic conditions: volume: 20μL, column: C18 reverse phase [Luna (2) 150 X 45 mm i.d, 5μm], mobile phase (A) water and (B) acetonitrile, run time: 40 min, flow rate: 1ml/min, detection: UV 280 nm. In Y-axis, scales are not the same.
![Figure 2. Representative HPLC chromatograms showing elution profile of: (A) standard (4-NP), (B) sample (Dashaswamedh ghat) and (C) standard + sample. Chromatographic conditions: volume: 20μL, column: C18 reverse phase [Luna (2) 150 X 45 mm i.d, 5μm], mobile phase (A) water and (B) acetonitrile, run time: 40 min, flow rate: 1ml/min, detection: UV 280 nm. In Y-axis, scales are not the same.](/cms/asset/d13c0e6b-b3ff-4d9e-9a81-b4cac0d6c41e/kend_a_981442_f0002_b.gif)
Bioaccumulation of 4-NP
The tissues showed differential accumulation of 4-NP. In general, the accumulation was both dose- and duration-dependent (, and and ). A greater accumulation was found in the high dose (160 μg/L 4-NP) groups, as compared to the low dose (64 μg/L 4-NP) groups. Brain recorded the highest accumulation, followed by gill, kidney, liver, ovary, plasma and muscle. In both resting and preparatory seasons, the concentration of 4-NP showed overall significant changes (p < 0 .001, 2-way ANOVA) in all the tissues and blood plasma.
Figure 3. Representative HPLC chromatograms showing 4-NP elution peaks in different water samples. 1-Ganga river at Dhashaswamedh ghat, 2-Varuna river at Chowka ghat, 3-Varuna river at Dhobi ghat, 4-Distilled water, 5-tap water, 6-tap water + standard, 7-4-NP treated water with fish (ohr), 8-4-NP treated water with fish (after 10hr). In Y axis scales are not the same.

Figure 4. Accumulation of 4-NP at low (64 μg/L) and high (160 μg/L) concentrations in different tissues (ng/mg tissue weight) of Heteropneustes fossilis during resting -preparatory phase. Data were expressed as mean ± SEM (n=5) and analyzed by two way ANOVA, followed by Newman-Keuls' test (p<0.05). * No significant difference between the controls at different durations. Groups with the same letter or symbol are not significantly different.

Figure 5. Accumulation of 4-NP at low (64 μg/L) and high (160 μg/L) concentrations in different tissues (ng/mg tissue weight) of Heteropneustes fossilis during preparatory- prespawning phase. Data were expressed as mean ± SEM (n=5) and analyzed by two way ANOVA, followed by Newman-Keuls' test (p<0.05). * No significant difference between the controls at different durations. Groups with the same letter or symbol are not significantly different.

Seasonality in tissue distribution
A season-wise comparison of the data by one-way ANOVA (P < 0.001) and Newman-Kuels’ test (P < 0.05) showed that brain accumulation of 4-NP was significantly high in the preparatory phase as compared to the resting phase at 15-day (F = 26.21) in the low dose group, while it was significantly low at other duration (30-day F = 421.54; 45-day F = 96.91; 60 day F = 12.81). In the high dose group, the concentration was significantly high in the preparatory phase at all duration (15-day F = 1789.77; 30-day F = 96.69; 45-day F = 17.27; 60-day F = 945.98). In liver, the accumulation of 4-NP was not significantly different in both seasons at 15- and 45-day in the low dose groups; however it was significantly higher in the preparatory phase at 30-day (F = 27.73) but lower at 60- day (F = 31.8) exposure. In the high dose groups, the liver accumulation of 4-NP decreased significantly in the preparatory phase at 15-day (F = 13.94), insignificantly increased at 30-day, and was significantly higher at 45- (F = 85) and 60 (F = 37.13) day. In gill, there was no significant change in the concentration of 4-NP at 15- and 60-day low dose exposure between the seasons; however it increased significantly at 30-day (F = 45.12) and decreased significantly at 45-day (F = 33.55) exposure. In the high dose groups, the concentration of 4-NP was significantly high in the preparatory phase at 15-day (F = 15.43) and 30-day (F = 24.86) exposure but decreased significantly at 45-day (F = 7.771) and 60- day (F = 67.33). Muscle showed no significant change in 4-NP accumulation in low dose groups at 15, 30 and 45-day exposure but it decreased significantly in the preparatory phase at 60- day (F = 81.41). For high dose exposure, muscle showed significantly high accumulation during the resting phase at all the duration (15-day F = 5.74; 30-day F = 10.56; 45-day F = 139.41; 60-day F = 41.48). In kidney, in the low dose groups there was a significant increase in 4-NP accumulation in the preparatory phase at 15- (F = 71.58), 30- (F = 82.17) and 45- (F = 190.09) day, and a significant decrease at 60-day (F = 16.42). In the high dose groups, there was no significant change in the accumulation of 4-NP at 15- day but there was a significant increase in the preparatory phase at 30- (F = 83.3), 45- (F = 965.55) and 60- (F = 38.73) day exposure compared to the resting phase. In ovary, in both low and high dose groups there was significantly higher accumulation of 4-NP in the resting phase, at 15- (low dose F = 38.37; high dose F = 30.85) and 30- (low dose F = 72.65; high dose F = 34.303) day exposure while it was significantly high in the preparatory phase at 45- (F = 15.86) and 60- (F = 64.21) day in the low dose groups and at 60- day (F = 82.09) in the high dose group. Plasma showed a significant retention of 4-NP at 30- (F = 8.28), 45- (F = 40.72) and 60- (F = 34.65) day in low dose exposure, and at all duration in the high dose groups, (15-day F = 58.55; 30-day F = 13.71; 45-day F = 52.88; 60-day F = 6.91) in the preparatory phase. In the resting phase, the 4-NP accumulation was significantly high but did not show any duration - dependent changes.
Discussion
This study has reported for the first time the prevalence of relatively a high concentration of 4-NP at 3 collection spots in Varanasi namely Dasashwamedhghat in the Western bank of river Ganges (16.295 ± 1.18 μg/L), Chowkaghat in the Southern bank of Varuna river (12.401 ± 1.106 μg/L) and at Dhobi ghat region (15.262 ± 1.26 μg/L). The collection sites are places of intense human activity/location of sewage treatment plants. At the Dasashwamedhghat, devotees take ritual baths daily and at the Chowkaghat the treated sewage water enters the river. The Dhobi ghat is a major cloth cleaning site by the washermen. These sites encounter a large use of detergents/wastewater, which are potential sources of 4-NP. Since there are no extensive survey of 4-NP in Indian waters, the present study, though limited in scope, is a pointer to the need for such a study. The concentration of NP in surface waters in streams, rivers, lakes, estuaries and oceans, and sediments is closely related with the discharge of effluents from sewage treatment plants near industrialized/urban areas and relatedanthropogenic activities such as storm water discharges and run-offs.Citation31-33 Consequently, the concentration of NP varies greatly in water bodies and sediments.
In the present study, we also found the presence of 4-NP in stored tap water (3.901 ± 0.23 μg/L), which was not detected in distilled water (control). This is slightly higher than the freshwater criterion of maximum concentration of 3 μg/L, according to national water quality criteria developed by the US EPA.Citation34 Compounds like NP, OP and BPA have been detected in drinking water samples, albeit in nanogram or picogram quantities, in surveys conducted in the USA and Germany, the sources being groundwater, wastewater or septage.Citation35-37 A few studies have shown that the NP present in drinking water can be chlorinated during chlorination in drinking water treatment plants (DWTPs), which show a higher level of estrogenic activity than parent compounds.Citation9,23 The source of 4-NP may be from the groundwater itself, storage tanks (cement/plastic tanks, water pipes and metal/plastic taps). Chlorination which is a common water treatment process can aggravate the formation of NP metabolites and its toxicity. The data cautions the need of public awareness of potential hazards of 4-NP contamination and other APs in drinking water. Though the concentration is low, daily intake of water can lead to detrimental effects on humans and animals. The detection of 4-NP in storage/aquarium water (control groups) must also take into account its presence during fish experimentation studies.
Considering bioaccumulation of NP by bacteria, algae, fish and aquatic birds living in and around contaminated waters, the total environmental burden of NP is partitioned between the medium and aquatic organisms.Citation6,38 To study this partitioning effect on total environmental NP level, we checked the concentration of 4-NP in water holding the catfish at 0 hr and 10 hr of the addition of the toxicant. The medium was free from any other organisms or sediments. It was found that the holding water concentration was reduced by about 67% during 10 hr of the introduction of the fish. It is inferred that the reduction was largely due to accumulation by the catfish. Other factors such as volatilization and/or adhesion to glass walls of aquaria, photolysis, UV lightmight not have affected the elimination process, since it was a short term study.Citation5,39,40
For any toxicity study, the determination of LC50 value for the toxicant is the first step. In Heteropneustes fossilis, the calculated 96 hr LC50 was 1600 μg/L in the resting phase and 1632 μg/L in the preparatory phase; however, the values did not vary significantly. A survey of the literature shows that the estimated 96hr LC50 values show great variations in fishes. The LC50 values for medaka varied with developmental stage: 460μg/L for the embryo–larval stage and 1.4 mg/L for adults.Citation41,42 The lowest chronic toxicity value (a no observed–effect concentration) reported for rainbow trout was 6 μg/LCitation43, In bluegill sunfish (Lepomis macrochirus), the estimated 96hr LC50 value was 209 μg/L (Brooke 1993) or 243 μg/LCitation34b. The 96hr LC50 for C. gariepinus (African catfish) exposed to NP was 3.48 mg/L24, which is higher than the value obtained in Heteropneustesfossilis. Summarizing aquatic toxicity results, ServosCitation6 stated that acute toxicity varied with organisms: fish (17–3000 μg/L), invertebrates (20–3000 μg/L) and algae (27–2500 μg/L) and toxicity increased with decreased ethoxylate chain. The wide variation in LC50 values in different fish species may be related to their habits. In fishes, gills are the primary site of water-borne toxicants like NP and are easily prone to damages and the consequent asphyxiation due to low uptake of dissolved oxygen.Citation34 Being air-breathing, Heteropneustes fossilis can live out of water in moist habitat and in derelict waters. The air-breathing mechanism may help the catfish to tolerate high levels of NP and other toxicants, and hence high LC50 values, unlike other non-air-breathing fishes. However, the very high LC50 value in C. gariepinus, another air-breathing fish, suggests either species differences in air-breathing habit or evaluation methodologies. Another factor that can be responsible for a high LC50 value is that being a scale-less and a bottom dwelling-mud strainer, like other catfishes, Heteropneustes fossilis may be adapted to the immediate environment (sediments) that contain higher concentration of 4-NP than surface water. Sediments can be a persistent source of 4-NP for extended periods.Citation32,44-46 In benthic fishes like flounder that buries in sediments, ingestion of food and sediment as well as uptake through skin may be an important route of exposure.Citation47 Carps (bottom dwellers) have higher bioaccumulation factor than bass (surface dwellers).Citation48 The bottom dwelling nature of catfishes may have acquired high tolerance of 4-NP. Mussels, in general, have high LC50 values and are resistant to NP contamination. This has been attributed to the presence of an efficient detoxification system.Citation49 Phenols are hydroxylated and conjugated with glucuronides or sulfates for their excretion in fishes.Citation50 In rainbow trout glucuronidation of 4-NP predominates over other routes of metabolic degradation in liver and isolated hepatocytes.Citation30
In the present study, we measured 4-NP accumulation in different tissues of the catfish under a staticwaterrenewal system in 2 different seasons, representing gonad inactive and gonad active phases. The chromatograms (supplementary file) show a distinct peak of 4-NP and its authenticity was checked by spiking with the standard. Additionally, we found a prominent peak after the 4-NP peak and also some more minor peaks in brain, liver, kidney and ovary. Due to the non-availability of standards, we could not identify them further. The chromatographic results show that 4-NP can be metabolized in all tissues except muscle and blood plasma, and this may be part of the detoxification process. Metabolic conversions, glucuronide conjugation or binding with macromolecules were described in tissues like liver, bile, kidney, gill and blood but in tissues like muscle, ovary and testis these are present as the parent compound.Citation20,30,51 Smith and HillCitation52 who analyzed 4-NP residues in organs of roach (Rutilusrutilus) found that brain, muscle and blood cells retained only 4-NP while bile, liver and intestine/feces contained more than 80% NP residue (residue 1, dibromo-4-nonylphenol, an analog of 4 –NP). Ovary, gills and blood plasma shared more than 50% of the analog with 4-NP, while kidney contained only less than 50%.
In the catfish, the tissue accumulation of 4-NP could be correlated with both concentration and duration of the exposure. The levels of 4-NP did not show any distinct pattern of seasonal differences but fluctuated considerably over 60 d exposure. It suggests that a steady-state level might not have reached up to 60 days, unlike in other species. Lozano et al.Citation48 reported that alkylphenols and alkylphenolethoxylates in water samples and fish tissues had seasonally variable values, higher in early spring colder water and lower in the late autumn warmer waters. The temperature difference may be a likely reason for differences in NP concentration in both water and tissues.
The tissues varied in the accumulation of 4-NP. Plasma showed low levels of 4-NP unlike the tissues and did not show any sign ofmetabolite formation.Brain showed the greatest accumulation among the tested tissues and was significantly higher in the preparatory phase, regardless of the dose and duration. In contrast, muscle showed the lowest accumulation of 4-NP among the tissues tested, and the concentration was higher in the resting phase in high concentration groups. Muscles are known to concentrate NP residues in wild fishes like Gudgeon, Chub flounder and cyprinids.Citation38,53 Coldham et al.Citation30 reported that the muscle tissue retained a substantial amount of parent compound (4-NP), despite rapid metabolism and excretion by the fish. Since muscles form the major part of fish biomass, even the low levels can have great implications to fish and human, as an edible food. Because of the large biomass, the muscle may serve as a depot for storage of 4-NP.
The concentration of 4-NP in tissues like gill, liver, kidney ovary and plasma was in the intermediate range between the brain and muscle. Gills are the primary route of entry of water- borne pollutants,Citation20 permitting both influx (absorption) and outflux (elimination). Therefore, the concentration of 4-NP in the gill may actually reflect the turnover of the contaminant. The concentration was higher in the high dose group at different intervals, barring the 15-day exposure. In cyprinid fish, the gill is an active site of alkylphenol metabolism unlike rainbow trout, and t-OP metabolites were identified.Citation51
Liver is the major site of alkylphenol metabolism in fishes and mammals and liver-biliary excretion is a major route of elimination.Citation20,30,54,55 In rainbow trout (Onchorhynchusmykiss), Uguzet al.Citation55 described time-dependent histopathological changes upon exposure to 220 μg/mL of 4-NP. In the catfish liver the additional major and minor peaks may indicate metabolites which may be glucuronides or sulfates of 4-NP or hydroxylated 4-NPCitation20,30that are excreted through the bile. The concentration of hepatic 4-NP was higher in the high dose groups at longer duration in the preparatory phase. During this phase, the hepatocytes are highly active with vitellogenin (Vg) and zonaradiata protein (ZRP) secretion under estrogen influence. In the catfish, plasma E2 and GtH (LH) level, serum and liver levels of P32-labeled phosphoprotein (Vg) and number of vitellogenic and post vitellogenic follicles are high during this period.Citation56 Being a weak estrogen, 4-NP may stimulate both Vg and ZRP synthesis through binding with estrogen receptors.Citation14,16,46,57 The concentration of 4-NP increased with exposure dose and time in the kidney of the catfish with higher levels in the preparatory fish. The kidney is a major site of 4-NP accumulation and metabolism, and the residues are excreted through urine as glucuronides in Atlantic salmon.Citation20
In the catfish, the ovary accumulated 4-NP which was both concentration and exposure- dependent. However, a distinct seasonal pattern was not observed. Ahel et alCitation37 detected NP (0.09 μg/g dry weight) in roe from Barbusbarbus. Pedersen and HillCitation51 reported extensive metabolism of t-OP in rudd testis and ovary. In rudd, the greatest concentration of t-OP was detected in the testis. Tertiary-OP may inhibit spermatogenesis direcly or indirectly.Citation51 In catfish ovary, 4-NP inhibits gametogenesis and steroidogenesis (unpublished data). The accumulation of APs may be enhanced by the presence of a large amount of lipoproteins (yolk). The yolk protein-bound xenobiotics can be transferred to the developing embryos and can produce teratogenic effects.
In conclusion, 4-NP is an environmentally relevant toxicant and is accumulated by the catfish. Tissues show differential accumulation and metabolism to detoxify the toxicant.
Material and Methods
Chemicals
4-Nonylphenol (99% pure) was purchased from Acros Organics (Geel, Belgium). Other chemicals were of analytical grade and purchased locally. 4-NP was dissolved in acetone and then diluted with water to obtain the required concentration. Laboratory reagents were of analytical and HPLC grade and purchased locally.
Animal collection and maintenance
Mature female catfish Heteropneustes fossilis (30–40 g) were purchased from a local fish market near Varunariver during resting (January) and preparatory (March) phases of the annual reproductive cycle. They were maintained in the laboratory in an aquarium (1m length × 60cm breadth × 60cm height) under normal photoperiod (12.5L: 11.5D) and temperature (18 ± 2ºC) until used for experiments. Approximately 20 fish were kept in each aquarium. The fish were fed goat liver twice daily ad libitum. Water was changed twice daily after feeding and the desired concentration of 4-NP was added. Experiments were conducted according to the guidelines of Banaras Hindu University on use of animals for experimentation.
Determination of 4-NP in water samples
Sample collection
Water samples were collected 5 times from different regions of river Ganges and Varuna in Varanasi in July (July 13, 2012 –July 21, 2012; ). Water samples were collected in 1liter glass containers, 5 samples each from Dasashwamedhghat, Chowkaghat and Dhobi ghat. The samples were processed as mentioned in Jeannotet al.Citation25.Briefly, as soon as the water samples were collected, formaldehyde (1–3%, V/V) was added immediately to prevent changes in composition due to biodegradation and the samples were filtered with Whatman filter paper. Filter cake was washed with 5 ml methanol and the filtrate was added to the filtered water.The samples were then stored in dark at 4°C before extraction and analysis. Water stored in overhead tanks in the animal house of the department and triple distilled water was also processed similarly, as controls.
4-NP extraction from water samples
4-NP was extracted from the water samples as per the protocol of Mann and BoddyCitation26 with minor modifications. Filtered water samples (one liter) were applied individually to C18 cartridges (60 mL, 10 g, Agilent technologies, USA). The cartridges were preconditioned using 60 mL methanol followed by 60 mL Milli-Q water. Water samples were allowed to pass through the cartridges and were washed with 60 mL of water: methanol (70:30) and air dried. Elution of 4-NP was achieved using 10 mL 100% HPLC grade methanol followed by 10 ml methyl acetate. Finally, the solvents were removed by drying under a stream of nitrogen. The residue was re-suspended in 1 mL HPLC grade methanol and then analyzed by HPLC.
High performance liquid chromatography (HPLC)
4-NP was assayed by HPLC according to the protocols of Bokernet al.Citation27 and Fountoulakis et al.Citation28. In brief, a Shimadzu (Kyoto, Japan) HPLC system with 2 pumps (LC-10ATVP), system controller (SCL-10AVP) and a UV detector (SPD-10AVP) with a variable wavelength (190–370 nm) range was used. The system was operated with Shimadzu Class VP series software. The analysis was made with a reversed phase C18 column (150 × 4.5 mm, i.d., 5 μm, Luna, Phenomenex, USA) connected to a guard column filled with the same material and absorbance was taken at 280 nm. The mobile phase was composed of solvent A – water and solvent B – acetonitrile. The run consisted of a 40 min linear gradient from 50% A to 50% B in 3 min to 34% A and 66% B in 17 min, to a final solvent ratio for next 20 min. Flow rate was 1mL/min. 4-NP was eluted at 23.65 ± 0.8 min.
Validation of HPLC determination of 4-Nonylphenol
Validation of the HPLC method was done as follows. Different concentrations of the standard (4-NP) were injected into the column to set up concentration vs. peak area curve. The response was linear with the concentration ranges used (0.16, 0.32, 0.64, 1.29, 1.61, 2.15, 2.6, 3.2, 6.4, 12.9, 19.4 and 25.8 ng/mL). Percentage recovery was calculated from the concentration of the standard injected directly and that measured after extraction. The percentage recovery (n = 10) for 4-NP was 96.89%. Water samples collected from both the river bodies were also co-eluted with known concentrations of the standard and compared with the elution of the standard for identification of the compound. No correction was made for the loss. The minimum detection limit of 4-NP was 0.16 ng/mL. Inter- and intra-assay variations were determined from 12 chromatograms of diluted standards. Inter- and intra-assay variations were 9.8% and 8.7%, respectively.
Determination of LC50 of 4-Nonylphenol in Heteropneustesfossilis
The LC50 of 4-NP was determined according to the method of Dede and Kaglo.Citation29 Over 130 acclimatized adult Heteropneustesfossilis (30–40 g) were used for the study. The test concentrations (10, 20, 40, 80, 160, 320, 640, 1280, 1920 and 2560 μg/L) were chosen on the basis of initial experiments. Parallel control groups were maintained simultaneously. Mortality of fish was recorded for each of the concentration of the toxicant during 96 hr exposure, and used for the estimation of LC50 using the following formula.
Number of fish per group
Tissue distribution of 4-NP in Heteropneustes fossilis
In the short-term study, 10 fish were exposed to 4-NP (25 μg/L). After 10 hr, the clearance of 4-NP was measured in the water samples by HPLC along with 0 hrcontrol (immediately after the addition of the chemical).
During the resting and preparatory phases acclimatized mature female catfish were divided into 3 groups of 40–50 fishes each. One group was kept as control and the other 2 (experimental groups) were exposed to 1/25th (64 μg/L) and 1/10th (160 μg/L) of the LC50 value of 4-NP for 60 d The first experiment was started in the resting phase and terminated in the preparatory phase (January 15 to March 16). The second experiment was started in the preparatory phase and terminated in the prespawning phase (March 15 to May 16). Parallel controls were maintained simultaneously. After 15, 30, 45 and 60 days, blood samples from the NP exposed and control fish were collected from caudal vein in heparinized centrifuge tubes and was centrifuged at 2000 g for 15 min at 4°C to separate the plasma.Tissues (brain, liver, gill, ovary and kidney) were sampled, homogenized in methanol and centrifuged at 3000 g for 15 min. Supernatant was collected and dried under nitrogen gas.Citation30 The samples (n = 5) were reconstituted in 100 μL methanol and 4-NP was assayed by the HPLC method, as described above. Data were expressed as ng /mg tissue weight.
Statistical analysis
Data were expressed as mean ± SEM (n = 5) and analyzed by 2-way ANOVA (P < 0.001), followed by Newman-Kuels’ test (P < 0.05). Seasonal changes in tissue accumulation of 4-NP was analyzed by one-way ANOVA (P < 0.001), followed by Newman-Kuels’ test (P < 0.05). The data of 4-NP uptake by fish in the short term study (10 hr) was analyzed by Student's 2-tailed test (P < 0.001).
Disclosure of Potential Conflicts of Interest
The authors declare that they have no conflict of interest.
Acknowledgments
The HPLC system used in this study was procured under DST-FIST Level-I (Phase I) support to Department of Zoology which is gratefully acknowledged.
Funding
The research work was partially supported by in house grant to KPJ and RC.
References
- Renner R. European bans on surfactant trigger transatlantic debate. Environ SciTechnol 1997; 31:316A-320A; PMID:21650741; http://dx.doi.org/10.1021/es972366q
- Benson WH, Nimrod A C. The estrogenic effects of alkylphenolethoxylates, a literature review submitted to Chemical Manufacturers Association Alkylphenols and Ethoxylates Panel 1994; 1.
- Metcalfe CD. Applications for nonylphenolethoxylate surfactants in Canada. Report on phase II. World Wildlife Fund Canada 1996; 3.
- Snyder SA, Keith TL, Naylor CG, Staples CA, Giesy J. Identification and quantitation method for nonylphenol and lower oligomer nonylphenolethoxylates in fish tissues Environ ToxicolChem 2001;20:1870-3; PMID:11521811; http://dx.doi.org/10.1002/etc.5620200903
- Porter AJ, Hayden NJ. Nonylphenol in the Environment: A Critical Review. In: Vermont University Publication, 2002; 1-37.
- Servos MR. Review of the aquatic toxicity, estrogenic responses and bioaccumulation of alkylphenols and alkylphenolpolyethoxylates Water Qual Res J Canada 1999; 34(1):123-77.
- Saito T, Kato K, Yokogawa Y, Nishida M, Yamashita N. Detoxification of bisphenol A and nonylphenol by purified extracellular laccase from a fungus isolated from soil J BiosciBioeng 2004; 98:64-6; PMID:16233667
- Neilson AH. Organic Chemicals in the Aquatic Environment - Distribution, Fate and Toxicology. Boca Raton, Florida: LewisPublishers/CRC Press; 1994.
- Soares A, Guieysse B, Jefferson B, Cartmell E, Lester JN. Nonylphenol in the environment: a critical review on occurrence, fate, toxicity and treatment in wastewaters. Environ Int 2008; 34:1033-49; PMID:18282600; http://dx.doi.org/10.1016/j.envint.2008.01.004
- Mao Z, Zheng X, Zhang Y, Tao X, Li Y, Wang W. Occurrence and biodegradation of nonylphenol in the environment. Int J Mol Sci 2012; 13:491-505; PMID:22312266; http://dx.doi.org/10.3390/ijms13010491
- Madsen SS, Mathiesen AB, Korsgaard B. Effects of 17-estradiol and 4-nonylphenol on smoltification and vitellogenesis in Atlantic salmon (Salmo salar). Fish PhysiolBiochem 1997; 17:303-12
- Ashfield LA, Pottinger TG, Sumpter JP. Exposure of female juvenile rainbow trout to alkylphenolic compounds results in modifications to growth and ovosomatic index. Environ Toxicol Chem 1998; 17:679-86; http://dx.doi.org/10.1002/etc.5620170423
- Chaube R, Gautam GJ, Joy KP. Teratogenic effects of 4-nonylphenol on early embryonic and larval development of the catfish Heteropneustes fossilis. Arch Environ ContamToxicol 2013; 64:554-61; PMID:23229197; http://dx.doi.org/10.1007/s00244-012-9851-7
- Jobling S, Sumpter JP. Detergent components in sewage effluent are weakly oestrogenic to fish: an in vitro study using rainbow trout (Oncorhynchusmykiss) hepatocytes. AquatToxicol 1993; 27:361-72.
- White R, Jobling S, Hoare SA, Sumpter JP, Parker MG. Environmentally persistent alkylphenolic compounds are estrogenic. Endocrinol 1994; 135:175-82; PMID:8013351
- Sumpter JP, Jobling S. Vitellogenesis as a biomarker for estrogenic contamination of the aquatic environment. Environ Health Perspect 1995; 103(Suppl 7):173-8; PMID:8593867; http://dx.doi.org/10.1289/ehp.95103s7173
- Nimrod AC, Benson WH. Environmental estrogenic effects of alkylphenolethoxylates. Cri Rev Toxicol 1996; 26(3):335-64; PMID:8726166; http://dx.doi.org/10.3109/10408449609012527
- Arukwe A, Knudsen FR, Goksøyr A. Fish zonaradiata (eggshell) protein: a sensitive biomarker for environmental estrogens. Environ Health Perspect 1997; 105:418-22; PMID:9189707; http://dx.doi.org/10.1289/ehp.97105418
- Knudsen FR, Pottinger TG. Interaction of endocrine disrupting chemicals, singly and in combination, with estrogen-, androgen-, and corticosteroid-binding sites in rainbow trout (Oncorhynchus mykiss). Aquat Toxicol 1999; 44:159-70
- Arukwe A, Celius T, Walther BT, Goksøyr A. Effects of xenoestrogen treatment on zonaradiata protein and vitellogenin expression in Atlantic salmon (Salmosalar). Aquat Toxicol 2000; 49:159-70; PMID:10856602
- Arukwe A, Kullman SW, Hinton DE. Differential biomarker gene and protein expressions in nonylphenol and estradiol-17β treated juvenile rainbow trout (Oncorhynchus mykiss) Comp BiochemPhysiol Part C 2001; 129:1-10; PMID:11369296
- Hill RL, JrJanz DM. Developmental estrogenic exposure in zebrafish (Danio rerio). I. Effects on sex ratio and breeding success. AquatToxicol 2003; 63:417-42; PMID:12758006
- Dupuis A, Migeot V, Cariot A, Albouy-Llaty M, Legube B, Rabouan S. Quantification of bisphenol A, 353-nonylphenol and their chlorinated derivatives in drinking water treatment plants. Environ Sci Pollut Res 2012; 19:4193-205; PMID:22648348; http://dx.doi.org/10.1007/s11356-012-0972-3
- Senthilkumaran S, Kavitha C, Ramesh M, Grumt T. Toxicity studies of nonylphenol and octylphenol: hormonal, hematological andbiochemical effects in Clarias gariepinus. J App Toxicol 2011; 31:752-61; PMID:21404308; http://dx.doi.org/10.1002/jat.1629
- Jeannot R, Sabik H, Sauvard E, Dagnac T, Dohrendorf K. Determination of endocrine-disrupting compounds in environmental samples using gas and liquid chromatography with mass spectrometry. J ChromA 2002; 974:143-59; PMID:12458934; http://dx.doi.org/10.1016/S0021-9673(02)01240-2
- Mann RM, Boddy MR. Biodegradation of a nonylphenolethoxylate by the autochthonous microflora in lake water with observations on the influence of light. Chemosphere 2000; 41:1361-9; PMID:11057572; http://dx.doi.org/10.1016/S0045-6535(00)00002-3
- Bokern M, Nimtz M, Harms HH. Metabolites of 4-n-nonylphenol in Wheat Cell Suspension Cultures. J Argic Food Chem 1996; 44:1123-7; http://dx.doi.org/10.1021/jf950376+
- Fountoulakis M, Drillia P, Pakou C, Kampioti A, Stamatelatou K, Lyberatos G. Analysis of nonylphenol and nonylphenolethoxylates in sewage sludge by high performance liquid chromatography following microwave-assisted extraction. J Chrom A 2005; 1089:45-51; PMID:16130770; http://dx.doi.org/10.1016/j.chroma.2005.05.109
- Dede EB, Kaglo HD. Aquatoxicological effects of Water Soluble Fractions (WSF) of diesel fuel on O. niloticusfingerlings. J App SciEnviron Mgt 2001; 5:93-96
- Coldham, NG, Sivapathasundaram, S, Dave, M, Ashfield, LA, Pottinger, TG, Goodall, C, Sauer, MJ. Biotransformation, tissue distribution, and persistence of 4-nonylphenol residues in juvenile rainbow trout (Oncorhynchusmykiss). Drug Metab Dispos 1998; 26:347-354; PMID:9531523
- Ahel M, Giger W & Koch M, Behavior of alkylphenolpolyethoxylate surfactants in the aquatic environment. 1. Occurrence and transformation in sewage-treatment. Water Res 1994; 28:1131-42; http://dx.doi.org/10.1016/0043-1354(94)90200-3
- Hale RC, Smith CL, de Fur PO, Harvey E, Bush EO. Nonylphenols in sediments and effluents associated with diverse wastewater outfalls. Environ Toxicol Chem 2000; 19:946-52; http://dx.doi.org/10.1002/etc.5620190423
- Corsi SR, Zitomer DH, Field JA, Cancilla DA. Nonylphenolethoxylates and other additives in aircraft de-icers, anti-icers, and waters receiving airport runoff. Environ Sci Technol 2003; 37:4031-7; PMID:14524432; http://dx.doi.org/10.1021/es034005i
- Liber K, Gangl JA, Corry TD, Heinis LJ, Stay FS. Lethality and bioaccumulation of 4-nonylphenol in bluegill sunfish in littoral enclosures. Environ Toxicol Chem 1999; 18:394-400; http://dx.doi.org/10.1002/etc.5620180305
- Rudel RA, Melly SJ, Geno PW, Sun G, Brody JG. Identification of alkylphenols and other estrogenic phenolic compounds in wastewater, septage, and groundwater on cape cod, massachusetts. Environ Sci Technol 1998; 32:861-9; http://dx.doi.org/10.1021/es970723r
- Kuch H, Ballschmiter K. Determination of endocrine-disrupting phenolic compounds and estrogens in surface and drinking water by HRGC-(NCI)-MS in the picogram per liter range. Environ Sci Technol 2001; 35:3201-6; PMID:11506003; http://dx.doi.org/10.1021/es010034m
- Petrovic M, Diaz A, Ventura F, Barcelo D. Occurrence and removal of estrogenic short-chain ethoxynonylphenolic compounds and their halogenated derivatives during drinking water production.Environ Sci Technol 2003; 37: 4442-8; PMID:14572098; http://dx.doi.org/10.1021/es034139w
- Ahel M, McEnvoy J, Giger W, Bioaccumulation of the lipophilic metabolites of nonionic surfactants in freshwater organisms. Environ Pollut 1993; 79:243-248; PMID:15091885; http://dx.doi.org/10.1016/0269-7491(93)90096-7
- Weltin D, Gehring M, Tennhardt L, Vogel D, Bilitewski B. The determination ofsteroids and phenolic xenoestrogens in wastewater, lysimeter leachate, soil, and sewage sludge using SPE and GC/MS. Beiträge zu Abfallwirtschaft und Altlasten 2004; 18:35-41; PMID:14978771
- Lietti E, Marin MG, Matozzo V, Polesello S, Valsecchi S. Uptake and elimination of 4 nonylphenol by the clam Tapes philippinarum. Arch Environ Contam Toxicol 2007; 53:571; PMID:17657455; http://dx.doi.org/10.1007/s00244-006-0250-9
- Yoshimura K. Biodegradation and fish toxicity of nonionic surfactants. J Am Oil Chem Soc 1986; 63:1590-6; http://dx.doi.org/10.1007/BF02553093
- Gray MA & Metcalfe CD. Induction of testis-ova in Japanese medaka (Oryziaslatipes) exposed to p-nonylphenol. Environ Toxicol Chem 1997; 16:1082-6; PMID:22230730
- Arslan OC, Parlak H, Oral R, Katalay S. The effects of nonylphenol and octylphenol on embryonic development of sea urchin (Paracentrotuslividus). Arch Environ Contam Toxicol 2007; 3:214-9; PMID:17587143; http://dx.doi.org/10.1007/s00244-006-0042-2
- Blackburn MA, Waldock MJ. Concentrations of alkylphenols in rivers and estuaries in England and Wales. Water Res 1995; 29:1623-9; http://dx.doi.org/10.1016/0043-1354(94)00340-D
- Bennie DT, Sullivan CA, Lee H-B, Peart TE, Maguire RJ. Occurrence of alkylphenols and alkylphenol mono- and diethoxylates in natural waters of the Laurentian Great Lakes basin and the upper St. Lawrence river. Sci Total Environ 1997; 193:263-75; http://dx.doi.org/10.1016/S0048-9697(96)05386-7
- Petrovic M, Sole M, de Alda M JL, Barcelo D. Endocrine disruptors in sewage treatment plants, receiving river waters, and sediments: integration of chemical analysis and biological effects on feral carp. Environ ToxicolChem 2002; 21:2146-56; PMID:12371491; http://dx.doi.org/10.1002/etc.5620211018
- Allen Y, Matthiessen P, Scott AP, Haworth S, Feist S, Thain JE. The extent of oestrogenic contamination in the UK estuarine and marine environments- further surveys of founder. Sci Total Environ 1999; 233:5-20; PMID:10492895; http://dx.doi.org/10.1016/S0048-9697(99)00175-8
- Lozano N, Rice CP, Pagano J, Zintek L, Barber LB, Murphy EW, Nettesheim T, Minarik T, Schoenfuss HL. Concentration of organic contaminants in fish and their biological effects in a wastewater-dominated urban stream. Sci Total Environ 2012; 420:191-201; PMID:22341470; http://dx.doi.org/10.1016/j.scitotenv.2011.12.059
- Quinn B, Gagné F, Blaise C, Costello M J, Wilson J G, Mothersill C. Evaluation of the lethal and sub-lethal toxicity and potential endocrine disrupting effect of nonylphenol on the zebra mussel (Dreissenapolymorpha), Comp Biochem Physiol Part C 2006; 142:118-27; PMID:16377254
- Kasokat T, Nagel R & Urich K. The metabolism of phenol and substituted phenols in zebra fish. Xenobiotica 1987; 17:1215-21; PMID:3424868; http://dx.doi.org/10.3109/00498258709167413
- Pederson R, Hill E. Tissue distribution and depuration of 4-tert-octylphenol residues in the cyprinid fish, Scardiniuserythrophthalmus. Environ Sci Technol 2002; 36:3275-83; PMID:12188353; http://dx.doi.org/10.1021/es010249w
- Smith MD, Hill EM. Uptake and metabolism of technical nonylphenol and its brominated analogues in the roach (Rutilusrutilus). Aquat Toxicol 2004; 69:359-70; PMID:15312719
- Blackburn MA, Kirby SJ, Waldock MJ. Alkylphenolpolyethoxylate residues in the UK aquatic environment. Mar Pollut Bull 1999; 38:109-18; http://dx.doi.org/10.1016/S0025-326X(98)00104-0
- Thibaut R, Debrauwer L, Rao D, Cravedi J-P. Urinary metabolites of 4-n-nonylphenol in rainbow trout (Oncorhynchusmykiss). Sci Total Environ 1999; 233:193-200; PMID:10492905; http://dx.doi.org/10.1016/S0048-9697(99)00225-9
- Uguz C, Iscan I, Ven-Ergu A, Isgor B, Togan I, The bioaccumulation of nonyphenol and its adverse effect on the liver of rainbow trout (Onchorynchus mykiss). Environ Res 2003; 92:262-70; PMID:12804523; http://dx.doi.org/10.1016/S0013-9351(03)00033-1
- Senthilkumaran B, Joy KP. Changes Effects of melatonin, p-chlorophenylalanine and a-methylparatyrosine on plasma gonadotropin level and ovarian activity in the catfish Heteropneustes fossilis: a study correlating changes in hypothalamic monoamines. Fish Physiol Biochem 1995; 14(6): 471-80; PMID:24197643; http://dx.doi.org/10.1007/BF00004347
- Ishibashi H, Hirano M, Matsumura N, Watanabe N, Takao Y, Arizono K, Reproductive effects and bioconcentration of 4-nonylphenol in medaka fish (Oryzias latipes), Chemosphere 2006; 65:1019-26; PMID:16677682; http://dx.doi.org/10.1016/j.chemosphere.2006.03.034