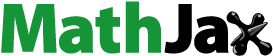
ABSTRACT
Gallbladder cancer (GBC) is commonly diagnosed at late stages when conventional treatments achieve only modest clinical benefit. Therefore, effective treatments for advanced GBC are needed. In this context, the administration of T cells genetically engineered with chimeric antigen receptors (CAR) has shown remarkable results in hematological cancers and is being extensively studied for solid tumors. Interestingly, GBC tumors express canonical tumor-associated antigens, including the carcinoembryonic antigen (CEA). However, the potential of CEA as a relevant antigen in GBC to be targeted by CAR-T cell-based immunotherapy has not been addressed. Here we show that CEA was expressed in 88% of GBC tumors, with higher levels associated with advanced disease stages. CAR-T cells specifically recognized plate-bound CEA as evidenced by up-regulation of 4-1BB, CD69 and PD−1, and production of effector cytokines IFN-γ and TNF-α. In addition, CD8+ CAR-T cells up-regulated the cytotoxic molecules granzyme B and perforin. Interestingly, CAR-T cell activation occurred even in the presence of PD-L1. Consistent with these results, CAR-T cells efficiently recognized GBC cell lines expressing CEA and PD-L1, but not a CEA-negative cell line. Furthermore, CAR-T cells exhibited in vitro cytotoxicity and reduced in vivo tumor growth of GB-d1 cells. In summary, we demonstrate that CEA represents a relevant antigen for GBC that can be targeted by CAR-T cells at the preclinical level. This study warrants further development of the adoptive transfer of CEA-specific CAR-T cells as a potential immunotherapy for GBC.
Introduction
Gallbladder cancer (GBC) is the world’s sixth most common gastrointestinal malignancy with a prevalence of two cases per 100.000Citation1. Despite the low incidence worldwide, this cancer shows a substantial geographic variation with high prevalence in Japan (7 cases per 100.000), American Indians in New Mexico, USA (8.9 cases per 100.000), females in India (21.5 cases per 100.000) and Mapuche Indians of Chile (12.3 and 27.3 cases 100.000 for males and females respectably)Citation2–5. GBC is usually detected at late stages, where conventional treatments achieve modest clinical benefit and is characterized by early metastasis to adjacent lymph nodes and a high mortality rate once diagnosedCitation6. Hence, more effective treatments are needed for advanced GBC.
T cell-based immunotherapy is emerging as an attractive approach to treating solid tumors, including several gastrointestinal cancersCitation7–9. However, due to the low incidence worldwide, immunotherapy for GBC is poorly explored. Even if research on GBC is scarce, emerging evidence indicates that GBC tumors are immunogenic and can be targeted by T cells. Higher tumor infiltration of CD8+ T cells has been associated with better clinical outcomes, such as improved overall survivalCitation10. Genomic analysis of GBC tumors revealed that they have a relatively high mutational burden, including non-synonymous mutations resulting in neoantigens that can be recognized by CD8+ T cells at least in vitroCitation11. Moreover, antibodies blocking inhibitory receptors, including the programmed cell death protein 1/programmed cell death ligand 1 (PD−1/PD-L1) axis, have emerged as a powerful approach to revert T cell suppression and mediate strong anti-tumor T cell immune responses, leading to clinical benefit in a wide variety of solid tumorsCitation12,Citation13 including advanced cancers of the biliary tract, such as GBCCitation14,Citation15. Moreover, a few case reports highlight the potential of combining immunotherapy with conventional treatments for advanced GBCCitation16–18.
Immunotherapies based on the administration of tumor-specific T cells hold great potential for different types of cancerCitation19,Citation20. In particular, adoptive transfer of T cells genetically engineered with “chimeric antigen receptors” (CAR) T cells have shown remarkable results in the treatment of hematological cancers and have been widely studied for solid tumorsCitation21,Citation22. Among the tumor antigens expressed on GBC, carcinoembryonic antigen (CEA) is a highly promising target for diagnosis and immunotherapies. CEA is expressed in the majority (57–89%) of GBC cancers and its expression correlated with worse overall survivalCitation23. Moreover, elevated serum CEA levels are significantly associated with GBCCitation24 and can be used as a predictor marker for metastatic diseaseCitation25.
Previous studies have shown the efficacy and safety of CEA-specific CAR-T cells against colorectal malignancies, including studies in transgenic animal modelsCitation26–28 and in patients with liver metastasesCitation29,Citation30. Altogether, these studies have demonstrated some efficacy of CEA-specific CAR-T cells without significant side effects in colorectal cancer. However, the potential of CEA as a relevant antigen in GBC to be targeted by CAR-T cells has not been addressed. Here, we demonstrate that T cells transduced with a CEA-specific CAR containing the signaling domains of CD3z and the co-stimulatory molecule OX40 were specifically activated by CEA even in the presence of PD-L1. Furthermore, CAR-T cells exhibited specific in vitro activation and cytotoxicity against GBC cell lines expressing CEA and PD-L1 and suppressed tumor growth in vivo. Taken together, these results highlight the potential of CEA-specific CAR-T cells as a therapy for GBC.
Materials and methods
Patients and tumor samples
A retrospective analysis of cholecystectomy specimens and clinical data from patients diagnosed with GBC from 2001 to 2015 at the Pathologic Anatomy Sub-department of the Hospital Base Valdivia (Chile) was performed for CEA expression analysis. 186 cases of primary invasive gallbladder adenocarcinoma cases were considered. In situ adenocarcinoma, squamous carcinoma, neuroendocrine carcinoma and metastases were excluded. Cases were selected using the code C.23, according to the international coding for oncologic disease CIE−0. For each tumor sample, 2–3 foci of neoplastic invasion were selected for the analysis under a light microscope, and these areas were marked. The study was performed in agreement with the Helsinki Declaration and approved by the “Comité Ético Científico Servicio de Salud Valdivia”. All patients signed a letter of informed consent for publication at the time of surgery.
Tissue microarrays and immunohistochemistry
Quick-Ray® UT06 Manual Tissue Microarrayer (Unitma Co., Ltda, Seoul, Korea) was used according to the manufacturer’s instructions. Representative blocks (tissue specimens fixed in 10% neutral buffered formalin and embedded in paraffin wax) were selected for immunohistochemistry analysis. Paraffin blocks were cut into 3 μm thick slides and mounted on positively charged slides together with their respective positive control tissue. CEA staining was performed with an automatic BenchMark GX Ventana system using an ultraview universal DAB detection kit (Roche, Arizona, USA), following the manufacturer’s instructions. The primary antibody used was an anti-CEA monoclonal mouse IgG, clone COL−1 (1:200 dilution; Thermo Scientific). CEA expression was evaluated blindly, by a pathologist, under light microscopy, and antigen expression was classified by comparison with positive tissue controls included in each slice.
Vector construction
The CEA-specific CAR was generated using the BW431/26 scFv pBULLET retroviral vector as backbone, a kind gift from Prof. Hinrich Abken (Cologne, Germany). The CAR was generated by linking the CEA-specific scFv BW431/26 CAR to a human IgG1 spacer, followed by a mouse CD4 transmembrane domain, an intracellular CD3ζ moiety and OX40 co-stimulation signaling domain. pBULLET coding for the green fluorescent protein (pGFP) was used as control. The lentiviral vector pLenti CMV-LUC-Puro (pLUC-Puro, Addgene plasmid #17477) was used to generate lentiviral particles for transduction of luciferase-expressing GB-d1 cell line. Lentiviral particles were produced by co-transfection of pLUC-Puro plasmid, psPAX2 (Addgene plasmid #12260), and pCMV-VSV-G (Addgene plasmid #8454) using CaCl2 method.
Cells
Phoenix-Ampho (ATCC® CRL−3213) and HT29 (ATCC® HTB−38) cell lines were obtained from ATCC. GB-d1, NOZ, 24TKB and OCUG−1 gallbladder cancer cell lines were kindly provided by Dr. Juan Carlos Roa (Department of Pathology, Pontificia Universidad Católica de Chile, Santiago, Chile). GB-d1 expressing luciferase was obtained by lentiviral transduction with pLUC-PuromycinCitation31. All cell lines were cultured in complete Dulbecco’s Modified Eagle Medium (DMEM) (ThermoFisher Scientific) or Roswell Park Memorial Institute (RPMI) 1640 Medium (ThermoFisher Scientific). All cell lines were routinely tested for mycoplasma contamination.
Transduction and selection of producer cell lines
4×105 Phoenix-Ampho cells were seeded on 6-well plates (Nunclon Delta Surface, Thermo Scientific). After 24 h, the cells were transfected with CAR-encoding plasmids using the CaCl2 precipitation method previously describedCitation32. After 48 h, the supernatants containing retroviral particles were recovered and passed through a 0.2 µM filter. Stable producer cell lines were generated by transduction of 5 × 105 Phoenix-Ampho cells cultured with retroviral supernatants and 4 μg/mL of polybrene (Sigma-Aldrich, Cat# 107689) and centrifuged for 90 minutes at 1200 g at 32°C. Transduction efficiency were assessed by flow cytometry after 48 h. IgG1+ cells were selected by cell sorter (FACS Aria II) until a > 95% producer population was obtained.
Activation and transduction of T cells
Peripheral blood mononuclear cells (PBMC) were obtained from 30–80 mL of blood from healthy donors by Ficoll-Parque™ separation (GE Healthcare, Cat.#17-5442-02). PBMC were cultured in RPMI complete medium supplemented with hIL−2 (0.2 µg/mL, BioLegend, Cat.#589108), αCD3 (0.1 µg/mL, BioLegend Cat.#317315), and αCD28 (1 µg/mL, BioLegend Cat.#302923). After 48 h, T cells were transduced by centrifugation in Retronectin-coated plates (12 µg/mL RetroNectin, Takara Cat.#T100B). Retroviral supernatants were loaded on Retronectin plates and centrifuged at 2000 g for 90 minutes at 32°C. 1 × 106 T cells per mL were seeded in a 1:1 ratio of fresh media with retroviral supernatants and centrifuged at 1200 g for 90 minutes at 37°C. 16 h later, the cells were recovered, and a second transduction was performed. Transduction efficiency was assessed 48 h after the second round of transduction.
CAR-T cells functional assays
Activation of CAR-T cells was performed by culturing 1 × 105 unsorted T cells on CEA-coated plates (1 µg/mL, Fitz Gerald, Cat.# 30-AC32) and PD-L1 (1–5 µg/mL Cat.# 156-B7-100) or with GBC cell lines at 1:1 ratio. After 16 h, the expression of activation markers was assessed by flow cytometry analyzing CAR+ T cells. Intracellular staining was performed by incubation with brefeldin A for 6 h according to the manufacturer’s instructions (Biolegend, Cat.# 420601). For the cytotoxicity assay, transduced T cells were purified by cell sorting (FACS Aria II) and cultured at different effector:target ratios with 1 × 104 cells GBC cell lines expressing luciferase. After 24 h, D-luciferin (0.5 µg/mL, Promega Cat.#E1602) was added to the media and luminescence was measured (Synergy HT, Bio Tek). The percentage of specific lysis was calculated by measuring relative light units (RLU) and applying the formula:
The maximal killing was calculated by permeabilization with −20°C methanol and control using tumor cells without effector T cells.
Flow cytometry staining
Monoclonal antibodies specific for human CD3-PeCy7, CD3-APC-Cy7 (Clone HIT3a), CD4-Alexa Fluor 488 (Clone OKT4), CD8-APC-Cy7, CD8-Brilliant Violet 421 (Clone RPA-T8), IgG1-PE (Clone HP6017), IgG1 Isotype-PE (Clone MOPC−21), 41BB-PE (Clone 4B4–1), PD−1-PeCy7, PD−1-Brilliant Violet 421 (Clone EH12.2H7), CD69-APC-Cy7 (Clone FN50), IFNγ-APC-Cy7 (Clone 4S.B3), TNFα-Alexa Fluor 488 (Clone Mab11), granzyme B-APC (Clone GB−11), perforin-PerCPCy5.5 (Clone B-D48), CEA-APC (Clone ASL−32), PD-L1-Brilliant Violet 421 (Clone 29E.2A3), TruStain fcX™ (Clone 93) and viability dye Zombie Aqua (ref 423,101), were purchased from Biolegend. Samples were analyzed in a FACSCanto II cytometer (BD Bioscience) and data were analyzed using FlowJo version X.0.7 (Tree Star, Inc.).
Tumor challenge
NOD.CB17-Prkdcscid/J (NOD/SCID) mice were purchased from Jackson Laboratories, kept at the animal facility of Fundación Ciencia & Vida and maintained according to the “Guide to Care and Use of Experimental Animals, Canadian Council on Animal Care”. This study was carried out in accordance with the recommendations of the “Guidelines for the welfare and use of animals in cancer research, Committee of the National Cancer Research Institute”. The protocol was approved by the “Comité de Bioética y Bioseguridad” from Fundación Ciencia & Vida. Blinding or randomization strategy was done whenever possible. No animals were excluded from the analysis and, male and female mice were used indistinctly. Mice were allocated randomly in the different experimental procedures. GB-d1 cells were inoculated by injecting subcutaneously 5 × 106 cells. Seven days after tumor inoculation, transduced human T cells containing 1 × 106 CAR+ or GFP+ T cells were transferred intravenously in 200 µL of sterile PBS. When indicated, 50.000 UI/mL of hIL2 and 200 µg anti-PD−1 antibody nivolumab (Bristol Maier Squib) were injected intraperitonially three consecutive days after T cell transfer. Tumor growth was monitored by measuring perpendicular tumor diameters with calipers. Tumor volume was calculated using the following formula: V = (D x d2)/2 where V is the volume (mm3), D is the larger diameter (mm) and d is the smaller diameter (mm). Mice were euthanatized when moribund or when the mean tumor diameter was ≥15 mm, according to the approved bioethical protocol.
Statistical analysis
Statistical analysis was performed using GraphPad Prism software (GraphPad Software Inc.). Unpaired t-tests were performed pairwise between relevant groups. Two-way ANOVA between relevant groups when analyzing tumor growth. Error bars in the figures indicate the mean plus SEM. P value < .05 was considered statistically significant; *P ≤ .05, **P ≤ .01, ***P ≤ .001 and ****P ≤ .0001.
Results
CEA is highly expressed in GBC tumor biopsies
We first sought to validate CEA as a potential target in GBC. To this end, we analyzed the expression of CEA (also known as CEACAM5) by immunohistochemistry in tumor biopsies obtained from gallbladder cancer (GBC) patientsCitation33. We collected samples from 186 primary tumors at different stages and categorized CEA expression as negative (0), low (1+), intermediate (2+) or high (3+) (). We observed that 86% of the GBC tumors expressed CEA at some level, with higher CEA levels associated with more advanced disease stages (). Interestingly, 23% and 54% of advanced GBC tumors (stages T3 and T4) expressed intermediate (2+) and high (3+) levels of CEA, respectively (). These findings support CEA as a clinically relevant antigen in GBC that can potentially be targeted by CAR-T-based immunotherapy.
Figure 1. CEA expression in GBC tumor biopsies.

CEA-specific CAR-T cells are activated in the presence of PD-L1
Next, we tested a CEA-specific CAR consisting of a humanized scFvCitation34 linked to an IgG1-derived spacer domain, a transmembrane domain, and intracellular signaling domains from OX40 and CD3ζ, a design similar to others previously describedCitation35,Citation36. Replication-deficient retroviral particles encoding the CAR or green fluorescent protein (GFP), as control, were produced using the Phoenix-Ampho cell lineCitation32. Then, human T cells obtained from peripheral blood mononuclear cells (PBMC) from healthy donors were activated in vitro with plate-bound anti-CD3 and anti-CD28 agonistic antibodies. Subsequently, activated T cells were transduced with the retroviral vectors, and transgene expression was evaluated 48–72 h later, observing 34–54% and 72–83% of transduction efficiency for both CAR and GFP constructs, respectively (Supplementary Figure S1a,b). The functionality of the CEA-specific CAR was tested in vitro by stimulating transduced T cells with plates coated with CEA alone or in combination with PD-L1 for 16 h. As a negative control, plates were coated with bovine serum albumin (BSA). CAR-expressing CD8+ T cells specifically recognized plate-bound CEA, as evidenced by strong surface up-regulation of activation markers 4-1BB, CD69 and, to a lesser extent, PD−1 (4-1BB: from 28.70% ± 6.26 to 69.44% ± 12.12; CD69: from 23.41 ± 4.71 to 56.72% ± 9.77; PD−1: from 18.69 ± 7.31 to 29.8 ± 5.83. Data is expressed as mean ± SEM). This CEA-specific activation was unaltered in the presence of PD-L1 (coated at 1 μg/mL), which has been demonstrated to inhibit CD28-based CAR constructsCitation37,Citation38. However, when the PD-L1 coating concentration was increased to 5 μg/mL (nearly 25 times the molar amount of CEA), the up-regulation of 4-1BB, CD69 and PD−1 was severely impaired () (4-1BB: 40.88% ± 8.32; CD69: 31.98% ± 3.26; PD−1: 10.88% ± 3.03). Similar up-regulation of activation markers, but to a lesser extent, was observed in CEA-activated CD4+ CAR-T cells (4-1BB: from 14.65% ± 4.52 to 33.59% ± 10.33; CD69: from 26.78 ± 3.37 to 44.92% ± 5.56; PD−1: from 18.14 ± 6.50 to 31.28% ± 7.62), which was also reduced by 5 μg/mL PD-L1 coating (4-1BB: 16.70% ± 5.71; CD69: 27.44% ± 2.15; PD−1: 13.98% ± 4.09) (). These results indicate that CAR-T cells can specifically recognize CEA, regardless of the presence of PD-L1, but are still sensitive to high levels of PD-L1. Next, we determined the production of effector molecules by CAR-T cells. For this purpose, T cells were stimulated as described in , followed by an additional 4 h culture in the presence of brefeldin A. Then, intracellular staining of cytokines and cytotoxic molecules was performed. CAR-T cells specifically produced IFN-γ and TNF-α upon CEA stimulation alone () (CD8+ T cells: IFN-γ: from 5.94% ± 2.44 to 27.94% ± 4.83; IFN-γ and TNF-α: from 3.16% ± 1.64 to 21.83 ± 3.97) (CD4+ T cells: IFN-γ: from 5.80% ± 3.94 to 13.62% ± 2.28, IFN-γ and TNF-α: from 3.43% ± 2.77 to 11.81% ± 3.49) or in the presence of PD-L1 (1 μg/ml coating). Increased PD-L1 partially reduced IFN-γ and TNF-α production by CD8+ () and CD4+ () CAR-T cells (CD8+ T cells: IFN-γ: 16.94% ± 5.60; IFN-γ and TNF-α: 12.54% ± 4.13) (CD4+ T cells: IFN-γ: 11.6% ± 7.23; IFN-γ and TNF-α: 8.30% ± 5.56). In addition, CD8+ CAR-T cells also up-regulated granzyme B () and perforin () in response to CEA stimulation (granzyme B: from 66.03% ± 9.93 to 93.05% ± 3.68; perforin: from 36.97% ± 7.88 to 60.63% ± 13.87), whereas high PD-L1 levels tended to reduce the expression of both cytotoxic molecules but this decrease was not significant. Taken together, these results indicated that CEA-specific CAR-T cells up-regulate effector cytokines and cytotoxic molecules in response to CEA but showed a limited sensitivity to PD-L1 inhibition. The different sensitivities to PD-L1-mediated inhibition observed when analyzing the up-regulation of surface molecules and intracellular effector molecules are likely due to differences in expression kinetics.
Figure 2. CEA-specific activation of CAR-T cells.

Figure 3. CEA-specific production of effector cytokines and cytotoxic molecules by CAR-T cells.

CAR-T cells recognize GBC cell lines expressing CEA and PD-L1
Then, we addressed the potential of CEA-specific CAR-T cells to recognize GBC cells expressing both CEA and PD-L1. To this end, CAR-T cells were co-cultured with the GBC cell lines GB-d1 and NOZ displaying variable levels of CEA (Supplementary Figure S2a, c) and high levels of PD-L1, which can be further up-regulated after IFN-γ treatment (Supplementary Figure S2b, d). After co-culture, CEA-specific activation was evidenced by up-regulation of surface markers 4-1BB, CD69 and PD−1 in both CD8+ () (4-1BB: 70.85% ± 11.17 for Gb-d1 and 56.68% ± 8.29 for NOZ; CD69: 32.92% ± 11.13 for Gb-d1 and 56.68% ± 8.29 for NOZ; PD−1: 59.83% ± 8.36 for Gb-d1 and 48.27% ± 9.31 for NOZ) and CD4+ () (4-1BB: 42.23% ± 8.76 for Gb-d1 and 28.18% ± 7.31 for NOZ; CD69: 39.35% ± 4.96 for Gb-d1 and 18.78% ± 3.10 for NOZ; PD−1: 36.52% ± 5.68 for Gb-d1 and 24.68% ± 7.37 for NOZ) CAR-T cells, to a similar extent than levels observed after stimulation with purified CEA-coated plates. We extended our analysis to include the OCUG−1 GBC cell line, which does not express CEA and PD-L1, as well as 24TKB cell line as a positive control expressing high levels of CEA but no PD-L1 (Supplementary Figure S2e-f)Citation39. Up-regulation of activation markers was observed after co-culturing CD8+ (Supplementary Figure S3a-b) and CD4+(Supplementary Figure S3c-d) CAR-T cells with 24TKB but no OCUG−1 cells (CD8+ T cells: 4-1BB: 21.27% ± 5.18 for OCUG−1 and 92.90 ± 1.4 for 24TKB; CD69: 13.54% ± 2.85 for OCUG−1 and 55.37% ± 11.85 for 24TKB. PD−1: 5.48% ± 2.12 for OCUG−1 and 47.85% ± 13.35 for 24TKB) (CD4+ T cells: 4-1BB: 13.9% ± 5.37 for OCUG−1 and 74.85% ± 6.95 for 24TKB; CD69: 17.81% ± 4.34 for OCUG−1 and 55.35% ± 8.25 for 24TKB. PD−1: 24.77% ± 11.71 for OCUG−1 and 57.2% ± 9.3 for 24TKB). These results show that CEA-specific CAR-T cell can specifically recognize CEA-expressing GBC cell lines regardless of PD-L1 expression.
Figure 4. CAR-T cell activation upon recognition of CEA-expressing GBC cell lines.

CAR-T cell-mediated cytotoxicity against CEA-expressing GBC cell lines
To investigate the cytotoxic potential of CAR-T cells, we evaluated the expression of cytotoxic molecules in co-culture experiments. As expected, CD8+ CAR-T cells up-regulate granzyme B and perforin in response to both GB-d1 and NOZ GBC cell lines () (granzyme B: 90.98% ± 5.92 for Gb-d1 and 91.40% ± 2.08 for NOZ; Perforin: 60.32% ± 8.04 for Gb-d1 and 57.18% ± 4.69 for NOZ). To assess in vitro anti-tumor cytotoxicity, co-culture experiments were performed with luciferase expressing GB-d1 cells at 1:1 and 1:5 target-to-effector ratios, and then luminescence was quantified. We observed CAR-T significantly eliminated GB-d1 cells compared to control GFP-transduced T cells () (Specific lysis at 1:5 Target:Effector ratio: 31.49% ± 5.83 for GFP T cells and 95.53 ± 3.00 for CAR-T cells). Finally, the ability of CAR-T cells to eliminate GBC cells was evaluated in vivo. To this end, NOD/SCID mice bearing palpable GB-d1 tumors received adoptive transfer of CAR-T cells. We observed a significant reduction in tumor growth following transfer of T cells transduced with the CEA-specific CAR compared to GFP-transduced T cells (). A similar reduction in tumor growth was observed in mice inoculated with colorectal adenocarcinoma cells HT−29, which express higher levels of CEA and lower levels of PD-L1 (Supplementary Figure S5a-c), indicating that CEA-specific CAR-T cells can target cells expressing limited levels of CEA and high amounts of PD−1. To test whether CAR-T cell-mediated tumor control could be improved, we combined CAR-T cells with systemic injections of IL−2 and an antibody blocking PD−1 (nivolumab). In this setting, intraperitoneal injections of anti-PD−1, did not increase the antitumor efficacy of CEA-specific CAR-T cells (Supplementary Figure S6a-b), suggesting that CAR-T cells can target GBC cells independently of PD−1-mediated suppression in this mouse model. Taken together, these results demonstrate that CEA-specific CAR-T cells display anti-tumor activity against GBC cell lines expressing CEA and PD-L1 at a preclinical level and warrant further development as a potential immunotherapy for GBC.
Figure 5. CAR-T cell-mediated cytotoxicity against CEA-expressing GBC cell lines.

Discussion
Cancer immunotherapy has emerged as a new standard of care for several malignancies. CAR-T cell immunotherapies have shown remarkable results in hematological cancers and have great potential for the treatment of solid tumors such as GBC. Here, we identified CEA as a tumor-associated antigen broadly expressed in GBC that can be targeted by a CAR-T cell immunotherapy at the preclinical level. We observed that most of the GBC samples analyzed expressed CEA at some level. Importantly, higher CEA expression was associated with advanced stage disease, making GBC a suitable disease for CEA-targeted immunotherapies. We showed that CEA-specific CAR-T cells were efficiently activated upon recognition of plate-bound CEA or CEA-expressing GBC cell lines, as assessed by up-regulation of surface markers (4-1BB, CD69 and PD−1), production of effector cytokines (IFN-γ and TNF-α), and cytotoxic molecules (granzyme B and perforin). Interestingly, CAR- T cell activation driven by both plate-bound CEA and CEA-expressing GBC cell lines, occurred even in the presence of PD-L1. In contrast to our results, other studies have shown that inhibition of TCR-mediated T cell activation can be observed with 10-fold lower levels of plate-bound PD-L1Citation40. CEA-specific CAR-T cells were still sensitive to PD−1 inhibition when PD-L1 coating concentration was significantly increased (5 µg/mL), suggesting a higher threshold for PD-L1 inhibition on CEA-specific CAR-T cells. Importantly, CAR-T cells efficiently eliminated Gb-d1 cells in vitro and significantly reduced tumor growth in immunodeficient mice to a similar extent as observed in HT29 tumors, a commonly used model of colorectal cancer that expresses low levels of PD-L1 and high levels of CEACitation41,Citation42, as other colorectal cancer cell linesCitation43. We selected the GBC cell lines GB-d1 and NOZ, which express intermediate levels of CEA and high levels of PD-L1, because they recapitulate key features of human tumors. Our results show that CEA-specific CAR-T cells exhibit antitumor activity regardless of the presence of PD-L1. Consistent with these in vitro results, we observed that the combination of CAR-T cells and a blocking antibody against PD−1, which is commonly used in the clinic, did not improve the control of GB-d1 tumors. However, whether this is particular to our model or a more general phenomenon in GBC remains to be elucidated. In this context, PD-L1 expression remains controversial as a predictive marker for GBC progression. An analysis of PD-L1 expression in 174 GBC tumors and tumor-infiltrating lymphocytes concluded that PD-L1 did not correlate with survival or prognosisCitation44. Another study analyzing 66 tumor samples found a correlation between high PD-L1 expression and worse overall survival (OS) but not progression-free survival (PFS), while the combination of CD8high and PD-L1 correlated with improved OS and PFSCitation45. Furthermore, another study in 75 GBC patients receiving PD−1 inhibitors and chemotherapy showed improved FPS but no significant difference in objective response or disease control rateCitation15. Future studies may help to clarify the role of PD−1 in GBC progression.
One of the major challenges of CAR-T cell-based immunotherapies in solid tumors is that the results obtained in mouse models do not always translate to the human setting. Therefore, testing the efficacy of CEA-specific CAR-T cells in humanized in vivo models is key to assessing the therapeutic potential of this type of immunotherapy for GBC. We observed that CEA-specific CAR-T cells significantly reduced the growth of GB-d1 tumors. Still, this type of humanized mouse model has some limitations. For example, the contribution of PD-L1 expressed on mouse cells in the tumor microenvironment, such as dendritic cells and macrophages, to the suppression of CAR-T cells expressing human PD−1 cannot be addressed. In addition, human CEA is not expressed in mice and mouse GBC tumor. Our model does not address potential side effects, particularly on-target/off-tumor toxicities. Fortunately, previous studies using transgenic mice expressing human CEA in colon epithelium have shown no inflammation in the colon due to CEA-specific CAR-T cell treatmentCitation27,Citation46,Citation47. This is probably because CEA is predominantly expressed in the lumen of the intestine, which reduces the likelihood of CAR T cells interacting with normal colon epithelium. Moreover, CEA-specific CAR-T cells have been well tolerated in clinical trials, and no important toxicities have been reported in colorectal cancer patientsCitation29,Citation48. Despite the promising results of CEA-specific CAR-T cells in preclinical models, there is still room for improvement of this immunotherapy in solid tumors. A key feature of CAR-T cells is that both CD4+ and CD8+ T cell subsets can directly recognize cancer cells, triggering T cell activation that leads to tumor killing. In our setting, CD4+ CAR-T cells showed lower up-regulation of activation markers, cytokine production and expression of cytotoxic molecules, as compared to CD8+ CAR-T cells. These results suggest that a CAR-T cell product composed exclusively of CD8+ T cells would show enhanced antitumor activity. However, CD4+ CAR-T cells may contribute to antitumor immunity by several other mechanisms. Indeed, cumulative evidence indicates that CD4+ CAR-T cells are more resistant to antigen-induced exhaustion and may play a key role in sustaining long-term antitumor responsesCitation49–51. Importantly, recent studies have shown promising results in improving CEA-specific CAR-T cells in preclinical models. Systemic administration of IL−12 increased CAR-T cell persistence and killing potential of several tumor cell linesCitation52. Activating IL−12 receptors by a domain contained within the CAR structure induced an NK cell-like signature on CAR-T cells, enabling them to recognize and eliminate CEA-negative cancer cellsCitation53 and shape the tumor microenvironment by recruiting macrophagesCitation54. In addition, the administration of CEA-targeted IL−2 improves CAR-T cell-mediated eradication of syngeneic tumors in CEA transgenic miceCitation46. Similar strategies may prove effective in improving CAR-T cell-based immunotherapies against GBC. Our work highlights the use of CEA-specific CAR-T cells as a potential immunotherapy for GBC.
Authors’ contributions
EL, SH, ER and JG performed CAR-T related experiments and analyzed the data. ES and CC performed the histological examination of GBC samples. CC and FSO analyzed and interpreted the GBC patient data. CHT, RP and MVG provided key material for carrying out the project. VB and AL supervised the project and interpreted the data. EL, VB and AL wrote the manuscript. All authors read and approved the final manuscript.
Supplemental Material
Download Zip (402.3 KB)Acknowledgments
This work was funded by: Centro Ciencia & Vida, FB210008, Financiamiento Basal para Centros Científicos y Tecnológicos de Excelencia from ANID - Agencia Nacional de Investigación y Desarrollo; Grants 1212070 and 11190897 from FONDECYT - Fondo Nacional de Desarrollo Científico y Tecnológico. Grants ID16I20369 and ID22I10070 from FONDEF - Fondo de Fomento al Desarrollo Científico y Tecnológico.
Disclosure statement
No potential conflict of interest was reported by the author(s).
Data availability statement
The data that support the findings of this study are available from the corresponding author, [AL], upon reasonable request.
Supplementary material
Supplemental data for this article can be accessed online at https://doi.org/10.1080/2162402X.2023.2225291
Additional information
Funding
References
- Hundal R, Shaffer EA, Gallbladder cancer: epidemiology and outcome. Clin Epidemiol. 2014;6:99–12. doi:10.2147/CLEP.S37357.
- Wang YF. Combined detection tumor markers for diagnosis and prognosis of gallbladder cancer. World J Gastroenterol. 2014;20(14):4085–4092. doi:10.3748/wjg.v20.i14.4085.
- Sharma A, Sharma KL, Gupta A, Yadav A, Kumar A. Gallbladder cancer epidemiology, pathogenesis and molecular genetics: recent update. World J Gastroenterol. 2017;23(22):3978–3998. doi:10.3748/wjg.v23.i22.3978.
- Cui X, Zhu S, Tao Z, Deng X, Wang Y, Gao Y, Liao Y, Ma W, Zhang Y, Ma X, et al. Long-term outcomes and prognostic markers in gallbladder cancer. Med. 2018;97(28):e11396. doi:10.1097/MD.0000000000011396.
- Zaidi MY, Maithel SK. Updates on Gallbladder Cancer management. Curr Oncol Rep. 2018;20(2):21. doi:10.1007/s11912-018-0664-3.
- Cherkassky L, Jarnagin W. Selecting treatment sequence for patients with incidental gallbladder cancer: a neoadjuvant approach versus upfront surgery. Updates Surg. 2019;71(2):217–225. doi:10.1007/s13304-019-00670-z.
- Pages F, Berger A, Camus M, Sanchez-Cabo F, Costes A, Molidor R, Mlecnik B, Kirilovsky A, Nilsson M, Damotte D, et al. Effector memory T cells, early metastasis, and survival in colorectal cancer. N Engl J Med. 2005;353(25):2654–2666. doi:10.1056/NEJMoa051424.
- Amedei A, Niccolai E, D’Elios MM, T cells and adoptive immunotherapy: recent developments and future prospects in gastrointestinal oncology. Clin Dev Immunol. 2011;2011:320571. doi:10.1155/2011/320571.
- Blair AB, Murphy A. Immunotherapy as a treatment for biliary tract cancers: a review of approaches with an eye to the future. Curr Probl Cancer. 2018;42(1):49–58. doi:10.1016/j.currproblcancer.2017.10.004.
- Fluxa P, Rojas-Sepúlveda D, Gleisner MA, Tittarelli A, Villegas P, Tapia L, Rivera MT, López MN, Catán F, Uribe M, et al. High CD8(+) and absence of Foxp3(+) T lymphocytes infiltration in gallbladder tumors correlate with prolonged patients survival. Bmc Cancer. 2018;18(1):243. doi:10.1186/s12885-018-4147-6.
- Pandey A, Stawiski EW, Durinck S, Gowda H, Goldstein LD, Barbhuiya MA, Schröder MS, Sreenivasamurthy SK, Kim S-W, Phalke S, et al. Integrated genomic analysis reveals mutated ELF3 as a potential gallbladder cancer vaccine candidate. Nat Commun. 2020;11(1):4225. doi:10.1038/s41467-020-17880-4.
- Yi M, Jiao D, Xu H, Liu Q, Zhao W, Han X, Wu K. Biomarkers for predicting efficacy of PD-1/PD-L1 inhibitors. Mol Cancer. 2018;17(1):129. doi:10.1186/s12943-018-0864-3.
- Sun L, Zhang L, Yu J, Zhang Y, Pang X, Ma C, Shen M, Ruan S, Wasan HS, Qiu S, et al. Clinical efficacy and safety of anti-PD-1/PD-L1 inhibitors for the treatment of advanced or metastatic cancer: a systematic review and meta-analysis. Sci Rep. 2020;10(1):2083. doi:10.1038/s41598-020-58674-4.
- Ahn S, Lee J-C, Shin DW, Kim J, Hwang J-H. High PD-L1 expression is associated with therapeutic response to pembrolizumab in patients with advanced biliary tract cancer. Sci Rep. 2020;10(1):12348. doi:10.1038/s41598-020-69366-4.
- Gou M, Zhang Y, Liu T, Si H, Wang Z, Yan H, Qian N, Dai G, PD-1 Inhibitors could improve the efficacy of chemotherapy as first-line treatment in biliary tract Cancers: a propensity score matching based analysis. Front Oncol. 2021;11:648068. doi:10.3389/fonc.2021.648068.
- Tomita K, Takano K, Shimazu M, Okihara M, Sano T, Chiba N, Kawachi S. Long-term survival of a recurrent gallbladder carcinoma patient with lymph node and peritoneal metastases after multidisciplinary treatments: a case report. Surg Case Rep. 2016;2(1):12. doi:10.1186/s40792-016-0135-8.
- Kawamoto M, Wada Y, Koya N, Takami Y, Saitsu H, Ishizaki N, Tabata M, Onishi H, Nakamura M, Morisaki T, et al. Long-term survival of a patient with recurrent gallbladder carcinoma, treated with chemotherapy, immunotherapy, and surgery: a case report. Surg Case Rep. 2018;4(1):115. doi:10.1186/s40792-018-0512-6.
- Rao J, Xia J, Yang W, Wu C, Sha B, Zheng Q, Cheng F, Lu L. Complete response to immunotherapy combined with an antiangiogenic agent in multiple hepatic metastases after radical surgery for advanced gallbladder cancer: a case report. Ann Transl Med. 2020;8(23):1609. doi:10.21037/atm-20-4420.
- June CH, O’Connor RS, Kawalekar OU, Ghassemi S, Milone MC. CAR T cell immunotherapy for human cancer. Sci. 2018;359(6382):1361–1365. doi:10.1126/science.aar6711.
- Anagnostou T, Riaz IB, Hashmi SK, Murad MH, Kenderian SS. Anti-CD19 chimeric antigen receptor T-cell therapy in acute lymphocytic leukaemia: a systematic review and meta-analysis. The Lancet Haematology. 2020;7(11):e816–e826. doi:10.1016/S2352-3026(20)30277-5.
- Cartellieri M, Bachmann M, Feldmann A, Bippes C, Stamova S, Wehner R, Temme A, Schmitz M, Chimeric antigen receptor-engineered T cells for immunotherapy of cancer. J Biomed Biotechnol. 2010;2010:1–13. doi:10.1155/2010/956304.
- Siegler EL, Wang P. Preclinical models in chimeric antigen receptor-engineered T cell therapy. Hum Gene Ther. 2018.
- Wen Z, Si A, Yang J, Yang P, Yang X, Liu H, Yan X, Li W, Zhang B. Elevation of CA19-9 and CEA is associated with a poor prognosis in patients with resectable gallbladder carcinoma. HPB (Oxford). 2017;19(11):951–956. doi:10.1016/j.hpb.2017.06.011.
- Sinha SR, Prakash P, Singh RK, Sinha DK. Assessment of tumor markers CA 19-9, CEA, CA 125, and CA 242 for the early diagnosis and prognosis prediction of gallbladder cancer. World J Gastrointest Surg. 2022;14(11):1272–1284. doi:10.4240/wjgs.v14.i11.1272.
- Sachan A, Saluja SS, Nekarakanti, PKN, Mahajan B, Nag HH, Mishra, PK. Raised CA19–9 and CEA have prognostic relevance in gallbladder carcinoma. Bmc Cancer. 2020;20(1):826. doi:10.1186/s12885-020-07334-x.
- Burga RA, Thorn M, Point GR, Guha P, Nguyen CT, Licata LA, DeMatteo RP, Ayala A, Joseph Espat N, Junghans RP, et al. Liver myeloid-derived suppressor cells expand in response to liver metastases in mice and inhibit the anti-tumor efficacy of anti-CEA CAR-T. Cancer Immunol Immunother. 2015;64(7):817–829. doi:10.1007/s00262-015-1692-6.
- Wang L, Ma N, Okamoto S, Amaishi Y, Sato E, Seo N, Mineno J, Takesako K, Kato T, Shiku H, et al. Efficient tumor regression by adoptively transferred CEA-specific CAR-T cells associated with symptoms of mild cytokine release syndrome. Oncoimmunol. 2016;5(9):e1211218. doi:10.1080/2162402X.2016.1211218.
- Yoon DH, Osborn M, Tolar J, Kim C. Incorporation of immune checkpoint blockade into chimeric antigen receptor t cells (CAR-Ts): combination or built-in CAR-T. IJMS. 2018;19(2):340. doi:10.3390/ijms19020340.
- Zhang C, Wang Z, Yang Z, Wang M, Li S, Li Y, Zhang R, Xiong Z, Wei Z, Shen J, et al. Phase i escalating-dose trial of CAR-T therapy targeting CEA(+) metastatic colorectal cancers. Mol Ther. 2017;25(5):1248–1258. doi:10.1016/j.ymthe.2017.03.010.
- Katz SC, Hardaway J, Prince E, Guha P, Cunetta M, Moody A, Wang LJ, Armenio V, Espat NJ, Junghans RP, et al. HITM-SIR: phase Ib trial of intraarterial chimeric antigen receptor T-cell therapy and selective internal radiation therapy for CEA(+) liver metastases. Cancer Gene Ther. 2020;27(5):341–355. doi:10.1038/s41417-019-0104-z.
- Tandon N. Generation of stable expression mammalian cell lines using lentivirus. Bio Protoc. 2018;8(21).
- Swift S. Rapid production of retroviruses for efficient gene delivery to mammalian cells using 293T cell-based systems. Curr Protoc Immunol, 2001. Chapter 10: p.Unit 10 17C.
- Carrasco C, Tittarelli A, Paillaleve N, Pozo MD, Rojas-Sepúlveda D, Barría O, Fluxá P, Hott M, Martin C, Quezada C, et al. The evaluation of 17 gastrointestinal tumor markers reveals prognosis value for MUC6. CK17, And CD10 In Gallbladder-Cancer Patients. Diagn (Basel). 2021;11(2):153. doi:10.3390/diagnostics11020153.
- Bosslet K, Lüben G, Schwarz A, Hundt E, Harthus HP, Seiler FR, Muhrer C, Kayser K, Klöppel G, Sedlacek HH. Immunohistochemical localization and molecular characteristics of three monoclonal antibody-defined epitopes detectable on carcinoembryonic antigen (CEA). Int J Cancer. 1985;36(1):75–84. doi:10.1002/ijc.2910360113.
- Hombach AA, Abken H. Costimulation by chimeric antigen receptors revisited the T cell antitumor response benefits from combined CD28-OX40 signalling. Int J Cancer. 2011;129(12):2935–2944. doi:10.1002/ijc.25960.
- Hombach AA, Heiders J, Foppe M, Chmielewski M, Abken H. OX40 costimulation by a chimeric antigen receptor abrogates CD28 and IL-2 induced IL-10 secretion by redirected CD4 + T cells. Oncoimmunol. 2012;1(4):458–466. doi:10.4161/onci.19855.
- Rafiq S, Yeku OO, Jackson HJ, Purdon TJ, van Leeuwen DG, Drakes DJ, Song M, Miele MM, Li Z, Wang P, et al. Targeted delivery of a PD-1-blocking scFv by CAR-T cells enhances anti-tumor efficacy in vivo. Nat Biotechnol. 2018;36(9):847–856. doi:10.1038/nbt.4195.
- Zolov SN, Rietberg SP, Bonifant CL. Programmed cell death protein 1 activation preferentially inhibits CD28.CAR-T cells. Cytotherapy. 2018;20(10):1259–1266. doi:10.1016/j.jcyt.2018.07.005.
- Rojas-Sepulveda D, Tittarelli A, Gleisner MA, Ávalos I, Pereda C, Gallegos I, González FE, López MN, Butte JM, Roa JC, et al. Tumor lysate-based vaccines: on the road to immunotherapy for gallbladder cancer. Cancer Immunol Immunother. 2018;67(12):1897–1910. doi:10.1007/s00262-018-2157-5.
- Mizuno R, Sugiura D, Shimizu K, Maruhashi T, Watada M, Okazaki I-M, Okazaki T, PD-1 primarily targets TCR signal in the inhibition of functional T cell activation. Front Immunol. 2019;10:630. doi:10.3389/fimmu.2019.00630.
- Zhang E, Yang P, Gu J, Wu H, Chi X, Liu C, Wang Y, Xue J, Qi W, Sun Q, et al. Recombination of a dual-CAR-modified T lymphocyte to accurately eliminate pancreatic malignancy. J Hematol Oncol. 2018;11(1):102. doi:10.1186/s13045-018-0646-9.
- Zhang BL, Li D, Gong Y-L, Huang Y, Qin D-Y, Jiang L, Liang X, Yang X, Gou H-F, Wang Y-S, et al. Preclinical evaluation of chimeric antigen receptor–modified T cells specific to epithelial cell adhesion molecule for treating colorectal cancer. Hum Gene Ther. 2019;30(4):402–412. doi:10.1089/hum.2018.229.
- Cai X, Wei B, Li L, Chen X, Liu W, Cui J, Lin Y, Sun Y, Xu Q, Guo W, et al. Apatinib enhanced anti-PD-1 therapy for colon cancer in mice via promoting PD-L1 expression. Int Immunopharmacol. 2020;88:106858. doi:10.1016/j.intimp.2020.106858.
- Neyaz A, Husain N, Kumari S, Gupta S, Shukla S, Arshad S, Anand N, Chaturvedi A. Clinical relevance of PD-L1 expression in gallbladder cancer: a potential target for therapy. Histopathol. 2018;73(4):622–633. doi:10.1111/his.13669.
- Lin J, Long J, Wan X, Chen J, Bai Y, Wang A, Yang X, Wu Y, Robson SC, Sang X, et al. Classification of gallbladder cancer by assessment of CD8(+) TIL and PD-L1 expression. Bmc Cancer. 2018;18(1):766. doi:10.1186/s12885-018-4651-8.
- Cha SE, Kujawski M, J Yazaki P, Brown C, Shively JE. Tumor regression and immunity in combination therapy with anti-CEA chimeric antigen receptor T cells and anti-CEA-IL2 immunocytokine. Oncoimmunol. 2021;10(1):1899469. doi:10.1080/2162402X.2021.1899469.
- Chmielewski M, Hahn O, Rappl G, Nowak M, Schmidt–Wolf IH, Hombach AA, Abken H. T cells that target carcinoembryonic antigen eradicate orthotopic pancreatic carcinomas without inducing autoimmune colitis in mice. Gastroenterol. 2012;143(4):1095–107 e2. doi:10.1053/j.gastro.2012.06.037.
- Patel U, Abernathy J, Savani BN, Oluwole O, Sengsayadeth S, Dholaria B. CAR-T cell therapy in solid tumors: a review of current clinical trials. E J Haem. 2022;3(Suppl 1):24–31. doi:10.1002/jha2.356.
- Wang D, Aguilar B, Starr R, Alizadeh D, Brito A, Sarkissian A, Ostberg JR, Forman SJ, Brown CE. Glioblastoma-targeted CD4+ CAR-T cells mediate superior antitumor activity. JCI Insight. 2018;3(10). doi:10.1172/jci.insight.99048.
- Li T, Wu B, Yang T, Zhang L, Jin K. The outstanding antitumor capacity of CD4(+) T helper lymphocytes. Biochim Biophys Acta Rev Cancer. 2020;1874(2):188439. doi:10.1016/j.bbcan.2020.188439.
- Boulch M, Cazaux M, Loe-Mie Y, Thibaut R, Corre B, Lemaître F, Grandjean CL, Garcia Z, Bousso P. A cross-talk between CAR-T cell subsets and the tumor microenvironment is essential for sustained cytotoxic activity. Sci Immunol. 2021;6(57). doi:10.1126/sciimmunol.abd4344.
- Chi X, Yang P, Zhang E, Gu J, Xu H, Li M, Gao X, Li X, Zhang Y, Xu H, et al. Significantly increased anti-tumor activity of carcinoembryonic antigen-specific chimeric antigen receptor T cells in combination with recombinant human IL-12. Cancer Med. 2019;8(10):4753–4765. doi:10.1002/cam4.2361.
- Hombach A, Barden M, Hannappel L, Chmielewski M, Rappl G, Sachinidis A, Abken H. IL12 integrated into the CAR exodomain converts CD8+ T cells to poly-functional NK-like cells with superior killing of antigen-loss tumors. Mol Ther. 2022;30(2):593–605. doi:10.1016/j.ymthe.2021.10.011.
- Chmielewski M, Kopecky C, Hombach AA, Abken H. IL-12 release by engineered T cells expressing chimeric antigen receptors can effectively Muster an antigen-independent macrophage response on tumor cells that have shut down tumor antigen expression. Cancer Res. 2011;71(17):5697–5706. doi:10.1158/0008-5472.CAN-11-0103.