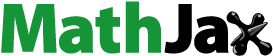
Abstract
Fly ash (FA) deposition originating from power stations and unprotected dumpsites, have been found to affect plant communities, potentially causing environmental degradation and food chain contamination. An analysis of plant composition, heavy metal concentration in plants and soil within 40 kilometers of the Morupule dumpsite was conducted to evaluate the impact of FA disposal. In addition, risks to the ruminants grazing in this area, were assessed. The windward transect had significantly higher species diversity and richness than the leeward transect, especially in areas closer to the dumpsite. This could be attributed to the inability of some plants to tolerate higher concentrations of toxic metal and alkalinity in areas near the dumpsite. In this study, average concentrations in plants of Cr (546.11 mg kg−1), Mn (905.69 mg kg−1), and Cu (128 mg kg−1) were above toxicity levels of 75-100 mg kg−1 for Cr, 400 mg kg−1 for Mn, and 100 mg kg−1 for Cu. Although the quantities of these metals were above the maximum allowable daily consumption, calculations of the daily plant intake by grazing animals in this area revealed a potential danger of exposure to Cr and Cu. Particularly in areas that were nearest to the FA dumpsite, ruminants grazing on the leeward transect were more exposed to Cu than Cr toxicity. Overall, it was found that FA deposition damaged the plant community and put grazing ruminants at greater danger. However, more investigation is required to pinpoint the precise level of hazardous metals found in the grazing animals in this area.
Introduction
Of concern globally is that the coal-fired generation of electricity will impact the environment by continuously producing fly ash as a waste product (Pandey Citation2020; Maiti and Pandey Citation2020; World Coal Association Citation2020). The disposal and management of FA is a major challenge. If not managed properly, FA deposition and dumping will lead to the degradation of adjacent lands and the deterioration of air and water quality. It may also result in the loss of vegetation, which will negatively affect animals and human beings. There are many metals, including Cr, Cd, Pb, Hg, Se, etc., metalloids, radioactive elements, and persistent organic contaminants (POP) found in FA which can be released into the environment depending on the chemical composition of coal and the ignition process (Pandey et al. Citation2020). Conversely, FA also contains various essential elements such as Si, Ca, Mg, Cu, Fe, Mn, Zn, and B which are instrumental for plant growth and survival (Ultra Citation2020; Gajaje et al. Citation2021). Due to its finer particles, FA is easily carried by wind and water, especially if left uncovered at a dumpsite. Airborne FA particles adhere to plant leaves, blocking the plant’s stomata which then decreases their transpiration rate (Gupta et al. Citation2002). FA also impacts plant growth and development by altering soil properties such as bulk density, soil texture, water-retaining capacity, and soil pH as well as by reducing the microbial diversity (Basu et al. Citation2009). In addition, FA contains a high number of soluble salts which cause the desorption of contaminants and allow them to move from the soil into groundwater through leaching (Khan and Umar Citation2019).
Currently, the global discharge of FA totals 1 billion tons per year. Developing countries like India have a total FA discharge of about 270 million tons per year (Yadav et al. Citation2022). In comparison, Botswana, as a developing country, produces about 650 000 tons of FA per year (Taupedi and Ultra Citation2022). In this study, the FA is physically contained in a dumpsite. This FA, which is deposited in uncovered piles, is easily carried from the dumpsite by wind during the dry season (April to August) and by water during the high rainy season (November to March). Because of the fact that the Morupule power station is the only main electricity supplier in Botswana, and it will continue to be in operation for the next 50 years, there is a need to assess the impact of FA on plant diversity. Botswana is a semi-arid country; therefore, the vegetation is already vulnerable to environmental factors and stress, such as drought, climate change, etc. The addition of FA will bring about more extreme factors which will affect plant diversity.
In an ecological region, the succession pattern, dominance, and community structure of plants is favorable for pollution-tolerant plants when they are exposed to varying levels of pollution in a stressed area. In contrast, in a pollution-free area, there is a normal assemblage of plants. Using biodiversity indices, Chowdhury and Maiti (Citation2016) compared the mangrove biodiversity of polluted and non-polluted sites exposed to tannery outflow in India. In the polluted site, they discovered a decrease in diversity and an increase in the dominance of four metal-tolerant species (Cryptocoryne ciliata (Roxb.), Heliotropium curassavicum L., Avicennia officinalis L., altissima (Poir.) Stapf & C.E. Hubb.). Mukhopadhyay et al. (Citation2017) have assessed the biodiversity of 29 species of plants growing in a metal-laden FA lagoon and screened out five species (Calotropis procera (Aiton) W.T. Aiton, Croton bonplandianus Baill., Cyperus rotundus L., Eclipta prostrata (L.) L., and Vernonia cinerea (L). Less.) as the most metal tolerant species due to their high relative density and frequency.
In comparison, phytoremediation of contaminated sites especially from anthropogenic sources such as mine tailings and fly ash dumpsites using naturally growing species, has been highly recommended and vigorously studied (Pandey, Bhattacharya, and Chakraborty Citation2016; Panda, Mandal, and Barik Citation2020; Gajaje et al. Citation2021). Gajaje et al. (Citation2021) indicated the dominant species inside the FA dumpsite of the Morupule power plant in Botswana were Nicotiana glauca Graham, Alternanthera pungens Kunth, Euphorbia inaequilatera Sond. var. inaquilatera, Cynodon dactylon (L.) Pers, and Dactyloctenium aegyptium (L.) Wild. These species are considered to be pioneer species as they can withstand harsh environmental factors such as high alkalinity of FA, high heavy metal content, by the development of an extensive root system (Gajić et al. Citation2018). This indicates that only selective plants would be able to survive inside this area. Other species from the surrounding areas would not be able to grow inside the FA dumpsite due to the higher concentration of heavy metals in the soil or the non-inhabitable properties of FA.
It is hypothesized that the current status of plant diversity, composition, and soil chemical properties are affected by the long term FA deposition from the power plant since 1989 to 2006 when the Morupule power plant had not installed the scrubbers, and from the dumpsite afterwards. Furthermore, the changes in these parameters are also affected by wind direction. To test this hypothesis, we used ANOVA to test the difference between sites according to distance and wind direction. The variability of the concentration of metals in plants and soil will lead to different levels of exposure for grazing animals, and thus the risk of bioaccumulation and exposure. To date, there are no published reports on the impact of Botswana’s Morupule power plant on the plant community and grazing animals. Therefore, assessing the plant diversity in the vicinity of the Morupule FA dumpsite will provide information on the impact of long-term FA deposition on the plant ecosystem and the risk associated with FA deposition on grazing animals. Additionally, information on the potential of several plant species for FA phytoremediation was supplied.
Materials and methods
Description of the study area
The Morupule power station (latitude 24° 30′ and 24° 31′ S and longitude 27° 02′ and 27° 03′ E) is situated 5 km away from Palapye and 30 km away from Serowe (). Morupule power station have been leased Bamangwato tribal land which covers an area of about 476 ha, of which the dumpsite occupies about 98.87 ha. The main land use activity in this area is small scale farming which involves cash crops or rearing of livestock and takes place in the unfenced remaining leased land (377.13 ha). In Botswana, livestock are reared under communal system where land is owned by the communities and stocking rates are not controlled, and there is free range grazing. This system leads to overgrazing as the land is not given time to rest, since there is no proper management.
Palapye is characterized by semi-arid climatic conditions with an average annual rainfall of 396 mm ranging between 129 mm and 600 mm (Akinyemi Citation2017). The land surface is nearly a flat terrain with an average altitude of 883 m above sea level. The international soil types in the study area are, Ferralic/Luvic Arenosols, Ferric/Haplic Lixisols, and Lithic Leptosols (Akinyemi, Tlhalerwa, and Eze Citation2021). Prevailing winds are north-easterly () with an average wind speed of 4 ms−1 which normally ranges between 0.5 ms−1 and 8.7 ms−1 (Zhai et al. Citation2009). The vegetation consists of bush and tree savanna mainly comprised of Terminalia sericea Burch. ex DC and Acacia species (Vachellia nilotica (L.) P.J.H. Hurter & Mabb, Senegalia nigrescens (Oliv.) P.J.H. Hurter, Vachellia tortilis (Forssk.) Galasso & Banfi, Dichrostachys cinerea (L.) Wight & Arn.).
Figure 2. Prevailing wind direction in Mahalapye from 1960 to 2016, representing Palapye area (department of meteorology, Botswana).
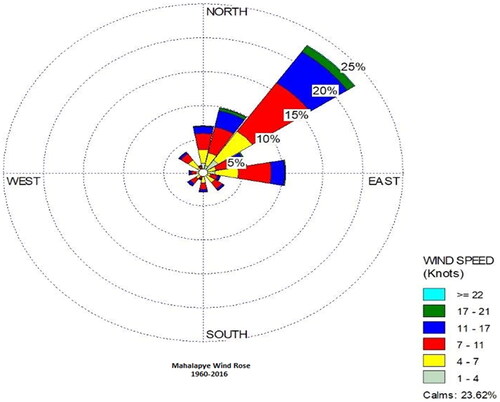
The FA dumpsite has been operating for almost 50 years. The study area is within a 40 km distance from the Morupule power station, in which two transects based on the prevailing wind direction were set up. In southwest, transect 1 is in the wind direction (NE to SW), while transect 2 (SW to NE) is the opposite direction of the FA dumpsite. Transect 1 represents the area that receives a high amount of deposition released from the FA dumpsite due to FA particles that are transported by wind during the dry season, while transect 2 represents the areas that are least affected by the FA carried by wind from the dumpsite.
Soil sampling and plants diversity assessment
The sampling points were established within the two transects emanating from the Morupule power plant, about 40 km in both the leeward (NE to SW) and the windward (SW to NE) wind directions. The orientation of the sampling points was selected with the assumption that the FA deposition would occur in the leeward direction (the southwest transect) and the deposition in transect would differ with distance from the Morupule power plant. In addition, the windward direction (the northeast transect) would serve as the reference sampling points as it had received a lesser amount of FA during the entire operation of the Morupule power plant. In each transect, sampling sites were established at intervals of 0.5 km, 1 km, 2 km, 5 km, 10 km, 20 km, and 40 km. In each sampling site, there were three quadrats (10 m x 10 m) established to gather the vegetation and soil data. Five surface soil samples (0 to 20 cm depth) were collected and mixed to form a composite sample for that particular quadrat. The 100 m2 quadrats were used to study tree species, and then five 1 m2 sub-quadrats were established in the four corners and in the center of the main quadrat for the assessment of the herbaceous, grasses, and shrubs species to measure their density and population. The plant species identification was done in the field with the help of a botanist and verified with several field guidebooks (Vernon Citation1983; Phillips Citation1991; Heath and Heath Citation2009; Oudtshoorn Citation2009). The frequency of these different plant species was recorded to determine species richness (EquationEquation 1(1)
(1) ), diversity (EquationEquation 2
(2)
(2) ), and evenness (EquationEquation 3
(3)
(3) ) using Menhinick’s richness index and the Shannon-Wiener index, respectively. (Davari, Jouri, and Ariapour Citation2011; Kanagaraj et al. Citation2017).
The species richness was calculated based on the Menhinick (Citation1964) formula adopted by Davari, Jouri, and Ariapour (Citation2011).
(1)
(1)
Where d is the species richness, s is the total number of species in the quadrat, and N is the total number of individual species in the quadrat.
(2)
(2)
Where the index (H) is the ratio of the number of species to their importance values within a trophic level or community, i is the total number of individuals in each species, pi (a decimal fraction) is the number of individuals in one species divided by population, and ln is the natural logarithm. The species evenness was determined based on the following EquationEquation 3(3)
(3) (Kanagaraj et al. Citation2017):
(3)
(3)
Where; (EH) was determining evenness whereas (H) was the Shannon-Wiener diversity index and s is the total number of species.
Chemical analysis of plant and soil
From each quadrat, two samples of dominant species of grass, herb, shrub, and tree were taken for chemical analysis. Shoot samples were taken for trees and shrubs while the entire plant was used for grasses and herbs. The plant samples were thoroughly washed with tap water to remove soil from the roots, followed by air drying and then oven drying at 70 °C for 48 hours before grinding them to powdered form. The heavy metal content of tissues was determined by digesting the samples with a nitric acid and hydrogen peroxide mixture (8:2) in a microwave digestion (Milestone ETHOS UP) at 200 °C for 1 hour. Inductively Coupled Plasma Optical Emission Spectroscopy (ICP OES, Thermo Scientific ICAP 7000) was then used to quantify the heavy metals in the samples. All samples were analyzed in replicate, and if the difference in values was greater than 5%, the samples were re-analyzed.
Soil samples were air-dried at room temperature for 24 hours and sieved with a 2.0 mm sieve and stored until they were analyzed. The pH and electrical conductivity (EC) of these samples were measured in a water suspension (1:5) after shaking them for 1 hour. Particle size distribution was determined using Bouyoucos hydrometer method (Bouyoucos Citation1962) with sodium hexametaphosphate as a soil dispersant agent. The soil organic matter was determined using loss of ignition (LOL) method (Ball Citation1964) in a furnace at 550°C and 1000°C. It was computed based on EquationEquation 4(4)
(4) .
(4)
(4)
After the determination of OM content, the calcium carbonate (CaCO3, %) content was derived from EquationEquation 5(5)
(5) .
(5)
(5)
Where a is the mass of crucible, c is the mass of sample – mass of crucible, d is the mass of sample + mass of crucible after 550°C, e is the mass of sample + mass of crucible after 1000°C. The total nitrogen content was determined using the Kjeldahl method, as outlined by Bremner and Mulvaney (Citation1982).
Total heavy metal content was determined after wet acid digestion of approximately 0.2 g of soil with microwave digestion (Milestone ETHOS UP) at 200 °C for 1 hour using an HNO3/HCl/HF mixture (7:1:2). The total Cr, Mn, Cu, Zn, As, and Pb content in FA/soil samples from quadrats was determined using ICP-OES after acid digestion. All the samples were analyzed in duplicate. Chemical analyses were carried out at the Soil Science Laboratory of the Department of Earth and Environment Sciences, BIUST, Palapye, Botswana.
Bioaccumulation factors
The bioaccumulation of heavy metals in various plant species was calculated based on Pandey (Citation2012) by dividing the average concentration of a specific heavy metal in the plant by the average concentration of that heavy metal in the soil (EquationEquation 6(6)
(6) ).
(6)
(6)
Risk assessment
The daily intake of metals by free-range ruminants was estimated based on the amount of biomass consumed and the amount of soil ingested according to EquationEquation 7(7)
(7) proposed by Nouri and Haddioui (Citation2016). According to Mphinyane, Tacheba, and Makore (Citation2015)'s findings, which examined the preferred diet and daily intake of free-range ruminants (goats, cattle, and sheep) in Botswana, different amounts of grasses, herbs, and shrubs/woody plants were consumed by these groups of ruminants and different amounts of these plants varied with their daily intake (). A modification of EquationEquation 6
(6)
(6) was adopted from Manyiwa et al. (Citation2021) in which the daily intake of metals was estimated by incorporating the proportion diet of each ruminant with an average concentration of metal in plants and soil from the study site as indicated in EquationEquation 8
(8)
(8) .
(7)
(7)
(8)
(8)
Table 1. Estimates of parameters used in the calculation of daily dietary intake of grazing ruminants from plants in the vicinity of Morupule power station.
Where:
DI = daily intake of a particular metal.
[Metal]Grass, [Metal]woody species, [Metal]Herbs = the average concentrations (mg kg−1) of metals for grasses, woody plant, and herbs, respectively presented in .
Igrass, Iwoody species, and Iherbs = the equivalent intake of grasses, woody plant, and herbs estimated as the daily dietary intake multiplied by the percentage intake of grasses, shrubs, and herbs for each ruminant.
Isoil = the amount of soil intake by each ruminant. These parameters were adapted from Manyiwa et al. (Citation2021).
Statistical analysis
Using IBM SPSS, data on the plant diversity index and the daily intake of heavy metals by animals feeding on plants near the Morupule power station was analyzed using ANOVA followed by Tukey’s test at a 5% level of significance.
Results
Chemical characterization and metal concentration in FA/soil
displays the physicochemical properties of the soils within the 40 km vicinity of the Morupule FA dumpsite. A significant difference (p < 0.005) was observed for the soil pH, organic carbon, CaCO3 content, and nitrogen content between the soils nearer (0.5 to 2 km) and far away (10 to 40 km) from the dumpsite. In the leeward direction, the pH of the soil, EC, and organic carbon ranged from 5.72 to 8.39, 6 to 4255 µScm−1, and 0.02 to 1.65%, respectively. The highest pH and EC were found at a distance of 0.5 km from the dumpsite, whereas the highest organic carbon was found at a 20 km distance from the dumpsite. In the windward direction, the soil pH, EC, and organic carbon ranged from 4.82 to 7.83, 9 to 2468 µScm−1, 0.01 to 2.62%. Similarly, the EC was highest at a 0.5 km distance, while pH was high at a 2 km distance, and organic carbon was highest at a 40 km distance from the dumpsite. In terms of soil texture, in areas nearer to the dumpsite(0.5 to 1 km) the soil particle size was classified as sandy loam, whereas the areas far away from the dumpsite (2 to 40 km) were classified as sandy clay loam soil. The CaCO3 was higher (2.07 to 10.02%) in areas nearer to the dumpsite and lower (0.52 − 1.91%) in areas far away from the dumpsite. The nitrogen content was low, ranging from 0 to 0.38% in areas nearer to the dumpsite compared to the areas far away from the dumpsite which ranged from 0.41% to 0.61%.
Table 2. Physicochemical properties and metal concentration for soils found in the vicinity of morupule power stationTable Footnote1.
A significant difference (p < 0.005) was also observed in the metal concentrations (Cr, Mn, Cu, Zn, As, and Pb) between the soils found in areas nearer and far from the dumpsite. Manganese was the most abundant metal within the 40 km vicinity of the dumpsite, with an average concentration of 278.14 mg kg−1, followed by Zn (55.56 mg kg−1) > Cr (50.57 mg kg−1) > Cu (30.59 mg kg−1) > Pb (7.36 mg kg−1) > As (3.78 mg kg−1). The areas closer to the dumpsite (0.5 km), in the leeward direction, contained the highest concentration of Cr (129 mg kg−1), Mn (1033 mg kg−1), As (6.8 mg kg−1), and Pb (22 mg kg−1). Similarly, in the windward direction at the same distance from the dumpsite, Cr, Mn, As, and Pb were contained at their highest concentrations of 106 mg kg−1, 897 mg kg−1, 6.9 mg kg−1, 29.47 mg kg−1, respectively. In contrast, in the areas in the leeward direction farther from the dumpsite (20 to 40 km), Cu and Zn were contained at a higher concentration of 24.33 mg kg−1 and 184. 59 mg kg−1, respectively. Similarly, in the windward direction, the highest concentrations of Cu (85.61 mg kg−1) and Zn (48.34 mg kg−1) were found at 10 km and 40 km, respectively.
Diversity index, species richness, and species evenness
There were 1,480 plant samples collected from the various quadrats; of these, 37 were trees of 10 species, 225 were shrubs of 11 species, 594 were herbs of 18 species, and 629 were grasses of 16 species. Among these 22 species were palatable as forage by livestock and wildlife (). The plant species that were found closest to the FA dumpsite (0.5 to 2 km away) were pioneer species. Among these plants, are N. glauca, Eragrostis rigidior Hochst, and D. cinerea, and T. sericea were the most prevalent species. The dominant species found in a region that was 40 km from the FA dumpsite were Dipcadi glaucum (Burch. ex Ker Gawl) Baker, Kyllinga buchananii C.B. Clarke, Diospyros lycioides Desf, Lantana rugosa Thunb, Aristida stipoides Lam, Chloris virgata Sw, Grewia bicolor Juss, Colophospermum mopane (J. Kirk ex Benth.) J. Kirk ex and Vachellia tortilis (Forssk.) Gallaso & Banfi. lists the diversity, evenness, and richness of plant species that can be found within a 40 km vicinity of the Morupule power plant. There was a significant difference in species richness, diversity index, and evenness between species discovered near the dumpsite and 40 kilometers distance. The Menhinick richness in areas closer to the dumpsite, especially in the leeward direction, (NE to SW) had low values ranging from 0.68 to 0.80 compared to the windward direction (SW to NE) which ranged from 0.60 to 0.90. The species richness was high in areas farthest from the dumpsite, in the windward direction, ranging from 1.11 to 1.51 whereas in the leeward direction they ranged from 0.74 to 1.11. The Shannon diversity values were low in areas closer to the dumpsite (1.48 to 1.66 and 1.75 to 1.78) in the windward and leeward directions, respectively. In areas near the dumpsite, the Shannon evenness displayed an uneven pattern throughout the study area, with values ranging from 0.68 to 0.80 and 0.76 to 0.95, in the windward and leeward directions respectively, and in areas farthest from the dumpsite they ranged from 0.82 to 0.84 and 0.69 to 0.75 at the windward and leeward directions, respectively.
Table 3. Palatable plant species from the vicinity of the morupule power station.
Table 4. Diversity index, species richness, and species evenness along the vicinity of morupule power station.
Heavy metal concentration in plant
Urochloa trichopus (Hochst.) Stapf was the grass species with the highest concentrations of Cr (610 mg kg−1), Mn (903 mg kg−1), Zn (184 mg kg−1), As (1.50 mg kg−1), and Pb (1.77 mg kg−1) among those that predominate within 0.5 to 2 kms from the FA dumpsite plant (). Cu was present in E. rigidior at the highest level (189 mg kg−1). The highest concentrations of Cr (640 mg kg−1) and Zn (199 mg kg−1) were found in Eragrostis pallens Hack in areas 20 to 40 km far from the dumpsite. Mn (1097 mg kg−1), As (1.92 mg kg−1), and Pb (2.45 mg kg−1) were the three elements with the highest concentrations in C. virgata. The highest amount of Cu was found in Panicum kalaharense Mez (204 mg kg−1).
Among the herb species () that were prevalent within 0.5 to 2 km of the dumpsite, Heliotropium ciliatum Kaplan had the highest concentration of Cu (88 mg kg−1) and Zn (91 mg kg−1). As and Pb were present in the highest concentrations of 1.65 mg kg−1 and 1.47 mg kg−1, respectively, in Flaveria bidents (L.) Kuntze. With concentrations of 591 mg kg−1 and 891 mg kg−1, respectively, C. ternatum had the highest levels of Cr and Mn. In regions that were 20 to 40 km from the dumpsite, the highest amounts of Cr (598 mg kg−1) and Pb (2.30 mg kg−1) were found in B. lugardii, while Cu’s highest concentration of 267 mg kg−1 was found in D. glaucum. The highest concentration of Mn (1486 mg kg−1) and Zn (215 mg kg−1) were found in V. poskeana.
Figure 3. Heavy metal concentration found in dominant herb species growing within 40 km distance of the fly ash dumpsite. (n = 11; mean ± standard deviation). Critical concentration are Cr =75-100a, Mn = 400a, Cu = 100b, Zn = 200b, as = 10-20a, Pb = 20b mg kg-1; aHajar, Sulaiman, and Sakinah (Citation2014) b Manyiwa et al. (Citation2021).
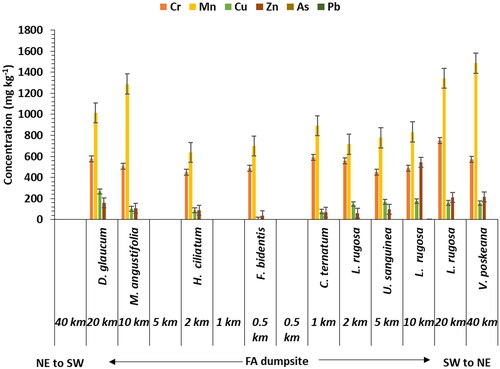
The shrub species B. petersiana had the highest levels of Cr (575 mg kg−1), Mn (1172 mg kg−1), Cu (154 mg kg−1), Zn (189 mg kg−1), and Pb (2.45 mg kg−1) among those that were prevalent within 0.5 to 2 km of the FA dumpsite (). The largest concentration of As was found in N. glauca, at 1.92 mg kg−1. D. cinerea had the highest concentration of Cr and Cu at a distance of 20 to 40 km from the dumpsite, at 585 mg kg−1 and 133 mg kg−1, respectively. V. nilotica had the highest levels of Zn (102 mg kg−1) and Pb (1.66 mg kg−1). The highest Mn (1096 mg kg−1) and As (1.74 mg kg−1) concentrations were found in D. lycioides and G. bicolor, respectively.
Figure 4. Heavy metal concentration found in dominant shrub species growing within 40 km distance of the fly ash dumpsite. (n = 11; mean ± standard deviation). Critical concentration are Cr =75-100a, Mn = 400a, Cu = 100b, Zn = 200b, as = 10-20a, Pb = 20b mg kg-1; aHajar et al. (2014) b Manyiwa et al. (Citation2021).
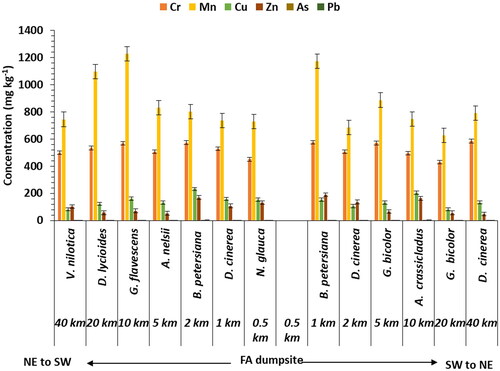
The only tree species discovered between 0.5 and 2 km from the dumpsite were D. cinerea and T. sericea. D. cinerea had the highest concentrations of Cr, Cu, Zn, As, and Pb at 515 mg kg−1, 139 mg kg−1, 84 mg kg−1, 1.22 mg kg−1, and 0.42 mg kg−1, respectively (). The highest concentration of Mn was found in T. sericea (1121 mg kg−1). Among the dominant species discovered 20 to 40 km from the dumpsite, Ximenia americana was the tree that had the highest concentrations of Cr (575 mg kg−1), Mn (911 mg kg−1), As (1.78 mg kg−1), and Pb (3.29 mg kg−1) in its composition. C. mopane had the highest levels of Cu (151 mg kg−1) and Zn (84 mg kg−1).
Figure 5. Heavy metal concentration found in dominant tree species growing within 40 km distance of the fly ash dumpsite. (n = 11; mean ± standard deviation). Critical concentration are Cr =75-100a, Mn = 400a, Cu = 100b, Zn = 200b, as = 10-20a, Pb = 20b mg kg-1; aHajar et al. (2014) b Manyiwa et al. (Citation2021).
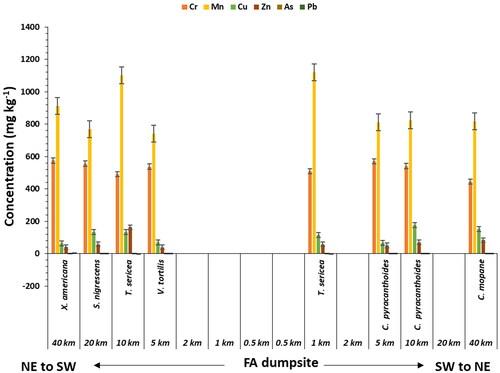
Bioaccumulation factor
For Cr, the bioaccumulation factor (BAF) values ranged from 3.29 to 25.63, for Mn, 0.68 to 16.73, for Cu, 0 to 11.41, for Zn, 0 to 24.83, for As, 0.73 to 0.84, and for Pb, 0.84 to 0.84 (). The BAF of Cr (25.63), Mn (11.83), As (0.71), and Zn (6.71) at a distance of 0.5 to 2 km from the FA dumpsite was highest in E. rigidior. Cu (11.41) and Pb (0.84) BAF values were highest in B. petersiana. At 20 to 40 km from the dumpsite, the highest BAF of Cr and Zn were found in L. rugosa at 21.40 and 8.73, respectively. D. glaucum had the highest BAF of Cu (10.97) and Pb (0.69). The highest BAF values for Mn (9.29) and As (0.52) were obtained from E. pallens and C. virgata, respectively.
Figure 6. Bioaccumulation factor of heavy metals in the dominating grass species growing within 40 km distance of the fly ash dumpsite (n = 11; mean ± standard deviation).
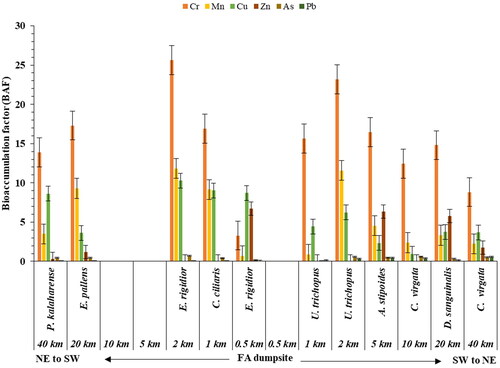
Figure 7. Bioaccumulation factor of heavy metals in the dominating herb species growing within 40 km distance of the fly ash dumpsite (n = 11; mean ± standard deviation).
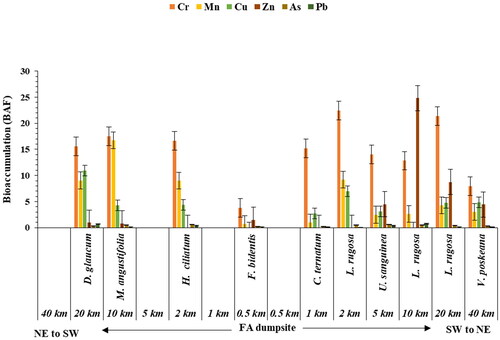
The estimated risk associated with animals
Estimated daily intake of Cu
details how Cu consumption by grazing animals was calculated using average soil metal concentrations and concentrations from various plant groups. Assuming that cattle consume 16.9 kg of feed per day made up of 75% grasses, 23% woody species, and 2% herbaceous species from the Morupule area, the daily intake of Cu by cattle ranged from 400.79 to 3400.18 mg day−1. Cu intake ranged from 73.03 to 169.5 mg day−1 for goats consuming 0.90 kg of feed per day, which was made up of 20% grasses, 78% woody species, and 2% herbs. For sheep, the average daily intake of 2.50 kg of food per day, consisting of 74% grasses, 21% woody species, and 5% herbs, contained Cu concentrations ranging from 55.24 to 495.12 mg day−1.
Table 5. Estimated daily intake of Cu of cattle, goat, and sheep grazing in the vicinity of Morupule power station.
Estimated daily intake of Cr
Within 40 km of the FA dumpsite area, the daily Cr intake ranged from 2029.10 mg day−1 to 11037 mg day−1 assuming cattle consume 16.9 kg day−1 which consists of 75% grasses, 23% woody species, and 2% herbaceous species. The daily Cr intake of goats with a daily feed intake of 0.90 kg day−1, which consisted of 20% grasses, 78% woody species, and 2% herbs, ranged from 366.44 mg day−1 to 514.55 mg day−1. The daily Cr consumption of sheep ranged from 274.05 mg day−15 to 1622.05 mg day−1, based on an average daily forage intake of 2.50 kg day−1, with grasses accounting for 74%, woody species accounting for 21%, and herbs accounting for 5%.
Discussion
The results presented in this paper corroborated the hypothesis that the fly ash long term deposition from the power plant and the dumpsite have altered the soil’s physicochemical properties of soils within the 40 km distance which affected the plant species composition in this area. It was observed in this study that in the leeward direction, especially in areas closest to the dumpsite, the soil pH and EC were significantly higher (8.39 and 4255 µS cm−1) compared to the windward direction where pH was 7.83 and the EC was almost half (2468 µS cm−1) of that found in the leeward direction (). The increase in pH and EC in the sites closer to the dumpsite could be attributed to solubilization of the basic metallic oxides of FA in soil and the alkalinization of H+ by alkali salts as also observed/described by Pani et al. Citation2015. The altered physicochemical properties of soil by FA such as pH, EC, organic matter, and plant-associated bacteria is known to affect the uptake of metals by plant roots (Alloway Citation2013). Additionally, FA's impact on soil microbial characteristics affects the microbial diversity, activity of enzymes, respiration, and soil nitrogen cycling activities, resulting in a nitrogen deficit in the soil (Panda and Biswal, Citation2018). These affect the plant community structure, by having only pioneer and perennial plant species dominant in areas closest to the dumpsite as also observed by Pandey et al. (Citation2015).
This study revealed a notable decrease in woody species in the vicinity of the FA dumpsite. This phenomenon could be attributed to the adverse impact of heavy metals on plant recruitment, leading to a decline of pollution-sensitive species and the invasion of tolerant species in the ecological niches that were left vacant. Similar findings were obtained by Mapaure et al. (Citation2011), who discovered that around the Kombat mine the areas, which were considered polluted near the mine dumps, had a decline in tree and shrub density as heavy metal concentrations negatively influenced vegetation development through reductions in recruitment of plants. In our study it was discovered that species diversity in areas nearer (0.5 to 1 km) to the dumpsite was significantly lower than in the areas at 20 to 40 km distance of the dumpsite. This was attributed to the high soil pH (7.76 to 8.39), sandy loam soil, low organic carbon (0.01 to 0.46%), high CaCO3 content (2.22 to 10.02%), lower N content (0 to 0.38%), high heavy metal content of these soils. Plant species which dominated areas closer to the FA dumpsite were pioneer species such as U. trichopus, D. cinerea, E. rigidior, B. petersiana, L. rugosa, N. glauca, and T. sericea. The dominance of perennial grasses such as U. trichopus and E. rigidior in areas closer to the dumpsite could be due to their characteristics such as their ability to grow in alkaline soil conditions and ability to grow in disturbed soils, (Kgosikoma, Mojeremane, and Harvie Citation2012; Al-Thani and Yasseen Citation2020). E. rigidior is considered to be and indicator of disturbed soil, but it is capable to protect bare soil, and such characteristics could explain its dominance in areas closer to the dumpsite. In terms of shrubs, species like D. cinerea were prevalent in areas that were 2 km away from the dumpsite and lacked available nitrogen. This species does not only have nitrogen-fixing ability, but it is also able to grow in a wide pH range, it is drought resistant, and it has a strong capacity for regeneration (Orwa et al. Citation2009; Mudzengi et al. Citation2013). Another dominant shrub found near the dumpsite was N. glauca, which has an extensive root system, grows rapidly, is drought resistant, and is tolerant to alkaline pH, salinity, and toxic metals (Negin et al. Citation2022).
In terms of species richness, a significant difference (p < 0.005, ) was observed between the species richness in areas nearer and far away from the dumpsite. The many hypotheses used to understand the changes in a plant community structure in response to environmental factors include geomorphological factors, climatic variability, physio-anatomical variations due to changes in abiotic parameters, biotic factors such as herbivory, and animal-plant interactions (Smith and Smith Citation2012; Mukhopadhyay et al. Citation2017). Additionally, an assessment of the plant diversity using the Shannon diversity index revealed that the areas (2 to 5 km) which receive most of the particulate matter from the Morupule power plant and the dumpsite (leeward or southwest) had 0.84x lower species diversity compared to the windward direction. The differences identified could be attributed to changing environmental conditions which include an increased concentration of heavy metals, pH, and salinity in soils. These diversity values were comparable to a study conducted by Akinyemi and Kgomo (Citation2019), where the diversity of the plant community at a 2 km distance from the Morupule power plant was 1.75, and that of a village located 37 km away was 2.27 on the southwest of the Morupule power plant. The Shannon diversity index reflects the environmental quality and adaptation of species to environmental variables, as the sensitive species are gradually eliminated from the habitat with an increase in the magnitude of environmental stress (Datta et al. Citation2010). Species evenness showed an uneven pattern throughout the study area, only the metal-tolerant species such as B. petersiana, D. cinerea, F. bidentis, L. rugosa, N. glauca, and U. trichopus were dominant in areas closer to the dumpsite. As for areas farthest from the dumpsite, the evenness could have been altered by other anthropogenic activities or inherent land characteristics relative to its geological position and hydrology as it was also observed by Akinyemi (Citation2017).
In the case of potentially toxic metals (Cr, Cu, Mn, As, Pb, and Zn) which were accumulated in plants growing in the vicinity of the dumpsite, Cr, Cu, and Mn were above the critical concentrations, 75 – 100 mg kg−1 for Cr, 100 mg kg−1 for Cu, and 400 mg kg−1 for Mn (). Also, these metals had BAF values that were > 1 in C. ciliaris, U. trichopus, E. rigidior, L. rugosa, H. ciliatum, B. petersiana, D. cinerea, N. glauca, and T. sericea among the species found within 0.5 to 2 km from the FA dumpsite. Although these plants accumulated Cr, Cu, and Mn above their critical concentrations, they did not show any physiological damage. Suitable plants for phytoremediation should have the capacity to accumulate heavy metal concentrations greater than the soil, as indicated by the bioaccumulation factor being greater than 1. Based on these criteria, E. rigidior, B. petersiana, U. trichopus, L. rugosa, and D. cinerea could be potential agents for Cr, Cu, and Mn. Some plants are potential phytoremediation agents of single metals such as E. pallens for Pb, U. trichopus for Mn, T. sericea for As, and A. crassicladus for Cu. These plants that can survive in harsh environments and have a BAF greater than one, have dominated the land areas near the dumpsites and can be considered potential candidates for phytoremediation in that area.
Figure 10. Heavy metal concentration found in dominant grass species growing within 40 km distance of the fly ash dumpsite. (n = 11; mean ± standard deviation). Critical concentration are Cr =75-100a, Mn = 400a, Cu = 100b, Zn = 200b, as = 10-20a, Pb = 20b mg kg-1; aHajar et al. (Citation2014) b Manyiwa et al. (Citation2021).
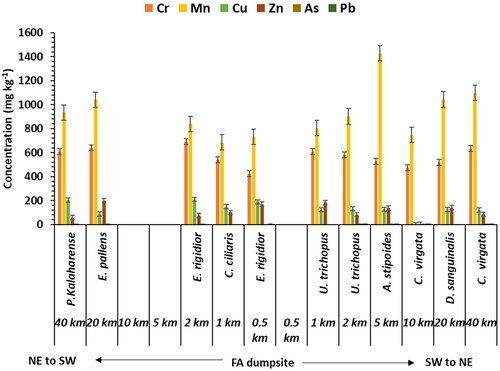
On the other hand, the As, Zn, and Pb which had accumulated in plants, were below their critical concentration. Among these, Zn only had BAF >1 at areas that are 20 to 40 km away from the dumpsite, especially in the opposite wind direction area. The alkaline pH has a negative effect on As, Zn and Pb availability because it increases negatively charged soil colloids and decreases the solubility of these metals. Moreover, the interaction of zinc with other nutrients, including calcium in fly ash, is potentially another reason for the reduced zinc accumulation. Due to their competition for the same absorption sites on the surface of the roots as well as in soil particles, zinc and calcium interact negatively (Moreno-Lora and Delgado Citation2020). According to Manyiwa et al. (Citation2021), the normal concentration of Zn in plants is in the range of 20 - 200 mg kg−1 and the bioaccumulation factor (< 0.1), low Zn availability in the rhizosphere is due to it being easily precipitated as an insoluble sulfate, which reduces absorption and transport to the leaves, making Zn hyperaccumulation extremely uncommon. In this study, only L. rugosa and V. poskeana had Zn concentrations exceeding 200 mg kg−1 and had a BAF value greater than 1 for Zn. Most of the plants in the study area had Zn-BAF > 0.1 which revealed that Zn was not mobile in alkaline conditions especially in areas at 2 km from the dumpsite. The higher Zn bioaccumulation was only observed in areas that were 20 to 40 km from the dumpsite, which indicate that soil pH had a major role in the mobility of Zn in soil.
Most of the species found either in areas closest or farthest from the dumpsite like X. americana, V. nilotica, D. lycioides, A. nigrescens, T. sericea, A. fleckii, D. cinerea, G. bicolor, B. petersiana, G. flavescens, E. pallens, E. rigidior, C. ciliaris, A. stripoides, U. trichopus, C. mopane, and C. virgata, are grazed by livestock (Owen-Smith and Cooper Citation1987; Aganga, Tsopito, and Adogla-Bessa Citation1998; Moleele et al. Citation2001; Tjelele, Dziba, and Pule Citation2012; Dambe et al. Citation2015; Kefelegn and Desta, Citation2021). These plant species accumulated Cr, Cu, and Mn beyond acceptable limits, and the livestock grazing on them could be exposed to metal toxicity. Also due to FA being deposited especially in the southwest of the FA dumpsite in the leeward direction, most species like X. americana, C. mopane, V. tortilis, U. trichopus, and K. buchananii had a low population which could affect the diet of livestock as these species are preferred by livestock. Based on the maximum daily intake of Cu (), grazing cattle could have a daily intake of Cu by more than 1.7x and 1.09x in the leeward and windward direction, respectively. Likewise, cattle grazing in the area will exceed the maximum daily allowable intake of Cr by more than 163x and 151x, in the leeward and windward directions, respectively. The intake of Cu by grazing sheep will exceed the maximum daily intake by more than 2x and 1.3x in the leeward and windward direction, respectively. Similarly, the sheep grazing in the same area, will also exceed the maximum allowable daily intake of Cr by more than 450x and 422x, respectively. As for goat grazing in this area, it will exceed maximum daily intake of Cr by more than 51x and 50x, in the leeward and windward directions, respectively. The livestock which feeds on Cu-contaminated pasture will slowly accumulate a small amount of Cu in the liver which could lead to chronic copper poisoning (Parkinson, Vermunt, and Malmo Citation2010). Once the liver is overloaded, Cu will then be released into the bloodstream which leads to intravascular hemolysis. This is the breakdown of blood cells in the blood vessels which leads to anemia (Johnston, Beasley, and MacPherson Citation2014). When combined with liver injury, this can result in acute toxicosis and recumbency, resulting in death within 24 - 48 hours. This illness causes extreme sadness, thirst, and anorexia in livestock, as well as pale or icteric mucous membranes and hemoglobinuria (Johnston, Beasley, and MacPherson Citation2014; Parkinson, Vermunt, and Malmo Citation2010). Pathological-anatomical alterations in the lungs, kidneys, and liver are common in Cr poisoning in animals (Pechova and Pavlata Citation2007). Hyperemia, erosion, and an inflammatory change in the respiratory system mucosa, occur post-Cr inhalation, affecting the lungs (Lee et al. Citation2002). The elevated levels of Cr and Cu in animal diets contribute to risk to public health since these elements accumulate in the milk and meat. Overall, the areas within 40 km distance of the dumpsite have high risk exposure to grazing cattle and sheep based on the estimated maximum allowable daily intake.
Conclusion
The assessment of the plant diversity using the Shannon diversity index indicated that different plant compositions existed at different distances from the Morupule fly-ash dumpsite. The differences identified could be attributed to changing environmental conditions which include an increased concentration of heavy metals, pH, and salinity in soils. FA deposits affected the plant community structure within a 40 km distance of the dumpsite. The species diversity and richness were lower in areas closer to the dumpsite, and they increase when moving away from the dumpsite. Only pioneer species such as C. ciliaris, U. trichopus, E. rigidior, L. rugosa, H. ciliatum, B. petersiana, D. cinerea, N. glauca, and T. sericea were able to grow within a 0.5 to 2 km distance. Most of these plant species could be considered as having the potential for phytoremediation of Cr, Mn, and Cu due to their higher BAF for Cr, Cu, and Mn. On the other hand, these plants could lead to heavy metal intake by grazing animals. The estimates of the daily intake of Cr and Cu by ruminants from the consumption of plants in the vicinity of the Morupule power station showed that they are exposed to potential risk of metal accumulation. More intensive studies are required to evaluate the actual level of heavy metals in grazing animals. Since FA is continuously produced in large quantities, the study also provided information on potential plant species for phytoremediation of the dumpsites due to their high BAF values for Cu, Cr, Mn, and Zn.
Acknowledgments
Our highest gratitude is given to the Botswana Power Cooperation-Morupule Power Station for allowing access to the study site and Mr. Morati Mpalo for assisting with the sampling map preparation. The Department of Meteorology, Botswana, for providing a wind rose diagram.
Disclosure statement
No potential conflict of interest was reported by the author(s).
Additional information
Funding
References
- Aganga, A. A., C. M. Tsopito, and T. Adogla-Bessa. 1998. Feed potential of Acacia species to ruminants in Botswana. Arch Zootec 47:659–68.
- Akinyemi, F. O. 2017. Climate change and variability in semiarid palapye, eastern Botswana: An assessment from smallholder farmers’ perspective. Weather, Climate, and Society 9 (3):349–65. doi: 10.1175/WCAS-D-16-0040.1.
- Akinyemi, F. O., and M. O. Kgomo. 2019. Vegetation dynamics in African drylands: An assessment based on the Vegetation Degradation Index in an agro-pastoral region of Botswana. Regional Environmental Change 19 (7):2027–39. doi: 10.1007/s10113-019-01541-4.
- Akinyemi, F. O., L. T. Tlhalerwa, and P. N. Eze. 2021. Land degradation assessment in an African dryland context based on the Composite Land Degradation Index and mapping method. Geocarto International 36 (16):1838–54. doi: 10.1080/10106049.2019.1678673.
- Alloway, B. J. 2013. Sources of heavy metals and metalloids in soils. In Heavy Metals in Soils, ed. B. J. Alloway, 11–50. Dordrecht; Springer. doi: 10.1007/978-94-007-4470-7.
- Al-Thani, R. F., and B. T. Yasseen. 2020. Phytoremediation of polluted soils and waters by native Qatari plants: Future perspectives. Environmental Pollution (Barking, Essex: 1987) 259:113694. doi: 10.1016/j.envpol.2019.113694.
- Ball, D. F. 1964. Loss-on-ignition as an estimate of organic matter and organic carbon in non-calcareous soils. Journal of Soil Science 15 (1):84–92. doi: 10.1111/j.1365-2389.1964.tb00247.x.
- Basu, M., M. Pande, P. B. S. Bhadoria, and S. C. Mahapatra. 2009. Potential fly-ash utilization in agriculture: A global review. Prog. Nat. Sci.19 (10):1173–86. doi: 10.1016/j.pnsc.2008.12.006.
- Bouyoucos, G. J. 1962. Hydrometer method improved for making particle size analyses of soils. Agronomy Journal 54 (5):464–5. doi: 10.2134/agronj1962.00021962005400050028x.
- Bremner, J. M., and C. S. Mulvaney. 1982. Nitrogen-Total. In Methods of soil analysis. Part 2. Chemical and microbiological properties, eds. A. L. Page, R. H. Miller, D. R. Keeney, 595–624. Madison, WI: American Society of Agronomy, Soil Science Society of America. doi: 10.2134/agronmonogr9.2.2ed.c31.
- Chowdhury, A., and S. K. Maiti. 2016. Identification of metal tolerant plant species in mangrove ecosystem by using community study and multivariate analysis: A case study from Indian Sunderban. Environmental Earth Sciences 75 (9):1–21. doi: 10.1007/s12665-016-5391-1.
- Dambe, L. M., K. Mogotsi, M. Odubeng, and O. E. Kgosikoma. 2015. Nutritive value of some important indigenous livestock browse species in semi-arid mixed Mopane bushveld, Botswana. Livestock Research for Rural Development 27:209. http://www.lrrd.org/lrrd27/10/mogo27209.htm.
- Datta, S. N., S. K. Chakraborty, A. K. Jaiswar, and G. Ziauddin. 2010. A comparative study on intertidal faunal biodiversity of selected beaches of Mumbai coast. Journal of Environmental Biology 31 (6):981–6.
- Davari, N., M. H. Jouri, and A. Ariapour. 2011. Comparison of measurement of indices of diversity, richness, dominance, and evenness in rangeland ecosystem (Case study; Jvaherdeh-Ramesa). Journal of Rangeland Science 2 (1):389–98.
- Gajaje, K., V. U. Ultra, P. W. David, and G. Rantong. 2021. Rhizosphere properties and heavy metal accumulation of plants growing in the fly ash dumpsite, Morupule power plant, Botswana. Environmental Science and Pollution Research International 28 (16):20637–49. doi: 10.1007/s11356-020-11905-7.
- Gajić, G., L. Djurdjević, O. Kostić, S. Jarić, M. Mitrović, and P. Pavlović. 2018. Ecological potential of plants for phytoremediation and eco-restoration of fly ash deposits and mine wastes. Frontiers in Environmental Science 6:1–24. doi: 10.3389/fenvs.2018.00124.
- Gupta, D. K., U. N. Rai, R. D. Tripathi, and M. Inouhe. 2002. Impacts of fly-ash on soil and plant responses. Journal of Plant Research 115 (6):401–9. doi: 10.1007/s10265-002-0057-3.
- Hajar, E. W. I., A. Z. B. Sulaiman, and A. M. Sakinah. 2014. Assessment of heavy metals tolerance in leaves, stems and flowers of Stevia rebaudiana plant. Procedia Environmental Sciences 20:386–93. doi: 10.1016/j.proenv.2014.03.049.
- Haque, M., K. Biswas, and S. S. Narayan. 2021. Phytoremediation strategies of some plants under heavy metal stress. Plant Stress Physiology. doi: 10.5772/intechopen.94406.
- Heath, A., and R. Heath. 2009. A field guide to the plants of Northern Botswana including the Okavango Delta. Richmond, UK: Henry Ling Ltd.
- Johnston, H., L. Beasley, and N. MacPherson. 2014. Copper toxicity in a New Zealand dairy herd. Irish Veterinary Journal 67:20. doi: 10.1186/2046-0481-67-20.
- Kanagaraj, S., M. Selvaraj, K. R. Das, and G. Munisamy. 2017. Assessment of tree species diversity and its distribution pattern in Pachamalai Reserve Forest, Tamil Nadu. Journal of Sustainable Forestry 36 (1):32–46. doi: 10.1080/10549811.2016.1238768.
- Kefelegn, G. A., and B. Desta. 2021. Ximenia americana: Economic importance, medicinal value, and current status in Ethiopia. The Scientific World Journal 2021:1–7. doi: 10.1155/2021/8880021.
- Kgosikoma, O., W. Mojeremane, and B. A. Harvie. 2012. Pastoralists’ perception and ecological knowledge on savanna ecosystem dynamics in semi-arid Botswana. Ecology and Society 17 (4):27–37. doi: 10.5751/ES-05247-170427.
- Khan, I., and R. Umar. 2019. Environmental risk assessment of coal fly ash on soil and groundwater quality, Aligarh, India. Groundwater for Sustainable Development 8:346–57. doi: 10.1016/j.gsd.2018.12.002.
- Lee, C. R., C. I. Yoo, J. H. Lee, and S. K. Kang. 2002. Nasal septum perforation of welders. Industrial Health 40 (3):286–9. doi: 10.2486/indhealth.40.286.
- Maiti, D., and V. C. Pandey. 2020. Metal remediation potential of naturally occurring plants growing on barren fly ash dumps. Environmental Geochemistry and Health 43 (4):1415–26. doi: 10.1007/s10653-020-00679-z.
- Manyiwa, T., V. U. Ultra, G. Rantong, K. A. Opaletswe, G. Gabankitse, S. B. Taupedi, and K. Gajaje. 2021. Heavy metals in soil, plants, and associated risk on grazing ruminants in the vicinity of Cu–Ni mine in Selebi-Phikwe, Botswana. Environmental Geochemistry and Health 44 (5):1633–48. doi: 10.1007/s10653-021-00918-x.
- Mapaure, I., P. M. Chimwamurombe, B. S. Mapani, and F. A. Kamona. 2011. Impacts of mine dump pollution on plant species diversity, composition and structure of a semiarid savanna in Namibia. African Journal of Range & Forage Science 28 (3):149–54. doi: 10.2989/10220119.2011.647753.
- Menhinick, E. F. 1964. A comparison of some species-individuals diversity indices applied to samples of field insects. Ecology 45 (4):859–61. doi: 10.2307/1934933.
- Moleele, N. M., S. Ringrose, W. Matheson, and C. Vanderpost. 2001. More woody plants? The status of bush encroachment in Botswana’s grazing areas. Journal of Environmental Management 64 (1):3–11. doi: 10.1006/jema.2001.0486.
- Moreno-Lora, A., and A. Delgado. 2020. Factors determining Zn availability and uptake by plants in soils developed under Mediterranean climate. Geoderma 376:114509. doi: 10.1016/j.geoderma.2020.114509.
- Mphinyane, W. N., G. Tacheba, and J. Makore. 2015. Seasonal diet preference of cattle, sheep, and goat grazing on the communal grazing rangeland in the Central District of Botswana. African Journal of Agricultural Research 10 (29):2791–803. doi: 10.5897/AJAR2014.9157.
- Mudzengi, C., S. Shakkie, C. Murungweni, E. Dahwa, X. Poshiwa, and M. Shoko. 2013. Woody species composition and structure in a semi-arid environment invaded by Dichrostachy cinerea (L.) Wight and Arn (Fabaceae). International Journal of Scientific and Research Publications 3:1–10. http://www.pensoft.net/natureconservation.
- Mukhopadhyay, S., V. Rana, A. Kumar, and S. K. Maiti. 2017. Biodiversity variability and metal accumulation strategies in plants spontaneously inhibiting fly ash lagoon, India. Environmental Science and Pollution Research International 24 (29):22990–3005. doi: 10.1007/s11356-017-9930-4.
- Negin, B., S. Hen-Avivi, E. Almekias-Siegl, L. Shachar, G. Jander, and A. Aharoni. 2022. Tree tobacco (Nicotiana glauca) cuticular wax composition is essential for leaf retention during drought, facilitating a speedy recovery following rewatering. The New Phytologist 237 (5):1574–89. doi: 10.1111/nph.18615.
- Nouri, M., and A. Haddioui. 2016. Human and animal health risk assessment of metal contamination in soil and plants from Ait Ammar abandoned iron mine, Morocco. Environmental Monitoring and Assessment 188 (1):6. 12. doi: 10.1007/s10661-015-5012-6.
- Orwa, C., A. Mutua, R. Kindt, R. Jamnadass, and A. Simons. 2009. Agroforestry Database; a tree reference and selection guide version 4.0. http://www.worldagroforestry.org/af/treedb.
- Oudtshoorn, F. V. 2009. Guide to grasses of Southern Africa. In Briza publications, 2nd ed. Pretoria: Briza Publications.
- Owen-Smith, N., and S. M. Cooper. 1987. Palatability of woody plants to browsing ruminants in a South African Savanna. Ecology 68 (2):319–31. doi: 10.2307/1939263.
- Panda, R. B., and T. Biswal. 2018. Impact of fly ash on soil properties and productivity. International Journal of Agriculture, Environment and Biotechnology 11 (2):275–83.
- Panda, D., L. Mandal, and J. Barik. 2020. Phytoremediation potential of naturally growing weed plants grown on fly ash amended soil for restoration of fly ash deposit. International Journal of Phytoremediation 22 (11):1195–203. doi: 10.1080/15226514.2020.1754757.
- Pandey, V. C. 2012. Phytoremediation of heavy metals from fly ash pond by Azolla caroliniana. Ecotoxicology and Environmental Safety 82:8–12. doi: 10.1016/j.ecoenv.2012.05.002.
- Pandey, S. K., T. Bhattacharya, and S. Chakraborty. 2016. Metal phytoremediation potential of naturally growing plants on fly ash dumpsite of Patratu thermal power station, Jharkhand, India. International Journal of Phytoremediation 18 (1):87–93. doi: 10.1080/15226514.2015.1064353.
- Pandey, V. C. 2020. Fly ash properties, multiple uses, threats, and management: An introduction. In Phytomanagement of fly ash, 1–34. Amsterdam: Elsevier. doi: 10.1016/b978-0-12-818544-5.00001-8.
- Pandey, V. C., P. Prakash, O. Bajpai, A. Kumar, and N. Singh. 2015. Phytodiversity on fly ash deposits: Evaluation of naturally colonized species for sustainable phytorestoration. Environmental Science and Pollution Research International 22 (4):2776–87. doi: 10.1007/s11356-014-3517-0.
- Pandey, V. C., A. Rai, L. Singh, and D. P. Singh. 2020. Understanding the role of litter decomposition in restoration of fly ash ecosystem. Bulletin of Environmental Contamination and Toxicology 108 (3):389–95. doi: 10.1007/s00128-020-02994-8.
- Pani, N. K., P. Samal, R. Das, and S. Sahoo. 2015. Effect of fly ash on growth and yield of sunflower (Helianthus annuus L.). Journal of Agronomy and Agricultural Research 7 (2):64–74. http://www.innspub.net/.
- Parkinson, T. J., J. J. Vermunt, and J. Malmo. 2010. Diseases of cattle in Australasia, 1st ed. Wellington: The New Zealand Veterinary Association Foundation for Continuing Education.
- Pechova, A., and L. Pavlata. 2007. Chromium as essential nutrient: A review. Veterinární Medicína 52 (1):1–18. https://www.agriculturejournals.cz/publicFiles/00554.pdf. doi: 10.17221/2010-VETMED.
- Popovic, D., T. Bozic, J. Stevanovic, M. Frontasyeva, D. Todorovic, J. Ajtic, and V. Spasic Jokic. 2010. Concentration of trace elements in blood and feed of homebred animals in Southern Serbia. Environmental Science and Pollution Research International 17 (5):1119–28. doi: 10.1007/s11356-009-0274-6.
- Phillips, M. 1991. A guide to the arable weeds of Botswana. London: Balding + Mansell Limited.
- Smith, T. M., and R. L. Smith. 2012. Elements of ecology, 3rd ed. Pearson Benjamin Cummings.
- Taupedi, S., and V. U. Ultra. 2022. Morupule fly ash as amendments in agricultural soil in central Botswana. Environmental Technology & Innovation 28:102695. doi: 10.1016/j.eti.2022.102695.
- Tjelele, T. J., L. E. Dziba, and H. T. Pule. 2012. Recovery and germination of Dichrostachys cinerea seeds fed to goats (Capra hircus). Rangeland Ecology & Management 65 (1):105–8. doi: 10.2111/REM-D-09-00161.1.
- Ultra, V. U. 2020. Fly ash and compost amendments and mycorrhizal inoculation enhanced the survival and growth of Delonix regia in Cu-Ni mine tailings. Philippine Journal of Science 149:479–89. https://www.researchgate.net/publication/342170684.
- Vernon, R. 1983. Field guide to important arable weeds of Zambia. In Mount Makulu central research station. Chilanga.
- World Coal Association. 2020. https://www.worldcoal.org/coal-facts/.
- Yadav, V. K., A. Gacem, N. Choudhary, A. Rai, P. Kumar, K. K. Yadav, M. Abbas, N. B. Khedher, N. S. Awwad, D. Barik, et al. 2022. Status of coal-based thermal power plants, coal fly ash production, utilization in India and their emerging applications. Minerals 12 (12):1503. doi: 10.3390/min12121503.
- Zhai, M., O. Totolo, M. P. Modisi, R. B. Finkelman, S. M. Kelesitse, and M. Menyatso. 2009. Heavy metal distribution in soils near Palapye, Botswana: An evaluation of the environmental impact of coal mining and combustion on soils in a semi-arid region. Environmental Geochemistry and Health 31 (6):759–77. doi: 10.1007/s10653-009-9260-7.