ABSTRACT
Combining the inherent strengths of school teaching and museum exhibitions on socio-scientific issues could be a complementary approach to prepare students in a holistic and reasonably time-effective way for major future challenges. However, at present it is not known how effective these exhibitions really are, nor to what extent topic interest and school-based knowledge would prove useful for learning in such exhibitions. This study examines these questions in the context of the energy transition from fossil to sustainable energy-sources as a current socio-scientific issue. We investigated how a visit to a modern socio-scientific museum exhibition implementing a multitude of perspectives associated with the energy transition fosters students’ critical energy literacy; and we explored how students’ development of energy literacy was influenced by their prior interest in the topic of the energy transition and conceptual knowledge of energy. Results showed a partial but significant increase in students’ critical energy literacy and that this increase was not related to prior topic interest but was mediated by students’ conceptual knowledge of energy. These findings suggest that modern socio-scientific exhibitions can indeed be a valuable resource for students to develop their critical scientific literacy, building on the conceptual knowledge currently taught in the classroom.
Introduction
A transition from fossil fuels to renewable energy, greater energy efficiency and energy conservation, while considering economic, environmental and social equity (Burke & Stephens, Citation2017; Miller et al., Citation2013), could directly or indirectly address many of the grand challenges of the twenty-first century. However, as such a transition is an open process that needs to be shaped by actors from all sectors of society, weighing up the pros and cons of different solutions, a global energy transition is as complex as it is necessary. The energy transition is also extremely urgent, as we are already living in ‘the decade of delivery for the Sustainable Development Goals’ (World Economic Forum, Citation2020, p. 37) and can be identified as a socio-scientific issue (SSI) that involves ‘social dilemmas with conceptual or technological links to science’ (Sadler, Citation2011, p. 513), where ‘courses of action are underdetermined by scientific evidence’ (Sadler et al., Citation2016, p. 75).
By promoting energy literacy, both formal and non-formal education can help students to become ‘productive citizens’ (Liu & Park, Citation2014, p. 182) who are able to address this crucial SSI and negotiate ‘possible solutions through open, critical dialogue and active participation in democratic mechanisms for effecting change’ (Hodson, Citation2003, pp. 653–654).
However, although the importance of energy literacy and the interdisciplinary approach most likely to foster it are widely recognized in formal education (Chen, Citation2014; Lee & Grapin, Citation2022), which aims to equip students with competencies for learning and decision-making outside of school to meet the existing challenges of the twenty-first century (Reimers Arias & Chung, Citation2016), current school education largely emphasizes the acquisition of conceptual knowledge and mostly revolves around separate domains (OECD, Citation2019). In the case of energy literacy, conceptual energy knowledge is assumed to be a prerequisite for the acquisition of more behavioral and attitudinal aspects of energy literacy (Chen, Citation2014; Kellberg et al., Citation2023; Nordine, Citation2016). Yet to date, there seems to be little to no empirical evidence for that assumption. In other words, we actually know little about ‘in what ways school-based learning substantively transfers to non-school life’ (Bransford et al., Citation2006, p. 216) and how conceptual energy knowledge might be helpful for students to develop energy literacy. Museums on the other hand, are not constrained by the ‘disciplinary boundaries that characterize school subjects’ (Evans & Achiam, Citation2021, p. 9). They recognize their responsibility to prepare their visitors for a more sustainable future (e.g. McGhie, Citation2020; Sutton, Citation2020) and are increasingly implementing SSIs into museums exhibitions (Kollmann et al., Citation2013, Yun et al. Citation2020), particularly critical and agential exhibitions. These novel exhibitions formats aim at responsible citizenship, informed decision-making, and activism (Pedretti & Navas Iannini, Citation2020, p. 60) and incorporate these aspects into their design in a way that might complement school education in promoting students’ critical scientific literacy in a comprehensive and effective way. As both exhibition-forms overlap, this study refers to them collectively as modern SSI exhibitions.
The purpose of this study is to examine how a modern SSI-exhibition might complement formal schooling and connect with student awareness and interest in the energy transition. To do this, we investigated how students’ critical energy literacy changed during a visit to a modern SSI-exhibition and how students’ critical energy literacy after their visit to a modern SSI-exhibition on the energy transition depended on students’ interest in the energy transition and conceptual knowledge of the energy concept as presented in school science instruction.
Defining critical energy literacy
An overarching goal of science education is to help students to become scientifically literate. The interpretation of scientific literacy varies from a focus on the transmission of scientific concepts (Vision I) to stressing the impact of science and technology on society (Vision II) (Roberts, Citation2007) and, more recently, to ‘the role of science as a tool for social change’ (Valladares, Citation2021, p. 558) (Vision III). The last interpretation, which involves greater social engagement and civic impact – aiming at socio-political action (Sjöström & Eilks, Citation2018) and emphasizing ‘knowing-in-action’ (Aikenhead, Citation2006) ultimately corresponds to what Hodson called ‘critical scientific literacy’, that aims at ‘the clarification of problems and negotiation of possible solutions through open, critical dialogue and active participation in democratic mechanisms for effecting change’ (Hodson, Citation2003, pp. 653–654).
Given the characteristics of the energy transition, energy literacy should also aim at such a form of critical evaluation and agency within this SSI. But even though the concept of energy literacy has received increasing attention in the last decades, an ultimate definition remains elusive (Gladwin & Ellis, Citation2023). Fundamental to later considerations were the works of DeWaters and Powers who described an energy literate person as someone with a basic understanding of the concept of energy, that has ideas about the impact that energy production and consumption have on the environment, and that ultimately arrives at energy saving behavior through critical thinking and evaluation. According to their conceptualization, energy literacy spans three domains: the cognitive domain the affective and the behavioral domain (DeWaters et al., Citation2007). However, much of the research on conceptualizing or measuring energy literacy remains in the realm of households and individual responsibility for energy consumption (Adams et al., Citation2022). In terms of the systemic scope of energy transition, though, it is important to note that a genuine transition does not emerge solely as a passive result of reduced (energy) consumption, but rather through ‘the driving force of multiple actors purposefully shaping transitions around a shared long-term vision for the future’ (Farla et al., Citation2012, p. 992). A view of energy literacy that covers this systemic view of energy literacy in terms of a holistic energy transition comes from Lowan-Trudeau and Fowler (Citation2021). They describe ‘critical energy literacy’, as a broad ‘understanding of the social, environmental, political and economic challenges, benefits and impacts of various energy sources, developments and technologies’ (Lowan-Trudeau & Fowler, Citation2021, p. 2) that enables energy literate citizens to judge and act in accordance with the goals of the energy transition goals. This kind of understanding of the pro and cons of the usage of renewable and conventional energy sources can be seen as a basis to enabling students to seek out the best possible solutions to the complex SSI that is the energy transition.
Developing critical energy literacy
Because of its complex mix of knowledge, skills, attitudes, and behaviors, energy literacy needs to be built up over a longer period of time and benefits from learning in different contexts (DoE, Citation2014). Students benefit from these contexts when the contexts present energy-related topics in an interdisciplinary way including scientific, social, technological, cultural or personal perspectives (Liu & Park, Citation2014, pp. 181–182); when the contexts address collective action (Jorgenson et al., Citation2019); and when they allow for independent learning (Wang & Wang, Citation2023).
The SSI movement has long emphasized the importance of such contextualized, topical, and participatory learning environments to ‘help students become better at dealing with complex issues’ and become ‘better prepared to engage in decision-making and position taking’ (Zeidler et al., Citation2019, p. 2) and has shown to increase students’ competency ‘for analysing environmental issues, and evaluating impacts and solutions’ (Kinslow et al., Citation2018, p. 1.).
For this to occur, students have to be supported in developing their scientific knowledge, critical thinking skills, and understanding of the complexities of SSI and ‘need to be encouraged to develop a sense that their viewpoints on the issues matter’ (Bossér, Citation2018, p. 82). Therefore, SSI-learning environments, need to give students the opportunity to explore various perspectives from multiple stakeholders (Zeidler & Newton, Citation2017, p. 57) onto the regarding socio-scientific issue as well as action options (Jorgenson et al., Citation2019) including ‘policies designed to address the issue’ (Presley et al., Citation2013, p. 29). In these learning environments, students have to rely on their own reasoning about the advantages and disadvantages of various alternative solutions when confronted with arguments from multiple perspectives (Knipfler, Citation2009, p. 37) and be allowed to ‘synthesize ideas’ (Herman et al., Citation2017, p. 146) and to ‘integrate what they have learned with their prior knowledge’ (Presley et al., Citation2013, p. 28), i.e. in the form of a debate or role play (Presley et al., Citation2013, p. 28).
However, although the effectiveness of this approach to various aspects of scientific literacy has been demonstrated (for an overview see Zeidler et al., Citation2019) and the desire for a stronger orientation to societal issues in science education (e.g. Lee & Grapin, Citation2022), implementation in formal education still proves difficult (Bossér, Citation2018). After all, this type of teaching requires substantial time (Sadler et al., Citation2007) as well as ‘multidisciplinary expertise’ (Liu & Park, Citation2014, pp. 181–182), yet instructional time is scarce and many teachers are only trained in specific areas of SSIs i.e. the energy transition (Lowan-Trudeau & Fowler, Citation2021, pp. 3 & 6). Therefore, the implementation of Vision II-III scientific literacy in students’ educational environments needs further research and support.
The (new) role of museums: SSI-exhibitions
Given the barriers to formal education, out-of-school interventions can provide new entry points for this research. Informal learning institutions such as museums, are not constrained by the ‘disciplinary boundaries that characterize school subjects’ (Evans & Achiam, Citation2021, p. 9) and have the necessary multidisciplinary expertise to prepare exhibitions that address socio-scientific issues (Pedretti & Navas Iannini, Citation2020).
Traditionally, museums have focused on housing and displaying collections, providing learning- and hands-on experiences (Bell, Citation2008), and designing environments for educational engagement in a broad sense (Pedretti & Navas Iannini, Citation2020, p. 41). In recent times, however, museums have increasingly recognized their responsibility to prepare their visitors for a more sustainable future (McGhie, Citation2018, Citation2020, Sutton, Citation2020; Sutton & Robinson, Citation2020) and have become important sites for fostering critical scientific literacy (Hine & Medvecky, Citation2015). This ‘powerful paradigm shift’ (Koster, Citation1999, p. 287), that already emerged at the end of the last century, lead to an increase in social science themes in science centers and museums (Kollmann et al., Citation2013; Yun et al., Citation2020). However, this trend has only recently translated to the development of SSI-exhibitions that encourage visitors to think critically, provide contextualized information from multiple perspectives, and allow visitors to express their opinions (Yun et al., Citation2020, p. 106). Pedretti and Navas Iannini (Citation2020) label this relatively novel kind of exhibitions ‘critical’ or ‘agential exhibitions’ (p. 61). As their name suggests, critical exhibitions encourage visitors to think critically about potentially controversial science and technology issues in their socio-cultural context. They approach SSIs from a variety of perspectives and invite visitors to active participation within the exhibition. The design of this exhibition type is often emotionally charged, stimulates visitors to question their own position, and emphasis dialogue and the understanding of complexities. Therefore, from an educational perspective this aligns nicely with scientific literacy goals in the Vision II-sense and the before discussed SSI-approach. Almost simultaneously with the continuum from science education to Vision III, which emphasizes the goal of (environmental and political) agency, Pedretti and Navas Iannini identified a new type of exhibition that explicitly pursues the same goals of ‘responsible citizenship, informed decision-making, action and activism’ (Pedretti & Navas Iannini, Citation2020, p. 60). According to the authors, agential exhibitions are, like critical exhibitions, situated at the intersection of Science, Technology, Society and Environment but additionally view their visitors as ‘political agents of change and transformation’ (Pedretti & Navas Iannini, Citation2020, p. 5) and encourage visitors to act on a personal or societal level. This exhibition type often includes opportunities for decision making and other exercises for cognitive and emotional engagement like role-play or voting stations (Pedretti & Navas Iannini, Citation2020, p. 75). Both types of exhibitions overlap and complement each other in addressing complex issues and their relationship to socio-cultural, political, environmental, and ethical considerations and are therefore referred to as modern SSI-exhibitions in this study.
But while these features fit well with descriptions of SSI learning environments and their potential to foster the desired critical scientific literacy, the creation of these exhibitions poses considerable challenges for individual museums as they work out how to deal with the inherent uncertainty, openness, and often object-lessness of SSIs (Yun et al., Citation2020, p. 100). Research into the learning outcomes and useful design features of this relatively new form of exhibition is therefore paramount in supporting interested museums in their efforts to engage with SSIs in a critical and activating way.
Prerequisites for learning (about energy) in museums
Learning in museum exhibitions is aptly described as free-choice learning: visitors are free to decide what they want to engage with, with whom and for how long, and where they are drawn to next. As such, visitors’ free-choice learning is primarily driven by their intrinsic motivations (Falk & Dierking, Citation2000) and highly dependent on their personal characteristics like their prior knowledge and interests (Falk et al., Citation2011). The preconditions for or influences on this kind of learning are naturally many and varied, and their study, as well as the precise characterization of museum learning itself, is an ongoing quest of museum researchers and professionals (Hohenstein & Moussouri, Citation2018). The ‘contextual model for learning’ (CML) describes the experience of a museum visit as an interplay between the personal, the sociocultural, and the physical context (Falk & Dierking, Citation2013, p. 27). But however personal and complex learning in museums is, it still can be assumed that ‘the better the additional concepts and ideas are connected to the visitor’s prior knowledge and previous experiences’ (Bamberger & Tal, Citation2008, p. 4), the more significant the learning experience. SSI-exhibitions deal with very complex questions without easy conclusions and often do so in unconventional forms of presentation, which often require a particularly active engagement. They might therefore not only benefit from a good fit between their content and prior knowledge and interest, but even depend on it, as they might simply overwhelm if the fit is too poor. The ability of an exhibition on the energy transition to promote critical energy literacy among students may thus depend highly on its fit with their prior school knowledge and interests. Therefore, it can also be assumed that students’ learning and acquirement of energy literacy in a modern SSI-exhibition depends on their prior conceptual energy knowledge and their interest in the topic of the energy transition.
Conceptual energy knowledge as a prerequisite for acquiring energy literacy in museums
There is a broad consensus that students need a deep understanding of energy in order to understand and assess the socio-scientific issues around climate change and energy (for an overview see Chen Citation2014). Consequently, energy is anchored as a basic and crosscutting concept in science education (National Research Council, Citation2012; OECD, Citation2019) that includes conceptual knowledge about energy forms and sources, energy transfer and transformation, energy degradation, and energy conservation (e.g. Duit & Neumann, Citation2014). It is assumed that with an understanding of these concepts and with ‘practice applying them to authentic problems related to energy resources, students are well positioned to develop the kind of energy literacy that is critical for tomorrow’s citizens and scientists’ (Chen, Citation2014, p. 137). In this way, conceptual energy knowledge can be seen as a foundational element of critical energy literacy that can be taught in a Vision-I scientific literacy sense but that at the same time also should prepare students for future learning and ultimately the acquirement of a critical energy literacy. But, up to date there seems to be little to no empirical evidence to support the assumption that conceptual energy knowledge is indeed useful for students to develop critical energy literacy. In fact, we know that knowledge per se does not make informed decision-makers (Falk & Dierking, Citation2010) and that the level of knowledge of students in the energy sector is fundamentally a cause for concern (e.g. Herrmann-Abell & DeBoer, Citation2017).
Thus, modern SSI-exhibitions on the energy transition are not only potentially appropriate learning environments for developing critical energy literacy in students but also, because of the free-choice nature of learning in these exhibitions, an excellent testing ground for the hypothesis that conceptual energy knowledge is indeed helpful to students in navigating these complex, multi-perspective exhibitions. This conceptual knowledge connection is particularly valuable because (1) school-based education has a strong focus on conceptual energy knowledge, and (2) unfortunately for educational research, Bell’s observation that ‘research on schools rarely builds on findings from research in informal settings and vice versa’ (Bell, Citation2009, p. 18) still holds true. In the present study, we therefore aim to provide some evidence as to the effectiveness of conceptual energy knowledge for the development of students’ critical energy literacy.
In light of the available evidence, in the present study we hypothesize that students’ conceptual energy knowledge prior to their exhibition visit positively influences gains in critical energy literacy after the exhibition visit (Hypothesis 2.1).
Topic interest as a prerequisite for acquiring energy literacy in museums
For learning to occur and to be effective, students must be motivated to engage with the learning environment and with the content. While school possesses strong external incentives, free-choice learning in museums depends mostly on intrinsic motivation (Falk & Dierking, Citation2000). Although out of school learning environments like museum exhibitions are very well known to promote personal interest and motivation of visitors (see Lewalter et al., Citation2021 for an overview), visitors bring varying levels of individual interest with them when entering any exhibition – and their individual interest arguably influences then how they engage with said exhibition and how they learn within it (Harackiewicz et al., Citation2016).
In fact, interest is known to be heavily intertwined with learning: It enhances attention, concentration, and joy when engaging with a topic and facilitates learning through higher engagement (for an overview see Renninger & Hidi, Citation2016). Specifically, individual interest in a topic is a fairly stable predisposition and momentarily manifests as situational interest in response to the affordances and characteristics of the learning opportunity (Harackiewicz et al., Citation2016).
Hence, we consider students’ individual interest i.e. their interest in the topic of the energy transition as an important motivational prerequisite for their engagement with the learning environment under study and thus their development of critical energy literacy through a modern SSI-exhibition on the energy transition.
Research questions and hypothesis
Stemming from the above summarized theoretical background and the resulting desiderates this study poses the following research questions and hypothesis:
RQ1: How does students’ critical energy literacy – operationalized as students’ arguments for and against the usage of renewable and conventional energy sources – change during a visit to a modern SSI-exhibition on the energy transition?
H1: Students’ critical energy literacy increases after their visit to the exhibition.
RQ2: How does students’ critical energy literacy after their visit to a modern SSI-exhibition on the energy transition depend on students’ prior conceptual energy knowledge and topic interest?
H2.1& 2.2: Students’ prior conceptual energy knowledge and topic interest will positively influence students’ critical energy literacy after the exhibition visit.
Methods
Setting: the energie.wenden exhibition
The modern SSI-exhibition visited by the students in this study was a special exhibition called energie.wenden (Deutsches Museum, from February 2017 to November 2018), on which the first author worked as chief-curator. The exhibition was developed by an interdisciplinary museum team in close consultation with external experts from science, business, industry, and politics, and was specifically aimed at adolescents and young adults to increase their understanding and participation in the energy transition (Newinger et al., Citation2017). At the center of this exhibition was an interactive role-playing game, in which visitors assumed the role of a politician and the mission to shape their own personal energy transition (). As politicians they encountered various stakeholders of the energy transition, who asked them to take energy political actions in their favor and used decision stations that provided them with different political measures illustrating knowledge in action and pro and contra-arguments to each possible solution ( and ). Thematic rooms additionally provided extensive and intentionally balanced background information on both conventional and renewable energies in order for them to make informed decisions (). In the end visitors’ decisions were logged and evaluated and the resulting energy transition persona – was printed directly on playing-cards that visitors had received upon entering the exhibition and used to activate the decision stations as little characterisations that inspired a lot of discussion and comparison among visitors ().
Figure 1. A visitor receiving the mission to design their energy transition. Foto © Daniel Strauch & Deutsches Museum.
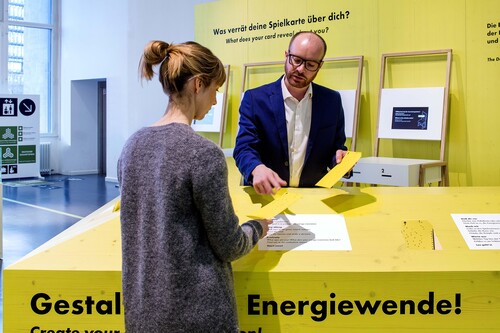
Figure 2. The stakeholders of the energy transition were depicted by actors on screens that activated when visitors passed by. Foto © Deutsches Museum.
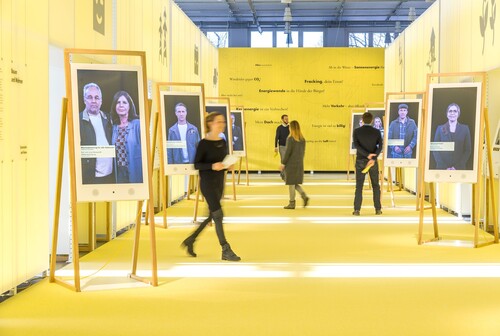
Figure 3. Decision stations provided visitors with pro and contra arguments to various solutions. Foto © Daniel Strauch & Deutsches Museum.
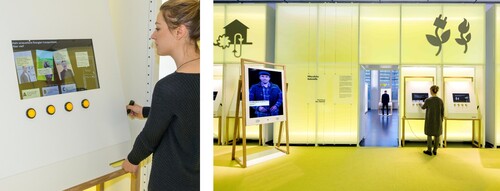
Figure 4. One of ten thematic rooms that provided extensive and balanced background information on the various sub-topics of the energy transition. Foto © Deutsches Museum.
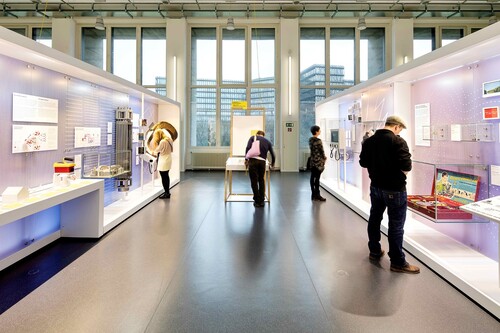
Figure 5. The playing-cards after the energy persona assessment. Foto © Daniel Strauch & Deutsches Museum.
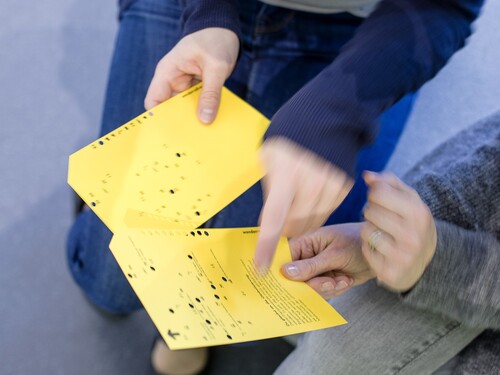
Overall the exhibition encouraged their visitors to engage critically with the contextualized topic of energy transition, presented multi-actor perspectives and action options (Jorgenson et al., Citation2019), facilitated independent learning (Wang & Wang, Citation2023) and treated their visitors as agents of change by putting the in charge during their exhibition visit. The exhibitions design thereby provided visitors with opportunities for perspective taking, socio-scientific reasoning and decision making (Presley et al., Citation2013; Sadler et al., Citation2016; Zeidler et al., Citation2019), possibilities to compare their own position with those of other actors in the energy transition and gain a sense that their own viewpoints on the issue matter (Bossér, Citation2018, p. 82) and therefore qualifies as a SSI-learning environments (Presley et al., Citation2013; Zeidler et al., Citation2019) as well as a modern SSI-exhibition with strong agential design features (Pedretti & Navas Iannini, Citation2020) (For a more detailed description of the exhibition see Kellberg & Newinger, Citation2018; Newinger et al., Citation2017 & Supplementary 1 of this paper).
Study design
The study was designed as a pre–post study and conducted between September and November 2017. Students were given a pen and pencil-test one week before (T1) and one week after (T2) their visit to exhibition. Testing took place at school and was consented to by a legal guardian of the students. In order to minimize the priming effect, the pretest was carried out one week before the exhibition visit. All tests were conducted, explained, and supervised by the first author. Students received a brief introduction to the exhibition before their 90-minute exhibition-visit, were explicitly told that they were free to use the exhibition as they saw fit and that they could decide for themselves whether or not to participate in the exhibition interactive format. Students then visited the exhibition in the intended free-choice form and chose to do so in pairs, small groups or alone. The respective formations could change during their visit and teachers had no influence on how their students interacted with the exhibition.
Sample
The study was conducted in southern Germany. 10 classes from five secondary schools, from grades eight to ten, took part in this study, a total of N = 222 students. For the analyses of this study, only students who attended the exhibition-visit and had data from the pre-and post-test were considered. The final data set consisted of 185 students 166 from 8th grade Gymnasium, the highest-performing track in the German school system, and 19 from 10th grade Realschule, the middle-performing track; ages 12–17 years, M (SD) = 13.58, (1.06), 42.2% female, 57.3% male, 0.5% gender neutral.
Instruments
Critical energy literacy: generation of arguments regarding conventional and renewable energies
Critical energy literacy includes a differentiated and elaborate understanding ‘of the social, environmental, political and economic challenges, benefits and impacts of various energy sources, developments and technologies’ (Lowan-Trudeau & Fowler, Citation2021, p. 2). This kind of knowledge was captured in one open-ended item each for renewable and conventional energy sources that required students to generate arguments pro and contra these energy sources (after Knipfler, Citation2009; Toplak & Stanovich, Citation2003) (see Supplementary 2). These arguments in the sense of ‘reasons’ do provide learners with an initial overview and can be used to measure a level of understanding (Böttcher et al., Citation2016).
Specifically, students were asked to write down any advantages and disadvantages of the usage of renewable and conventional energy sources that came to their minds. For each listed argument, they were asked to add a ‘+’ or ‘−’ to make clear which aspect was to be interpreted as a pro- or contra-argument. From the answers to these two items we derived three variables for each energy source: the overall number of arguments; the number of pro arguments; and the number of contra arguments. These variables allowed us to track quantitative changes in students’ generation of arguments from before to after the exhibition visit.
To capture qualitative changes in students’ arguments, students’ responses were also analyzed on a content-level by means of qualitative content analysis (Mayring, Citation2000). For this purpose and in several iterations, two raters inductively extracted categories from a subset of 30% of the given arguments, applied them to half of the data, and refined the categories. The final categories (see Supplementary 3) were then applied to all responses by two raters. The achieved inter rater-reliability of Cohen’s κ = .81 was very good. Where ratings differed, responses were assigned to their respective categories in a joint discussion between the raters.
Interest in the energy transition
Topic interest was measured as students’ interest in the energy transition. We used four items based on a widely used conceptualization which includes positive emotional experience during the interest action, individual value attribution to the object of interest, and epistemic orientation (Krapp, Citation2002); items were adapted in their wording to the topic of energy transition. Students were asked how much they agreed (five-point Likert scale from ‘not at all’ to ‘very’) with statements like: ‘For me, dealing with the topic of the energy transition is personally significant’ or ‘I like to acquire new knowledge on the subject of the energy transition’. Reliability was very good (Cronbach’s alpha = .84).
Conceptual energy knowledge
To gain insight in students’ prior conceptual energy knowledge as acquired in formal science education at school, a single choice-test was included in the pre-test. The test is an established test for secondary students’ conceptual energy knowledge (Energy Concept Assessment, ECA by Neumann et al., Citation2013) and consists of four key ideas about energy: energy forms and sources, energy transfer and transformation, energy degradation, and energy conservation (e.g. Duit & Neumann, Citation2014). The teaching and learning of these key ideas were included in the curricula for the classes included in this study’s sample.Footnote1 Twenty four items were selected to balance between content in relation to the energy transition, representation of the four key ideas, and levels of difficulty. Every item consists of a question and four possible answers for students to choose from, including only one correct answer. Items were coded as 0 for wrong and as 1 for correct answers; an overall score was calculated that represents the percentage of correct answers.Footnote2
Statistical data analysis
Since the t-test is highly sensitive towards a violation of the assumption of normal distribution, and because the outcome variables were not normally distributed, we chose to conduct two sets of Wilcoxon signed-rank tests to investigate our first research question on the changes in students’ energy literacy from before to after their visit to the exhibition. This non-parametric test is used to determine whether the central tendencies of two dependent samples are different by comparing rank orders at two time points.
To answer our second research question regarding the effect of students’ conceptual energy knowledge and their topic interest on their energy literacy after the exhibition visit, we conducted multiple linear regressions. In addition to the independent variables of conceptual knowledge of energy and interest in the topic of the energy transition students’ prior levels of energy literacy (i.e. the number of arguments) served as covariates in the respective regression model.
Because we were further interested in whether a certain level of conceptual energy knowledge and topic interest is necessary for students’ gain in energy literacy after visiting the exhibition, we also conducted Necessary Condition Analysis (NCA) on those outcomes that were shown to be affected by our independent variables. NCA is a relatively new analytic tool which can be used to identify any ‘necessary determinant [that] must be present for achieving an outcome’ (Dul, Citation2016, p. 1) – without however making high levels of that determinant being a guarantee for achieving that outcome (Tynan et al., Citation2020, p. 1). In addition to the regression, which shows the average influence of a variable (i.e. draws a diagonal line through the center of the data), NCA draws a ceiling line on top of the data, separating areas with observations from areas without observations (Tynan et al., Citation2020, p. 7). Thereby NCA identifies which value of x is needed to achieve a certain level of y, thus identifying potential thresholds of a variable that must be present for, say, a certain amount of energy literacy after an exhibition visit to occur. The accuracy (described by the number of observations at or below the ceiling line divided by the total number of observations multiplied by 100%) of this line should be above 95%. The empty space above the ceiling line defines the effect (via common d-value standards) of said necessity, which is then tested for statistical significance (Dul, Citation2016). The ceiling line can be drawn in two different ways, which are typically used with different kind of data (Tynan et al., Citation2020). We used the ceiling envelopment-free disposal hull (CE-FDH) a step-function ceiling line as a nonparametric technique (Dul, Citation2016, p. 28). Such a ceiling line (dotted line), as well as the empty space above can be seen in and depicting our data analysis. Furthermore, bottlenecks show what level of which determent variable is required for what level of the outcome variable. Bottlenecks regarding our data are included in and as well.
Results
Descriptives
summarizes the descriptive statistics of all study variables including number of arguments as well as arguments pro and contra renewable and conventional energies before (T1) and after (T2) students’ visit to the exhibition. Students’ conceptual energy knowledge varied between a score of zero (all items solved incorrectly) and 0.96 (96% of items solved correctly). Also, students’ prior interest as well as arguments at T1 and T2 varied considerably with students showing moderate interest in the energy transition on average (M = 3.34). Descriptively, the number of arguments regarding renewable energies is larger after (average number of arguments M = 3.28) than before (M = 3.23) the museum visit, possibly due to the increase in contra-arguments for renewable energies (T1: M = 1.00, T2: M = 1.35). For conventional energies, we also saw a descriptive rise in the number of arguments (T1: M = 2.68, T2: M = 3.05), which could be based on an increase in both pro- (T1: M = 0.66, T2: M = 0.85) and contra-arguments (T1: M = 1.70, T2: M = 1.96).
Table 1. Descriptive statistics for study variables.
Changes in students’ energy literacy after their exhibition visit
To test the extent to which number of renewable and conventional energy-arguments changed from before to after the exhibition visit, we conducted two sets of Wilcoxon signed-rank tests ( and ). For students’ renewable energies-arguments (), the total number of renewable energies-arguments was not statistically different after the exhibition visit compared to before. However, while the increase in pro-arguments was not statistically significant, students’ arguments contra renewable energies-arguments increased significantly (z = −3.516, p = <.001, r = .27). As revealed by our qualitative analysis of arguments content, students less often mentioned general aspects of environmental protection (e.g. ‘are good for the environment’) after visiting the exhibition, but more often considered more specific aspects of the usage of resources (e.g. rare earths or land regarding renewable energies) (see Supplementary 4). Therefore, students generated significantly more aspects of and more specific ideas about renewable energies that they consider disadvantageous or problematic after their exhibition visit.
Table 2. Results of Wilcoxon signed-rank test for changes in student’s renewable energies-arguments before (T1) and after (T2) their exhibition-visit.
Table 3. Results of Wilcoxon signed-rank test for changes in student’s conventional energies-arguments before (T1) and after (T2) their exhibition-visit.
For students’ arguments regarding conventional energies, the results () show that the total number of conventional energies-arguments was significantly higher after the exhibition than before (z = −2.834, adj. p = .007, r = .21). This increase could be tied to a significant increase in both pro- and contra-arguments regarding conventional energies. As to arguments content, students significantly more often brought up climate change and aspects describing current costs that are often perceived as lower as their alternatives in the momentary energy supply system after their exhibition visit (see Supplementary 5). Thus, students were able to generate significantly more arguments regarding conventional energies and increasingly saw both the benefits and the challenges of these energy sources in our current energy supply system.
Influences of students’ prior conceptual energy knowledge and topic interest
To answer our second research question regarding the prerequisites for developing energy literacy in an agential exhibition, we first conducted multiple stepwise linear regressions for students’ number of renewable and conventional energies-arguments at T2, predicted by their interest in the energy transition and conceptual energy knowledge and controlling for prior levels of arguments (T1) (see and ).
Table 4. Results of multiple linear regressions testing the influence of students’ prior renewable energies-arguments (T1), conceptual energy knowledge and students interest in the energy transition on student’s renewable energies-arguments after their exhibition-visit (T2).
Table 5. Results of multiple linear regressions testing the influence of students’ prior conventional energies-arguments (T1), conceptual energy knowledge and students interest in the energy transition on student’s conventional energies-arguments after their exhibition-visit (T2).
For students’ arguments regarding renewable energies (), there were strong effects of prior number of arguments on post arguments (total number: β = .56, pro, β = .43, contra, β = .41), explaining about a fifth to a third of the T2 variance, respectively. Above and beyond these effects, only students’ conceptual energy knowledge contributed significantly to total number of arguments after the visit (β = .16, p = .03). Taking a closer look at pro- and contra-arguments, there was no corresponding effect of conceptual knowledge on T2 pro-arguments (β = .09, p = .19), but students’ conceptual knowledge significantly predicted number of contra-arguments after the visit (β = .20, p = .007) when controlling for prior number of contra arguments.
Regarding students’ arguments regarding conventional energies, shows that students’ number of arguments prior to their exhibition visit (T1) had a strong effect (β = .62, p < .001) on arguments after the visit. Correspondingly, there were similar effects for pro (β = .46, p < .001) and contra-arguments (β = .53, p < .001). Overall, prior number of arguments explained about a quarter of the variance in number of post arguments. When adding students’ topic interest and conceptual knowledge to the equation and controlling for prior levels in arguments, again only their conceptual energy knowledge remained as a significant contributor. Specifically, and while there was no effect of conceptual knowledge on overall number of arguments (β = .10, p = .14) and contra-arguments (β = .05, p = .50), there was a unique effect of conceptual knowledge on pro-arguments for conventional energy sources (β = .24, p < .001).
In sum, our results show that students’ conceptual energy knowledge as acquired in school, but not their topic interest, contributed to their gains in arguments regarding energy sources. Further, we observed a pattern in that conceptual energy knowledge seemed particularly helpful in gains for contra-arguments regarding renewable and pro-arguments regarding conventional energies.
For these, we calculated two NCAs: one for contra-arguments regarding renewable energies and one for pro-arguments regarding conventional. As independent variables for the NCA, we included the respective T1 values, interest and conceptual energy knowledge in the analysis. shows the results of both NCAs. The accuracy of all CE-FDH was 100%. With respect to students’ arguments contra renewable energies at T2, we could not identify conceptual energy knowledge nor topic interest as a necessary condition. Instead, an above average level of contra arguments regarding renewable energies at T1 proved to be a necessary condition for a high T2 value. Meaning, that students had to be able to name a certain prior number of arguments contra renewable energies (p = <.001, d = .120) to be able to come up with a certain outcome number of arguments contra renewable energies after their exhibition visit. What that number was can be gleaned from depicting the bottleneck analysis. It shows that for an amount of up to 80% of the maximum number of contra renewable energies-arguments (T2), no specific prior level of contra renewable energies-arguments (T1) was necessary. But in order to know more than 90% of the outcome maximum number of contra renewable energies-arguments (=9.9 arguments), students had to be able to name 60% of the maximum number of contra renewable energies-arguments prior to the exhibition (=3 arguments).
Table 6. Results of necessary conditions analysis.
However, with respect to students’ pro conventional energies-arguments (T2), conceptual energy knowledge proved to be a necessary condition (p = .016, d = 0.205). shows that students had to visit the exhibition with 18.2% of the maximum value of conceptual energy knowledge equaling a score of 0.175 at T1 to generate any pro conventional energies-arguments at all afterwards. To be able to generate more than 60% of pro conventional energies-arguments T2 (=2.4 arguments), 22.7% of the maximum value of conceptual energy knowledge equaling a score of 0.218 at T1 was necessary. This means that students had to answer approximately 1/6 of the conceptual energy knowledge items correctly to be able to generate any argument pro conventional energies after visiting the exhibition, and had to solve approximately 1/5 of the test correctly to generate more than two arguments pro conventional energies after the visit.
Discussion
Students’ critical energy literacy increases after their exhibition visit
With regard to our first research question, we found that students’ renewable energies-arguments did not change in comparison from before to after the exhibition visit. However, the proportion of arguments contra renewable energy increased significantly. This means that after their exhibition-visit, students stated significantly more aspects of renewable energy and its use that they rated as negative or difficult. In contrast, for students’ conventional energies-arguments, we found that their number was significantly higher after visiting the exhibition, and this was due to the significant increase in both pro- and contra-arguments. Thus, students were able to identify significantly more negative as well as positive aspects of conventional energy sources after visiting the exhibition than before.
At first glance, these results seem to contradict a successful intervention in favor of the energy transition, since the students seem to have learned more negative aspects about renewable energies and positive aspects about conventional energies and their use. But we argue that this must not be the case for the following reasons. Firstly, students started the intervention with a higher knowledge about positive aspects of renewable energies and even afterwards name much more arguments pro renewable energies than pro conventional energies on average. Secondly, we were able to observe that, overall, all students were in favor of the energy transition both before and after visiting the exhibition. Therefore, the circumstance that they seem to have learned more positive facts about conventional energy and more negative aspects of renewable energies, can ‘be regarded as key indicators of deliberate opinion formation and reflective judgement’ (Knipfler, Citation2009, p. 39) as these facts are against their own bias. And thirdly, knowing about negative aspects of renewable energies and the advantages of conventional energies is ultimately necessary to understand why the energy transition is as complex and challenging as it is and might actually help a critical energy literate person to address those challenges in a realistic way (Lowan-Trudeau & Fowler, Citation2021). In light of these considerations, our findings can be interpreted in a way that by visiting the agential exhibition, students were able to increase their critical energy literacy. This presents an important step forward in understanding the role that such agential, modern SSI-exhibitions play in fostering energy literacy and scientific literacy in general, thus effectively supplementing formal science education.
Conceptual energy knowledge helps students acquire critical energy literacy
Study findings showed that conceptual energy knowledge, but not topic interest, acted as a prerequisite to the acquisition of energy literacy: conceptual knowledge influenced students’ arguments pro conventional and contra renewable-energies after their exhibition visit, with robust effects when controlling for the respective T1 values. Additionally, conceptual energy knowledge was a necessary condition for students to generate a meaningful number of arguments for conventional energy sources. These results are consistent with the assumption that conceptual energy knowledge may be useful in interpreting the complex systemic and interdisciplinary information regarding the energy transition in SSI-learning environments. The present study findings are therefore in line with evidence on the importance of prior knowledge in learning (e.g. Bransford, Citation2000) and on the prominent role that conceptual energy knowledge should play in energy literacy (Chen, Citation2014; DoE, Citation2014; Nordine, Citation2016).
However, the results of the NCAs also showed that students needed to solve 20% of items in the conceptual energy knowledge test correctly (on average, students solved 48% of the items correctly) in order to substantially increase their arguments about conventional energies. In other words, only those students at the very low end of conceptual energy knowledge (which accounted for about 8%) had a hard time making use of the exhibit; most students, however, had a sufficient level of energy knowledge to be able to make some sense of the exhibit.
The fact that we did not detect any influence of prior topic interest on students’ acquisition of critical energy literacy during their exhibition visit is not consistent with previous research that typically identifies individual interest as a good predictor of engagement and learning in corresponding learning environments (Carman et al., Citation2021; Krapp, Citation2002; Renninger & Hidi, Citation2016). Perhaps the motivating effect of personal interest in the topic was limited by the fact that the students did not go to the exhibition voluntarily and precisely guided by their interests, but had to visit the exhibition with their classes anyway. Another explanation might be, that students’ situational interest during their exhibition visit, particularly the exhibition's user-centered interactive game (Duan et al., Citation2021), may have overridden students’ prior topic interest, as experiencing situational interest can also directly promote learning by increasing attention and engagement (Harackiewicz et al., Citation2016, p. 221). As triggers for situational interest are highly personal (Renninger & Bachrach, Citation2015), students’ engagement and learning in the exhibition, therefore, might be due to the multi-perspective design of the exhibition and its complex yet innovative format arousing students’ situational interest, rather than their prior interest in the topic.
In conclusion, this study does indicate that conceptual knowledge is to some extent beneficial and important for making use of free-choice learning activities in socio-scientific contexts. Specifically, study findings show that the impact of this learning activity is also meaningful for students with less-than-average prior conceptual energy knowledge in that only very basic levels of conceptual knowledge were necessary for making meaning of the exhibition. In total, the present study therefore contributes to answering the open question ‘in what ways school-based learning substantively transfers to nonschool life’ (Bransford et al., Citation2006, p. 216).
Implications
Despite the limitations of this study, i.e. the lack of experimentation of participation vs. no participation in the interactive game of the exhibition and the only partial operationalization of a critical energy literacy (for the investigation of the behavioral component, Kellberg et al., Citation2023), the results of this study are significant to the fields of formal and informal education for various reasons.
First, the evidence that the present research provides for the promotion of critical energy literacy through a modern SSI-exhibition in itself is of great value for the museum field. Because, although the emergence of critical and agential exhibitions demonstrates that museums have the potential and the will to ‘foster the changes the world needs most’ (Sutton et al., Citation2017), many museums are actually still quite hesitant to develop such exhibitions (Cameron, Citation2005, p. 216 and Pedretti & Navas Iannini, Citation2020, p. 708). Showing how effective these exhibitions can be, might support hesitant museums in their will to invest in these complex and somewhat costly exhibitions.
Second, there are strong indications that the learning observed in this study was related to the balanced presentation of a wide variety of perspectives on the energy transition (see exhibition description) and the novelty of the exhibition’s game (Duan et al., Citation2021), which crystallizes the exhibitions’ multi-perspectivity in a particularly strong way. These features of the researched agential SSI-exhibition appear not only desirable but also transferrable to new SSI-exhibitions or even formal-learning settings.
Third, this study confirms the assumption that conceptual energy knowledge is indeed helpful for learning in complex learning SSI-environments (e.g. Chen, Citation2014; DoE, Citation2014; Nordine, Citation2016). Thus, this study was able to provide evidence for a foundational assumption in educational research. Considering the competing visions of scientific literacy in school and the difficulties of implementing SSI-approaches in formal education this is of great value, as it shows that conceptual energy knowledge, which can be considered a goal of scientific literacy Vision I (Roberts, Citation2007) in itself, also plays an important part in the development of a critical scientific literacy in the Vision II-III sense (Valladares, Citation2021). Despite this influence being relatively small, this result still demonstrates the complementary relationship of formal and informal learning venues for supporting critical scientific literacy.
Ethics statement
The authors confirm that the study complies with the ethical guidelines and legal requirements of the country where the study was conducted (Germany). The Bavarian Ministry of State for Education and Culture, Science and Art in Germany approved the research. Student consent forms were signed by a legal guardian.
tsed-2023-0292-b-File019
Download MS Word (31 KB)tsed-2023-0292-b-File018
Download MS Word (31.1 KB)tsed-2023-0292-b-File017
Download MS Word (27.9 KB)tsed-2023-0292-b-File016
Download MS Word (27.5 KB)tsed-2023-0292-b-File015
Download MS Word (21.2 KB)Acknowledgements
We would like to thank the Bavarian Ministry of State for Education and Culture, Science and Art for approving this study and the Deutsches Museum for making this study possible as well as all students willing to participate in this educational research.
Disclosure statement
No potential conflict of interest was reported by the author(s).
Data availability statement
The data that form the basis for the conclusions of this article will be provided by the corresponding author upon request.
Additional information
Funding
Notes
1 https://d-nb.info/1074264452/34; https://www.lehrplanplus.bayern.de/fachprofil/realschule/physik/9.
2 Students who did not complete at least 50% of the conceptual knowledge test were excluded from further analyses that included conceptual energy knowledge, leaving 169 students to be included (N = 169).
References
- Adams, J., Kenner, A., Leone, B., Rosenthal, A., Sarao, M., & Boi-Doku, T. (2022). What is energy literacy? Responding to vulnerability in Philadelphia’s energy ecologies. Energy Research & Social Science, 91, September 2022, 102718. https://doi.org/10.1016/j.erss.2022.102718
- Aikenhead, G. S. (2006). Science education for everyday life: Evidence-based practice. Ways of knowing in science and mathematics series. Teachers College Press.
- Bamberger, Y., & Tal, T. (2008). Multiple outcomes of class visits to natural history museums: The students’ view. Journal of Science Education and Technology, 17(3), 274–284. https://doi.org/10.1007/s10956-008-9097-3
- Bell, L. (2008). Engaging the public in technology policy: A new role for science museums. Science Communication, 29(3), 386–398. https://doi.org/10.1177/1075547007311971
- Bell, P. (2009). Learning science in informal environments: People, places, and pursuits. Washinton, DC: The National Academies Press.
- Bossér, U. (2018). Exploring the complexities of integrating socioscientific issues in science teaching [Doctoral Dissertation, Linneaus University Dissertations: Vol. 304. Linnaeus University Press].
- Böttcher, F., Hackmann, A., & Meisert, A. (2016). Argumente entwickeln, prüfen und gewichten: Bewertungskompetenz im Biologieunterricht kontextübergreifend fördern – Konzeptentwicklung. MNU Journal, 3, 150–157.
- Bransford, J., Stevens, R., Schwartz, D., Meltzoff, A. N., Pea, R., Roschelle, J., Vye, N., Kuhl, P. K., Bell, P., Barron, B., Reeves, B., & Sabelli, N. (2006). Learning theories and education: Toward a decade of synergy. In P. Alexander, & P. Winne (Eds.), Handbook of educational psychology. (pp. 209–244). Erlbaum.
- Bransford, J. D. (2000). How people learn: Brain, mind, experience, and school: Expanded edition. National Academies Press.
- Burke, M. J., & Stephens, J. C. (2017). Energy democracy: Goals and policy instruments for sociotechnical transitions. Energy Research & Social Science, 33(2), 35–48. https://doi.org/10.1016/j.erss.2017.09.024
- Cameron, F. (2005). Contentiousness and shifting knowledge paradigms: The roles of history and science museums in contemporary societies. Museum Management and Curatorship, 20(3), 213–233. https://doi.org/10.1016/j.musmancur.2005.05.002
- Carman, J., Zint, M., Burkett, E., & Ibáñez, I. (2021). The role of interest in climate change instruction. Science Education, 105(2), 309–352. https://doi.org/10.1002/sce.21610
- Chen, R. F. (Ed.). (2014). Teaching and learning of energy in K-12 education. Springer.
- DeWaters, J. E., Powers, S. E., & Graham, M. (2007). Developing an energy literacy scale. Proceedings of the 2007 ASEE Annual Conference and Exposition, Honolulu, HI.
- DoE. U.S. Department of Energy. (2014). Energy literacy: Essential principles and fundamental concepts for energy education. A framework for energy education for learners of all ages. U.S. Department of Energy.
- Duan, R. J., Walker, G. J., & Orthia, L. A. (2021). Interest, emotions, relevance: Viewing science centre interactive exhibit design through the lens of situational interest. International Journal of Science Education, Part B, 11(3), 191–209. https://doi.org/10.1080/21548455.2021.1938740
- Duit, R., & Neumann, K. (2014). Ideas for a teaching sequence for the concept of energy – Understanding the effects of energy within all the sciences. School Science Review, 596(354), 63–66.
- Dul, J. (2016). Necessary condition analysis (NCA). Organizational Research Methods, 19(1), 10–52. https://doi.org/10.1177/1094428115584005
- Evans, H. J., & Achiam, M. (2021). Sustainability in out-of-school science education: Identifying the unique potentials. Environmental Education Research, 46, 1–22. https://doi.org/10.1080/13504622.2021.1893662
- Falk, J. H., & Dierking, L. D. (2000). Learning from museums: Visitor experiences and the making of meaning. American Association for State and Local History Book Series. AltaMira Press.
- Falk, J. H., & Dierking, L. D. (2010). The 95 percent solution: School is not where most Americans learn most of their science. American Scientist, 98, 486–493. https://doi.org/10.1511/2010.87.486. http://www.americanscientist.org/ issues/id.87/past.aspx.
- Falk, J. H., & Dierking, L. D. (2013). Museum experience revisited. Left Coast Press. https://doi.org/10.4324/9781315417851. https://www.taylorfrancis.com/books/9781315417851
- Falk, J. H., Dierking, L. D., & Adams, M. (2011). Living in a learning society: Museums and free-choice learning. In S. MacDonald (Ed.), Blackwell companions in cultural studies: Vol. 12. A Compagnion to Museum Studies (4th ed., pp. 323–339). Wiley-Blackwell.
- Farla, J., Markard, J., Raven, R., & Coenen, L. (2012). Sustainability transitions in the making: A closer look at actors, strategies and resources. Technological Forecasting and Social Change, 79(6), 991–998. https://doi.org/10.1016/j.techfore.2012.02.001
- Gladwin, D., & Ellis, N. (2023). Energy literacy: Towards a conceptual framework for energy transition. Environmental Education Research, 1–15. https://doi.org/10.1080/13504622.2023.2175794
- Harackiewicz, J. M., Smith, J. L., & Priniski, S. J. (2016). Interest matters: The importance of promoting interest in education. Policy Insights from the Behavioral and Brain Sciences, 3(2), 220–227. https://doi.org/10.1177/2372732216655542
- Herman, B. C., Sadler, T. D., Zeidler, D. L., & Newton, M. H. (2017). A socioscientific issues approach to environmental education. In G. Reis, & J. Scott (Eds.), Environmental discourses in science education ser: v.3. International perspectives on the theory and practice of environmental education (pp. 145–161). Springer. https://doi.org/10.1007/978-3-319-67732-3_11
- Herrmann-Abell, C. F., & DeBoer, G. E. (2017). Investigating a learning progression for energy ideas from upper elementary through high school. Journal of Research in Science Teaching, 9(2-3), 71. https://doi.org/10.1002/tea.21411
- Hine, A., & Medvecky, F. (2015). Unfinished science in museums: a push for critical science literacy. ICOM, 14(2), A04.
- Hodson, D. (2003). Time for action: Science education for an alternative future. International Journal of Science Education, 25(6), 645–670. https://doi.org/10.1080/09500690305021
- Hohenstein, J., & Moussouri, T. (2018). Museum learning: Theory and research as tools for enhacing practice. Abingdon, England: Routledge.
- Jorgenson, S. N., Stephens, J. C., & White, B. (2019). Environmental education in transition: A critical review of recent research on climate change and energy education. The Journal of Environmental Education, 50(3), 160–171. https://doi.org/10.1080/00958964.2019.1604478
- Kellberg, S., & Newinger, C. (2018). Turning energy around: An interactive exhibition experience. Science Museum Group Journal, 9(9), 1–17. https://doi.org/10.15180/180909
- Kellberg, S., Nordine, J., Keller, M., & Lewalter, D. (2023). Fostering students’ willingness to act pro-environmentally through an identity-oriented socio-scientific exhibition on the energy transition. Frontiers in Education, 8, 1081633. https://doi.org/10.3389/feduc.2023.1081633
- Kinslow, A. T., Sadler, T. D., & Nguyen, H. T. (2018). Socio-scientific reasoning and environmental literacy in a field-based ecology class. Environmental Education Research, 20(2), 1–23. https://doi.org/10.1080/13504622.2018.1442418
- Knipfler, K. (2009). Pro or con nanotechnology? Support for critical thinking and reflective judgement at science museums [Dissertation].
- Kollmann, E. K., Reich, C., Bell, L., & Goss, J. (2013). Tackling tough topics: Using socio-scientific issues to help museum visitors participate in democratic dialogue and increase their understandings of current science and technology. Journal of Museum Education, 38(2), 174–186. https://doi.org/10.1080/10598650.2013.11510768
- Koster, E. H. (1999). In Search of relevance: Science centers as innovators in the evolution of museums. Daedalus, 28(2), 277–296.
- Krapp, A. (2002). Structural and dynamic aspects of interest development: Theoretical considerations from an ontogenetic perspective. Learning and Instruction, 12(4), 383–404. https://doi.org/10.1016/S0959-4752(01)00011-1
- Lee, O., & Grapin, S. E. (2022). The role of phenomena and problems in science and STEM education: Traditional, contemporary, and future approaches. Journal of Research in Science Teaching, 59(7), 1301–1309. https://doi.org/10.1002/tea.21776
- Lewalter, D., Gegenfurtner, A., & Renninger, K. A. (2021). Out-of-school programs and interest: Design considerations based on a meta-analysis. Educational Research Review, 34(1), 100406. https://doi.org/10.1016/j.edurev.2021.100406
- Liu, X., & Park, M. (2014). Contextual dimensions of the energy concept and implications for energy teaching and learning. In R. F. Chen (Ed.), Teaching and learning of energy in K-12 education (pp. 175–186). Springer. https://doi.org/10.1007/978-3-319-05017-1_10
- Lowan-Trudeau, G., & Fowler, T. A. (2021). Towards a theory of critical energy literacy: The youth strike for climate, renewable energy and beyond. Australian Journal of Environmental Education, 17, 1–11. https://doi.org/10.1017/aee.2021.15
- Mayring, P. (2000). Qualitative inhaltsanalyse // qualitative content analysis. Forum Qualitative Sozialforschung / Forum: Qualitative Social Research, 1(2), Art. 20. https://doi.org/10.17169/fqs-1.2.1089
- McGhie, H. (2018). Museums as key sites to accelerate climate change education, action, research and partnerships: Non-party stakeholder submission to the Talanoa Dialogue from the ‘International Symposium on Climate Change and Museums'.
- McGhie, H. (2020). Evolving climate change policy and museums. Museum Management and Curatorship, 35(6), 653–662. https://doi.org/10.1080/09647775.2020.1844589
- Miller, C. A., Iles, A., & Jones, C. F. (2013). The social dimensions of energy transitions. Science as Culture, 22(2), 135–148. https://doi.org/10.1080/09505431.2013.786989
- National Research Council. (2012). A framework for K-12 science education: Practices, crosscutting concepts, and core ideas. Committee on a Conceptual Framework for New K-12 Science Education Standards. Board on Science Education, Division of Behavioral and Social Sciences and Education. Washington, DC: National Academies Press. https://doi.org/10.17226/13165
- Neumann, K., Viering, T., Boone, W. J., & Fischer, H. E. (2013). Towards a learning progression of energy. Journal of Research in Science Teaching, 50(2), 162–188. https://doi.org/10.1002/tea.21061
- Newinger, C., Geyer, C., & Kellberg, S. (Eds.). (2017). Energie.Wenden: Energy transitions as chance and challenge in our time. oekom verlag.
- Nordine, J. (Ed). (2016). Teaching energy across the sciences, K-12. NSTApress National Science Teachers Association.
- OECD. (2019). Pisa 2018 assessment and analytical framework. PISA, OECD Publishing. https://doi.org/10.1787/b25efab8-en
- Pedretti, E., & Navas Iannini, A. M. (2020). Controversy in science museums: Re-imagining spaces and practice. Routledge.
- Presley, M. L., Sickel, A. J., Muslu, N., Merle-Johnson, D., Witzig, S. B., Izci, K., & Sadler, T. S. (2013). A framework for socio-scientific issues based education. Science Educator, 22(1), 26–32.
- Reimers Arias, F., & Chung, C. K. (Eds.). (2016). Teaching and learning for the twenty-first century: Educational goals, policies, and curricula from six nations. Harvard Education Press.
- Renninger, K. A., & Bachrach, J. E. (2015). Studying triggers for interest and engagement using observational methods. Educational Psychologist, 50(1), 58–69. https://doi.org/10.1080/00461520.2014.999920
- Renninger, K. A., & Hidi, S. (2016). The power of interest for motivation and engagement. Routledge. https://doi.org/10.4324/9781315771045
- Roberts, D. A. (2007). Scientific literacy / science literacy. In S. K. Abell, & N. G. Lederman (Eds.), Handbook of research on science education (pp. 729–780). Erlbaum.
- Sadler, T. D. (Ed.). (2011). Socio-scientific issues in the classroom (Vol. 39). Springer Netherlands. https://doi.org/10.1007/978-94-007-1159-4
- Sadler, T. D., Barab, S. A., & Scott, B. (2007). What do students gain by engaging in socioscientific inquiry? Research in Science Education, 37(4), 371–391. https://doi.org/10.1007/s11165-006-9030-9
- Sadler, T. D., Foulk, J. A., & Friedrichsen, P. J. (2016). Evolution of a model for socio-scientific issue teaching and learning. International Journal of Education in Mathematics, Science and Technology, 5(1), 75. https://doi.org/10.18404/ijemst.55999
- Sjöström, J., & Eilks, I. (2018). Reconsidering different visions of scientific literacy and science education based on the concept of Bildung, 24, 65–88. https://doi.org/10.1007/978-3-319-66659-4_4.
- Sutton, S. (2020). The evolving responsibility of museum work in the time of climate change. Museum Management and Curatorship, 35(6), 618–635. https://doi.org/10.1080/09647775.2020.1837000
- Sutton, S., & Robinson, C. (2020). Museums and public climate action. Journal of Museum Education, 45(1), 1–4. https://doi.org/10.1080/10598650.2020.1722513
- Sutton, S., Wylie, E., Economopolous, B., O'Brien, C., Shapiro, S., & Xu, S. (2017). Museums and the future of a healthy world: “Just, verdant and peaceful”. Curator: The Museum Journal, 60(2), 429–441. doi: 10.1111/cura.12200
- Toplak, M. E., & Stanovich, K. E. (2003). Associations between myside bias on an informal reasoning task and amount of post-secondary education. Applied Cognitive Psychology, 17(7), 851–860. https://doi.org/10.1002/acp.915
- Tynan, M. C., Credé, M., & Harms, P. D. (2020). Are individual characteristics and behaviors necessary-but-not-sufficient conditions for academic success? A demonstration of Dul’s (2016) necessary condition analysis. Learning and Individual Differences, 77(7), 101815. https://doi.org/10.1016/j.lindif.2019.101815
- Valladares, L. (2021). Scientific literacy and social transformation: Critical perspectives about science participation and emancipation. Science & Education, 30(3), 557–587. https://doi.org/10.1007/s11191-021-00205-2
- Wang, J. C., & Wang, T. H. (2023). Learning effectiveness of energy education in junior high schools: Implementation of action research and the predict-observe-explain model to STEM course. Heliyon, 9(3), e14058. https://doi.org/10.1016/j.heliyon.2023.e14058
- World Economic Forum. (2020). The Global Risks Report 2020. Insight Report. Geneva: World Economic Forum.
- Yun, A., Shi, C., & Jun, B. G. (2020). Dealing with socio-scientific issues in science exhibition: A literature review. Research in Science Education, 52(1), 99–110. https://doi.org/10.1007/s11165-020-09930-0
- Zeidler, D. L., Herman, B. C., & Sadler, T. D. (2019). New directions in socioscientific issues research. Disciplinary and Interdisciplinary Science Education Research, 1(1), 717. https://doi.org/10.1186/s43031-019-0008-7
- Zeidler, D. L., & Newton, M. H. (2017). Using a socioscientific issues framework for climate change education: An ecojustice approach (pp. 56–65). https://doi.org/10.4324/9781315629841-5