Abstract
A new calix[Citation4]pyrrole-based macrocycle, meso-tetramethyltetrakis-[4-(2-ethoxy)ethoxyphenyl]calix[Citation4]pyrrole, (2) has been prepared and characterized. The binding ability of (2) for metal cations were investigated by ultraviolet–visible spectrophotometry in acetonitrile at 298.15 K. Stability constant and thermodynamic parameters for 1:1 binding interaction between (2) and Pb(II) cation were measured at different temperatures. Ether calix[Citation4]pyrrole polymer, (4) was also synthesized. Solid-phase extraction of Pb(II) cation by (4) from the aqueous media has been studied using the batch method. Various significant extraction parameters (mass, lead concentration, temperature, and time) have been evaluated. Analysis of equilibrium data shows linear fitting with the Langmuir isotherm. Uptake capacity was found to be 80 mg g−1 at 298.15 K. Kinetic studies show a pseudo-second-order reaction mechanism. The thermodynamic parameters of extractions were also calculated.
Introduction
Toxic metal contamination results from scientific and technological progress is threatening human health with serious problems [Citation1–3]. Heavy metal ions such as Pb(II), Cd(II), Hg(II), and Ni(II) are toxic and carcinogenic even at relatively low concentrations [Citation4,Citation5]. Heavy metals are generally discharged to the environments via automobile emissions, mining activities, battery industry, fossil fuels [Citation6], metal plating and electronic industries with diverse routes [Citation7]. In order to remove heavy metal ions from various environments, the techniques such as precipitation, adsorption, ion exchange [Citation8], reverse osmosis, electrochemical treatments [Citation9], hyper filtration [Citation10], membrane separation, evaporation, coagulation, flotation [Citation11] are widely used. The material selectivity and cost expenses are the most controlling factors in water treatment.
Calix[Citation4]pyrrole belong to an area in chemistry known as supramolecules [Citation12,Citation13]. The key features in this field of chemistry involve recognition, where the host shows selectivity toward a targeted substrate, and chemical transformation in the nature of the guest upon interaction with host [Citation13–15]. These characteristics give calix[Citation4]pyrrole preference to complex with different anions through its –NH groups [Citation16]. Lately, modifications was carried out on the meso position of calix[Citation4]pyrrole produce new derivatives capable of complexation with metal cations [Citation17,Citation18].
In this article, we report the synthesis and characterization of a calix[Citation4]pyrrole derivative, namely, meso-tetramethyltetrakis-[4-(2-ethoxy)ethoxyphenyl]calix[Citation4] pyrrole, 2. Its interactions with heavy metals [Pb(II), Hg(II) and Cd(II)] were investigated. Polymerization of 2 was also achieved to improve its capacity for removal of Pb(II) cations from water.
Experimental
Chemicals: All materials, reagents and solvents used were of analytical grade or highest purity available and used without further purification. Pyrrole (>99%), para-hydroxyacetophenone (>98%), 2-chlorodiethyl ether (99%), ethylenediamine tetraacetic acid (98%), formic acid, methane sulfonic acid, potassium carbonate (98%), 18-crown-6 (99%), sodium hydroxide (98%) were all purchased from Aldrich Chemical Co. The solvents, acetonitrile, formaldehyde (37%), dichloromethane, diethyl ether, methanol, hexane, ethanol and tetrahydrofuran (THF), were all purchased from Aldrich Chemical Co. Lead nitrate (98%) (used for extraction studies in water) and perchlorates of lead, cadmium and mercury (99.9%) (used for complexation studies in acetonitrile) were purchased from Aldrich Chemical Co. Deuterated chololform (d3-CDCl3), and dimethylsulfoxide (d6-DMSO) were purchased from Aldrich Chemical Co.
Equipments: Absorbance spectra measurements were made using computerized double beam ultraviolet (UV)–Vis Spectrophotometer (Jasco V-530, spectral band width 2 nm), and matched quartz cell with length 10 mm. Heavy metal cation concentration was determined using the atomic absorption spectrophotometer Bruker Scientific. Shaking was conducted with a rotary Shaker Model named Wiggen Hausher OS −150 at a speed 180 rpm. 1H nuclear magnetic resonance (NMR) experiment was recorded at 298 K on a Bruker Ac-300 MHz NMR spectrometer. Typical operation conditions for routine proton measurements involved “pulse” or flip angle of 30°, spectral frequency of 300.135 MHz, delay time of 1.60 s, acquisition time of 1.819 s, and line broadening of 0.55 Hz. The sample solution in the appropriate deuterated solvent were placed in an NMR tube of 5 mm, using tetramethylsilane (TMS) as the internal reference to measure the spectrum of ligand; shifts were reported in ppm versus TMS. Elemental analysis of C, H, and N was performed at the Elemental Analysis Lab – University of Surrey, Guildford, Surrey, UK. Thermogravimetric analysis (TGA) and differential thermal analysis (DTA) or differential scanning calorimetry (DSC) curves were recorded on SETARAM-LABSYS Thermal analyzer in the flow of N2 within 20–700°C temperature range, with a heating rate of 3°C min−1.
Synthesis
Synthesis of meso-tetramethyltetrakis-[4-hydroxyphenyl] calix[Citation4]pyrrole, 1: This ligand was prepared by following a previously reported procedure [Citation19].
Synthesis of meso-tetramethyltetrakis-[4-(2-ethoxy) ethoxyphenyl]calix[Citation4]pyrrole, 2: To a stirring solution of 1 (2.60 g, 3.51 mmol) dissolved in acetonitrile (150 mL), both potassium carbonate (1.94 g, 14.05 mmol) and 18-crown-6 (0.93 g, 3.51 mmol) were added and the solution was left to stir under reflux for 2 h. A solution of 2-chlorodiethyl ether (3.05 mL, 28.10 mmol) was then added and the reaction mixture was left under reflux for 48 h with continuous stirring. The reaction mixture was monitored by a thin-layer chromatography, using dichloromethane/hexane/methanol (8/1.4/0.6 mL) as a developing solvent. The obtained brownish solution was collected and the solvent was removed by rotary evaporation. The formed solid was dried under vacuum at 90°C with 17% yield. This compound was characterized using 1H-NMR, Fourier transform infra red (FTIR) and elemental analysis.
Synthesis of meso-tetramethyltetrakis-[4-hydroxyphenyl] calix[Citation4]pyrrole polymer, 3: To a stirring solution of tetramethyltetrakis-[4-hydoxyphenyl]calix[Citation4]pyrrole, 1, (2.5 g, 3.38 mmol, 740 g.mol−1) in THF (50 mL), a mixture of formaldehyde (37%) (54.05 mmol, 4.08 mL), formic acid (2 mL) and methane sulfonic acid (1 mL) was added gradually over a period of 5 min. The reaction was stirred for 2 h at room temperature and then filtered gravitationally. The precipitate was collected and washed with 20 mL of THF, 30 mL of acetonitrile and 100 mL water. The obtained solid was dried under vacuum giving 4.811 g of 3. This polymer was characterized by FTIR and thermal analysis.
Synthesis of meso-tetramethyltetrakis-[4-(2-ethoxy) ethoxyphenyl]calix[Citation4]pyrrole polymer, 4: To a stirring solution of 3 (2.41 g, 3.26 mmol) dissolved in acetonitrile (150 mL) both potassium carbonate (1.80 g, 13.04 mmol) and 18-crown-6 (0.86 g, 3.26 mmol) were added and the solution was left stirring under reflux for 2 h. Then 2-chlorodiethyl ether (2.83 mL, 26.09 mmol) was added and the reaction mixture was left under reflux for 48 h with continuous stirring. The obtained residue was filtered off, collected, washed with acetonitrile and left under a vacuum at 90°C yielding 2 g of 4. The obtained polymer was characterized using FTIR and thermal analysis.
Stability constant measurements
Spectrophotometric measurements: Mole ratio method has been used to determine the stoichiometry of complexation between heavy metals (Pb2+, Cd2+ and Hg2+ as perchlorates) and host ligand 2 [Citation20]. In this method, the solution absorbance was measured upon the addition of incremental quantities of metal ion of known concentration (10−4 mol.dm−3) to a fixed volume of 2 of known concentration (10−4 mol.dm−3). The measurements were taken at wavelength equivalent to 240 nm.
Thermodynamic study: UV titration experiments in acetonitrile was carried out with Jasco UV – visible at different temperatures (298–318 K) in order to determine the stability constant [Citation21–23] of complexation of 2 with lead (II) cation. This titration was carried out through mixing an equivalent amount of cation salt (10−4 mol.dm−3) and 2 (10−4 mol.dm−3) in the same solution of acetonitrile. The absorbance of the solutions was recorded after equilibrium was attained. The data were collected and processed to calculate the complex formation constant (Kf) of ML complex at each temperature respectively. Thermodynamic parameters were determined using the Van't Hoff method by plotting ln Kf versus 1/T.
Adsorption studies of lead ions by polymer 4
Kinetic study for lead removal: Kinetic studies for lead uptake using polymer 4 were performed under the same conditions (neutral pH and optimum mass). Based on optimum mass studies, 0.1 g of 4 was weighted and added to a series of volumetric flasks (100 mL). To each flask the same amount of lead (II) solution (200 ppm) was added. These flasks were shaken mechanically for different time intervals (15–180 min) and then the phases were separated and the concentration of the lead (II) cation remained in the solution was determined.
Temperature effect on lead removal: To investigate the temperature effect on the extraction ability of 4, a fixed amount of solid (0.1 g) was equilibrated with a volume (50 mL) of lead (II) solution (200 ppm) at different temperatures ranging from 25 to 45°C under the same conditions (time, shaking, pH). The phases were then separated and the concentrations of lead (II) solutions were determined.
Concentration effect on the lead removal: The affinity of 4 to lead (II) cation was determined under static conditions. 0.1 g of solid was added to a volume (50 mL) of lead (II) solution of different concentration (100 ppm–800 ppm) and the mixtures were mixed and shaken mechanically at 25°C for 24 h to attain equilibrium. After equilibrium was attained, the phases were separated and the solutions were analyzed to determine the concentration of the remaining lead (II) ions concentrations.
Results and discussion
Characterization of materials
Proton NMR (1H-NMR)
meso-tetramethyltetrakis-[4-(2-ethoxy)ethoxyphenyl]calix[Citation4]pyrrole (2): (d6-DMSO, δ in ppm): 9.37 (bs, 4H, NH), 6.89 (d, 8H, ArH), 6.72 (d, 8H, ArH), 5.94 (d, 8H, pyrrole–H), 3.62 (t, 8H, CH2–O–phenyl), 3.62 (t, 8H, CH2–O–CH2CH3), 3.55 (q, 8H, O–CH2–CH3) 1.75 (s, 12H, CH3–bridge), 1.15(t, 12H, CH3–CH2–O). (d3-CDCl3, δ in ppm): 7.81 (bs, 4H, NH), 6.83 (d, 8H, ArH), 6.70 (d, 8H, ArH), 5.92 (d, 8H, pyrrole–H), 4.14 (t, 8H, CH2–O–phenyl), 3.79 (t, 8H, CH2–O–CH2CH3), 3.72 (q, 8H, O–CH2–CH3) 1.91 (s, 12H, CH3–bridge), 1.13(t, 12H, CH3–CH2–O).
Elemental analysis
The elemental analysis data () is consistent with the proposed structure of the compounds 1 and 2.
FTIR analysis
FTIR analysis was employed to determine specific functionality of the prepared ligands and polymers. The characteristic absorption bands of these materials and their assignments are displayed in . The results indicate that the stretching band (3400 cm−1) due to in 1 was shifted to a higher frequency (3420 cm−1) in 2. This may be attributed to the introduction of pendent arm in 2. The appearance of an additional band (1120–1125 cm−1) in FTIR spectrum of 2 suggests the modification of ligand 1 by a successful connection of diethyl ether pendent arm to this ligand at hydroxyl group of phenol rings. The disappearance of the stretching band of OH groups from FTIR spectrum of 2 also confirms that the substitution reaction at hydroxyl group was effectively performed. Similarly, the appearance of a new band at 1100 cm−1 and disappearance of OH stretching band from a spectrum of 4 as compared to 3 suggests the modification of 3 by the attachment of the diethyl ether group to its structure to produce polymer 4. Therefore, the spectral results are consistent with the proposed structures for materials 1, 2, 3 and 4.
Table 2. Fundamental IR spectral data of calix[Citation4]pyrrole compounds (1, 2, 3 and 4).
Thermal analysis
The results of TGA for calix[Citation4]pyrrole compounds are shown in and . The data are listed in . The compounds were heated to 700–800°C. All the relevant weight losses were completed by 700°C. The TGA-DTA/DSC curves show that all compounds are stable up to 100°C unless if water molecules are present which is associated with an endothermic heat change.
Figure 1. TGA-DSC of meso-tetramethyltetrakis-[4-hydroxyphenyl]calix[Citation4]-pyrrole (1).
![Figure 1. TGA-DSC of meso-tetramethyltetrakis-[4-hydroxyphenyl]calix[Citation4]-pyrrole (1).](/cms/asset/94474d46-3fde-4383-b7c0-cfc0c56ac572/tcme_a_884940_f0001_c.jpg)
Figure 2. TGA/differential thermogravimetry-DTA of meso-tetramenthyltetrakis-[4-(2-ethoxy)ethoxyphenyl] calix[Citation4]pyrrole polymer (4).
![Figure 2. TGA/differential thermogravimetry-DTA of meso-tetramenthyltetrakis-[4-(2-ethoxy)ethoxyphenyl] calix[Citation4]pyrrole polymer (4).](/cms/asset/8125044d-54d0-460a-ac66-b81bf2215aa8/tcme_a_884940_f0002_c.jpg)
Table 3. Thermal analysis data, TG, DSC/DTA for the compounds (1, 3 and 4).
The results of TGA for meso-tetramethyltetrakis-[4-hydroxyphenyl]-calix[Citation4]pyrrole (1), including % mass loss and enthalpy changes (J g−1) at each temperature peak (°C) is shown in and presented in . The % weight of the obtained black ash residue is 12.37%. The major % mass loss for 1 occurs at temperature 292.34°C. This loss corresponds to the degradation followed by the decomposition of calix[Citation4]pyrrole units (C28H36N4) since the fragmentation temperature is close to the its melting point (295–298°C).
The results of TGA of meso-tetramenthyltetrakis-[4-hydroxyphenyl]-calix[Citation4]pyrrole polymer 3 is presented in . The % weight of residue obtained is 24.091% of black carbonaceous materials. The major % mass loss for 3 occurs at temperature 472.61°C. The difference in the mass loss as a function of temperature indicates that the polymerization of 1 occurred, producing polymer 3 with high temperature resistance since a graduated loss of mass occurred between 150 and 700°C without a sharp peak.
The results of TGA for meso-tetramenthyltetrakis-[4-(2-ethoxy)-ethoxyphenyl]calix[Citation4]pyrrole polymer 4, including % mass loss and enthalpy changes (J g−1) at each temperature peak (°C) is shown in and presented in . The presence of diethylether pendent arm in polymer 4 increases its temperature resistance since the major loss occurred at 385.0°C compared to 292.34°C occurring in 1.
Complexation studies
Formation constant
As far as lead (II) cation is concerned, the plot of complex absorbance at 240 nm versus the mole ratio (mmole of Pb2+/mmole of (2)) gives ML complex (1:1 ratio) (). The increase in the absorbance of solution is related to the increase in the amount of complex formed. After that the absorbance remains constant, meaning that all the amount of 2 was used in the formation of the metal complex. In case of cadmium (II) cation or mercury (II) cation, the plot of absorbance versus the mole ratio (Cd2+/(2)) or (Hg2+/(2)) ( and ); shows a very slight change in the measured absorbance of 2, suggesting the absence of interaction between the guest (Cadmium (II) or Mercury (II) cation) and ligand 2. Therefore, ligand 2 contains oxygen donor atoms in its pendent arms, a hard donor atom, and as such, able to interact with hard metal cations (Mg, Ca, Sr) and to some extent with intermediate metals such as lead, while soft metals such as cadmium and mercury are less expected to interact with hard atoms such as oxygen.
Thermodynamic of complexation
Thermodynamic parameters of interaction between lead (II) cation and calixpyrrole derivative 2, in acetonitrile were obtained using equations (1)–(3) and the complex formation constant (Kf) values at different temperatures ().
Table 4. Values of Kf for complexation of Pb2+ with (2) obtained at different temperatures in acetonitrile.
Van't Hoff method was applied to determine enthalpy (Δ Hc, kJ mol−1) and entropy (Δ Sc, J mol−1 K−1) of complexation by plotting ln Kf versus 1/T. The results are displayed in and .
Table 5. Thermodynamic parameters for complexation of Pb2+ with (2) in acetonitrile.
Figure 6. Plot of the variation in Kf for complexation of Pb2+ with (2) as a function of (1/T) in acetonitrile.
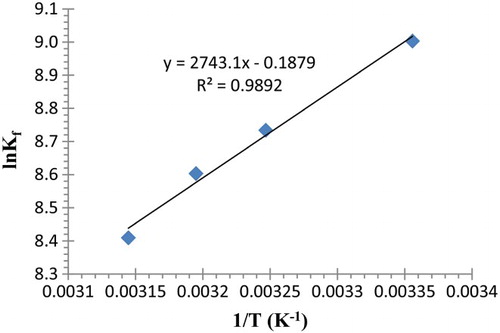
Comparison of complex stability constant at different temperature show that this values decreases with increasing temperature. The negative value of Δ G indicates that the formation of the ML complex in all cases is thermodynamically favored “Spontaneous” while the negative values of Δ H indicates that these processes are exothermic and favorable at low temperature. As a result, the high values of Δ H and negative values of Δ S indicate that the complex formation is enthalpically controlled and entropically unfavored.
Adsorption studies of lead ion by polymer 4
The extraction ability of polymer 4 for lead (II) cation from aqueous solution has been studied by using batch method. The amount of lead ion extracted (%E) was calculated according to the following equation;
Shaking time
The influence of shaking time on adsorption percentage of metal ion using 4 was studied. Samples were shaken for different time intervals (15–240 min). The outcome of this study is shown in where the % adsorption was plotted against time (min). As can be seen from , % adsorption increases with increasing shaking time and equilibrium was attained after 180 min. This equilibrium occurs when the receptor sites of the polymer (active sites) were covered and saturated by the Pb(II) ion.
Adsorption kinetics
As the adsorption rate is dependent on the rate at which the metal ion was transferred from bulk liquid phase to the adsorption sites, the adsorption mechanism was studied using different expressions. Lagergren first-order kinetic expression (equation (5)) was used to show the uptake rate of different materials [Citation24,Citation25]. The equation is defined as:
Where Qt was determined based on the following equation:
Another equation that explains the adsorption mechanism is known as the pseudo-second-order (equation (7)). The pseudo-second-order kinetic model is used as a presentation of chemisorption behavior of the reaction [Citation26–28].
The equation is defined as:
The rate constant and correlation values of the first-order model and the pseudo-second-order at 298.15 K are presented in and , respectively. The values of rate parameters and constants for different kinetic models at 298.15 K are shown in . From these data, the extraction process did not comply well with first-order model for lead (II) cation uptake while a better linear fitting with pseudo-second-order was obtained. Also, the obtained Qmax value in case of the second order is closer to Qmax value obtained at infinite time. Therefore, the extraction of lead ion from water in this study can be described as the chemisorptions process.
Table 6. Pseudo-first-order and second-order constants for lead (II) cation uptake at 298.15 K (cal.=calculated; exp.=experimental).
Effect of temperature
The adsorption dependence on temperature (298–313 K) is presented in . Extraction ability of polymer 4 increases with increasing temperature indicating that the adsorption process is endothermic. This can be attributed to the mode of interaction between the active sites of polymer 4 and lead (II) cation in addition to the ability of the ion to transfer from liquid phase to solid phase.
Adsorption thermodynamics
The standard enthalpy change and standard entropy change
were calculated by plotting ln K versus 1/T (), equations (1) and (3). Gibbs free energy (Δ G) of adsorption was calculated from equation (2), where K is the thermodynamic equilibrium adsorption constants. The results are shown in . An inspection of the results indicates that the adsorption process is endothermic. Moreover, the negative values of the Gibbs free energy for the uptake of Pb(II) cations by polymer 4 suggest that the process is spontaneous. Positive values of
indicate that extraction is dependent on the mobility of the metal ions and reflect the affinity of 4 toward metal ions in aqueous solution [Citation29,Citation30].
Table 7. Thermodynamic parameters for lead (II) cation uptake using 4 at different temperatures.
Adsorption isotherm
The interaction between the adsorbent and adsorbate is explained by three adsorption isotherms (Freundlich, Langmuir and Temkin). These models were also used to verify the uptake performance of polymer 4 [Citation2,Citation3].
Freundlich model is based on a monolayer uptake by the material with a heterogeneous energy distribution of active sites and is described in equation (8) [Citation31]:
The results are shown in . The 1/n value obtained in this study (0.1<1/n<1.0) indicates that this material can be used effectively for the removal of the lead ion from aqueous solution. The Freundlich isotherm constants obtained from slope and intercept are included in .
Table 8. Langmuir, Freundlich and Temkin isotherm constants for the uptake of lead (II) cation at 298.15 K.
Langmuir isotherm is probably the most widely applied isotherm. The basic assumption of the Langmuir theory is that the uptake process takes place at specific homogeneous sites within the material [Citation32]. This isotherm was developed by assuming that a fix number of accessible sites are available on the polymer surface (equation (10)) [Citation33].
Results are shown in and isotherm constants calculated from slope and intercept are presented in .
Inspection of and shows that Langmuir isotherm has a better linearity (R2=0.996) than Freundlich isotherm (R2=0.973). The result suggests that the uptake of lead cation by polymer 4 from aqueous media mainly occur through the accessible sites of the material.
Langmuir model can be used to assess whether the uptake process is favorable or not. To determine the characteristic behavior of this process, dimensionless equilibrium parameter (RL) is used as indicated in equation (12) [Citation34].
Table 9. RL Langmuir values for the uptake of lead (II) cation at 298.15 K.
Temkin is the isotherm model [Citation35,Citation36] assumes that the heat of adsorption process decreases linearly with the increase in coverage of the adsorbent surface, and that adsorption is characterized by a uniform distribution of binding energies up to a maximum binding energy [Citation37]. Temkin isotherm is described by equation (13).
Comparison with other sorbent materials
Although direct comparison of 4 with other materials is difficult, owing to the different applied experimental conditions, material structure and lack of similar applications, it was found, in general, that the removal capacity of 4 for lead cation 80 mg g−1 is comparable with that of other materials [Citation38–43] and in fact greater than certain adsorbents (). Moreover, the nature of the functional groups presented on the surface of this material plays an important role in determining its selectivity. The amount of lead ion removed per unit mass by various adsorbents (in mg g−1) is presented in .
Table 10. Calix[Citation4]pyrrole uptake capacity of lead in comparison with other materials.
Conclusion
A new calix[Citation4]pyrrole-based macrocycle 2 was synthesized and fully characterized using elemental analysis, FTIR and 1H-NMR. Complexation studies of this compound 2 with heavy metals such as Pb2+, Cd2+ and Hg2+ was studied using spectrophotometric techniques. This compound forms 1: 1 complex with Pb2+ in acetonitrile. This selectivity may be attributed to presence of electron donor oxygen atoms in the pendent arm of 2 which has high affinity to lead ions. Thermodynamic investigations for complexation of lead cation with 2 in acetonitrile shows that the stability constant for complexation decreases with increasing temperature and the formation of ML complexes is exothermic and enthalpically favored.
The synthesis of a polymer 4 was performed through the condensation reaction and it was characterized through FTIR and thermal analysis. The uptake of Pb(II) cations by 4 in water was influenced by experimental parameters. Studies show that favorable uptake of lead cation by 4 . Langmuir adsorption capacity of 4 was found to be 80 mg g−1 within 3 h as optimum time. Kinetic studies show a linear fitting with pseudo-second-order, indicating that the process has a chemisorptions behavior. Thermodynamic studies of adsorption show that the uptake of this cation is endothermic in nature. These findings show that polymer 4 can have great potential for removal of heavy metals from polluted water.
Acknowledgements
The authors gratefully acknowledge the help of Prof. Angela Danil de Namor at University of Surrey, UK.
REFERENCES
- S.H. Jang, G.Y. Jeonga, B.G. Mina, W.S. Lyoob, S.C. Lee. J. Hazard. Mater., 159, 294 (2008). doi: 10.1016/j.jhazmat.2008.02.018
- L. Zhou, Y. Wang, Z. Liu, Q. Huang. J. Hazard. Mater., 161, 995 (2009). doi: 10.1016/j.jhazmat.2008.04.078
- Y.T. Zhou, H.L. Nie, C.B. White, Z.Y. He, L.M. Zhu. J. Colloid Interface Sci., 330, 29 (2009). doi: 10.1016/j.jcis.2008.10.026
- C. Liu, R. Bai, Q.S. Ly. Water Res., 42, 1511 (2008). doi: 10.1016/j.watres.2007.10.031
- F. Iemma, G. Crillo, U.G. Sipizzirri, F. Puoci, O.I. Parisi, N. Picci. Eur. Polym. J., 44, 1183 (2008). doi: 10.1016/j.eurpolymj.2008.01.024
- M.R. Guilherme, A.V. Reis, A.T. Paulino, A.R. Fajardo, E.C. Muniz, E.B. Tambourgi. J. Appl. Polym. Sci., 105, 2903 (2007). doi: 10.1002/app.26287
- A. Denizli, B. Garipcan, A. Karabakan, H. Senoz. Mater. Sci. Eng. C, 25, 448 (2005). doi: 10.1016/j.msec.2004.12.001
- H. Zhao, H. Mitomo. J. Appl. Polym. Sci., 110, 1388 (2008). doi: 10.1002/app.28718
- S.H. Jang, G.Y. Jeonga, B.G. Mina, W.S. Lyoob, S.C. Lee. J. Hazard. Mater., 159, 294 (2008). doi: 10.1016/j.jhazmat.2008.02.018
- S. Chen, Y. Zou, Z. Yan, W. Shen, S. Shi, X. Zhang, H. Wang. J. Hazard. Mater., 161, 1355 (2009). doi: 10.1016/j.jhazmat.2008.04.098
- R. Akkaya, U. Ulusoy. J. Hazard. Mater., 151, 380 (2008). doi: 10.1016/j.jhazmat.2007.05.084
- J.M. Lehn. Science, 260, 1762 (1993). doi: 10.1126/science.8511582
- J.M. Lehn. Supramolecular Chemistry, VCH, New York (1995).
- J.-M. Lehn, J.L. Atwood, J.E.D. Davies, D.D. MacNicol, D.D. MacNicol, F. Vögtle. Comprehensive Supramolecular Chemistry, Vols. 1–11, Pergamon/Elsevier, Oxford (1996).
- J.W. Steed, J.L. Atwood. Supramolecular Chemistry, 2nd Edn, p. 1002, Wiley, Chichester (2009).
- A.F. Danil de Namor, M. Shehab, I. Abbas, M.V. Withams, J. Zvietcovich-Guerra. J. Phys. Chem. B, 110, 12653 (2006). doi: 10.1021/jp060859o
- I. Abbas, J. Chaaban. J. Supramol. Chem., 24, 213 (2012). doi: 10.1080/10610278.2011.643795
- A.F. Danil de Namor, I. Abbas, H. Hammud. J. Phys. Chem. B, 111, 3098 (2007). doi: 10.1021/jp067798e
- P. AnzenbacherJr., K. Jursikova, V.M. Lynch, P.A. Gale, J.L. Sessler. J. Am. Chem. Soc., 121, 11020 (1999). doi: 10.1021/ja993195n
- D.A. Skoog. Fundamentals of Analytical Chemistry, 8th Edn., p. 1176, Thomson-Brooks/Cole, Belmont, CA (2004).
- M.S. Masoud, H.H. Hammud, H. Beidas. Thermochim. Acta, 381, 119 (2001). doi: 10.1016/S0040-6031(01)00691-8
- S.A. Tirmizi, M.H.S. Wattoo, S. Sarwar, F.H. Wattoo, A.B. Ghanghro, J. Iqbal. Arab. J. Sci. Eng., 35, 93 (2010).
- A. Ashnagar, N.G. Naseri, B. Khanaki. E-J. Chem., 4, 550 (2007). doi: 10.1155/2007/502718
- S. Lagergren. Handlingar, 24, 1 (1898).
- Y.S. Ho. Scientometrics, 59, 171 (2004). doi: 10.1023/B:SCIE.0000013305.99473.cf
- Y.S. Ho, G. McKay. Process Biochem., 34, 451 (1999). doi: 10.1016/S0032-9592(98)00112-5
- M.C. Ncibi, B. Mahjoub, M. Seffen. J. Hazard. Mat., 139, 280 (2007). doi: 10.1016/j.jhazmat.2006.06.029
- K. Marungrueng, P. Pavasant. J. Environ. Man., 78, 268 (2006). doi: 10.1016/j.jenvman.2005.04.022
- H.S. Altundogan, S. Altundogan, F. Tumen, M. Bildik. Waste Manage., 20, 761 (2000). doi: 10.1016/S0956-053X(00)00031-3
- H. Genc-Fuhrman, J.C. Tjell, D. Mcconchie. Environ. Sci. Technol., 38, 428 (2004). doi: 10.1021/es035207h
- H.M.F. Freundlich. Zeitschrift fur Physikalische Chemie., 57, 385 (1907).
- I. Langmuir. J. Am. Chem. Soc., 38, 2221 (1916). doi: 10.1021/ja02268a002
- H. Schonherr, G.J. Vansco, B.H. Huisman, F.C.J. Veggel, N. Reinhoudt. Langumir, 13, 1567 (1997). doi: 10.1021/la961084l
- T.W. Webi, R.K. Chakkravorti. AlChE J., 20, 228 (1974). doi: 10.1002/aic.690200204
- M.I. Temkin. Zh. Fiz. Chim., 15, 296 (1941).
- H. Hammud, L. Fayoumi, H. Holail. Int. J. Chem., 3, 147 (2011). doi: 10.5539/ijc.v3n4p147
- N.A. Oladoja, C.O. Aboluwoye, Y.B. Oladimeji. Turkish J. Eng. Environ., 32, 303 (2008).
- K.G. Bhattacharyya, S.S. Gupta. Adsorption Sci. Technol., 27, 47 (2009). doi: 10.1260/026361709788921614
- H. Faghihian, M. Nejati-Yazdinejad. Adsorption Sci. Technol., 27, 107 (2009). doi: 10.1260/026361709788921588
- P. Salehi, B. Asghari, F. Mohammadi. J. Water Resour. Prot., 2, 701 (2010). doi: 10.4236/jwarp.2010.28080
- A. Wilke, R. Buchholz, G. Bunke. Environ. Biotechnol., 2, 47 (2006).
- E. Romera, F. Gonzalez, A. Ballester, M.L. Blazquez, J.A. Munoz. Bioresour. Technol., 98, 3344 (2007). doi: 10.1016/j.biortech.2006.09.026
- K.B. Payne, T.M. Abdel-Fattah. J. Environ. Sci. Health A Tox. Hazard. Subst. Environ. Eng., 39, 2275 (2004). doi: 10.1081/ESE-200026265